ABSTRACT
Introduction: The newest intravenous (IV) iron products show an improved safety profile over predecessors, allowing for the rapid administration of relatively high doses. Ferric derisomaltose (FDI; also known as iron isomaltoside), ferric carboxymaltose (FCM), and ferumoxytol (FER), are successful treatments for iron deficiency (Europe; FDI and FCM) and iron deficiency anemia (US; FDI, FCM, and FER).
Areas covered: This review focusses on the chemistry and structure of FDI, FCM, and FER, and on three key aspects of IV iron safety: (1) hypersensitivity; (2) hypophosphatemia and sequelae; (3) cardiovascular safety.
Expert opinion: Although the safety of modern IV iron has improved, immediate infusion reactions and the development of hypophosphatemia must be appreciated and recognized by those who prescribe and administer IV iron. Immediate infusion reactions can occur with any IV iron and are usually mild; severe reactions – particularly anaphylaxis – are extremely rare. The recognition and appropriate management of infusion reactions is an important consideration to the successful administration of IV iron. Severe, persistent, hypophosphatemia is a specific side effect of FCM. No cardiovascular safety signal has been identified for IV iron. Ongoing trials in heart failure will provide additional long-term efficacy and safety data.
1. Introduction
Since the early 20th century, intravenous (IV) iron has been used as a successful treatment for iron deficiency (Europe) and iron deficiency anemia (IDA) (US), in diverse patient populations and across a wide range of therapeutic indications, including conditions related to nephrology, gastroenterology, women’s health, and oncology. The use of IV iron has expanded in recent years, most notably in cardiology, and as part of perioperative blood management, which aims to identify and manage anemia before, during, and after surgery.
Administration of early parenteral iron formulations in the 1950s was associated with serious toxic reactions caused by ‘labile’ or ‘rapid’ iron release [Citation1,Citation2]. Later formulations of IV iron incorporated a carbohydrate coating to surround the core, allowing for a slower release of iron [Citation1]. These early iron–carbohydrate complexes addressed the issue of rapid iron release, but due to reports of anaphylactic reactions, although rare, their use was restricted to circumstances when no other options were available [Citation1,Citation2]. Subsequently, various formulations of IV iron have been developed to improve the safety profile of these products, including the three newest IV iron formulations – ferric derisomaltose (FDI; also known as iron isomaltoside), ferric carboxymaltose (FCM), and ferumoxytol (FER). A tighter binding of elemental iron in the iron–carbohydrate complex reduces the risk of toxicity resulting from labile iron, and the use of carbohydrate moieties with reduced immunogenic activity lowers the risk of serious hypersensitivity reactions [Citation3,Citation4]. The strong binding provides a slow release of iron allowing for FDI, FCM, and FER to be administered at relatively high doses in short infusion times [Citation5–9]. outlines various characteristics of these newer IV iron formulations. Despite the widespread use of IV iron and the improved safety profile of the newer products, some healthcare providers remain wary of using IV iron due to negative perceptions around safety. A literature review on the safety of IV iron evaluated information from 76 articles selected from various sources [Citation11]. The review identified three main safety concerns with the use of IV iron – hypersensitivity reactions, hypophosphatemia, as well as oxidative stress and cardiovascular (CV) events [Citation11].
Table 1. Characteristics of FDI, FCM, and FER [Citation5–10]
The present review article uses published literature to discuss the chemistry and structure of the newer IV irons – FDI, FCM, and FER – and reviews the available evidence for the safety of these three IV iron products, with a focus on hypersensitivity, hypophosphatemia, and CV safety. The implications for the field of IV iron, now and in the future, are captured by expert opinion.
2. Chemistry and structure
All IV iron products including FDI, FCM, and FER are iron–carbohydrate nanoparticles [Citation12], which belong to the class of ‘non-biological complex drugs’ (NBCDs) [Citation13]. The characteristics of iron nanoparticles (and other NBCDs) are defined by their composition, and by the specific proprietary manufacturing process for each product, resulting in differences in their efficacy and safety profiles [Citation13,Citation14]. Classically, IV iron complexes consist of an iron-oxyhydroxide core, surrounded by a carbohydrate coating, which increases the stability of the complex and keeps the particles in colloidal suspension with minimal release of labile iron [Citation12]. FDI, FCM, and FER differ in terms of their chemical and physical properties, including the nature of the carbohydrate moiety, the structure of the iron oxyhydroxide, the size of the molecule, and the surface charge [Citation4].
2.1. Carbohydrate chemistry
The nature of the carbohydrate moiety of IV iron products is one aspect of their chemistry that is often oversimplified, obscuring fundamental differences that are relevant to the properties of IV iron products.
shows the different chemical structures of the carbohydrate moieties used in FDI, FCM, FER, and iron dextran (included mainly for comparison of the carbohydrate structures, since iron dextran is outside the remit of this review). The three carbohydrates – derisomaltose, carboxymaltose, and polyglucose sorbitol carboxymethylether – as well as dextran, consist of glucose or modified glucose units linked through glycosidic bonds.
Figure 1. Chemical structures of the carbohydrate moieties in FDI, FCM, and FER, relative to dextran
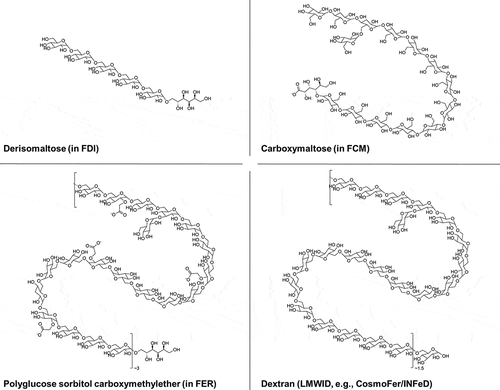
FDI contains derisomaltose, which consists of linear, unbranched hydrogenated isomalto-oligosaccharides with an average molecular weight of 1 kDa (corresponding to 5–6 glucose units) and a narrow molecular weight distribution that is almost devoid of mono- and disaccharides () [Citation4,Citation6].
FCM contains carboxymaltose, which has been derived from a commercially available maltodextrin through the oxidation of the reducing end groups to a carboxylic acid [Citation8,Citation15,Citation16]. Carboxymaltose is made up of 5–20 glucose units and has a predominantly linear α-(1,4) backbone, with branches that are mainly α-(1,6)-linked () [Citation15–17].
FER contains polyglucose sorbitol carboxymethylether, which is produced from a dextran with an approximate molecular weight of 10 kDa [Citation18,Citation19]. Polyglucose sorbitol carboxymethylether is a predominantly linear α-(1,6)-linked glucose polysaccharide, in which the reducing end groups have been hydrogenated, and which has been partially carboxymethylated at positions C-2, C-3, or C-4 [Citation20]. Polyglucose sorbitol carboxymethylether has a low degree of branching (1–2%), mainly of the α-(1,3) type () [Citation20].
For comparison, also presents the chemical structure of the dextran carbohydrate moiety used in low molecular weight iron dextran (LMWID; INFeD® in the US, CosmoFer® outside the US). LMWID consists of a dextran with a molecular weight of between 5 and 7.5 kDa [Citation21], which is structurally similar to the dextran starting material used to produce polyglucose sorbitol carboxymethylether in FER, although somewhat smaller. Generally, the degree of branching in dextran is related to the molecular weight – a lower molecular weight is associated with a lesser degree of branching [Citation22]. Therefore, given its lower molecular weight, the dextran of LMWID is less branched than the dextran used to produce polyglucose sorbitol carboxymethylether.
2.2. Iron–carbohydrate structure
FDI is structurally dissimilar to the classical iron core–carbohydrate shell composition of FCM and FER [Citation4]. The short, linear, nonionic structure of derisomaltose enables the synthesis of a matrix structure that is composed of interchanging layers of linear derisomaltose with iron atoms placed in cavities between, and within, the oligosaccharide molecules [Citation4,Citation6].
