ABSTRACT
Objectives
Despite the current pneumococcal vaccination program in England for older adults and adults with underlying conditions, disease burden remains high. We evaluated cost-effectiveness of 20-valent pneumococcal conjugate vaccine (PCV20) compared to current pneumococcal recommendations for adults in England.
Methods
Lifetime outcomes/costs of invasive pneumococcal disease (IPD) and community-acquired pneumonia (CAP) among adults aged 65–99 years and adults aged 18–64 years with underlying conditions in England were projected using a deterministic cohort model. Vaccination with PCV20 was compared with 23-valent pneumococcal polysaccharide vaccine (PPV23) from the National Health Service perspective.
Results
PCV20 was cost saving compared with PPV23 in base case and most sensitivity analyses. In the base case, replacing PPV23 with PCV20 prevented 7,789 and 140,046 cases of IPD and hospitalized CAP, respectively, and 22,199 associated deaths, resulting in incremental gain of 91,375 quality-adjusted life-years (QALYs) and incremental savings of £160M. In probabilistic sensitivity analyses, PCV20 (vs. PPV23) was cost saving in 85% of simulations; incremental cost per QALY was below £30,000 in 99% of simulations.
Conclusions
PCV20 vaccination in adults aged 65–99 years and those aged 18–64 years with underlying comorbidities in England is expected to prevent more hospitalizations, save more lives, and yield lower overall costs than current recommendations for PPV23.
1. Introduction
Streptococcus pneumoniae (pneumococcus) causes significant morbidity and mortality in both children and adults in the United Kingdom (UK) [Citation1]. Invasive pneumococcal disease (IPD) is the most serious manifestation causing severe illness with a high risk of mortality [Citation2–4]. Community-acquired pneumonia (CAP), however, is far more common and associated with significant morbidity and mortality [Citation5–8]. Between 2010 and 2017, there were more than 4,000 cases of IPD in England and Wales annually [Citation3]. Estimates of the burden of hospitalized CAP vary considerably, ranging from 80,000 to half a million cases, and a recent study of UK adults found 37% of persons hospitalized with CAP between 2013 and 2018 had pneumococcal pneumonia [Citation5,Citation6,Citation9].
To reduce the risk of pneumococcal infection in the UK, routine vaccination is currently recommended by the Joint Committee on Vaccination and Immunisation (JCVI) for infants, at-risk children and adults (i.e. aged ≥2 years), and all older adults (i.e. aged ≥65 years) [Citation10]. Two vaccines are currently available for use in the UK: a 23-valent pneumococcal polysaccharide vaccine (PPV23) manufactured by Merck Sharpe & Dohme (MSD), for which evidence suggests protection against vaccine-type IPD (VT-IPD) only [Citation11–26], and Pfizer’s 13-valent pneumococcal conjugate vaccine (PCV13), which has been shown to protect against VT-IPD and vaccine-type CAP (VT-CAP) [Citation27].
PPV23 has been recommended for all persons aged ≥2 years with underlying conditions (e.g. chronic comorbidities and immunosuppressive conditions) since 1992 and for all older adults since 2003 [Citation28]. Although PPV23 coverage is high (65% among adults aged ≥65 years), surveillance data suggest that its use has provided only low to moderate short-term direct protection against IPD and has not had a population-level impact on IPD burden given that serotypes unique to PPV23 continue to account for a large proportion of IPD among older adults [Citation2,Citation29]. Immunization of children aged <2 years with PCVs – including 7-valent PCV (PCV7) from 2006–2009 and PCV13 from 2010 to present – has prevented a significant number of cases of pneumococcal disease and associated deaths through the direct protection of young children and indirect protection of older children and adults [Citation3,Citation30]. Nonetheless, the burden of both IPD and CAP remains substantial among adults [Citation6,Citation9,Citation30].
Two new, higher valent PCVs – PCV15 and PCV20 – have recently been approved by the Medicines and Healthcare products Regulatory Agency for use among adults in the UK, however, at the time of writing JCVI has not published revised pneumococcal vaccination guidelines since their approval. PCV15 (manufactured by MSD) includes the serotypes in PCV13 (1, 3, 4, 5, 6A, 6B, 7F, 9V, 14, 18C, 19A, 19F, 23F) plus two additional serotypes (22F, 33F) and PCV20 (manufactured by Pfizer) includes the serotypes in PCV15 plus five additional serotypes (8, 10A, 11A, 12F, 15B). Although PCV13 is only recommended among a very limited subgroup of high-risk UK adults, it is anticipated that these new PCVs may reduce disease burden in adults versus PPV23 – due to their broader serotype coverage than existing conjugate vaccines and expected greater, longer-lasting protection compared to PPV23 [Citation2,Citation31–36] – and thus may be a cost-effective use of healthcare resources. However, analyses of cost-effectiveness of use of higher-valent PCVs among adults in England have not been previously published. Accordingly, we evaluated the cost-effectiveness of PCV20 alone as well as various other strategies against a single dose of PPV23 in a population of adults currently eligible for pneumococcal vaccination in England.
2. Methods
2.1. Model description
A deterministic model with a Markov-type process was used to depict the lifetime risk of clinical outcomes and economic costs of pneumococcal disease in a hypothetical closed population comprising all adults aged 65–99 years and adults aged 18–64 years with underlying conditions in England (i.e. those adults currently eligible for vaccination with PPV23) (). The model population is stratified at model entry by age (i.e. in one-year increments) and risk profile (i.e. low, moderate, or high risk). Persons may transition to a higher risk group, but not to a lower risk group, during the modeling horizon. Persons in the model population may be assumed to receive any of the following at model entry or subsequently: PCV20, PCV20→PPV23 (i.e. PCV20 at model entry [i.e. year 1] followed by PPV23 at start of year 2), PCV15→PPV23 (i.e. PCV15 at model entry followed by PPV23 in year 2), PPV23, PPV23+PPV23 (i.e. PPV23 at model entry and again at start of year 6), or no vaccine.
