Abstract
The mechanism of inhalation anesthesia remains to be fully elucidated. While certain neuronal membrane proteins are considered sites of action, cytosolic proteins may also be targets. We hypothesize that inhaled anesthetics may act via glyceraldehyde 3-phosphate dehydrogenase (GAPDH), which has recently been shown to participate in neuronal inhibition. We examined the effects of sevoflurane, a halogenated ether anesthetic, on the catalytic and fluorescence properties of GAPDH. Initial rates of oxidoreductase activity decreased approximately 30% at saturating levels of sevoflurane. NADH-stimulated oxidoreductase activity (25 μM NADH; 0.8 mM NAD+) increased with sevoflurane. Sevoflurane quenched tryptophan fluorescence emission and increased polarization. Additionally, sevoflurane increased the susceptibility of GAPDH to thermal denaturation suggesting an effect on conformation. Our findings warrant further research on sevoflurane's effect on GAPDH and indicate that this approach may lead to delineation of a novel contribution to the mechanism of anesthesia.
Introduction
Sevoflurane, a halogenated ether (), is an inhaled agent [Citation1] for induction and maintenance of general anesthesia. Its low water-solubility facilitates a rapid induction of anesthesia [Citation2], suggesting the importance of lipophilicity in promoting anesthesia. The molecular mechanisms of general anesthesia, however, have yet to be fully elucidated. Several approaches relate the mechanism of action to lipophilic partitioning of the inhaled anesthetic affecting diverse target membrane proteins: potassium channel [Citation3], plasma membrane Ca2+-ATPase [Citation4] and GABAA receptor [Citation5]. Less is known about effects on soluble proteins such as cytosolic enzymes, which contain core and surface hydrophobic patches theoretically capable of binding inhaled anesthetics.
In the present study we examined the effects of sevoflurane on glyceraldehyde 3-phosphate dehydrogenase (GAPDH), which is a glycolytic enzyme that plays a role in the regulation of the GABAA receptor [Citation6], suggesting a link between GAPDH and anesthesia. Neuronal GAPDH localizes to the post-synaptic region [Citation7] by binding to the inner surface of the plasma membrane [Citation8] in juxtaposition to several membrane proteins such as Na+,K+-ATPase [Citation9] and GABAA receptor. The GAPDH-phosphoglyerate kinase (PGK) complex locally produces ATP that fuels the ionic pump and provides a substrate for the phosphorylation of the GABAA receptor [Citation6]. We propose that inhaled anesthetics may act on the GABAA receptor via GAPDH. In order to determine if inhaled anesthetics act on GAPDH we studied the direct effects of sevoflurane on GAPDH activity. Sevoflurane-induced conformational changes of GAPDH were also examined providing a mechanism for the modulation of GAPDH activity.
Materials and methods
Materials
Glyceraldehyde, Tris-maleate, NADH and NAD+ were purchased from Sigma Chemical Co. (St. Louis, MO). EDTA was obtained from Mallinckrodt (Hazelwood, MO). Rabbit muscle glyceraldehyde 3-phosphate dehydrogenase (GAPDH) [EC 1.2.1.12] was purchased as a lyophilized solid from Sigma and prepared in a Tris-maleate buffer (pH 8.0) prior to use. Sevoflurane was from Abbott Laboratories (North Chicago, IL). All other chemicals were of reagent grade.
Incubation conditions
We calculated that there would be 77.5 μmoles of sevoflurane in a 0.5 mL compartment of brain at 1 MAC (minimum alveolar concentration, the level of volatile anesthetic at which 50% of patients do not move in response to noxious stimuli), which is 1.85%. Under physiological conditions, this would represent a 3000:1 ratio of sevoflurane to GAPDH. Sevoflurane is not miscible in water. To maximize sevoflurane partitioning into the protein, we carefully mixed sevoflurane with GAPDH prior to dilution in a 100 mM Tris-maleate buffer (pH 8.0) to final concentrations of GAPDH (0.2–0.7 mg/mL). After mixing, unbound sevoflurane separated from the aqueous solution and remained on the bottom of the vial. Additionally, there was no change in protein concentration, ruling out non-specific binding that would partition the protein in the sevoflurane phase and suggesting that only a very small amount of sevoflurane binds to protein. Samples were then tested by the following assays.