FER differs from FDI and FCM in terms of the iron-oxyhydroxide structure – for FER, it is similar to that of magnetite, whereas for FDI and FCM the structure is more consistent with that of akaganeite [Citation4,Citation23,Citation24]. Different iron cores have different thermodynamic stabilities, such that iron complexes containing a core with a higher stability show slower degradation of the complex and a lower level of labile iron [Citation23]. Dissolution analyses have shown that magnetite has a higher dissolution rate than akaganeite [Citation23,Citation25].
2.3. Iron release and pharmacokinetics
Labile iron provides a source of iron for the generation of highly toxic hydroxyl free radicals that can cause oxidative stress to the body’s vital cellular components [Citation26]. The resulting oxidative damage has been speculated to result in detrimental effects, such as cell death, tissue necrosis, and degenerative diseases, or to changes in cell phenotype and cancer formation [Citation26]. However, solid clinical evidence for any of these effects is lacking.
In vitro physicochemical analyses have shown that, generally, FDI, FCM, and FER are associated with lower levels of labile iron than the previous generations of IV iron products [Citation4,Citation23]. This reflects the nature of the iron oxyhydroxide, as well as the tighter binding and higher stability of the iron–carbohydrate complex of these newer products. From a safety perspective, a lower level of labile iron, as observed for FDI, FCM, and FER versus previous generations of IV iron, translates into a lower potential for associated toxicity [Citation23], such as that described earlier.
In 2020, Garbowski and colleagues reported that the level of non-transferrin-bound iron (NTBI) – a measure of labile iron– was 7 -fold lower with FDI than with FCM, and 9-fold lower than with iron sucrose () [Citation27]. The low level of NTBI for FDI versus FCM and iron sucrose, combined with a longer half-life (20.3 hours for FDI versus 6.82 hours for FCM and 3.43 hours for iron sucrose) [Citation27], indicates that FDI is the most stable molecule, in vivo, of the three newer IV iron products.
Figure 2. Bead-NTBI levels following a single dose of FDI, FCM, or iron sucrose [Citation27]
![Figure 2. Bead-NTBI levels following a single dose of FDI, FCM, or iron sucrose [Citation27]](/cms/asset/46e11d29-a1e0-4e6e-9b6e-4cb7758ec5c1/ieds_a_1912010_f0002_b.gif)
The study also reported a higher ferritin level with FCM than with FDI, which was not associated with a greater hemoglobin response [Citation27], a finding that has been observed in comparative randomized controlled trials (RCTs) of FDI, FCM and FER (based on hemoglobin per gram of iron administered to account for dosing differences) [Citation28,Citation29]. These observations suggest that a greater ferritin response, shortly after high-dose IV iron administration, is not reflective of more bioavailable iron.
Separate to the Garbowski et al. study, in vivo pharmacokinetic profiling has confirmed that FDI, FCM, and FER differ in terms of their half-life, which is longest for FDI at 27 hours [Citation6], followed by FER at 15 hours [Citation9], and shortest for FCM at 7–12 hours [Citation8].
3. Safety
3.1. Hypersensitivity
Data from head-to-head RCTs, specifically designed to evaluate the incidence of hypersensitivity reactions with different IV iron products, have been published in the past 3 years [Citation29–32]. As highlighted by the authors of a recently published meta-analysis, much of the previously published data comparing the rate of hypersensitivity reactions between IV iron products come from studies based on spontaneous reporting of adverse drug reactions (ADRs) (recorded in pharmacovigilance databases) in combination with sales data (to estimate exposure) [Citation33]. Whilst ADR reports can provide useful information to identify potential reactions outside of RCTs, they can be associated with reporting bias and scientific limitations. Definitive conclusions about ADRs cannot be made based on spontaneous reporting, and these reports should not be used to compare the safety of IV iron products [Citation34,Citation35].
RCTs are the ‘gold-standard’ of clinical trial design, providing the highest-quality evidence for clinical outcomes that can be obtained from clinical research [Citation36]. Since 2018, data from five RCTs evaluating the incidence of hypersensitivity reactions (as primary or secondary endpoints) occurring with FDI, FCM, and FER, have been published (some of these trials included iron sucrose) [Citation28–32]. The FIRM trial evaluated the composite incidence of moderate-to-severe hypersensitivity reactions (including anaphylaxis) and moderate-to-severe hypotension as the primary endpoint, reporting rates of 0.7% for FCM and 0.6% for FER [Citation29]. The FERWON-IDA trial reported an incidence of serious or severe hypersensitivity reactions (co-primary endpoint alongside change in hemoglobin) of 0.3% for FDI and 0.4% for iron sucrose [Citation30]. The similarly-designed FERWON-NEPHRO trial reported corresponding rates of 0.3% for FDI, with no events reported for iron sucrose [Citation31]. In a pre-specified combined analysis of the two FERWON trials, the incidence of serious or severe hypersensitivity reactions was 0.3% for FDI and 0.2% for iron sucrose [Citation32]. In the FIRM and FERWON trials, reported hypersensitivity reactions were confirmed and adjudicated by a blinded independent committee [Citation29–31]. A pre-specified pooled analysis of data from the PHOSPHARE-IDA trials (PHOSPHARE-IDA04 and PHOSPHARE–IDA05) has shown an incidence of serious or severe hypersensitivity reactions (evaluated as a secondary endpoint) of 0.8% for FDI and 1.7% for FCM [Citation28]. Data from these trials indicate a low incidence of serious or severe hypersensitivity reactions with the newest formulations of IV iron [Citation28–32].
Meta-analysis methodology provides the highest-quality evidence to evaluate the incidence of outcomes with a low event rate, such as serious or life-threatening hypersensitivity reactions with IV iron products. A recent meta-analysis evaluated the incidence of serious or severe hypersensitivity reactions with FDI and FCM (and iron sucrose) in 8,599 patients from clinical trials [Citation33]. Alongside the results from RCTs, this meta-analysis confirms the low incidence of these events with FDI and FCM, as well as with iron sucrose [Citation33].
All IV iron products have the potential to cause mild infusion reactions [Citation37–39]. Such reactions following IV iron treatment are not infrequent [Citation40], and include Fishbane reactions (characterized by flushing in the face, acute chest and/or back pain, and chest tightness, sometimes with dyspnea) [Citation5,Citation37,Citation39], which might be caused by mild manifestations of labile iron toxicity [Citation37], and/or non-allergic complement activation-related pseudo-allergy [Citation41,Citation42]. Most often, the symptoms of mild infusion reactions resolve without treatment after the infusion is stopped and do not re-appear when the infusion is restarted, often at a slower rate [Citation37–39]. Expert opinion suggests that the IV iron infusion can be restarted and completed approximately 15 minutes after the symptoms have abated [Citation37,Citation39]. To minimize the risk of recurrent reactions, patients with urticaria can be pre-medicated with antihistamines (H2 receptor antagonists) prior to re-challenge; corticosteroids can also be considered as pre-medication [Citation38,Citation43]. Healthcare providers may be reluctant to use IV iron due to uncertainty surrounding the management of minor acute infusion reactions (e.g. Fishbane reactions) and mild manifestations of complement activation-related pseudo-allergy reactions [Citation41], which can mimic the early symptoms of a more severe reaction. Various management algorithms have been developed to help healthcare providers manage acute infusion reactions should they occur [Citation37–39].
In summary, the most recent evidence, generated through the conduct of ‘gold–standard’ RCTs that were specifically designed to evaluate hypersensitivity, shows a low incidence of hypersensitivity reactions for FDI, FCM, and FER that is similar between products.
3.2. Hypophosphatemia
Hypophosphatemia is an increasingly recognized side effect of certain IV iron products [Citation7,Citation8,Citation44–47]. FCM, in particular, is consistently associated with significantly higher rates of hypophosphatemia than either FDI or FER [Citation44,Citation46,Citation47].