Expected clinical outcomes and economic costs are projected annually for the model population, based on age, risk profile, disease/fatality rates, vaccination status, and unit costs of vaccinations and medical care. IPD includes bacteremia and meningitis, and all-cause CAP is stratified by care setting. Vaccinated persons may be at lower risk of future IPD and all-cause CAP; the magnitude of vaccine-associated risk reduction depends on clinical presentation, vaccine type(s), proportion of disease that is vaccine-preventable, age, time since vaccination, and risk profile. Risk of death from IPD, all-cause CAP, and other causes depends upon age and risk profile. Expected medical treatment costs for IPD and all-cause CAP are generated based on event rates and unit costs in relation to care setting. Vaccination costs are tallied in the year of vaccine administration. Clinical outcomes and economic costs projected for each vaccination strategy include IPD and all-cause CAP cases and attributable deaths, life-years (LYs), quality-adjusted LYs (QALYs), and costs of vaccination and medical treatment.
2.2. Model estimation
A summary of methods employed to estimate key model parameters is set forth below; corresponding parameter values are summarized in . A detailed description of methods employed to estimate all model parameters is provided in Supplement A.
Table 1. Base case model input values.
2.2.1. Population
The model population size (N = 15.6 M) and age distribution were based on the estimated population in England in 2022, the year when PCV20 became available for adults [Citation37]. Persons in each age group were allocated into low-, moderate- (immunocompetent with underlying medical conditions), and high-risk (immunocompromised) subgroups based on risk factors defined in The Green Book of Immunisation against infectious disease, Chapter 25 [Citation28] and published risk distributions [Citation38] (see Supplement A for a comprehensive description of conditions considered).
2.2.2. Rates of IPD
Annual incidence of IPD was estimated by age (in one-year increments) and risk profile using the most recent age-specific disease rates available [Citation2,Citation3], age-specific distributions by risk profile, and age-specific odds ratios (ORs) for IPD by risk profile [Citation38]. IPD rates were apportioned between bacteremia and meningitis based on a recent prospective study [Citation4].
2.2.3. Rates of all-cause CAP
Annual incidence of all-cause hospitalized CAP was estimated by age and risk profile using age-specific rates of radiologically confirmed pneumonia from a recent prospective study conducted in Bristol, UK [Citation5], age-specific distributions by risk profile [Citation38], and age-specific fully adjusted ORs for all-cause hospitalized CAP by risk profile [Citation39]. All-cause outpatient CAP was not considered in base case analyses.
2.2.4. Effectiveness of PCVs
For PCVs, vaccine effectiveness (VE) was based principally on randomized control trial data from the Community-Acquired Pneumonia Immunization Trial in Adults (CAPiTA) [Citation27], which provides efficacy estimates for PCV13 and has been used in previous PCV20 cost-effectiveness analyses [Citation40]. Initial effectiveness of PCVs against VT-IPD and VT-CAP for low- and moderate-risk persons aged ≥18 years was based on data from CAPiTA and post hoc analyses [Citation27,Citation41]. Initial VE against VT-IPD and VT-CAP for high-risk persons aged 18–99 years was assumed to be equal to 80% of corresponding values for low-/moderate-risk persons [Citation42,Citation43]. Initial VE-PCV was assumed to persist for 5 years, consistent with CAPiTA [Citation27,Citation44], and to wane thereafter, as follows: 5% annual decline during years 6–10, 10% annual decline during years 11–15, and no efficacy from year 16 through the end of the modeling horizon [Citation41].
2.2.5. Effectiveness of PPV23
For VE-PPV23, we used the most recent real-world effectiveness studies conducted among UK adults, which are in line with assumptions for VE-PPV23 employed in previously published cost-effectiveness analyses. Risk-specific VE-PPV23 against VT-IPD for persons aged ≥18 years was derived from the study by Djennad et al. [Citation2]. VE-PPV23 against VT-CAP was assumed to be zero based on findings by Lawrence et al. which do not provide robust evidence that PPV23 confers any meaningful protection against hospitalized CAP to older UK adults and is consistent with findings of a recent meta-analysis [Citation26,Citation45]. Beyond year 1 of the modeling horizon, VE-PPV23 against VT-IPD was assumed to decline [Citation2] linearly, as follows: decline to 76.2% of initial VE by year 5, and decline to no efficacy by year 10.
2.2.6. Serotype coverage
Age-specific vaccine serotype coverage for IPD was based on prospectively collected surveillance data for 2016/2017 [Citation2,Citation3]. Vaccine serotype coverage for all-cause CAP was based on serotype coverage for pneumococcal CAP and the proportion of CAP due to pneumococcus in 2017/2018 [Citation6]. Serotype coverage for pneumococcal CAP was assumed to be the same irrespective of age.
Herd effects for serotypes unique to PCV15 and PCV20 (vs. PCV13) were assumed to begin one year after the corresponding pediatric immunization program is expected to be implemented (i.e. model years 3 and 4, respectively). Serotype coverage for PPV23 was also reduced to account for the impact of herd effects on serotypes common to PPV23 and PCV20. Reductions in serotype coverage due to herd effects from childhood vaccination were based on the observed impact of PCV13 on the five additional serotypes in PCV13 not in PCV7 (excluding serotype 3) [Citation3]. Reduction in serotype coverage was assumed to peak by year 10 and to be sustained at that level thereafter.
2.2.7. Costs
Costs of IPD (bacteremia, £4,542; meningitis, £5,600) and all-cause hospitalized CAP (£4,192) were derived based on average costs per finished consultant episode (FCE) from the 2019/2020 National Health Service (NHS) reference costs [Citation46] and the average number of FCEs per admission from the Hospital Episode Statistics for England [Citation9]. Prices of PPV23 (£16.80), PCV13 (£49.10), PCV15 (£50.30), and PCV20 (£56.50) were based on list values [Citation47,Citation48]. Vaccine administration cost (£10.06) was based on the General Practice Contract (2019) [Citation49].