Enzyme activity
Enzyme activity was measured according to a spectrophotometric procedure, which was previously described [Citation10] demonstrating the use of glyceraldehyde as a viable substrate. Unless otherwise indicated, the assay conditions included a 100 mM Tris-maleate buffer (pH 8.0) containing 33.3 mM glyceraldehyde, 0.8 mM NAD+, 1.5 mM EDTA, 50 mM Pi and 1.2–4.5 μM GAPDH. Assays were run at 25°C. Enzyme activity was measured in the presence and absence of sevoflurane at molar ratios of sevoflurane to GAPDH exceeding 10000:1. The oxidoreductase reaction was followed by changes in 340 nm absorbance, which monitored NAD+ reduction, using disposable cuvets in an Ultrospec 4000 spectrophotometer from GE Healthcare, formerly Amersham Biosciences (Piscataway, NJ). Calculations included the extinction coefficient for NADH (6.22 absorbance of one μmole NADH/mL; 1 cm light path). In experiments that tested NADH-stimulated activity, NADH (5–30 μM) was added 30 s following start of the assay and changes in activity were recorded. Oxidoreductase activities are given as nmole/min per mg protein and presented as basal and NADH-stimulated activities.
Intrinsic fluorescence
Intrinsic fluorescence and polarization due to the presence of 3 tryptophan residues per subunit were measured using an LS50B fluorometer (Perkin Elmer Corporation, Shelton, CT). Intensity and polarization values were read at 24°C with integration times set at 10 s (excitation: 293 nm; emission: 342 nm; slit widths at 2.5 nm). Intensities are given as arbitrary units (au). Fluorescence spectra were obtained with a scanning speed of 600 nm/min over an emission range of 300–500 nm (slit width, 2.5 nm). Using screwcap vials with PTFE/silicone septa (Supelco, Bellefonte, PA), sevoflurane was mixed thoroughly with GAPDH (12 μM) at a molar ratios of greater than 10000:1. Samples were diluted and incubated for 15 min at 37°C and allowed to cool to room temperature prior to fluorometric analysis.
Thermal denaturation
Thermal denaturation profiles of sevoflurane-treated samples were determined by measuring changes in light scattering as previously described [Citation11]. Peltier-heated quartz cuvets were used in an Ultrospec 4000 spectrophotometer. Following addition of assay components samples were purged with nitrogen and capped. Heat-induced denaturation of GAPDH (3.6–5.4 μM), which leads to unfolding/aggregation and increased light scattering, was recorded from 25 to 65°C in the presence and absence of sevoflurane at a ratio greater than 19000:1 with a temperature rate of 0.5°C/min.
Results
GAPDH activity
Sevoflurane, which was at a 34000:1 molar ratio relative to GAPDH, inhibited the oxidoreductase activity by about 30% () Activities were determined from initial rates of NAD+ reduction prior to NADH accumulation (less than 10 μM). Substrates (glyceraldehyde, NAD+ and Pi) were in excess to insure that substrate depletion did not occur during the reaction and that maximal rates were being measured. The changes in absorbance over a brief initial time period were linear.
Table I. Effects of sevoflurane on GAPDH.
Nadh-stimulated Gapdh Activity
We observed that NADH increased the oxidoreductase activity of GAPDH (, filled circles). Upon addition of NADH (5–30 μM) to an assay medium containing untreated GAPDH (4.5 μM) with excess NAD+(0.8 mM), oxidoreductase activity increased from a basal rate of approximately 45 nmole/min per mg protein to 115.8. NADH, the reaction product, stimulated activity and further production of NADH, which still represented less than 10% of the reducible substrate NAD+. When this activity was examined in the presence of sevoflurane, there was a marked increase in activity (, open circles; ).
Figure 2 Effects of micromolar NADH on GAPDH activity. GAPDH (4.5 μM) was incubated with (open circles) and without (closed circles) sevoflurane prior to assay at a ratio of 34000:1 (sevoflurane to GAPDH). Glyceraldehyde and NAD+ were at saturating concentrations, NADH (5–30 μM) was added to the assay during continuous monitoring of the reaction, and rates were recorded.
