ABSTRACT
Background
Current clinical trial data on PARP inhibitors (PARPis)-related acute renal failure (ARF) are not entirely representative of real-world situations. Therefore, in this study, the US Food and Drug Administration Adverse Event Reporting System (FAERS) was used to evaluate PARPis-related ARF.
Research design and methods
Data were obtained from 1 January 2015, to 30 September 2023. ARF event reports were analyzed based on four algorithms. The time-to-onset (TTO) and clinical outcomes of PARPis-associated ARF were assessed.
Results
The total included cases were 2726. Significant signals were observed for olaparib, niraparib, and rucaparib (reporting odds ratio (ROR): 1.62, 95% confidence interval (CI): 1.49–1.78, 1.25, 95% CI: 1.19–1.32 and 1.59, 95% CI: 1.47–1.72 respectively). The median TTO of ARF onset was 57, 36, and 85 days for olaparib, niraparib, and rucaparib, respectively. The proportion of deaths with olaparib (9.88%) was significantly higher than for niraparib (2.52%) and rucaparib (2.94%) (p < 0.005). The proportion of life-threatening adverse events associated with niraparib (4.89%) was significantly higher than for rucaparib (0.98%) (p < 0.005).
Conclusions
ARF and PARPi were related, with the exception of talazoparib. More emphasis should be given to PARPis-related ARF due to the high proportion of serious AEs and delayed adverse reactions.
1. Introduction
Cancer is the leading cause of death worldwide and a significant barrier to increasing life expectancy [Citation1]. Poly (ADP-ribose) polymerases (PARPs) are enzymes that are essential for DNA damage repair that identify and repair single-strand breaks [Citation2]. The repair of DNA double strand breaks (DSBs) by BRCA1 and BRCA2 occurs through the homologous recombination (HR) repair pathway, which is a significant role for these two tumor suppressors [Citation3]. PARP inhibitors (PARPis) have been proven to target tumor cells with HR pathway defects, leading to synthetic lethality in these cells [Citation4]. HR and non-homologous end joining (NHEJ) are the primary ways to repair DSBs [Citation5]. HR deficiency is usually corrected by NHEJ, a repair system that is error-prone and results in accumulation of genomic instability and ultimately cancer cell death [Citation6,Citation7]. NHEJ happens quicker than HR and tends to happen during the G1 phase. NHEJ is believed to function throughout the cell cycle. Apart from the proteins already known (Ku70/80, DNA-PKcs, Artemis, DNA pol λ/μ, DNA ligase IV-XRCC4, and XLF), new proteins such as PAXX, MRI/CYREN, TARDBP of TDP-43, IFFO1, ERCC6L2, and RNase H2 are involved in NHEJ. MRI/CYREN has a dual function, both stimulating NHEJ in the G1 phase of the cell cycle and inhibiting the pathway in the S and G2 phases [Citation8]. In the past decade, PARP inhibitors have become a new focus in cancer treatment [Citation9]. The genomic landscape of several malignancies is now better understood due to large-scale sequencing efforts. In newly diagnosed prostate cancer, germline BRCA mutations occur at an incidence of 1.2–2%. The risk of developing prostate cancer is 4-fold and 8-fold for BRCA1/2 carriers, respectively [Citation10]. In high-grade serous ovarian cancer (OC), germline BRCA1/2 is responsible for 22.6% of mutations [Citation11]. The discovery of DNA deficiency offered a strong theoretical foundation for the development of PARPis.
The US Food and Drug Administration (FDA) and European Medicines Agency (EMA) have granted approval of four PARP inhibitors: olaparib, rucaparib, niraparib, and talazoparib. Olaparib, niraparib, and rucaparib have a potency that is approximately 100 times greater than veliparib, while talazoparib has the highest trapping power [Citation12]. In 2014, olaparib was approved for use as maintenance therapy in platinum-sensitive advanced OC characterized by germline mutations in the DNA repair gene BRCA1/2. In 2016, rucaparib was approved as a therapy for advanced OC with both germline and somatic BRCA1/2 mutations. Furthermore, olaparib, rucaparib, and niraparib were approved as maintenance treatments for recurrent epithelial ovarian, fallopian tube, or primary peritoneal cancers in 2017 and 2018, respectively. In 2018, olaparib and talazoparib were approved for the treatment of human epidermal growth factor receptor type 2 negative locally advanced or metastatic breast cancer in individuals with germline BRCA1/2 mutations [Citation13].
The renal pathway is a route of excretion of PARP inhibitors [Citation14]. The elimination of olaparib and niraparib involves both renal and other pathways [Citation15]. The elimination of olaparib and its metabolites occurs through both the renal and hepatic pathways, with approximately 44% removal in urine and 42% in the feces [Citation16]. Approximately 47.5% of niraparib is eliminated via urinary excretion and 38.8% via the feces [Citation14]. Talazoparib is primarily excreted through the urine, with 68.7% of the dose eliminated in urine and 19.7% in the feces [Citation14,Citation17]. In contrast, rucaparib is primarily excreted through the feces (17.4% of the dose is eliminated in the urine and 71.9% in the feces) [Citation14].