2.2.8. Utilities
Age- and risk-specific general population health-state utility values (HSUVs) were based on a published study of HSUVs for English adults [Citation50]. For persons who experienced IPD or all-cause CAP, an annual utility decrement (0.13) was applied during the year in which the event occurred [Citation51]. Both utilities and disutilities were estimated based on data sources which employed the EQ-5D instrument.
2.3. Analyses
2.3.1. Base Case
Clinical outcomes and economic costs were projected for the model population under PCV20 alone, PCV20→PPV23, PCV15→PPV23, and PPV23+PPV23 (hypothetical recommendations) and PPV23 (single dose, currently recommended for eligible NHS patients). Vaccine coverage for each strategy was assumed on par with current PPV23 coverage levels [Citation29]. Future costs, LYs, and QALYs were discounted at 3.5% annually; analyses were conducted from the NHS (i.e. healthcare system) perspective. Cost-effectiveness was assessed considering the £20,000–30,000/QALY gained threshold range employed by the National Institute for Health and Care Excellence (NICE) and JCVI [Citation52].
2.3.2. Subgroup and Sensitivity
Subgroup analyses were conducted comparing PCV20 versus PPV23 among all adults aged 65–99 years and adults aged 18–64 years with underlying conditions, respectively. Selected sensitivity analyses comparing PCV20 versus PPV23 were conducted in which alternative input values were employed for key model parameters. Probabilistic sensitivity analyses (PSA) were conducted (1,000 replications) for all strategies considered to account for uncertainty surrounding estimates of key model parameters. Inputs employed in sensitivity analyses and corresponding methods of estimation are described in Supplement A.
3. Results
3.1. Base case
Clinical and economic outcomes and full incremental cost-effectiveness results for all strategies evaluated are presented in . The cost-efficiency frontier – which comprises the PPV23 single dose strategy, PCV20 alone strategy, and PCV20→PPV23 strategy – indicates that the PCV15→PPV23 and PPV23+PPV23 strategies are dominated by strategies involving PCV20 (). Although PCV20→PPV23 is on the cost-efficiency frontier, it was not cost-effective because its incremental cost-effectiveness ratio (ICER) compared with PCV20 alone (£686,948/QALY) was above the upper bound of the cost-effectiveness range. PCV20 alone was therefore deemed the most relevant hypothetical vaccination strategy.
Figure 2. Cost-effectiveness plane for alternative vaccination strategies among moderate- and high-risk adults aged 18–64 years and all adults aged 65–99 years in England (N = 15,635,909).
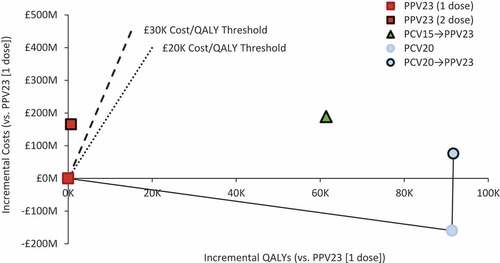
Table 2. Results of base case analyses of alternative vaccination strategies among moderate- and high-risk adults aged 18–64 years and all adults aged 65–99 years in England (N = 15,635,909).
Compared with PPV23 (single dose), PCV20 alone would prevent an additional 7,789 IPD cases, 140,046 hospitalized CAP cases, and 22,199 pneumococcal-related deaths and save £538.31 million in medical care costs (). Total vaccination costs with PCV20 would increase by £378.70 million, and thus use of PCV20 would save the NHS £159.61 million. With total net costs lower by £159.61 million and discounted QALYs higher by 91,375, PCV20 was dominant versus PPV23; incremental net monetary benefit was £1.99 billion at £20,000/QALY gained and £2.90 billion at £30,000/QALY gained.
Table 3. Incremental results of base case, subgroup, and sensitivity analyses comparing PCV20 alone vs. PPV23 (single dose) among moderate- and high-risk adults aged 18–64 and all adults aged 65–99 years in England (N = 15,635,909).
3.2. Subgroup and Sensitivity
PCV20 was found to be cost-effective – if not cost saving – versus PPV23 in all subgroup and sensitivity analyses (). Sensitivity analyses also suggest that PCV20 alone would remain cost saving (vs. PPV23) at prices up to 4.4 times (£73.23) the PPV23 list price, and would be cost-effective at prices up to 15.8 to 21.5 times (£264.82 and £360.61) the PPV23 list price at £20,000/QALY and £30,000/QALY, respectively. In PSA, PCV20 alone was cost saving versus PPV23 in 85% of simulations and cost-effective in 99% of simulations (WTP = £30,000/QALY) (). The cost-effectiveness acceptability frontier indicates that PCV20 is always the optimal strategy ().
Figure 3. Scatterplot for cost-effectiveness of PCV20 alone versus PPV23 (single dose) among moderate- and high-risk adults aged 18–64 years and all adults aged 65–99 years in England (N = 15,635,909).WTP: willingness-to-pay
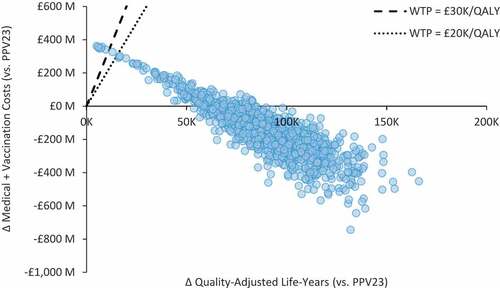
Figure 4. Cost-effectiveness acceptability curve for alternative pneumococcal vaccination strategies among moderate- and high-risk adults aged 18–64 years and all adults aged 65–99 years in England (N = 15,635,909).CEAF: cost-effectiveness acceptability frontier*The CEAF illustrates the probability of greatest net monetary benefit
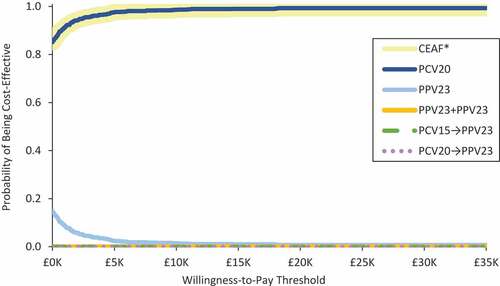
4. Discussion
With recent approval of next generation PCVs with expanded serotype coverage, we undertook a new evaluation to examine the potential cost-effectiveness of pneumococcal vaccination strategies – including PCV20 alone and others – relative to PPV23 alone (single dose). Our findings suggest replacing PPV23 with PCV20 alone for vaccination of adults aged 65–99 years as well as adults aged 18 to 64 years with underlying conditions in England would save lives and reduce health care resources and costs to the NHS. Furthermore, PCV20 alone was the most efficient vaccination strategy among those explored, dominating the strategy involving revaccination with PPV23 (2 doses total), as well as PCV15 in sequence with PPV23. Our finding that PCV20 alone is a cost saving replacement for PPV23 reflects the latest pre-COVID serotype coverage for pneumococcal disease among adults [Citation2,Citation3,Citation6] and the expected greater and longer-lasting protection provided by PCV20 compared to PPV23, which largely offsets the 7% difference in IPD serotype coverage between vaccines (PPV23 non-PCV20 serotypes: 2, 9N, 17F, and 20; PCV20 non-PPV23 serotypes: 6A/6C) [Citation2,Citation27,Citation44,Citation53,Citation54].