3.2.1. Incidence of hypophosphatemia in the setting of IV iron treatment
The most compelling evidence for the differences in hypophosphatemia rates between IV iron products comes from four head-to-head RCTs evaluating the incidence of moderate-to-severe hypophosphatemia [Citation28,Citation48,Citation49]. The first trial, published in 2013, reported that FCM was associated with a high incidence of hypophosphatemia (defined as a serum phosphate level of <2.0 mg/dL [0.65 mmol/L]) compared with iron dextran (58.8% and 0.0%, respectively) [Citation48]. In the FCM group, hypophosphatemia persisted in 35.3% of patients at Day 35, with levels normalizing by Day 80 [Citation48].
Pre-specified secondary analyses of the FIRM trial were published in 2018, which reported high rates of hypophosphatemia following treatment with FCM (50.8% versus 0.9% for FER; p < 0.001) [Citation49]. Hypophosphatemia was defined as a serum phosphate level <2.0 mg/dL (0.65 mmol/L), which was termed ‘severe’ [Citation49]. ‘Extreme’ hypophosphatemia (serum phosphate level: <1.3 mg/dL [0.4 mmol/L]) was observed in 10.0% of FCM-treated patients and in 0.0% of the FER treatment group [Citation49]. Severe hypophosphatemia persisted at Day 35 in 29.1% of FCM-treated patients, including 4.7% with extreme hypophosphatemia, versus 0.0% of FER-treated patients (p < 0.001) [Citation49].
The most recent evidence comes from the two PHOSPHARE-IDA trials, published in 2020 [Citation28]. These two identically-designed trials were powered to directly compare the incidence of hypophosphatemia (defined as a serum phosphate level of <2.0 mg/dL [0.65 mmol/L]) between two IV iron products – FDI and FCM [Citation28]. The findings of the pre-specified pooled analysis of the PHOSPHARE-IDA trials are presented in . The incidence of hypophosphatemia at any post-baseline assessment was significantly higher with FCM versus FDI (74.4% versus 8.0%, respectively; p < 0.001) [Citation28]. Severe hypophosphatemia (serum phosphate level: ≤1.0 mg/dL [0.32 mmol/L]) occurred in 11.3% of FCM-treated patients and in 0.0% of FDI-treated patients (p < 0.001) [Citation28]. A sub-analysis of the PHOSPHARE-IDA data has shown that, in the FCM-treated patients who developed incident hypophosphatemia, the condition was persistent in 57.3% of patients (the remaining patients recovered); persistent hypophosphatemia was not observed in FDI-treated patients [Citation50].
Figure 3. Incidence of hypophosphatemia reported during the PHOSPHARE-IDA trials [Citation28,Citation51]
![Figure 3. Incidence of hypophosphatemia reported during the PHOSPHARE-IDA trials [Citation28,Citation51]](/cms/asset/0ec68f25-6dfe-46fe-8604-bbd285063b30/ieds_a_1912010_f0003_b.gif)
The different rates of hypophosphatemia for FCM versus FDI reported in the PHOSPHARE-IDA trials are in accordance with the findings of the double-blind HOMe aFers 1 trial (75.0% for FCM versus 7.7% for FDI; p = 0.001) and the prospective observational Detlie et al. study (56.9% for FCM versus 5.7% for FDI; p < 0.01) [Citation52,Citation53].
In summary, the existing RCT evidence demonstrates a much higher incidence of hypophosphatemia with FCM versus FDI and FER [Citation28,Citation49,Citation52] and is consistent with the findings of numerous published literature reviews and meta-analyses [Citation44,Citation46,Citation47].
3.2.2. Mechanism of hypophosphatemia
Comprehensive research into the underlying mechanism of hypophosphatemia has shown that FCM causes an increase in intact fibroblast growth factor 23 (iFGF23) [Citation28,Citation48,Citation49,Citation54–56].
The three trials published by Wolf et al. (discussed in the previous section) reported that an increase in iFGF23 levels induced by FCM is also associated with reductions in the levels of 1,25-dihydroxyvitamin D (the hormonally active metabolite of vitamin D) and calcium, followed by an increase in parathyroid hormone [Citation28,Citation48,Citation49]. This secondary hyperparathyroidism maintains the increase in phosphate excretion even when iFGF23 levels have normalized, causing hypophosphatemia to persist [Citation28,Citation48,Citation49]. The biochemical cascade of events triggered by FCM has been termed the ‘6H-syndrome’: 1) high FGF23; 2) hyperphosphaturia; 3) hypophosphatemia; 4) hypovitaminosis D; 5) hypocalcemia; and 6) secondary hyperparathyroidism [Citation45]. While FDI and FER can cause hypophosphatemia, although with a lower frequency and severity than has been observed for FCM (as described in Section 3.2.1.), the 6H-syndrome is not induced by these compounds [Citation28,Citation48,Citation49]. summarizes the biochemical changes underlying the 6H-syndrome.
Figure 4. Biochemical basis for hypophosphatemia and associated clinical consequences [Citation28,Citation48,Citation49,Citation57–67]
![Figure 4. Biochemical basis for hypophosphatemia and associated clinical consequences [Citation28,Citation48,Citation49,Citation57–67]](/cms/asset/ecd95321-3718-4063-b97f-6f1ec2fad170/ieds_a_1912010_f0004_b.gif)
3.2.3. Clinical consequences of hypophosphatemia in the setting of IV iron treatment
In the short term, hypophosphatemia following IV iron treatment may be severe and accompanied by acute symptoms, such as pain, muscle weakness and fatigue [Citation44]. In the head-to-head trials described earlier, severe hypophosphatemia was observed following treatment with FCM, but not with FDI [Citation28] or FER [Citation49]. Although these trials did not report on related symptoms, numerous isolated patient reports describing acute symptomatic hypophosphatemia following treatment with FCM have been published [Citation68–73].
In a review paper published in 2017, Zoller et al. discussed the long-term complications of hypophosphatemia following IV iron treatment, such as bone fractures and osteomalacia [Citation44]. Several cases of bone complications were associated with previous FCM treatment [Citation44]. Published patient cases have reported the development of hypophosphatemic osteomalacia and fractures after repeated dosing of FCM [Citation74–76]. No cases of bone complications occurring with FDI or FER have been identified in the literature.
The 6H-syndrome can result in a prolongation of adverse metabolic bone disease effects following an infusion of FCM, which provides a potential mechanistic explanation for the association of FCM with the development of bone complications over the long term [Citation45]. As a downstream consequence of the changes in bone and mineral metabolism, significant increases in alkaline phosphatase (ALP) and bone-specific ALP have been observed after a single treatment course of FCM [Citation28]. Furthermore, in patients who have developed moderate-to-severe hypophosphatemia following treatment with FCM, ALP has been shown to be significantly elevated for up to 60 days, indicative of persistent hormonal perturbations [Citation77]. This raises a particular concern for the significant number of patients who require multiple treatment courses of IV iron, and for those with relatively normal renal function, such as patients with inflammatory bowel disease (IBD). Such individuals are expected to be at a greater risk of developing persistent hypophosphatemia with repeated FCM dosing, which has the potential to progress into a protracted manifestation of the 6H-syndrome. In chronic kidney disease (CKD), where the need for repeated dosing is relevant, the situation is more complex given the role of FGF23 in the development of kidney disease [Citation78]. Although hypophosphatemia following IV iron treatment, generally, occurs less frequently in patients with non-dialysis-dependent CKD (NDD-CKD) [Citation46,Citation49], iFGF23 has been reported to increase in parallel with a reduction in 1,25-dihydroxyvitamin D following FCM treatment in this population [Citation54], suggesting that certain elements of the 6H-syndrome may develop in these patients. Similarly, patients undergoing kidney transplant may represent an additional population of concern [Citation79,Citation80]. However, to date, no studies have investigated the development and clinical consequences of the 6H-syndrome in patients with reduced kidney function.
Evidence to support the real-world clinical relevance of hypophosphatemia following FCM treatment comes from a recently published retrospective study of an inpatient cohort [Citation77]. The authors report that the presence of moderate-to-severe hypophosphatemia following FCM was associated with administration of IV phosphate supplementation, and also with significantly longer hospital stays than in patients with no or mild hypophosphatemia [Citation77]. These findings suggest that there are clinically significant consequences of hypophosphatemia following IV iron treatment that go unrecognized, highlighting the need for further research and a continued effort to raise awareness of the condition.