The use of PARPis can lead to an increase in blood creatinine levels, as observed with olaparib in advanced ovarian cancer [Citation18]. In a phase III trial of niraparib maintenance therapy for advanced ovarian cancer, the incidence of any grade of elevated blood creatinine levels was 17.6% versus 3.9% in the placebo group [Citation19]. In the ARIEL3 trial, a 15% increase in creatinine (of any grade) was observed in the rucaparib group compared to 2% in the placebo group [Citation20]. As a result, increased serum creatinine levels can cause a decline in the glomerular filtration rate (GFR) or renal failure.
Most current research suggests that elevated creatinine levels are more likely caused by the inhibition of renal transporters than by actual kidney injury [Citation21,Citation22]. Clinical trial data with strict inclusion criteria and cohorts with limited sample sizes may not accurately reflect the reality. Updating our understanding and providing a general overview of the risks and characteristics of acute renal failure (ARF) after PARPi treatment are crucial for its prevention and management. Therefore, we sought to assess and compare the relationship between different PARPi therapies and ARF in a large population using the FDA Adverse Event Reporting System (FAERS). We also assessed the time-to-onset (TTO), death, life-threatening adverse events (AEs), and hospitalization associated with ARF related to various PARPi drugs.
2. Methods
2.1. Data source
This retrospective pharmacovigilance study was conducted using the FAERS database [Citation23]. The FAERS database consists of AE reports from health professionals, patients, and manufacturers worldwide. These data are available publicly. PARPis data were extracted from the FAERS database between the first quarter (Q1) of 2015 and the third quarter (Q3) of 2023.
2.2. Procedures
Data on ARF were obtained from the REAC files according to the Medical Dictionary for Regulatory Activities (MedDRA, version 23.0) at the preferred term (PT) level based on ‘acute renal failure’ for the Standardized MedDRA Query 20000003 [Citation24]. PARPis included olaparib (Lynparza), niraparib (Zejula/Akeegat), rucaparib (Rubraca), and talazoparib (Talzenna). Generic names were used to record all drugs in the DRUG file, and the role_code chosen was either ‘PS’ (primary suspect) or ‘SS’ (secondary suspect). To remove duplicate reports, the last FDA_DT was selected when the CASEID was identical, and the PRIMARY_ID with the greater value was selected when the CASEID and FDA_DT were identical, as directed in the FAERS user instructions [Citation23]. The clinical features of patients with PARPis-associated ARF were synthesized using descriptive analyses of the data collected from the FAERS database. We analyzed the TTO and ARF outcomes for the various PARPis.
2.3. Statistical analysis
Based on the core principles of Bayesian and non-proportional analyses, we used the reporting odds ratio (ROR), proportional reporting ratio (PRR), Bayesian confidence propagation neural network (BCPNN), and multi-item gamma poisson shrinker (MGPS) algorithms. Our objective was to investigate the signals associated with suspected AEs. The ROR signal was considered significant when the lower limit of the 95% confidence interval (CI) (ROR025) exceeded 1 and at least three cases were reported. For the PRR, the signal was considered significant when PRR ≥ 2, chi-squared ≥4, and a minimum of three cases were reported. The information component (IC) was considered significant when the IC025 value (lower bound of the 95% CI) >0. For the MGPS, the signal was considered noteworthy if the number of reported cases is > 0 and the lower 90% one-sided CI of EBGM (EB05) ≥2. The presence of a significant signal suggests that a particular drug may be responsible for the adverse reactions. Categorical variables were analyzed using the chi-square test for single comparisons and the Kruskal – Wallis test for comparisons of multiple independent samples [Citation25]. Statistical significance was set at p < 0.05, accompanied by 95% CIs. All statistical analyses were conducted using SPSS software (version 22.0; IBM Corp., Armonk, NY, USA).
3. Results
3.1. Descriptive analysis
The FAERS database extracted 44,772,516 reports from January 2015 to September 2023, and 35,703,831 reports were included in the final analysis. Of these, 195,267 reports on PARPis and 2,726 cases of ARF associated with PARPis as the suspected drug were identified, as shown in . The clinical features of the patients are summarized in . The average age of the patients was 67 years (interquartile range (IQR): 60–73 years). The percentage of female patients (71.67%) was much higher than that of male patients (3.71%). The percentage of reports from health professionals was slightly higher than that from non-health professionals (40.84% vs. 37.97%). North American states had the highest number of reports (69.77%).
Figure 1. Process of selecting cases of acute renal failure (ARF) associated with the use of PARPis ((Poly (ADP-ribose) polymerase inhibitors) from the US Food and Drug Administration Adverse Event Reporting System (FAERS) database.
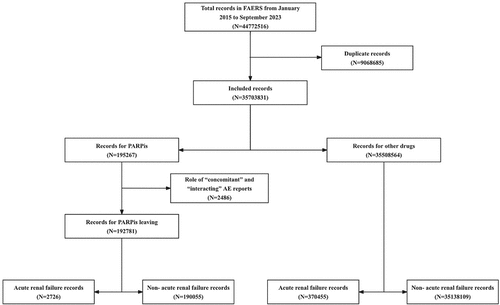
Table 1. Characteristics of patients with acute renal failure (ARF) associated with PARP inhibitor (PARPi) use in the FAERS database (January 1, 2015, to September 30, 2023).
3.2. Signal values associated with PARPis
The signal values of ARF associated with PARPis are shown in . Statistically significant ARF signals were observed in relation to total PARPis (ROR 1.36, 95% CI: 1.31–1.42, IC025: 0.38). Except for talazoparib, ARF signals for the three other PARPis were significant (olaparib, ROR: 1.62, 95% CI: 1.49–1.78, IC025:0.55; niraparib, ROR: 1.25, 95% CI: 1.19–1.32, IC025: 0.24; for rucaparib, ROR: 1.59, 95% CI: 1.47–1.72, IC025:0.54). Furthermore, olaparib and rucaparib showed higher signal values than niraparib.