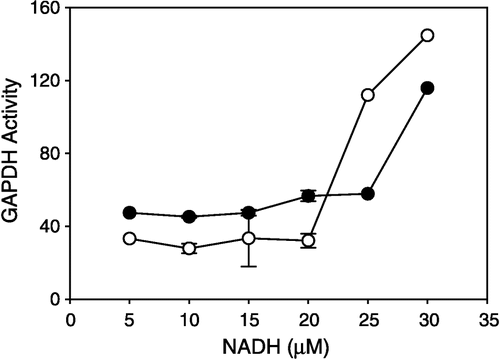
Fluorescence properties of GADPH
The intrinsic fluorescence emission spectra (excitation: 290 nm; emission: 300–500 nm) of GAPDH exhibited a small but reproducible change following incubation with sevoflurane (). Spectral shifts were undetectable under these conditions, but the fluorescence intensity at the wavelength of peak emission (342 nm) decreased (). The molar ratio of sevoflurane to GAPDH was approximately 13000:1. Additionally, under the same conditions fluorescence polarization (excitation: 293 nm; emission: 342 nm) in the presence of sevoflurane was higher than control (). These observations suggest that sevoflurane binds to GAPDH and that this interaction may contribute to the changes in catalytic function.
Thermal denaturation
Sevoflurane (at a molar ratio to GAPDH of 39000:1) increased heat-induced denaturation (). We observed a left shift in the thermal denaturation curve in the presence of sevoflurane. The Tm (the temperature at which there is 50% denaturation) decreased in the presence of sevoflurane (sevoflurane, 51.1°C; control, 53.7). This observation suggests that the packing of the domains in the subunit as well as the subunit interactions may be affected by the binding of sevoflurane to GAPDH.
Figure 4 Sevoflurane increased GAPDH susceptibility to thermal denaturation. Heat-induced unfolding/aggregation of GAPDH (4.1 μM) was recorded as light scattering (25–65°C) at 450 nm in the presence or absence of sevoflurane at a ratio of 19000:1 (sevoflurane to GAPDH). The rate of temperature change was 0.5°C/min.
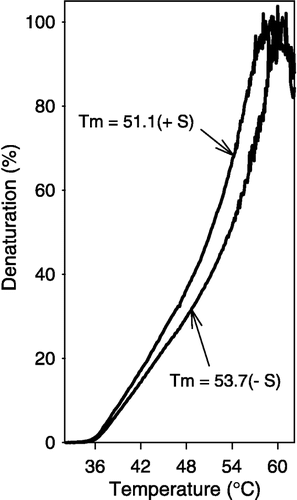
Discussion
We report that sevoflurane () modulated the oxidoreductase activity of GAPDH. Sevoflurane inhibited the basal oxidoreductase activity (; ) suggesting that sevoflurane prevented intra-subunit conformational changes. This observation is consistent with the literature. Halothane inhibits GAPDH activity [Citation12] presumably by affecting its conformation [Citation13]. In our model, GAPDH phosphorylated glyceraldehyde to 1-phosphoglycerate using Pi and reduced NAD+ to NADH. The catalytic steps include the formation of an acyl thioester bond that connects glyceraldehyde to GAPDH and the movement of electrons from the bond to NAD+. The process requires intra-subunit movement since the glyceraldehyde and NAD+ binding sites are on different domains. We propose that sevoflurane impairs the intra-subunit movement required during catalysis.
Sevoflurane increased NADH-stimulated activity (; ), suggesting that sevoflurane promoted subunit interaction that may be mediated by dinucleotides. NADH-stimulated activity was measured at 25–30 μM NADH with 0.8 mM NAD+ and 2.0 μM GAPDH. Oligomerization of GAPDH occurs above 1.0 μM and subunit interactions persist at physiological levels (approximately 50 μM). Product inhibition of GAPDH [Citation10,Citation14] requires much higher concentrations of NADH. Interestingly, micromolar concentrations of NAD+ stimulate the backward reaction of GAPDH [Citation15,Citation16]. Binding of NAD+ to GAPDH rigidifies the molecule [Citation17], promotes an asymmetry of the tetramer [Citation18] and produces negative cooperativity [Citation19]. NADH, on the other hand, loosens the tetrameric structure [Citation20], and may therefore relieve a quaternary-induced inhibition and thus promote a conformation that enhances the catalytic activity. GAPDH crystallizes as a dimer [Citation21] and may function as a pair of interacting dimers regulated by the different dinucleotides. Both tetrameric and dimeric forms exhibit enzymatic activity though the oligomeric relationship to catalysis is still poorly understood.