Driven by the growing body of clinical data showing an association of FCM with the risk of hypophosphatemia and associated clinical consequences, the product label for FCM has been updated in Europe, the US, and Brazil. The updated label highlights the risk of symptomatic hypophosphatemia, and includes an instruction to monitor phosphate levels [Citation7,Citation8,Citation81,Citation82].
3.3. Cardiovascular safety
Iron is an essential micronutrient, which plays a central role in many of the body’s cellular processes [Citation83]. Iron is incorporated into the heme group of hemoglobin, which is fundamental for oxygen transport; myoglobin, which is important for oxygen storage; and cytochromes, which are important in cellular metabolism [Citation83–85]. In addition, iron is associated with non-heme moieties, such as enzymes involved in lipid metabolism, gene regulation, and DNA synthesis [Citation84,Citation85]. Consequently, iron deficiency is detrimental to various cellular processes including those of the CV system, which may benefit from iron supplementation.
In contrast, it is possible that IV iron could have a negative effect on the CV system through the production of labile iron. It has been hypothesized that an increase in labile iron induces CV damage and contributes to the progression of CV disease through the production of reactive oxygen species (ROS) [Citation26,Citation86]. As described in Section 2, the levels of labile iron vary between IV iron products [Citation4,Citation23]. However, tighter binding of the iron–carbohydrate complex in the newer products – FDI, FCM, and FER – reduces the release of labile iron compared with previous generations of IV iron [Citation4,Citation23,Citation27]. These lower levels of labile iron could, in theory, reduce the subsequent generation of toxic ROS and, potentially, alleviate the risk of CV disease and/or damage.
Data from existing clinical trials can be explored for relevant insights, which may be used to infer the potential effects of IV iron on the CV system – positive or negative – and to inform future research. Various clinical trials have addressed the CV safety of IV iron through pre-defined endpoints. Two trials of FDI – FERWON-NEPHRO and FERWON-IDA – included composite CV adverse events (adjudicated and confirmed by a blinded adjudication committee) as a secondary safety endpoint [Citation30,Citation31]. Six trials of FCM – FAIR-HF, CONFIRM-HF, EFFECT-HF, AFFIRM-AHF, REPAIR-IDA, and Onken et al. (2014) – included various CV safety endpoints, such as death and hospitalizations due to a CV event or worsening of heart failure (HF), non-fatal myocardial infarction or stroke [Citation87–92]. With the exception of AFFIRM-AHF [Citation90], none of these trials were designed or powered to evaluate CV morbidity and mortality endpoints. The aforementioned FERWON trials were conducted in patients with IDA [Citation30,Citation31]. In the dedicated HF trials, the presence of iron deficiency was a prerequisite for inclusion; however, upon further screening, some of the patients were also found to be anemic [Citation87–90].
The AFFIRM-AHF trial was designed to assess hospitalizations for HF and CV death as a composite primary endpoint [Citation90]. The trial randomized 1,132 patients hospitalized with acute HF to FCM or placebo treatment before discharge with a follow-up period of up to 52 weeks [Citation90]. The primary endpoint of HF hospitalizations and CV mortality was numerically reduced in the FCM group compared to the placebo group; however, the difference between the groups did not reach statistical significance – rate ratio (RR): 0.79 (95% confidence interval [CI]: 0.62, 1.01; p = 0.059) [Citation90]. One component of the composite primary endpoint, total HF hospitalizations, showed a statistically significant benefit for FCM (RR: 0.74 [95% CI: 0.58, 0.94]; p = 0.013), whereas there was no signal for a benefit of FCM on CV death (hazard ratio: 0.96 [95% CI: 0.70, 1.32]; p = 0.81) [Citation90]. As the COVID-19 pandemic became a potential issue for trial conduct during the time of follow-up in AFFIRM-AHF, a pre-COVID-19 sensitivity analysis was performed [Citation90]. It showed a statistically significant difference favoring FCM over placebo for the primary endpoint (RR: 0.75 [95% CI: 0.59, 0.96]; p = 0.024) [Citation90]. The authors concluded that, although it was not possible to determine the influence of COVID-19 on the treatment effect, the pandemic may have had a negative effect on patient follow-up and protocol compliance, as well as there being fewer hospitalizations [Citation90]. Such factors may mean that it was harder to observe a treatment effect [Citation90].
In addition to AFFIRM-AHF, the PIVOTAL trial has also assessed death as part of a composite primary endpoint, alongside major CV adverse events [Citation93]. The PIVOTAL trial randomized a total of 2,141 maintenance hemodialysis patients and demonstrated a benefit for a higher dose versus lower dose IV iron regime (using iron sucrose) on all-cause survival and CV outcomes [Citation93]. Patients receiving high-dose IV iron therapy were less likely to have a fatal or non-fatal myocardial infarction or to be hospitalized for HF than those who received the low-dose regime [Citation93]. Although some patients receiving the low-dose regime required supplemental iron to maintain a serum ferritin of >200 µg/L [Citation93], these patients were highly unlikely to have fulfilled the criteria for ‘iron deficiency’. It is acknowledged that the high-dose regime used in PIVOTAL (targeting ferritin of 700 µg/L [Citation93]) was not compared against contemporary UK practice, which was to maintain serum ferritin at approximately 450–500 µg/L in most dialysis units. The findings of the PIVOTAL trial support favorable CV effects with a proactive high-dose approach compared with a conservative reactive low-dose approach in this population of patients with end-stage kidney disease [Citation93]. The REVOKE trial was a relatively small single-center study comparing IV iron with oral iron on the primary outcome, progression of CKD (99 patients completed the trial) [Citation94]. Safety analysis highlighted an increased risk of CV events with IV iron versus oral iron (p = 0.033); however, the events occurred in fewer patients in the IV iron group than in the oral iron group (17 versus 19, respectively) [Citation94]. The methodology of this trial has received criticism [Citation95,Citation96].
No CV safety signal for IV iron has manifested across all trials in which IV iron was evaluated against a non-IV iron comparator, mainly in HF patients – AFFIRM-AHF, FAIR-HF and CONFIRM-HF (FCM versus placebo), EFFECT-HF (FCM versus standard of care), Onken et al. (2014) (FCM versus oral iron), and FERRIC-HF II (FDI versus placebo) [Citation87–90,Citation92,Citation97]. In AFFIRM-AHF, FCM and placebo showed a comparable rate of cardiac disorders reported as an adverse event (40% and 44%, respectively) [Citation90]. FAIR-HF reported comparable CV mortality and hospitalization data for FCM and placebo, and CONFIRM-HF reported a significant benefit for FCM over placebo on hospitalizations due to HF worsening, or death (p = 0.03) [Citation87,Citation88]. In EFFECT-HF, an equal number of patients in the FCM and standard of care groups were hospitalized for worsening of HF (13 patients in each group), whereas a higher number of hospitalizations for CV reasons other than worsening of HF was reported for FCM than for standard of care (13 and 3 hospitalizations, respectively) [Citation89]. In the FERWON-NEPHRO trial, enrolling patients with IDA and NDD-CKD, significantly fewer FDI-treated patients reported CV adverse events versus those treated with iron sucrose (4.1% versus 6.9%, respectively; p = 0.025) [Citation31]. In contrast, no significant difference between the groups was observed in the FERWON-IDA trial, enrolling patients with IDA [Citation30]. These observations suggest that the difference in CV adverse events between FDI and iron sucrose is more pronounced in a population with a higher risk of CV complications, such as patients with CKD [Citation32].