Table 2. Associations of acute renal failure (ARF) with different PARP inhibitors (PARPis).
3.3. The signal spectrum of ARF in PARPis therapy
ARF signals were detected after different PARPi therapies (). Although many disproportionality signals were detected, this study focused mainly on the three most significant disproportionality signals (based on the ROR025 value) for each PARPi product. For olaparib, seven disproportionality signals were significant, including decreased creatinine renal clearance (ROR025: 3.75), increased blood creatinine (ROR025: 3.53), and renal impairment (ROR025: 3.07). For niraparib, 10 disproportionality signals were significant, including abnormal renal function test results (ROR025: 4.81), decreased GFR (ROR025: 3.88), and increased blood creatinine levels (ROR025: 3.88). For rucaparib, seven disproportionality signals were significant, including abnormal renal function test results (ROR025: 25.43), increased blood creatinine level (ROR025: 6.11), and decreased GFR (ROR025: 3.35). Our study showed that rucaparib had an extremely strong abnormal renal function test value (ROR025: 25.43), followed by niraparib (ROR025: 4.81), and olaparib (ROR025: 1.07).
3.4. TTO of PARPis-associated ARF
The TTO of ARF for each PARPi is shown in . The median TTO for ARF was 57 days (IQR: 16–255 days) for olaparib, 36 days (IQR: 13–178 days) for niraparib, and 85 days (IQR: 28–156 days) for rucaparib. The median TTO of ARF for all PARPis was 42 days (IQR: 14–167 days). The highest ARF percentages for olaparib and niraparib were observed within 10 days (14.57% and 21.64%, respectively). Between 61 and 90 days saw the highest percentage of rucaparib use, accounting for 28.57%.
3.5. Death, life-threatening AEs, and hospitalization due to ARF associated with PARPis
We evaluated the prognosis of ARF-associated PARPis by assessing the proportions of deaths, life-threatening AEs, and hospitalizations caused by ARF following the use of different PARPis. The results are shown in . In all reported cases, ARF caused death, life-threatening AEs, and hospitalization in 4.27%, 3.57%, and 19.80% of patients, respectively. The proportion of deaths among patients with ARF associated with olaparib (9.88%) was significantly higher than that reported for niraparib (2.52%) and rucaparib (2.94%) (p < 0.005, chi-squared test). The proportion of life-threatening AEs associated with niraparib (4.89%) was significantly higher than that reported for rucaparib (0.98%) (p < 0.005, chi-squared test). The proportion of hospitalizations was 18.59% for olaparib, 18.32% for niraparib, and 23.29% for rucaparib, with no statistical significance (p = 0.051, chi-square test).
4. Discussion
Our study is the first and the most comprehensive collection of information to assess and compare the relationship between PARPi therapies and ARF in a real-world setting. The FAERS pharmacovigilance database provided data for our assessment in a large population, which is different from clinical trials data. This study demonstrated a relationship between PARPis and ARF and revealed the characteristics linked to different PARPi therapies.
OC has a high death-to-incidence ratio, making it the most deadly form of gynecologic cancer. The treatment landscape has been transformed by PARPis (olaparib, niraparib, and rucaparib), which have been extensively studied in OC [Citation26]. Olaparib and niraparib was approved as first-line maintenance monotherapy in the first-line setting. Olaparib plus bevacizumab also provides benefit in patients with newly diagnosed advanced OC for homologous recombination deficiency (HRD) positive. Clinical trial data support the use of PARPis (olaparib, niraparib, rucaparib) as second-line or later maintenance therapy in patients with platinum-sensitive relapsed OC [Citation27]. The higher benefit is experienced by BRCA mutated patients; an intermediate benefit is given to HRD/BRCA wild type [Citation28]. However, new combinations of PARP inhibitors and different drugs, such as antiangiogenic medication and immune checkpoint inhibitors, were explored to overcome drug resistance and improve the effect of patients without a BRCA mutation [Citation27]. In recent years, many steps forward have been made to fill the gap in the screening tests for the diagnosis of OC. The combination of CA125 and HE4 was supposed to be the most efficient biological diagnostic tool to diagnose OC. The use of circulating tumor DNA detection can be applied for the early diagnosis and staging of cancer, evaluating tumor efficacy, monitoring tumor recurrence, and evaluating prognosis [Citation29].
Creatinine is an endogenous product of creatine metabolism in the muscles and is primarily filtered through the glomeruli; however, a significant portion of creatinine clearance is attributed to active tubular secretion facilitated by drug transporters [Citation30]. In clinical practice, creatinine is used as a marker to determine the GFR and as a functional parameter to determine renal function in patients [Citation31]. Drugs may interfere with laboratory tests, which can impact clinical decision-making. An increase in the serum creatinine concentration observed after the administration of an investigational drug could raise concerns about potential drug-induced renal impairment. This might prompt a decision to either decrease the clinical dose or halt the drug development program [Citation32].