To the best of our knowledge, this is the first study to report on cost-effectiveness of PCV20 use among adults in England, however, several analyses have been conducted focused on the United States (US). Findings presented by the US Advisory Committee on Immunization Practices (ACIP) pneumococcal work group suggest that use of PCV20 alone among US adults aged ≥65 years as well as those aged <65 years with risk factors would be cost saving compared to the 2019 US adult pneumococcal vaccines recommendation [Citation55–57]. Based on consideration of all domains included in the evidence-to-recommendation framework, including cost-effectiveness analyses, the ACIP recently recommended the use of PCV20 alone among moderate- and high-risk adults aged 19–64 years and all adults aged ≥65 years [Citation55].
Base case analyses described herein were conducted taking a conservative approach to several aspects of model parameterization. Although higher-valent PCVs have been neither licensed nor recommended for infants and children in England, herd effects from future pediatric use of PCV20 and PCV15 were considered. In addition, base case analyses did not account for the considerable burden of outpatient CAP [Citation58]. Long-term clinical consequences and costs of pneumococcal disease also were not included though common following acute illness [Citation7,Citation59,Citation60]. Finally, despite evidence suggesting that risk of death may remain elevated for several years following an acute hospitalization for IPD or CAP [Citation7,Citation8,Citation60] – especially among the elderly and those with comorbidities (i.e. 65% of the English population) [Citation8,Citation37–39] – we considered only in-hospital pneumonia deaths.
Robust estimates of the incidence of hospitalized CAP in the UK are limited. In base case analyses, we employed recently published estimates from the first prospective study of CAP in the UK by Hyams et al. [Citation5] which suggest that CAP incidence is three to five times higher than previous estimates based on data from Nottingham, which were employed in the 2016 JCVI analyses [Citation5,Citation6,Citation61,Citation62]. To evaluate the impact of CAP incidence rates on model outcomes, we conducted sensitivity analyses in which we conservatively assumed CAP incidence rates based on Pick et al. (Nottingham data) and twice the rates from Pick et al., respectively. The latter analysis was similar to one conducted by JCVI in 2016 to address concerns of likely substantial CAP under ascertainment [Citation6]. Despite the low incidence rates, PCV20 was found to be cost-effective versus PPV23, with ICERs of approximately £6,500/QALY and £1,100/QALY, respectively. The difference in rates reported by Pick et al. and Hyams et al. is believed to be largely attributable to differences in study design. The Nottingham study was designed primarily to measure serotype distribution and trends for CAP [Citation1,Citation6,Citation61]. The study by Hyams et al., however, was designed to estimate CAP incidence and also employed a novel, potentially more robust approach to estimate the population denominator used to calculate incidence rates [Citation5]. Estimates by Hyams et al. are comparable to rates reported in other industrialized countries and are better aligned with the number of hospital admissions for pneumonia in the UK (based on ICD-10 code J18) [Citation9,Citation63].
Because the observed decline in serotype 3 disease among UK adults after the introduction of PCV13 among infants was lower than the decline in disease due to the other five serotypes that are in PCV13 but not in PCV7, and because incidence of IPD due to serotype 3 began increasing again in 2013/2014, there is some concern that PCV13 – and therefore also PCV20 – may be less effective in preventing serotype 3 disease [Citation3]. However, as summarized in a recently published review article, evidence suggests that PCV13 is indeed effective against IPD and CAP due to serotype 3 [Citation64–67]. We therefore conducted a scenario analysis using estimates of VE-PCV20 against serotype 3 CAP based on the study by Gessner et al. and found PCV20 alone to be dominant versus PPV23 [Citation67].
Assumed effectiveness of PPV23 against CAP is an area of parameter uncertainty. Consistent with findings of multiple meta-analyses, assumptions employed in recently published economic evaluations, and the 2015 JCVI position, we assumed that PPV23 conferred no benefit against CAP in the base case [Citation11–25,Citation54,Citation68,Citation69]. However, given recent literature suggesting some limited effectiveness of PPV23 against VT-CAP, we conducted a scenario analysis based on estimates from the study by Lawrence et al. which employed real-world data from the UK [Citation45,Citation70]. Although the differences in cases and deaths due to hospitalized CAP and medical care costs were reduced, PCV20 remained dominant (vs. PPV23). Due to the high degree of uncertainty surrounding recent literature – both within and across studies – findings from analyses in which PPV23 is assumed to provide some protection against CAP should be considered with caution until higher-quality data in support of this assumption become available. We also note that in line with NICE and JCVI methodological guidance [Citation52,Citation71], analyses considering no vaccine (i.e. as a standalone strategy) vs. PPV23 were not conducted because PPV23 is the current standard of care in the UK.