Complementing the positive CV safety profile for IV iron that has been observed in clinical trials, these investigations also signaled a beneficial effect of IV iron on other pre-specified endpoints, some of which are CV-related. For example, in AFFIRM-AHF, FCM was associated with significantly fewer days lost due to HF hospitalizations and CV death than placebo (RR: 0.67 [95% CI: 0.47, 0.97]; p = 0.035) [Citation90]. FAIR-HF and CONFIRM-HF have shown improvements for FCM over placebo on measures of functional outcome (6-minute walk test), disease status (New York Heart Association [NYHA] class), and quality of life (Kansas City Cardiomyopathy Questionnaire [KCCQ]) [Citation87,Citation88]. FERRIC-HF II has shown improvements for FDI in disease status and symptoms (NYHA class, Borg dyspnea score) [Citation97]. In contrast, EFFECT-HF reported minimal change for FCM on the primary endpoint of exercise capacity, assessed through the peak oxygen consumption (VO2), although it was noted that VO2 decreased in the standard of care arm; the difference between the two groups was significant (p = 0.020) [Citation89]. A significant improvement was observed for FCM versus the control group in NYHA class (p < 0.05) [Citation89]. Improvements in functional endpoints are suggested to be, at least partly, related to the enhancement in exercise capacity by stimulating skeletal muscle energetics, which has been demonstrated with FDI in HF patients with reduced ejection fraction (HFrEF) and iron deficiency [Citation97]. The effect of FDI on exercise capacity is being further investigated in the IronIC trial, in heart transplant patients with iron deficiency, and the IIISAS trial, in patients with severe aortic stenosis and iron deficiency [Citation98,Citation99]. How IV iron supplementation may affect the myocardium in HF patients with iron deficiency remains to be established, as does the long-term potential benefits and safety of IV iron in HF patients.
Large clinical trials are ongoing to confirm the potential benefit of IV iron in mainly non-acute HF patients – IRONMAN, FAIR-HF 2, and HEART-FID. Each of these trials has been specifically designed to assess, as the primary endpoint, the incidence of hospitalizations for HF, death, or CV death, following IV iron infusion (specific endpoints differ between trials) () [Citation100–102].
Table 2. Ongoing clinical studies of IV iron in patients with HF and iron deficiency
4. Conclusion
FDI, FCM, and FER are the newest IV iron products to enter the market. Their success in treating iron deficiency and IDA across diverse patient populations and therapeutic indications is testament to the process of research and refinement, spanning 100 years, to ensure that the modern complexes are well tolerated, and that they can be administered as high doses in short infusion times. Such benefits have positive resource implications through the need for fewer visits, which is in the interest of the patients, physicians, and payers.
This review presents the most recent evidence, from RCTs specifically designed to evaluate hypersensitivity as primary or secondary endpoints, for a low incidence of hypersensitivity reactions with FDI, FCM, and FER. These data should help to guide rational treatment decisions for iron-deficient patients. Even though severe reactions are rare, appropriate resuscitation facilities and qualified healthcare professionals must be available in settings where IV iron is administered. If infusion reactions do occur, they are usually mild, but can mimic the early symptoms of a more severe reaction. It is important that these mild reactions are managed correctly, aided by the treatment algorithms that have been developed for this purpose.
The available clinical data suggest that FCM is associated with a considerably greater risk of hypophosphatemia than FDI and FER, which can be severe, symptomatic and/or persistent. Importantly, hypophosphatemia that is induced by FCM may be just one element of a wider network of inter-related consequences, recently described as the 6H-syndrome.
As highlighted in this review, the effect of IV iron on the CV system is an area that is still undergoing investigation. Results from the first trials designed to evaluate CV outcomes in HF patients are promising, whereas long-term efficacy and safety beyond 1 year remains to be established. Such observations are supported by the well-established findings that IV iron can improve functional and symptomatic endpoints in HF patients. Several ongoing large-scale studies evaluating FDI and FCM on CV morbidity and mortality will shed further light on the potential benefit of these agents in the field of HF.
5. Expert opinion
Globally, iron deficiency is the leading cause of anemia [Citation103]. IDA is ranked among the ten most prevalent global conditions, affecting 1,200 million people, and accounting for approximately one half of the total anemia burden, worldwide [Citation103,Citation104]. Consequently, the prevention and treatment of iron deficiency and IDA is of major importance to public health. IV iron is an important treatment option for patients with iron deficiency and IDA, when oral iron is not effective or well-tolerated, or when there is a clinical need to rapidly correct anemia.
Over the last 100 years, IV iron has evolved from the early products associated with toxicity due to weakly bound iron, to the tightly-bound, highly-stable, iron–carbohydrate complexes that are available today – FDI, FCM, and FER – enabling simple, fast, and safe delivery of large doses of iron, meeting the needs of physicians and patients. Although the efficacy of IV iron is well-established, there has been a lack of robust RCTs directly comparing the safety of different IV iron products. However, this knowledge gap has been addressed in recent years – five head-to-head RCTs have been conducted, comparing the incidence of hypersensitivity reactions between the newer IV iron products – FDI, FCM, and FER – as primary or secondary endpoints. Together, these RCTs have consistently demonstrated that the newer high-dose IV iron products have a low incidence of hypersensitivity reactions that is comparable between products. Such high-quality clinical evidence should help to change the mind-set of physicians who are reluctant to use IV iron due to a long-standing fear of serious hypersensitivity reactions. In addition, tools and initiatives to educate physicians on the management of mild infusion reactions in clinical practice may also help to alleviate such fears.
Hypophosphatemia is, increasingly, being recognized as a clinically relevant side effect of FCM and recent recommendations from regulatory authorities mandate the monitoring of phosphate in at-risk patients [Citation7,Citation8,Citation81,Citation82]. Pre-existing risk factors for hypophosphatemia include vitamin D deficiency, calcium and phosphate malabsorption, secondary hyperparathyroidism, IBD, and osteoporosis [Citation8,Citation81,Citation82]. Given that the duration and severity of hypophosphatemia cannot be predicted, it would be prudent clinical practice to monitor phosphate in all patients before and after treatment with FCM. Furthermore, given the underlying mechanism of the 6H-syndrome, it is also clear that simple phosphate substitution will not sustainably correct hypophosphatemia that occurs in the context of FCM treatment [Citation68,Citation72,Citation77,Citation105]. Burosumab – a therapeutic monoclonal antibody against FGF23 [Citation106] – could represent a treatment option to mitigate the consequences of FCM-induced 6H-syndrome [Citation105]. Further research into the duration and potential prevention of hypophosphatemia after FCM is necessary before FCM can be recommended for use without concerns regarding the occurrence of hypophosphatemia.
Historically, CV safety has been considered one of the main concerns associated with IV iron, due to the potential risk of exposing patients to labile iron [Citation26,Citation86]. However, high doses of the newer formulations of IV iron have, recently, been evaluated in large trials and no acute CV safety concerns were identified [Citation30,Citation31,Citation91,Citation92]. Longer-term investigations, specifically evaluating the net clinical benefits of IV iron on CV endpoints, have been limited to patients with HF and to patients with end-stage kidney disease receiving hemodialysis [Citation87–90,Citation93]. Again, these trials have not identified any CV safety signals and, in the hemodialysis population, higher doses of IV iron have shown a benefit on CV endpoints compared with lower dose IV iron [Citation93]. Data from clinical trials of IV iron in patients with systolic HF have shown improvements in quality of life and physical performance, and the recent data from the AFFIRM-AHF trial shows a reduction in the rate of hospitalization for HF [Citation87–90]. However, CV safety and efficacy for FDI and FCM, in terms of mortality and hospitalization for HF beyond 1 year, is yet to be established in HF patients with iron deficiency. Such evidence is anticipated from the large ongoing CV outcome trials – IRONMAN, FAIR-HF, and HEART-FID [Citation100–102].
Article highlights
IV iron products have evolved from poorly tolerated formulations with limited efficacy to modern well-tolerated and highly effective drugs for the treatment of iron deficiency (e.g. in heart failure) and iron deficiency anemia (of mixed etiology).
FDI, FCM, and FER are the three newest IV iron formulations to enter the market, which can be administered in high doses in short infusion times.
The most recent evidence from randomized controlled trials shows a low incidence of hypersensitivity reactions – a long-standing concern with the use of IV iron – for FDI, FCM, and FER. Immediate infusion reactions are usually mild, and management of these events is straightforward with the appropriate training; severe reactions are extremely rare.