Membrane-bound proteins, also known as transporters, move substrates across biological membranes and transport drugs [Citation33]. In general, transporters are categorized into solute carrier (SLC) and ATP-binding cassette (ABC) families [Citation34]. SLC transporters are primarily responsible for the uptake of smaller molecules into the cells, whereas ABC transporters use energy from ATP hydrolysis for efflux transport [Citation35]. SLC transporters include organic anion transporters (OATs), organic anion transporting polypeptides (OATPs), organic cation transporters (OCTs), organic cations and carnitine transporters (OCTNs), peptide transporters (PEPTs) and multidrug and toxin extrusion proteins (MATEs). ABC families that have been extensively studied include P-glycoproteins (P-gp), multi-drug resistance proteins (MRPs), and breast cancer resistance proteins (BCRPs) [Citation36]. Multiple SLC transporters in the kidney mediate the active tubular secretion of organic compounds, including drugs, toxins, and endogenous compounds [Citation37]. OCT2 is predominantly expressed in the proximal kidney tubule cells and is typically recognized as a renal uptake transporter. It plays a key role in mediating the uptake of substrates into the kidneys and facilitates their excretion into the urine [Citation38]. OCTN1 is highly expressed in the kidneys, and in smaller amounts in other tissues [Citation39]. MATE1 and MATE2K play important roles in organic cation transport [Citation40]. MATE1 is highly expressed in the kidneys and bile canaliculi, whereas MATE2K is specifically expressed in the kidneys. These transporters are responsible for exporting the substrates taken up by OCT1 and OCT2 [Citation41]. PEPT2 is mainly present in the kidneys and plays a significant role in renal reabsorption of small peptides and peptide-like compounds [Citation42].
Creatinine undergoes transporter-mediated active secretion in the renal proximal tubules; approximately 10–40% of creatinine is removed due to the transporter-mediated active secretion by MATE1, MATE2-K, OCT2, and OAT2 [Citation43]. PARPis are substrates of transporters [Citation44–46]. Five PARP inhibitors act as substrates for P-gp and BRCP [Citation47,Citation48]. Furthermore, PARPis can inhibit transporters [Citation49]. Olaparib inhibits P-gp, BCRP, OATP1B1, OCT1, OCT2, OAT3, MATE1, and MATE2-K [Citation50]. Niraparib can inhibit MATE1 and MATE2-K but does not inhibit BCRP and OCT1 [Citation34]. Rucaparib inhibits P-gp, BCRP, OATP1B1, OATP1B3, OAT1, OAT3, MATE1, MATE2-K, OCT1, OCT2, and MRP4 [Citation34]. At present, there is no evidence of talazoparib-inhibiting transporters [Citation34], which may explain why talazoparib is the only PARPi in this study that does not have an ARF signal. Our findings showed that olaparib, niraparib, and rucaparib all displayed positive signs of elevated creatinine levels and decreased GFR, resulting in ARF.
An in vitro study showed that olaparib may have clinical significance through the inhibition of MDR1, OCT1, OCT2, OATP1B1, OAT3, MATE1, and MATE2-K [Citation50]. Olaparib treatment can cause an increase in serum creatinine levels, possibly due to the inhibition of active tubular secretion. Damage to nephrons can cause an increase in creatinine levels, leading to a decrease in the GFR and creatinine clearance. A study that measured the GFR after taking olaparib showed that olaparib may have caused a reversible and dose-dependent increase in creatinine; however, it did not affect the GFR or prevent underestimation, suggesting that cystatin C could be used to calculate the estimated GFR (eGFR) [Citation51].
The Japanese Adverse Reaction Reporting (JADER) database was used to investigate the adverse reactions to PARPis; renal dysfunction signals were found following treatment with niraparib, which is in accordance with our own findings [Citation52]. Following the introduction of niraparib therapy, a study showed that eGFR decreased by 28%, and 18% of patients developed acute kidney disease [Citation53]. This study did not find evidence of tubular injury through urinary retinol-binding protein testing, although it was assessed in only five patients. Blood creatinine elevation was not limited to the inhibition of tubular creatinine secretion. During niraparib treatment, patients typically experience high blood pressure and increased pulse rates. An increase in dopamine and norepinephrine concentrations may cause a decrease in the eGFR, which may be a consequence of hemodynamic impairment [Citation54].
In a preliminary clinical trial, patients experienced an increase in serum creatinine levels immediately after receiving rucaparib treatment, which then plateaued during continuous rucaparib treatment [Citation20]. Among creatinine elevations, the majority were grade 1 events (<1.5 × the upper limit of normal) [Citation18]. The observed elevation in creatinine levels can be attributed to the inhibition of MATE1, MATE2-K, and OCT2 [Citation55]. Some studies have proposed that an increase in serum creatinine levels among patients taking PARPis may not necessarily indicate a genuine decrease in the GFR. As a result, serum creatinine levels may be deemed less reliable as a surrogate marker for renal function after rucaparib treatment [Citation56].
The timing of ARF caused by PARPis is currently being explored in very few studies. Within 7 days of dosing in the phase I study of olaparib, creatinine clearance was found to have been affected [Citation16]. Our study revealed that most ARF cases occurred within 10 days. In a study on nephrotoxicity associated with niraparib, the GFR decreased from 4 to 70 days (median: 24 days) after starting treatment [Citation53]. Using the JADER database, the average time for patients taking niraparib for renal impairment was 26.5 days [Citation52]. The increase in creatinine levels with rucaparib is usually observed within the first few weeks of treatment [Citation18]. Rupaprib caused the highest rate of ARF between 61 and 90 days, with the TTO of all PARPis occurring between 30 and 90 days after administration in this study. It is important for physicians to be aware of the characteristics of PARPis nephrotoxicity and to provide continuous follow-up of patients.