To account for uncertainty in the future price of PCV20, we considered alternative prices and found that PCV20 alone remained cost saving (vs. PPV23) at prices up to 4.4 times the PPV23 list price and was cost-effective at prices up to 21.5 times the PPV23 list price (assuming WTP = £30,000/QALY). As PPV23 recently changed to being centrally procured [Citation72], it may now be procured at a discounted price, in which case the price of PPV23 employed in our analyses may somewhat overestimate the current cost of adult pneumococcal vaccination to the NHS.
The true reduction in hospitalization costs with PCV20 (vs. PPV23) is underestimated in our base case analysis as the cost of IPD and CAP cases treated in intensive care – estimated at 5.2% of all hospitalized CAP cases in 2018/19 [Citation73] – was not accounted for. Published literature suggests intensive care hospitalizations for pneumonia span 11 days on average at a cost of £1,500 per bed-day [Citation74,Citation75]. Given that patients with IPD generally experience more severe symptoms, it is likely that the proportion of patients admitted to intensive care for IPD is even greater, however, we have not found published estimates on IPD intensive care admissions. In addition, new research suggests NHS reference costs fail to account for the opportunity cost of hospitalization [Citation76,Citation77]. This may be especially relevant in the context of pneumonia, which tends to peak during winter when many hospitals are at capacity. Further research is also needed to estimate the impact of pneumonia on social care.
Societal costs of pneumococcal disease were also omitted from these analyses in line with JCVI guidance [Citation52], thus excluding the benefits of vaccination in avoiding productivity loss to patient and carers. The adoption of a societal perspective in health technology assessments of vaccines has been discussed in the literature [Citation78,Citation79]. A recent cost-effectiveness analysis of COVID-19 vaccination by Public Health England and London School of Hygiene & Tropical Medicine researchers demonstrates the relevance of the broader impact of vaccinations [Citation75].
The burden of pneumococcal disease in England has been greatly reduced in the past two years due to measures implemented to reduce transmission of COVID-19 (i.e. lockdowns, social distancing measures, mask-wearing) [Citation80]. However, in light of recent reports of out-of-season respiratory syncytial virus outbreaks across the globe [Citation81–85] as well as data showing PCV13-type IPD cases increased in 2021 (vs. prior years) in England and elsewhere [Citation86–88], there is concern that major outbreaks of common respiratory disease may occur in England as pandemic restrictions are further relaxed [Citation89]. It is therefore critical that delivery of adult vaccinations be prioritized, especially for older adults and others at elevated risk [Citation90].
5. Conclusions
Results of our analyses suggest that the use of PCV20 in lieu of a single dose of PPV23 among all adults aged 65–99 years and adults aged 18–64 years with underlying conditions in England would be cost saving (i.e. more effective and less costly than the current program). Other strategies considered were either too costly or less efficient than PCV20. The prolonged duration of protection and greater effectiveness against vaccine-type IPD and CAP with use of PCV20 results in greater protection of vulnerable adults, and therefore has the potential to substantially reduce the clinical, resource, and financial burden of pneumococcal disease on the NHS.
Declaration of interest
The research described herein and manuscript development was supported by Pfizer Inc. D Mendes, R Sato, A Vyse, J Campling, G Ellsbury, and T Mugwagwa are employees and shareholders of Pfizer; no other relationships or activities that could appear to have influenced the submitted work. M Slack has received personal fees from GSK, Pfizer, Merck, AstraZeneca and Sanofi Pasteur as a speaker at international meetings and as a member of advisory boards (outside the scope of the submitted work). M Slack has also worked as a contractor for Pfizer and received remuneration from Pfizer. M Atwood, A Averin, and D Weycker are employees of Policy Analysis Inc. (PAI), which received financial support from Pfizer Inc. for this study (including manuscript preparation). The authors have no other relevant affiliations or financial involvement with any organization or entity with a financial interest in or financial conflict with the subject matter or materials discussed in the manuscript apart from those disclosed.
Reviewer disclosures
Peer reviewers on this manuscript have no relevant financial or other relationships to disclose.
Author contributions
Authorship was designated based on guidelines promulgated by the International Committee of Medical Journal Editors (2004). All persons who met criteria for authorship were included in the author list. The contribution of each of these persons to this study is as follows: (1) conception and design (all authors), acquisition of data (A Averin, D Mendes, A Vyse, T Mugwagwa), analysis or interpretation of data (all authors); and (2) preparation of manuscript (all authors), critical review of manuscript (all authors). All authors have read and approved the final version of the manuscript. The study sponsor, Pfizer Inc., reviewed the study research plan and study manuscript; data management, processing, and analyses were conducted by PAI. All final analytic decisions and the decision to submit for publication were made solely by study investigators.
Supplemental Material
Download Zip (704.4 KB)Supplementary material
Supplemental data for this article can be accessed online at https://doi.org/10.1080/14737167.2022.2134120
Additional information
Funding
References
- Chalmers J, Campling J, Ellsbury G, et al. Community-acquired pneumonia in the United Kingdom: a call to action. Pneumonia. 2017;9(1):15.
- Djennad A, Ramsay ME, Pebody R, et al. Effectiveness of 23-valent polysaccharide pneumococcal vaccine and changes in invasive pneumococcal disease incidence from 2000 to 2017 in those aged 65 and over in England and Wales. EClinicalMedicine. 2018;6:42–50.
- Ladhani SN, Collins S, Djennad A, et al. Rapid increase in non-vaccine serotypes causing invasive pneumococcal disease in England and Wales, 2000-17: a prospective national observational cohort study. Lancet Infect Dis. 2018;18(4):441–451.
- Amin-Chowdhury Z, Collins S, Sheppard C, et al. Characteristics of invasive pneumococcal disease caused by emerging serotypes after the introduction of the 13-valent pneumococcal conjugate vaccine in england: a PROSPECTIVE OBSERVATIONAL COHORT STUDY, 2014–2018. Clinl Infect Dis. 2020;71(8):e235–e243.
- Hyams C, Begier E, Gonzalez MG Incidence of acute lower respiratory tract disease hospitalisations, including pneumonia, among adults in Bristol, UK, 2019, estimated using both a prospective and retrospective methodology. BMJ Open. 2022 Jun 15;12(6):e057464. DOI: 10.1136/bmjopen-2021-057464.