Hypophosphatemia is an increasingly reported side effect of FCM; recognition of this side effect and its clinical complications requires vigilance.
FCM also triggers a cascade of biochemical changes that are associated with adverse effects on bone metabolism, which may explain the long-term bone complications that have been reported. This is particularly relevant for patients with preserved kidney function, pre-existing bone disease, or malnutrition.
Evidence to support the use of IV iron to treat iron deficiency in heart failure, and to reduce hospitalizations in this population is promising. Existing evidence does not reveal an adverse cardiovascular safety signal; ongoing clinical trials will provide additional long-term safety data.
This box summarizes key points contained in the article.
Declaration of interest
I Blumenstein declares that she has received honoraria for lecturing and consultancy fees from the manufacturer of ferric derisomaltose – Pharmacosmos A/S Holbæk, Denmark.
S Shanbhag declares that he has received consulting fees from Takeda Oncology, GSK, Daiichi-Sankyo/American Regent, Kura Oncology, Celgene, and BeiGene. He has also received institutional research grant support from the manufacturer of ferric carboxymaltose – Daiichi Sankyo/American Regent.
P Langguth declares that he has received research support from Pharmacosmos A/S, Holbæk, Denmark as well as from Serumwerk Bemburg AG, and travel support from Vifor, Switzerland.
P Kalra declares that he has received honoraria for lecturing and consultancy fees from the manufacturer of ferric derisomaltose – Pharmacomos A/S, Holbæk, Denmark. He has also received research grant support and lecture fees from Vifor, Switzerland.
H Zoller declares that he has received research grants, honoraria for lecturing and consultancy fees from the manufacturer of ferric derisomaltose (Pharmacomos A/S, Holbæk, Denmark), from the distributor of ferric derisomaltose (Medice, Hallein, Austria), from the manufacturer of ferric carboxymaltose (Vifor International, Glattbrugg, Switzerland), and from the distributor of ferric carboxymaltose (Vifor Austria, Vienna, Austria).
W Lim declares that she has received honoraria for lecturing and consultancy fees from the manufacturer of ferric derisomaltose – Pharmacomos A/S, Holbæk, Denmark, and from the distributor (Pfizer Canada).
The authors have no other relevant affiliations or financial involvement with any organization or entity with a financial interest in or financial conflict with the subject matter or materials discussed in the manuscript. This includes employment, consultancies, honoraria, stock ownership or options, expert testimony, grants or patents received or pending, or royalties.
Acknowledgments
Writing and editorial support was provided by Cambridge – a Prime Global Agency, funded by Pharmacosmos A/S.
Correction Statement
This article has been republished with minor changes. These changes do not impact the academic content of the article.
Additional information
Funding
References
- Auerbach M, Ballard H. Clinical use of intravenous iron: administration, efficacy, and safety. Hematology Am Soc Hematol Educ Program. 2010;2010(1):338–347.
- Auerbach M, DeLoughery T. Single-dose intravenous iron for iron deficiency: a new paradigm. Hematology Am Soc Hematol Educ Program. 2016;2016(1):57–66.
- Auerbach M, Coyne D, Ballard H. Intravenous iron: from anathema to standard of care. Am J Hematol. 2008;83(7):580–588.
- Jahn MR, Andreasen HB, Fütterer S, et al. A comparative study of the physicochemical properties of iron isomaltoside 1000 (Monofer®), a new intravenous iron preparation and its clinical implications. Eur J Pharm Biopharm. 2011;78(3):480–491.
- Monofer (ferric derisomaltose). Summary of product characteristics. Pharmacosmos UK Ltd; Sep 2020.
- Monoferric (ferric derisomaltose). Product information. Pharmacosmos A/S; Jul 2020.
- Ferinject (ferric carboxymaltose). Summary of product characteristics. Vifor Pharma UK Ltd; Nov 2020.
- Injectafer (ferric carboxymaltose). Prescribing information. Vifor International, Inc; Sep 2020.
- Feraheme (ferumoxytol). Prescribing information. AMAG Pharmaceuticals, Inc; Sep 2020.
- European Medicines Agency. Public statement. Rienso: withdrawal of the marketing authorisation in the European Union. EMA/437901/2015; EMEA/H/C/2215. 10 Jul 2015. [cited 2021 Jan 27]. Available from: https://www.ema.europa.eu/en/documents/public-statement/public-statement-rienso-ferumoxytol-withdrawal-marketing-authorisation-european-union_en.pdf
- Kalra PA, Bhandari S. Safety of intravenous iron use in chronic kidney disease. Curr Opin Nephrol Hypertens. 2016;25(6):529–535.
- Danielson BG. Structure, chemistry, and pharmacokinetics of intravenous iron agents. J Am Soc Nephrol. 2004;15(Suppl 2):S93–S98.
- Rottembourg J. The non-biologic-complex-drug concept. Int J Biopharm Sci. 2018;1(1):104.
- Borchard G, Flühmann B, Mühlebach S. Nanoparticle iron medicinal products – Requirements for approval of intended copies of non-biological complex drugs (NBCD) and the importance of clinical comparative studies. Regul Toxicol Pharmacol. 2012;64(2):324–328.
- WO 2007/081744 A2 – Methods and compositions for administration of iron. International Application No. PCT/US2007/000176. International filing date: 2007 Jan 8; [cited 2021 Jan 27]. Available from: https://patents.google.com/patent/WO2007081744A2/en.
- US 9,376,505 B2 – Aqueous iron carbohydrate complexes, their production and medicaments containing them. Application No. 13/556,733. International filing date: 2012 Jul 24; [cited 2021 Jan 27]. Available from: https://patents.google.com/patent/US9376505B2/en.
- Sigma-Aldrich. Product Specification. Maltodextrin 4.0–7.0. Product Number: 419672. [cited 2020 Sep 17]. Available from: https://www.sigmaaldrich.com/catalog/substance/maltodextrin12345905036611?lang=en®ion=GB.
- US 8,501,158 B2 – Polyol and polyether iron oxide complexes as pharmacological and/or MRI contrast agents. Application No. 12/963,308. Filing date: 2010 Dec 8; [cited 2021 Jan 27]. Available from: https://patents.google.com/patent/US8501158B2/en.
- US 8,895,612 B2 – Methods and compositions for administration of iron. Application No. 14/100,717. Filling date: 2013 Dec 9; [cited 2021 Jan 27]. Available from: https://patents.google.com/patent/US8895612B2/en.
- Neiser S, Rentsch D, Dippon U, et al. Physico-chemical properties of the new generation IV iron preparations ferumoxytol, iron isomaltoside 1000 and ferric carboxymaltose. Biometals. 2015;28(4):615–635.
- British Pharmacopoeia 2017. Volume III. Jan 2017.
- De Belder AN. Dextran. © Amersham Biosciences AB 2003.
- Fütterer S, Andrusenko I, Kolb U, et al. Structural characterization of iron oxide/hydroxide nanoparticles in nine different parenteral drugs for the treatment of iron deficiency anaemia by electron diffraction (ED) and X-ray powder diffraction (XRPD). J Pharm Biomed Anal. 2013;86:151–160.
- Nikravesh N, Borchard G, Hofmann H, et al. Factors influencing safety and efficacy of intravenous iron-carbohydrate nanomedicines: from production to clinical practice. Nanomedicine. 2020;26:102178.
- Sidhu PS, Gilkes RJ, Cornell RM, et al. Dissolution of iron oxides and oxyhydroxides in hydrochloric and perchloric acids. Clays Clay Miner. 1988;29(4):269–276.
- Kruszewski M. Labile iron pool: the main determinant of cellular response to oxidative stress. Mutat Res. 2003;531(1–2):81–92.
- Garbowski MW, Bansal S, Porter JB, et al. Intravenous iron preparations transiently generate non-transferrin-bound iron from two proposed pathways. Haematologica. 2020.