This study is the first to explore the clinical outcomes of ARF caused by PARPis. Although many studies believe that PARPis-induced creatinine elevation is transient and reversible, does not imply a decline in GFR, and requires dose adjustment [Citation18,Citation22], the results of the present study are not optimistic. Approximately one-fifth of patients treated with PARPis are hospitalized, which is relatively high. It should be noted that the proportion of deaths caused by olaparib and the life-threatening outcomes caused by niraparib are exceptionally high. Due to potentially serious outcomes, it is crucial to pay close attention to elevated creatinine levels and ARF associated with PARPis in clinical practice.
Our study has some limitations. FAERS is a spontaneous reporting system (SRS). The absence of denominator data makes it impossible to make direct safety comparisons, quantify associations, or calculate incidence rates [Citation53]. The quantification of adverse reaction signals based on the total number of adverse reactions was not possible using the collected data. The purpose was to provide a qualitative indicator using the signal intensity between the drugs and the reactions alone. Compared with clinical trials and cohort studies, SRS data are generally less reliable. The identification and reporting of AEs within SRS are subject to less stringent controls. Third, it is difficult to identify significant risk factors for PARPis and ARF because of the lack of the patients’ own physical conditions, preexisting kidney disorders and comorbidities that may have an impact on the renal function. Furthermore, this study was not restricted to a particular disease, which is significantly different from clinical trials. Fourth, the proportion of reports on talaparib was only 0.54%, which may have caused considerable bias due to underreporting. Fifth, calculation, justification, and power analyses for the selected sample size in this study were not conducted because the intention was to include all eligible adverse drug reactions. Finally, data mining revealed imperfect reporting, with inaccuracies and incomplete entries, potentially causing analytical bias. Despite the limitations of FAERS, our findings illuminate the essential aspects of PARPis-associated ARF and provide a framework for rigorous research to validate these findings.
5. Conclusion
Using the FAERS database, we investigated ARF associated with various PARPi therapies and provided information on their occurrence, clinical features, and prognosis. ARF is associated with the use of some PARPis, although not with talazoparib. The use of olaparib, niraparib, and rucaparib led to increased creatinine levels and decreased GFRs. Research on PARPi-related ARF deserves more attention because of the high proportion of serious AEs and delayed onset of ARF.
Declaration of interests
The authors have no relevant affiliations or financial involvement with any organization or entity with a financial interest in or financial conflict with the subject matter or materials discussed in the manuscript. This includes employment, consultancies, honoraria, stock ownership or options, expert testimony, grants or patents received or pending, or royalties.
Reviewer disclosures
Peer reviewers on this manuscript have no relevant financial or other relationships to disclose.
Ethics statement
Ethical approval was not required for this study because we used the FAERS database, which is a free open-access database.
Availability of data and material
The data that support the findings of this study are available upon from the corresponding author, Yanfeng Wang, on reasonable request. The raw data can be obtained from the FAERS database at the following link: FAERS Quarterly Data Extract Files (fda.gov).
Author contribution statment
XR drafted the manuscript. All authors participated in data analysis and interpretation, and manuscript revision. All authors have read and approved the manuscript.
Acknowledgments
We thank Xin Zhou for his contributions regarding the data processing and image editing. We also appreciate Yiran Chen’s guidance on PARPis treatment for ovarian cancer.
Additional information
Funding
References
- Sung H, Ferlay J, Siegel RL, et al. Global Cancer Statistics 2020: GLOBOCAN estimates of incidence and mortality worldwide for 36 cancers in 185 countries. CA Cancer J Clin. 2021 May;71(3):209–249. doi: 10.3322/caac.21660 Epub 2021 Feb 4. PMID: 33538338.
- Lord CJ, Ashworth A. PARP inhibitors: Synthetic lethality in the clinic. Science. 2017 Mar 17;355(6330):1152–1158. doi: 10.1126/science.aam7344 Epub 2017 Mar 16. PMID: 28302823; PMCID: PMC6175050.
- Boussios S, Karihtala P, Moschetta M, et al. Veliparib in ovarian cancer: a new synthetically lethal therapeutic approach. Invest New Drugs. 2020 Feb;38(1):181–193. doi: 10.1007/s10637-019-00867-4 Epub 2019 Oct 24. PMID: 31650446.
- Helleday T. The underlying mechanism for the PARP and BRCA synthetic lethality: clearing up the misunderstandings. Mol Oncol. 2011 Aug;5(4):387–393. doi: 10.1016/j.molonc.2011.07.001 Epub 2011 Jul 22. PMID: 21821475; PMCID: PMC5528309.
- Aliyuda F, Moschetta M, Ghose A, et al. Advances in ovarian cancer treatment beyond PARP inhibitors. Curr Cancer Drug Targets. 2023;23(6):433–446. doi: 10.2174/1568009623666230209121732 PMID: 36757037.
- Hatano Y, Tamada M, Matsuo M, et al. Molecular Trajectory of BRCA1 and BRCA2 Mutations. Front Oncol. 2020 Mar 25;10:361. doi: 10.3389/fonc.2020.00361 PMID: 32269964; PMCID: PMC7109296.