- Pick H, Daniel P, Rodrigo C, et al. Pneumococcal serotype trends, surveillance and risk factors in UK adult pneumonia, 2013–18. Thorax. 2020;75(1):38–49.
- Myint PK, Hawkins KR, Clark AB, et al. Long-term mortality of hospitalized pneumonia in the EPIC-Norfolk cohort. Epidemiol Infect. 2016;144(4):803–809.
- Averin A, Shaff M, Weycker D, et al. Mortality and readmission in the year following hospitalization for pneumonia among US adults. Respir Med. 2021;185:106476.
- NHS Digital. Hospital Admitted Patient Care Activity 2019-20. 2020.
- Health and Social Care Public Health Agency. Changes to the infant pneumococcal conjugate vaccine schedule: information for healthcare practitioners. 2019.
- Boccalini S, Bechini A, Levi M, et al. Cost-effectiveness of new adult pneumococcal vaccination strategies in Italy. Hum Vaccin Immunother. 2013;9(3):699–706.
- Cho B-H, Stoecker C, Link-Gelles R, et al. Cost-effectiveness of administering 13-valent pneumococcal conjugate vaccine in addition to 23-valent pneumococcal polysaccharide vaccine to adults with immunocompromising conditions. Vaccine. 2013;31(50):6011–6021.
- Evers SMAA, Ament AJHA, Colombo GL, et al. Cost-effectiveness of pneumococcal vaccination for prevention of invasive pneumococcal disease in the elderly: an update for 10 Western European countries. Eur J Clin Microbiol Infect Dis. 2007;26(8):531–540.
- Fry AM, Zell ER, Schuchat A, et al. Comparing potential benefits of new pneumococcal vaccines with the current polysaccharide vaccine in the elderly. Vaccine. 2002;21(3):303–311.
- Heo JY, Seo YB, Choi WS, et al. Cost-effectiveness of pneumococcal vaccination strategies for the elderly in Korea. PLOS ONE. 2017;12(5):e0177342.
- S-l H, Kondo M, Okubo I. Economic Evaluation of Immunisation Programme of 23-Valent Pneumococcal Polysaccharide Vaccine and the Inclusion of 13-Valent Pneumococcal Conjugate Vaccine in the List for Single-Dose Subsidy to the Elderly in Japan. PLOS ONE. 2015;10(10):e0139140.
- Johnstone J, Marrie TJ, Eurich DT, et al. Effect of pneumococcal vaccination in hospitalized adults with community-Acquired Pneumonia. Arch Internal Med. 2007;167(18):1938–1943.
- Kuhlmann A, Theidel U, Pletz MW, et al. Potential cost-effectiveness and benefit-cost ratios of adult pneumococcal vaccination in Germany. Health Econ Rev. 2012;2(1):4.
- Moberley S, Holden J, Tatham DP, et al. Vaccines for preventing pneumococcal infection in adults. Cochrane Database Syst Rev. 2013; 1. DOI:10.1002/14651858.CD000422.pub3.
- Örtqvist Å, Hedlund J, Burman L-Å, et al. Randomised trial of 23-valent pneumococcal capsular polysaccharide vaccine in prevention of pneumonia in middle-aged and elderly people. Lancet. 1998;351(9100):399–403.
- Simberkoff MS, Cross AP, Al-Ibrahim M, et al. Efficacy of Pneumococcal Vaccine in High-Risk Patients. N Engl J Med. 1986;315(21):1318–1327.
- Smith KJ, Wateska AR, Nowalk MP, et al. Modeling of Cost Effectiveness of Pneumococcal Conjugate Vaccination Strategies in U.S. Old Adults Am J Prev Med. 2013;44(4):373–381.
- Stoecker C, Kim L, Gierke R, et al. Incremental cost-effectiveness of 13-valent pneumococcal conjugate vaccine for adults age 50 Years and older in the United States. J Gen Intern Med. 2016;31(8):901–908.
- Stoecker C, Kobayashi M, Matanock A, et al. Cost-effectiveness of continuing pneumococcal conjugate vaccination at age 65 in the context of indirect effects from the childhood immunization program. Vaccine. 2020;38(7):1770–1777.
- Latifi-Navid H, Latifi-Navid S, Mostafaiy B, et al. Pneumococcal disease and the effectiveness of the PPV23 vaccine in adults: a two-stage bayesian meta-analysis of observational and RCT reports. Sci Rep. 2018;8(1):11051.
- Tin Htar M T, Stuurman AL, Ferreira G, et al. Effectiveness of pneumococcal vaccines in preventing pneumonia in adults, a systematic review and meta-analyses of observational studies. PLOS ONE. 2017;12(5):e0177985.
- Bonten MJM, Huijts SM, Bolkenbaas M, et al. Polysaccharide conjugate vaccine against pneumococcal pneumonia in adults. N Engl J Med. 2015;372(12):1114–1125.
- Ramsay M. The Green Book for immunisation against infectious disease: chapter 25, Pneumococcal. Public Health England. 2020.
- UK Health Security Agency. Pneumococcal Polysaccharide Vaccine (PPV) coverage report, England, April 2019 to March 2020. Health Prot Rep. 2020;15(19).
- Waight PA, Andrews NJ, Ladhani SN, et al. Effect of the 13-valent pneumococcal conjugate vaccine on invasive pneumococcal disease in England and Wales 4 years after its introduction: an observational cohort study. Lancet Infect Dis. 2015;15(5):535–543.
- Adler H, German EL, Mitsi E, et al. Experimental human pneumococcal colonization in older adults is feasible and safe, not immunogenic. Am J Respir Crit Care Med. 2021;203(5):604–613.
- Andrews NJ, Waight PA, George RC, et al. Impact and effectiveness of 23-valent pneumococcal polysaccharide vaccine against invasive pneumococcal disease in the elderly in England and Wales. Vaccine. 2012;30(48):6802–6808.