- Wolf M, Rubin J, Achebe M, et al. Effects of iron isomaltoside vs ferric carboxymaltose on hypophosphatemia in iron-deficiency anemia: two randomized clinical trials. JAMA. 2020;323(5):432–443.
- Adkinson NF, Strauss WE, Macdougall IC, et al. Comparative safety of intravenous ferumoxytol versus ferric carboxymaltose in iron deficiency anemia: a randomized trial. Am J Hematol. 2018;93(5):683–690.
- Auerbach M, Henry D, Derman RJ, et al. A prospective, multi-center, randomized comparison of iron isomaltoside 1000 versus iron sucrose in patients with iron deficiency anemia; the FERWON-IDA trial. Am J Hematol. 2019;94(9):1007–1014.
- Bhandari S, Kalra PA, Berkowitz M, et al. Safety and efficacy of iron isomaltoside 1000/ferric derisomaltose versus iron sucrose in patients with chronic kidney disease: the FERWON-NEPHRO randomized, open-label, comparative trial. Nephrol Dial Transplant. 2021;36(1):111–120.
- Wolf M, Auerbach M, Kalra PA, et al. Safety of ferric derisomaltose and iron sucrose in patients with iron deficiency anemia: the FERWON-IDA/NEPHRO trials. Am J Hematol. 2021;96(1):E11–E15.
- Pollock RF, Biggar P. Indirect methods of comparison of the safety of ferric derisomaltose, iron sucrose and ferric carboxymaltose in the treatment of iron deficiency anemia. Expert Rev Hematol. 2020;13(2):187–195.
- Wysowski DK, Swartz L, Borders-Hemphill BV, et al. Use of parenteral iron products and serious anaphylactic-type reactions. Am J Hematol. 2010;85(9):650–654.
- European Medicines Agency (EMA). Rapid response to BMJ. Re: pandemrix vaccine: why was the public not told of early warning signs. EMA/659264/2018. 2018 [cited 2021] Jan 27. Available from: https://www.ema.europa.eu/en/documents/other/european-medicines-agency-rapid-response-british-medical-journal-pandemrix_.pdf
- OCEBM Levels of evidence working group. The Oxford 2011 Levels of Evidence.
- Rampton D, Folkersen J, Fishbane S, et al. Hypersensitivity reactions to intravenous iron: guidance for risk minimization and management. Haematologica. 2014;99(11):1671–1676.
- Lim W, Afif W, Knowles S, et al. Canadian expert consensus: management of hypersensitivity reactions to intravenous iron in adults. Vox Sang. 2019;114(4):363–373.
- Achebe M, DeLoughery TG. Clinical data for intravenous iron – debunking the hype around hypersensitivity. Transfusion. 2020;60(6):1154–1159.
- Macdougall IC, Bircher AJ, Eckardt KU, et al. Iron management in chronic kidney disease: conclusions from a “Kidney Disease: Improving Global Outcomes” (KDIGO) controversies conference. Kidney Int. 2016;89(1):28–39.
- Macdougall IC, Vernon K. Complement activation-related pseudo-allergy: a fresh look at hypersensitivity reactions to intravenous iron. Am J Nephrol. 2017;45(1):60–62.
- Szebeni J, Fishbane S, Hedenus M, et al. Hypersensitivity to intravenous iron: classification, terminology, mechanisms and management. Br J Pharmacol. 2015;172(21):5025–5036.
- Gómez-Ramírez S, Shander A, Spahn DR, et al. Prevention and management of acute reactions to intravenous iron in surgical patients. Blood Transfus. 2019;17(2):137–145.
- Zoller H, Schaefer B, Glodny B. Iron-induced hypophosphatemia: an emerging complication. Curr Opin Nephrol Hypertens. 2017;26(4):266–275.
- Schaefer B, Meindl E, Wagner S, et al. Intravenous iron supplementation therapy. Mol Aspects Med. 2020;75:100862.
- Schaefer B, Tobiasch M, Viveiros A, et al. Hypophosphatemia after treatment of iron deficiency with intravenous ferric carboxymaltose or iron isomaltoside – a systematic review and meta‐analysis. Br J Clin Pharmacol. 2020.
- Glaspy JA, Lim-Watson MZ, Libre MA, et al. Hypophosphatemia associated with intravenous iron therapies for iron deficiency anemia: a systematic literature review. Ther Clin Risk Manag. 2020;16:245–259.
- Wolf M, Koch TA, Bregman DB. Effects of iron deficiency anemia and its treatment on fibroblast growth factor 23 and phosphate homeostasis in women. J Bone Miner Res. 2013;28(8):1793–1803.
- Wolf M, Chertow GM, Macdougall IC, et al. Randomized trial of intravenous iron-induced hypophosphatemia. JCI Insight. 2018;3(23):e124486.
- Wolf M, Schaefer B, Zoller H. Persistent hypophosphatemia after ferric carboxymaltose is associated with persistent changes in biomarkers of bone metabolism. Blood. 2020;136(Suppl 1):13–14.
- Iqbal TH, Wolf M, Schaffalitzky de Muckadell P, et al. PHOSPHARE studies: important changes in phosphate homeostasis and bone metabolism after IV iron. Poster presented at the British Society of Gastroenterology (BSG) Annual Meeting, Glasgow, 17–20 Jun, 2019.
- Emrich IE, Lizzi F, Siegel JD, et al. Hypophosphatemia after high-dose iron repletion with ferric carboxymaltose and ferric derisomaltose – the randomized controlled HOMe aFers study. BMC Med. 2020;18(1):178.
- Detlie TE, Lindstrøm JC, Jahnsen ME, et al. Incidence of hypophosphatemia in patients with inflammatory bowel disease treated with ferric carboxymaltose or iron isomaltoside. Aliment Pharmacol Ther. 2019;50(4):397–406.
- Huang LL, Lee D, Troster SM, et al. A controlled study of the effects of ferric carboxymaltose on bone and haematinic biomarkers in chronic kidney disease and pregnancy. Nephrol Dial Transplant. 2018;33(9):1628–1635.
- Stöhr R, Sandstede L, Heine GH, et al. High-dose ferric carboxymaltose in patients with HFrEF induces significant hypophosphatemia. J Am Coll Cardiol. 2018;71(19):2270–2271.
- Fang W, Kenny R, Rizvi QU, et al. Hypophosphataemia after ferric carboxymaltose is unrelated to symptoms, intestinal inflammation or vitamin D status. BMC Gastroenterol. 2020;20(1):183.
- Amanzadeh J, Reilly RF Jr. Hypophosphatemia: an evidence-based approach to its clinical consequences and management. Nat Clin Pract Nephrol. 2006;2(3):136–148.
- Geerse DA, Bindels AJ, Kuiper MA, et al. Treatment of hypophosphatemia in the intensive care unit: a review. Crit Care. 2010;14(4):R147.
- Gravelyn TR, Brophy N, Siegert C, et al. Hypophosphatemia-associated respiratory muscle weakness in a general inpatient population. Am J Med. 1988;84(5):870–876.
- Fukumoto S. Phosphate metabolism and vitamin D. Bonekey Rep. 2014;3:497.
- Hardy S, Vandemergel X. Intravenous iron administration and hypophosphatemia in clinical practice. Int J Rheumatol. 2015;2015:468675.
- Imel EA, Econs MJ. Approach to the hypophosphatemic patient. J Clin Endocrinol Metab. 2012;97(3):696–706.
- Pasquali M, Tartaglione L, Rotondi S, et al. Calcitriol/calcifediol ratio: an indicator of vitamin D hydroxylation efficiency? BBA Clin. 2015;3:251–256.
- Penido MG, Alon US. Phosphate homeostasis and its role in bone health. Pediatr Nephrol. 2012;27(11):2039–2048.
- Prié D, Ureña Torres P, Friedlander G. Latest findings in phosphate homeostasis. Kidney Int. 2009;75(9):882–889.
- Schaefer B, Glodny B, Zoller H. Blood and bone loser. Gastroenterology. 2017;152(6):e5–e6.