- Garg V, Oza AM. Treatment of ovarian cancer beyond PARP inhibition: current and future options. Drugs. 2023 Oct;83(15):1365–1385. doi: 10.1007/s40265-023-01934-0 Epub 2023 Sep 22. PMID: 37737434; PMCID: PMC10581945.
- Boussios S, Rassy E, Moschetta M, et al. BRCA mutations in ovarian and prostate cancer: bench to bedside. Cancers (Basel). 2022 Aug 11;14(16):3888. doi: 10.3390/cancers14163888 PMID: 36010882; PMCID: PMC9405840.
- Mateo J, Lord CJ, Serra V, et al. A decade of clinical development of PARP inhibitors in perspective. Ann Oncol. 2019 Sep 1;30(9):1437–1447. doi: 10.1093/annonc/mdz192 PMID: 31218365; PMCID: PMC6771225.
- Ghose A, Moschetta M, Pappas-Gogos G, et al. Genetic aberrations of DNA repair pathways in prostate cancer: translation to the clinic. Int J Mol Sci. 2021 Sep 10;22(18):9783. doi: 10.3390/ijms22189783 PMID: 34575947; PMCID: PMC8471942.
- Alsop K, Fereday S, Meldrum C, et al. BRCA mutation frequency and patterns of treatment response in BRCA mutation-positive women with ovarian cancer: a report from the Australian Ovarian Cancer Study Group. J Clin Oncol. 2012 Jul 20 [cited 2012 Jun 18];30(21):2654–2663. doi: 10.1200/JCO.2011.39.8545 Erratum in: J Clin Oncol. 2012 Nov 20;30(33):4180. PMID: 22711857; PMCID: PMC3413277.
- Boussios S, Abson C, Moschetta M, et al. Poly (ADP-Ribose) polymerase inhibitors: Talazoparib in ovarian cancer and beyond. Drugs R D. 2020 Jun;20(2):55–73. doi: 10.1007/s40268-020-00301-8 PMID: 32215876; PMCID: PMC7221042.
- Slade D. PARP and PARG inhibitors in cancer treatment. Genes Dev. 2020 Mar 1;34(5–6):360–394. doi: 10.1101/gad.334516.119 Epub 2020 Feb 6. PMID: 32029455; PMCID: PMC7050487.
- Zhao D, Long X, Wang J. Metabolism related pharmacokinetic drug drug interactions with poly (ADPribose) polymerase inhibitors (Review). Oncol Rep. 2022 Jan;47(1):20. doi: 10.3892/or.2021.8231 Epub 2021 Nov 23. PMID: 34812476.
- Zhao D, Long X, Wang J. Dose adjustment of Poly (ADP‑Ribose) polymerase inhibitors in patients with hepatic or renal impairment. DDDT. 2022 Nov 14;16:3947–3955. doi: 10.2147/DDDT.S387920 PMID: 36405648; PMCID: PMC9673935.
- Rolfo C, de Vos-Geelen J, Isambert N, et al. Pharmacokinetics and safety of olaparib in patients with advanced solid tumours and renal impairment. Clin Pharmacokinet. 2019 Sep;58(9):1165–1174. doi: 10.1007/s40262-019-00754-4 PMID: 30877569.
- Salem AH, Giranda VL, Mostafa NM. Population pharmacokinetic modeling of veliparib (ABT-888) in patients with non-hematologic malignancies. Clin Pharmacokinet. 2014 May;53(5):479–488. doi: 10.1007/s40262-013-0130-1 PMID: 24452810.
- Oza AM, Tinker AV, Oaknin A, et al. Antitumor activity and safety of the PARP inhibitor rucaparib in patients with high-grade ovarian carcinoma and a germline or somatic BRCA1 or BRCA2 mutation: Integrated analysis of data from Study 10 and ARIEL2. Gynecol Oncol. 2017 Nov;147(2):267–275. doi: 10.1016/j.ygyno.2017.08.022 Epub 2017 Sep 4. PMID: 28882436.
- Li N, Zhu J, Yin R, et al. Treatment with Niraparib maintenance therapy in patients with newly diagnosed advanced ovarian cancer: a phase 3 randomized clinical trial. JAMA Oncol. 2023 Sep 1;9(9):1230–1237. doi: 10.1001/jamaoncol.2023.2283 PMID: 37440217; PMCID: PMC10346505.
- Coleman RL, Oza AM, Lorusso D, et al. ARIEL3 investigators. Rucaparib maintenance treatment for recurrent ovarian carcinoma after response to platinum therapy (ARIEL3): a randomised, double-blind, placebo-controlled, phase 3 trial. The Lancet. 2017 Oct 28;390(10106):1949–1961. doi: 10.1016/S0140-6736(17)32440-6 Epub 2017 Sep 12. Erratum in: Lancet. 2017 Oct 28;390(10106):1948. PMID: 28916367; PMCID: PMC5901715.
- LaFargue CJ, Dal Molin GZ, Sood AK, et al. Exploring and comparing adverse events between PARP inhibitors. Lancet Oncol. 2019 Jan;20(1):e15–e28. doi: 10.1016/S1470-2045(18)30786-1 PMID: 30614472; PMCID: PMC7292736.
- Friedlander M, Lee YC, Tew WP. Managing adverse effects associated with Poly (ADP-ribose) polymerase inhibitors in ovarian cancer: a synthesis of clinical trial and real-world data. Am Soc Clin Oncol Educ Book. 2023 Jun;43:e390876. doi: 10.1200/EDBK_390876 PMID: 37285556.