- Collins AM, Wright AD, Mitsi E, et al. First human challenge testing of a pneumococcal vaccine. double-blind randomized controlled trial. Am J Respir Crit Care Med. 2015;192(7):853–858.
- German EL, Solórzano C, Sunny S, et al. Protective effect of PCV vaccine against experimental pneumococcal challenge in adults is primarily mediated by controlling colonisation density. Vaccine. 2019;37(30):3953–3956.
- Jackson LA, Gurtman A, van Cleeff M, et al. Influence of initial vaccination with 13-valent pneumococcal conjugate vaccine or 23-valent pneumococcal polysaccharide vaccine on anti-pneumococcal responses following subsequent pneumococcal vaccination in adults 50 years and older. Vaccine. 2013;31(35):3594–3602.
- Jochems SP, Weiser JN, Malley R, et al. The immunological mechanisms that control pneumococcal carriage. PLoS Pathog. 2017;13(12):e1006665.
- Office for National Statistics (UK). 2018-based National Population Projections. 2019.
- van Hoek Aj, Andrews N, Waight PA, et al. The effect of underlying clinical conditions on the risk of developing invasive pneumococcal disease in England. J Infect. 2012;65(1):17–24.
- Campling J, Jones D, Chalmers JD, et al. The impact of certain underlying comorbidities on the risk of developing hospitalised pneumonia in England. Pneumonia. 2019;11(1):4.
- Stoecker C Economic Assessment of PCV15 & PCV20. Meeting of the ACIP: 2021 Jun 25; Virtual.
- Mangen MJ, Rozenbaum MH, Huijts SM, et al. Cost-effectiveness of adult pneumococcal conjugate vaccination in the Netherlands. Eur Respir J. 2015;46(5):1407–1416.
- Klugman KP, Madhi SA, Huebner RE, et al. A Trial of a 9-valent pneumococcal conjugate vaccine in children with and those without HIV infection. N Engl J Med. 2003;349(14):1341–1348.
- French N, Gordon SB, Mwalukomo T, et al. A trial of a 7-valent pneumococcal conjugate vaccine in HIV-Infected adults. N Engl J Med. 2010;362(9):812–822.
- Patterson S, Webber C, Patton M, et al. A post hoc assessment of duration of protection in CAPiTA (Community Acquired Pneumonia immunization Trial in Adults). Trials Vaccinol. 2016;5:92–96.
- Lawrence H, Pick H, Baskaran V, et al. Effectiveness of the 23-valent pneumococcal polysaccharide vaccine against vaccine serotype pneumococcal pneumonia in adults: a case-control test-negative design study. PLoS Med. 2020;17(10):e1003326.
- Department of Health. NHS reference costs 2019 to 2020. 2021.
- Joint Formul Comm British Nat Formul. 2017.
- MIMS Database. 2022.
- NHS England. Directed enhanced service specification: seasonal influenza and pneumococcal polysaccharide vaccination program 2019/20. 2019 Mar 29.
- Ara R, Brazier JE. Using health state utility values from the general population to approximate baselines in decision analytic models when condition-specific data are not available. Value Health. 2011;14(4):539–545.
- Mangen M-J-J, Huijts SM, Bonten MJM, et al. The impact of community-acquired pneumonia on the health-related quality-of-life in elderly. BMC Infect Dis. 2017;17(1):208.
- Joint Committee on Vaccination and Immunisation. Code of Practice June 2013.
- Essink B, Sabharwal C, Cannon K, et al. Pivotal phase 3 randomized clinical trial of the safety, tolerability, and immunogenicity of 20-valent pneumococcal conjugate vaccine in adults aged ≥18 years. Clinl Infect Dis. 2022;75(3):390–398.
- Joint Committee on Vaccination and Immunisation. Minutes of the meeting on 2015 Oct 07.
- Kobayashi M, Farrar JL, Gierke R, et al. Use of 15-valent pneumococcal conjugate vaccine and 20-valent pneumococcal conjugate vaccine among U.S. adults: updated recommendations of the advisory committee on immunization practices - United States, 2022. MMWR Morb Mortal Wkly Rep. 2022;71(4):109–117.
- Matanock A, Lee G, Gierke R, et al. Use of 13-valent pneumococcal conjugate vaccine and 23-valent pneumococcal polysaccharide vaccine among adults aged ≥65 years: updated recommendations of the advisory committee on immunization practices. MMWR Morb Mortal Wkly Rep. 2019;68(46):1069–1075.
- Leidner AJ Summary of three economic models assessing pneumococcal vaccines in US adults. Pneumococcal Vaccines. Meeting of the ACIP: September 29, 2021; Atlanta, GA.
- Sun X, Douiri A, Gulliford M. Pneumonia incidence trends in UK primary care from 2002 to 2017: population-based cohort study. Epidemiol Infect. 2019;147:e263.
- Campling J, Jones D, Chalmers J, et al. Clinical and financial burden of hospitalised community-acquired pneumonia in patients with selected underlying comorbidities in England. BMJ Open Respir Res. 2020;7(1):e000703.
- Hughes GJ, Wright LB, Chapman KE, et al. Serotype-specific differences in short- and longer-term mortality following invasive pneumococcal disease. Epidemiol Infect. 2016;144(12):2654–2669.
- Rodrigo C, Bewick T, Sheppard C, et al. Impact of infant 13-valent pneumococcal conjugate vaccine on serotypes in adult pneumonia. Eur Respir J. 2015;45(6):1632–1641.
- van Hoek AJ, Miller E . Cost-effectiveness of vaccinating immunocompetent ≥65 year olds with the 13-valent pneumococcal conjugate vaccine in England. PLOS ONE. 2016;11(2):e0149540.
- Shi T, Denouel A, Tietjen AK, et al. Global and regional burden of hospital admissions for pneumonia in older adults: a systematic review and meta-analysis. J Infect Dis. 2019;222(Supplement_7):S570–S576.
- Vyse A, Campling J, Czudek C, et al. A review of current data to support decision making for introduction of next generation higher valency pneumococcal conjugate vaccination of immunocompetent older adults in the UK. Expert Rev Vaccines. 2021;20(10):1311–1325.