- Zhao Y, Li Z, Shi Y, et al. Effect of hypophosphatemia on the withdrawal of mechanical ventilation in patients with acute exacerbations of chronic obstructive pulmonary disease. Biomed Rep. 2016;4(4):413–416.
- Blazevic A, Hunze J, Boots JMM. Severe hypophosphataemia after intravenous iron administration. Neth J Med. 2014;72(1):49–53.
- Fierz YC, Kenmeni R, Gonthier A, et al. Severe and prolonged hypophosphatemia after intravenous iron administration in a malnourished patient. Eur J Clin Nutr. 2014;68(4):531–533.
- Anand G, Schmid C. Severe hypophosphataemia after intravenous iron administration. BMJ Case Rep. 2017;2017:bcr2016219160.
- Ifie E, Oyibo SO, Joshi H, et al. Symptomatic hypophosphataemia after intravenous iron therapy: an underrated adverse reaction. Endocrinol Diabetes Metab Case Rep. 2019;2019(1):19-0065.
- Harris RE, Armstrong L, Curtis L, et al. Severe hypophosphataemia following ferric carboxymaltose infusion in paediatric patients with inflammatory bowel disease. Frontline Gastroenterol. 2019;11(4):324–326.
- Vasquez-Rios G, Chapel A, Philip I, et al. Life-threatening hypophosphatemia following intravenous iron infusion. Nefrologia. 2020;S0211-6995(20):30071-0.
- Bart G, Glemarec J, Lerhun M, et al. A rusty man … or how iron can be responsible for bone pain. Symptomatic hypophosphataemic osteomalacia after iron carboxymaltose treatment. The Rheumatologist’s Newsletter. No. 428;2017.
- Burckhardt P. Iron-induced osteomalacia. Osteologie. 2018;27(1):20–23.
- Klein K, Asaad S, Econs M, et al. Severe FGF23-based hypophosphataemic osteomalacia due to ferric carboxymaltose administration. BMJ Case Rep. 2018. DOI: https://doi.org/10.1136/bcr-2017-222851.
- Fragkos KC, Sehgal V, Rogers J, et al. Hypophosphataemia after intravenous iron therapy with ferric carboxymaltose – real world experience from a tertiary centre in the UK. GastroHep. 2020;2(5):205–214.
- Musgrove J, Wolf M. Regulation and effects of FGF23 in chronic kidney disease. Annu Rev Physiol. 2020;82(1):365–390.
- Baia LC, Heilberg IP, Navis G, et al. NIGRAM investigators. Phosphate and FGF-23 homeostasis after kidney transplantation. Nat Rev Nephrol. 2015;11(11):656–666.
- Sari V, Atiqi R, Hoorn EJ, et al. Ferric carboxymaltose-induced hypophosphataemia after kidney transplantation. Neth J Med. 2017;75(2):65–73.
- Ferinject (ferric carboxymaltose). Prescribing information [Brazil]. Vifor (International) Inc; Sep 2020.
- GOV.UK. Drug safety update. Ferric carboxymaltose (Ferinject): risk of symptomatic hypophosphataemia leading to osteomalacia and fractures. [cited 2020 Nov 16]. Available from: https://www.gov.uk/drug-safety-update/ferric-carboxymaltose-ferinject-risk-of-symptomatic-hypophosphataemia-leading-to-osteomalacia-and-fractures.
- Kang CK, Pope M, Lang CC, et al. Iron deficiency in heart failure: efficacy and safety of intravenous iron therapy. Cardiovasc Ther. 2017;35(6):e12301.
- Cairo G, Bernuzzi F, Recalcati S. A precious metal: iron, an essential nutrient for all cells. Genes Nutr. 2006;1(1):25–39.
- Anderson GJ, Vulpe CD. Mammalian iron transport. Cell Mol Life Sci. 2009;66(20):3241–3261.
- Panth N, Paudel KR, Parajuli K. Reactive oxygen species: a key hallmark of cardiovascular disease. Adv Med. 2016;2016:9152732.
- Anker SD, Comin Colet J, Filippatos G, et al. Ferric carboxymaltose in patients with heart failure and iron deficiency. N Engl J Med. 2009;361(25):2436–2448.
- Ponikowski P, Van Veldhuisen DJ, Comin-Colet J, et al. CONFIRM-HF investigators. Beneficial effects of long-term intravenous iron therapy with ferric carboxymaltose in patients with symptomatic heart failure and iron deficiency. Eur Heart J. 2015;36(11):657–668.
- Van Veldhuisen DJ, Ponikowski P, Van Der Meer P, et al. Effect of ferric carboxymaltose on exercise capacity in patients with chronic heart failure and iron deficiency. Circulation. 2017;136(15):1374–1383.
- Ponikowski P, Kirwan B-A, Anker SD, et al. Ferric carboxymaltose for iron deficiency at discharge after acute heart failure: a multicentre, double-blind, randomised, controlled trial. Lancet. 2020;396(10266):1895–1904.
- Onken JE, Bregman DB, Harrington RA, et al. Ferric carboxymaltose in patients with iron-deficiency anemia and impaired renal function: the REPAIR-IDA trial. Nephrol Dial Transplant. 2014;29(4):833–842.
- Onken JE, Bregman DB, Harrington RA, et al. A multicenter, randomized, active-controlled study to investigate the efficacy and safety of intravenous ferric carboxymaltose in patients with iron deficiency anemia. Transfusion. 2014;54(2):306–315.
- Macdougall IC, White C, Anker SD, et al. Intravenous iron in patients undergoing maintenance hemodialysis. N Engl J Med. 2019;380(5):447–458.
- Agarwal R, Kusek JW, Pappas MK. A randomized trial of intravenous and oral iron in chronic kidney disease. Kidney Int. 2015;88(4):905–914.
- Bhandari S, Kalra PA, Coyne DW. Data confusion. Kidney Int. 2015;88(6):1445.
- Macdougall IC, Roger SD. New data on the safety of IV iron – but why the discrepancy with FIND-CKD? Kidney Int. 2015;88(6):1445–1446.
- Charles-Edwards G, Amaral N, Sleigh A, et al. Effect of iron isomaltoside on skeletal muscle energetics in patients with chronic heart failure and iron deficiency. Circulation. 2019;139(21):2386–2398.
- ClinicalTrials.gov. Intravenous iron supplement for iron deficiency in cardiac transplant recipients (IronIC). NCT03662789. 2 Mar 2020.
- ClinicalTrials.gov. Intravenous iron supplement for iron deficiency in patients with severe aortic stenosis (IIISAS). NCT04206228. 3 Apr 2020.
- ClinicalTrials.gov. Intravenous iron treatment in patients with heart failure and iron deficiency: IRONMAN (IRONMAN). NCT02642562. 6 Oct 2020.
- ClinicalTrials.gov. Intravenous iron in patients with systolic heart failure and iron deficiency to improve morbidity & mortality (FAIR-HF2). NCT03036462. 8 May 2020.
- ClinicalTrials.gov. Randomized placebo-controlled trial of FCM as treatment for heart failure with iron deficiency (HEART-FID). NCT03037931. 3 Nov 2020.
- Kassebaum NJ, Jasrasaria R, Naghavi M, et al. A systematic analysis of global anemia burden from 1990 to 2010. Blood. 2014;123(5):615–624.
- Global Burden of Disease (GBD) 2016. Disease and injury incidence and prevalence collaborators. Global, regional, and national incidence, prevalence, and years lived with disability for 328 diseases and injuries for 195 countries, 1990–2016: a systematic analysis for the Global Burden of Disease Study 2016. Lancet. 2017;390(10100):1211–1259.
- Amarnani R, Travis S, Javaid MK. Novel use of burosumab in refractory iron-induced FGF23-mediated hypophosphataemic osteomalacia. Rheumatology (Oxford). 2020;59(8):2166–2168.
- CRYSVITA (burosumab). Summary of product characteristics. [cited 2020 Nov 4]. Available from: https://www.ema.europa.eu/en/documents/product-information/crysvita-epar-product-information_en.pdf