- US Food and Drug Administration. FDA Adverse Event Reporting System (FAERS) quarterly data extract files. 2021 [cited 2021 Oct 20]. Available from: https://fis.fda.gov/extensions/fpd-qde-faers/fpd-qde-faers.html
- Maintenance and support service organization. Support documentation: MedDRA Version 23.0 English March/April 2020. [cited 2021 Oct 6]. Available from: https://www.meddra.org/how-to-use/support-documentation/English
- Bräuer D. Zum Vergleich unabhängiger Stichproben. Comparison of independent samples: the Kruskal-Wallis test and the Conover multiple median value comparison. Z Arztl Fortbild (Jena). 1988;82(18):883–884. German. PMID: 3213086.
- O’Malley DM, Krivak TC, Kabil N, et al. PARP inhibitors in ovarian cancer: a review. Target Oncol. 2023 Jul;18(4):471–503. doi: 10.1007/s11523-023-00970-w Epub 2023 Jun 3. PMID: 37268756; PMCID: PMC10344972.
- Giannini A, Di Dio C, Di Donato V, et al. PARP inhibitors in newly diagnosed and recurrent ovarian cancer. Am J Clin Oncol. 2023 Sep 1;46(9):414–419. doi: 10.1097/COC.0000000000001024 Epub 2023 Jun 12. PMID: 37314974.
- Fischetti M, Di Donato V, Palaia I, et al. Advances in small molecule maintenance therapies for high-grade serous ovarian cancer. Expert Opin Pharmacother. 2023 Jan;24(1):65–72. doi: 10.1080/14656566.2022.2154144 Epub 2022 Dec 12. PMID: 36458890.
- Golia D’Augè T, Giannini A, Bogani G, et al. Treatment and follow-up of gynecological cancers: state of art and future perspectives. Clin Exp Obstet Gynecol. 2023;50(8):160. doi: 10.31083/j.ceog5008160
- Levey AS, Perrone RD, Madias NE. Serum creatinine and renal function. Annu Rev Med. 1988;39:465–490. doi: 10.1146/annurev.me.39.020188.002341 PMID: 3285786.
- Colombi A. Die klinische Bedeutung der Nierenfunktionsprüfungen [The clinical significance of kidney function tests]. Schweiz Med Wochenschr. 1989 Jun 17;119(24):854–858. German. PMID: 2749216.
- Nakada T, Kudo T, Kume T, et al. Estimation of changes in serum creatinine and creatinine clearance caused by renal transporter inhibition in healthy subjects. Drug Metab Pharmacokinet. 2019 Aug;34(4):233–238. doi: 10.1016/j.dmpk.2019.02.006 Epub 2019 Mar 2. PMID: 31176593.
- Nigam SK. What do drug transporters really do? Nat Rev Drug Discov. 2015 Jan;14(1):29–44. doi: 10.1038/nrd4461 Epub 2014 Dec 5. PMID: 25475361; PMCID: PMC4750486.
- Zhao D, Long X, Wang J. Transporter mediated drug drug interactions involving poly (ADPribose) polymerase inhibitors (Review). Oncol Lett. 2023 Mar 7;25(4):161. doi: 10.3892/ol.2023.13747 PMID: 36936025; PMCID: PMC10017913.
- Rives ML, Javitch JA, Wickenden AD. Potentiating SLC transporter activity: Emerging drug discovery opportunities. Biochem Pharmacol. 2017 Jul 1;135:1–11. doi: 10.1016/j.bcp.2017.02.010 Epub 2017 Feb 16. PMID: 28214518.
- Beis K. Structural basis for the mechanism of ABC transporters. Biochem Soc Trans. 2015 Oct;43(5):889–893. doi: 10.1042/BST20150047 PMID: 26517899.
- Yonezawa A, Inui K. Importance of the multidrug and toxin extrusion MATE/SLC47A family to pharmacokinetics, pharmacodynamics/toxicodynamics and pharmacogenomics. Br J Pharmacol. 2011 Dec;164(7):1817–1825. doi: 10.1111/j.1476-5381.2011.01394.x PMID: 21457222; PMCID: PMC3246706.
- Samodelov SL, Kullak-Ublick GA, Gai Z, et al. Organic cation transporters in human physiology, pharmacology, and toxicology. Int J Mol Sci. 2020 Oct 24;21(21):7890. doi: 10.3390/ijms21217890 PMID: 33114309; PMCID: PMC7660683.
- Pochini L, Galluccio M, Scalise M, et al. OCTN: a small transporter subfamily with great relevance to human pathophysiology, drug discovery, and diagnostics. SLAS Discov. 2019 Feb;24(2):89–110. doi: 10.1177/2472555218812821 Epub 2018 Dec 7. PMID: 30523710.
- Nies AT, Damme K, Kruck S, et al. Structure and function of multidrug and toxin extrusion proteins (MATEs) and their relevance to drug therapy and personalized medicine. Arch Toxicol. 2016 Jul;90(7):1555–1584. doi: 10.1007/s00204-016-1728-5 Epub 2016 May 10. PMID: 27165417.
- Motohashi H, Inui K. Organic cation transporter OCTs (SLC22) and MATEs (SLC47) in the human kidney. Aaps J. 2013 Apr;15(2):581–588. doi: 10.1208/s12248-013-9465-7 Epub 2013 Feb 22. PMID: 23435786; PMCID: PMC3675737.