- McLaughlin JM, Jiang Q, Gessner BD, et al. Pneumococcal conjugate vaccine against serotype 3 pneumococcal pneumonia in adults: a systematic review and pooled analysis. Vaccine. 2019;37(43):6310–6316.
- Sings HL, De Wals P, Gessner BD, et al. Effectiveness of 13-valent pneumococcal conjugate vaccine against invasive disease caused by serotype 3 in children: a systematic review and meta-analysis of observational studies. Clinl Infect Dis. 2018;68(12):2135–2143.
- Gessner BD, Jiang Q, Van Werkhoven CH, et al. A post-hoc analysis of serotype-specific vaccine efficacy of 13-valent pneumococcal conjugate vaccine against clinical community acquired pneumonia from a randomized clinical trial in the Netherlands. Vaccine. 2019;37(30):4147–4154.
- Sisk JE, Whang W, Butler JC, et al. Cost-effectiveness of vaccination against invasive pneumococcal disease among people 50 through 64 years of age: role of comorbid conditions and race. Ann Intern Med. 2003;138(12):960–968.
- Smith KJ, Zimmerman RK, Lin CJ, et al. Alternative strategies for adult pneumococcal polysaccharide vaccination: a cost-effectiveness analysis. Vaccine. 2008;26(11):1420–1431.
- Suzuki M, Dhoubhadel BG, Ishifuji T, et al. Serotype-specific effectiveness of 23-valent pneumococcal polysaccharide vaccine against pneumococcal pneumonia in adults aged 65 years or older: a multicentre, prospective, test-negative design study. Lancet Infect Dis. 2017;17(3):313–321.
- National Institute for Health and Care Excellence. NICE health technology evaluations: the manual. Jan 2022.
- Public Health England. Pneumococcal polysaccharide vaccine: change to the supply route from. Jun 2021.
- Lim WS, Lawrence H. National audit report: adult community-acquired pneumonia audit 2018-2019. Brit Thoracic Soc. 2019;10(4).
- Ostermann H, Garau J, Medina J, et al. Resource use by patients hospitalized with community-acquired pneumonia in Europe: analysis of the REACH study. BMC Pulm Med. 2014;14(1):36.
- Sandmann FG, Davies NG, Vassall A, et al. The potential health and economic value of SARS-CoV-2 vaccination alongside physical distancing in the UK: a transmission model-based future scenario analysis and economic evaluation. Lancet Infect Dis. 2021;21(7):962–974.
- Sandmann FG, Shallcross L, Adams N, et al. Estimating the hospital burden of norovirus-associated gastroenteritis in England and its opportunity costs for nonadmitted patients. Clinl Infect Dis. 2018;67(5):693–700.
- Sandmann FG, Robotham JV, Deeny SR, et al. Estimating the opportunity costs of bed-days. Health Econ. 2018;27(3):592–605.
- Bloom DE, Brenzel L, Cadarette D, et al. Moving beyond traditional valuation of vaccination: needs and opportunities. Vaccine. 2017;35:A29–A35.
- Jit M, Hutubessy R. Methodological challenges to economic evaluations of vaccines: is a common approach still possible? Appl Health Econ Health Policy. 2016;14(3):245–252.
- Brueggemann AB, Jansen van Rensburg MJ, Shaw D, et al. Changes in the incidence of invasive disease due to streptococcus pneumoniae, haemophilus influenzae, and Neisseria meningitidis during the COVID-19 pandemic in 26 countries and territories in the invasive respiratory infection surveillance initiative: a prospective analysis of surveillance data. Lancet Digital Health. 2021;3(6):e360–e370.
- Eden J-S, Sikazwe C, Xie R, et al. Off-season RSV epidemics in Australia after easing of COVID-19 restrictions. Nat Commun. 2022;13(1):2884.
- Fourgeaud J, Toubiana J, Chappuy H, et al. Impact of public health measures on the post-COVID-19 respiratory syncytial virus epidemics in France. Eur J Clin Microbiol Infect Dis. 2021;40(11):2389–2395.
- Hsu H-T, Huang F-L, Ting P-J, et al. The epidemiological features of pediatric viral respiratory infection during the COVID-19 pandemic in Taiwan. J Microbiol Immunol Infect. 2021. DOI:10.1016/j.jmii.2021.09.017.
- Lavoie PM, Reicherz F, Solimano A, et al. Potential resurgence of respiratory syncytial virus in Canada. Can Med Assoc J. 2021;193(29):E1140.
- Olsen SJ, Winn AK, Budd AP, et al. Changes in influenza and other respiratory virus activity during the COVID-19 PANDEMIC - United States, 2020-2021. MMWR Morb Mortal Wkly Rep. 2021;70(29):1013–1019.
- UK Health Security Agency. Pneumococcal disease: cases caused by strains covered by Prevenar 13 vaccine 2021. [cited 2021 Dec 15]. Available from: https://www.gov.uk/government/publications/pneumococcal-disease-cases-caused-by-strains-covered-by-prevenar-13-vaccine/pneumococcal-disease-cases-caused-by-strains-covered-by-prevenar-13-vaccine
- Casanova C, Küffer M, Leib SL, et al. Re-emergence of invasive pneumococcal disease (IPD) and increase of serotype 23B after easing of COVID-19 measures, Switzerland, 2021. Emerg Microbes Infect. 2021;10(1):2202–2204.
- Perniciaro S, van der Linden M, Weinberger DM. Re-emergence of invasive pneumococcal disease in germany during the spring and summer of 2021. Clinl Infect Dis. 2022;75(7):1149–1153.
- Baker RE, Park SW, Yang W, et al. The impact of COVID-19 nonpharmaceutical interventions on the future dynamics of endemic infections. Proc Nat Acad Sci. 2020;117(48):30547.
- Privor-Dumm LA, Poland GA, Barratt J, et al. A global agenda for older adult immunization in the COVID-19 era: a roadmap for action. Vaccine. 2021;39(37):5240–5250.