- Brecht K, Schäfer AM, Meyer Zu Schwabedissen HE. Uptake Transporters of the SLC21, SLC22A, and SLC15A Families in anticancer therapy-modulators of cellular entry or pharmacokinetics? Cancers (Basel). 2020 Aug 12;12(8):2263. doi: 10.3390/cancers12082263 PMID: 32806706; PMCID: PMC7464370.
- Mathialagan S, Rodrigues AD, Feng B. Evaluation of renal transporter inhibition using creatinine as a substrate in vitro to assess the clinical risk of elevated serum creatinine. J Pharm Sci. 2017 Sep;106(9):2535–2541. doi: 10.1016/j.xphs.2017.04.009 Epub 2017 Apr 14. PMID: 28416419.
- Tew WP, Lacchetti C, Ellis A, et al. PARP Inhibitors in the management of ovarian cancer: ASCO guideline. J Clin Oncol. 2020 Oct 20;38(30):3468–3493. doi: 10.1200/JCO.20.01924 Epub 2020 Aug 13. PMID: 32790492; PMCID: PMC8942301.
- Rose M, Burgess JT, O’Byrne K, et al. PARP Inhibitors: clinical relevance, mechanisms of action and tumor resistance. Front Cell Dev Biol. 2020 Sep 9;8:564601. doi: 10.3389/fcell.2020.564601 PMID: 33015058; PMCID: PMC7509090.
- Hennes ER, Dow-Hillgartner EN, Bergsbaken JJ, et al. PARP-inhibitor potpourri: a comparative review of class safety, efficacy, and cost. J Oncol Pharm Pract. 2020 Apr;26(3):718–729. doi: 10.1177/1078155219895066 Epub 2020 Jan 6. PMID: 31902284.
- Martins Ml F, Loos NHC, Mucuk S, et al. P-Glycoprotein (ABCB1/MDR1) controls brain penetration and intestinal disposition of the PARP1/2 inhibitor Niraparib. Mol Pharm. 2021 Dec 6;18(12):4371–4384. doi: 10.1021/acs.molpharmaceut.1c00553 Epub 2021 Nov 3. PMID: 34730366.
- Durmus S, Sparidans RW, van Esch A, et al. Breast cancer resistance protein (BCRP/ABCG2) and P-glycoprotein (P-GP/ABCB1) restrict oral availability and brain accumulation of the PARP inhibitor rucaparib (AG-014699). Pharm Res. 2015 Jan;32(1):37–46. doi: 10.1007/s11095-014-1442-z Epub 2014 Jun 25. PMID: 24962512.
- Dufour R, Daumar P, Mounetou E, et al. BCRP and P-gp relay overexpression in triple negative basal-like breast cancer cell line: a prospective role in resistance to Olaparib. Sci Rep. 2015 Aug 3;5:12670. doi: 10.1038/srep12670 PMID: 26234720; PMCID: PMC4522660.
- McCormick A, Swaisland H. In vitro assessment of the roles of drug transporters in the disposition and drug-drug interaction potential of olaparib. Xenobiotica. 2017 Oct;47(10):903–915. doi: 10.1080/00498254.2016.1241449 Epub 2016 Oct 26. PMID: 27684210.
- Bruin MAC, Korse CM, van Wijnen B, et al. A real or apparent decrease in glomerular filtration rate in patients using olaparib? Eur J Clin Pharmacol. 2021 Feb;77(2):179–188. doi: 10.1007/s00228-020-03070-0 Epub 2020 Dec 14. PMID: 33319340; PMCID: PMC7803870.
- Yamaoka K, Fujiwara M, Uchida M, et al. Comprehensive analysis of adverse events induced by PARP inhibitors using JADER and time to onset. Life (Basel). 2022 Aug 31;12(9):1355. doi: 10.3390/life12091355 PMID: 36143391; PMCID: PMC9504973.
- Lazareth H, Delanoy N, Cohen R, et al. Nephrotoxicity associated with Niraparib. Am J Kidney Dis. 2020 Dec;76(6):898–900. doi: 10.1053/j.ajkd.2020.05.021 Epub 2020 Jul 14. PMID: 32679152.
- Bruin MAC, Sonke GS, Beijnen JH, et al. Pharmacokinetics and pharmacodynamics of PARP inhibitors in oncology. Clin Pharmacokinet. 2022 Dec;61(12):1649–1675. doi: 10.1007/s40262-022-01167-6 Epub 2022 Oct 11. PMID: 36219340; PMCID: PMC9734231.
- Liao M, Jaw-Tsai S, Beltman J, et al. Evaluation of in vitro absorption, distribution, metabolism, and excretion and assessment of drug-drug interaction of rucaparib, an orally potent poly(ADP-ribose) polymerase inhibitor. Xenobiotica. 2020 Sep;50(9):1032–1042. doi: 10.1080/00498254.2020.1737759 Epub 2020 Mar 18. PMID: 32129697.
- Zibetti Dal Molin G, Westin SN, Msaouel P, et al. Discrepancy in calculated and measured glomerular filtration rates in patients treated with PARP inhibitors. Int J Gynecol Cancer. 2020 Jan;30(1):89–93. doi: 10.1136/ijgc-2019-000714 Epub 2019 Dec 1. PMID: 31792084.