We propose that sevoflurane up- and downregulates GAPDH at NADH concentrations above and below 25 μM, respectively. These observations suggest that GAPDH's effect on the GABAA receptor may be associated with the metabolic state of the neuron. NADH levels vary with metabolism [Citation22]. High metabolic rates in brain trauma cause damage and isoflurane may mitigate the excitotoxicity by controlling hyperglycolysis [Citation23]. Our study suggests that glycolytic inhibition by inhaled anesthetics at low [NADH] may occur at GAPDH.
Sevoflurane-induced increase in NADH-stimulated activity may be due to effects on inter-subunit interaction. Sevoflurane binding likely occurs close to hydrophobic patches similar to the binding sites of dinucleotides [Citation24], maintaining the tetramer in a more open NADH-receptive conformation. This open conformation is consistent with the observation that sevoflurane increases GAPDH susceptibility to thermal denaturation ().
We observed a sevoflurane-induced change in tryptophan emission and polarization (; ). There are three tryptophans per subunit: W84, W193 and W310 [Citation21]. In its dimeric form, N-terminal W84 and C-terminal W310 are 8.5 and 11 angstrom, respectively, from the dinucleotide binding site on one subunit and W193 is 8.0 angstrom from the dinucleotide binding site on the neighboring subunit. Furthermore, W193 is 4.0 angstrom from hydrophobic residues on its neighboring subunit, suggesting it may play a role in inter-subunit interaction. The sevoflurane-induced decrease in tryptophan emission may be due to binding in close proximity to tryptophans and by affecting long distance conformational changes. Further research is required to identify sevoflurane's site of action.
It is generally accepted that the GABAA receptor plays a pivotal role in anesthesia [Citation25]. The exact mechanism, however, remains unknown. The GABAA receptor is regulated by the ATP-generating GAPDH-PGK reaction [Citation6]. Regulation presumably involves reversible receptor phosphorylation, suggesting that GAPDH has ATP-dependent kinase-like activity. Our observation that sevoflurane modulates GAPDH oxidoreductase activity and mediates conformational changes suggests that sevoflurane may affect the GAPDH regulation of the GABAA receptor. Anesthesia occurs through neuronal inhibition, which may be triggered by the GAPDH-mediated chemical modification of the GABAA receptor that is catalytic, rapid and reversible, making GAPDH a strong candidate as a specific target for sevoflurane or other inhaled anesthetics.
In summary, sevoflurane, which is a commonly used inhaled anesthetic, modulated GAPDH activity. The basal activity of GAPDH decreased and the NADH-stimulated activity increased with sevoflurane. Additionally, sevoflurane quenched fluorescence emission and increased fluorescence polarization. These observations provide evidence that sevoflurane binds to GAPDH, presumably in the region of the hydrophobic dinucleotide sites, and alters protein conformation and oligomerization. Lastly, we observed that sevoflurane promoted heat-induced denaturation, suggesting that sevoflurane maintained the GAPDH in the ‘open’ conformation, which is conducive to NADH binding.
Acknowledgements
The authors acknowledge the contributions of Heather D. Craig. The study was supported in part by a grant from the Division of Research, Kansas City University of Medicine and Biosciences and research funds from the Department of Anesthesiology, University of Missouri – Kansas City/Saint Luke's Hospital, Kansas City, Missouri.
References
- Jones RM. Desflurane and sevoflurane: Inhalation anaesthetics for this decade?. Br J Anaesth 1990; 65: 527–536
- Wallin RF, Regan BM, Napoli MD, Stern IJ. Sevoflurane: A new inhalational anesthetic agent. Anesth Analg 1975; 54: 758–766
- Franks NP, Lieb WR. An anaesthetic-activated potassium channel. Alcohol Alcohol Suppl 1991; 1: 197–202
- Lopez MM, Kosk-Kosicka D. How do volatile anesthetics inhibit Ca(2+)-ATPases?. J Biol Chem 1995; 270: 28239–28245
- Rudolph U, Antkowiak B. Molecular and neuronal substrates for general anaesthetics. Nat Rev Neurosci 2004; 5: 709–720
- Laschet JJ, Minier F, Kurcewicz I, Bureau MH, Trottier S, Jeanneteau F, Griffon N, Samyn B, Van Beeumen J, Louvel J, Sokoloff P, Pumain R. Glyceraldehyde-3-phosphate dehydrogenase is a GABAA receptor kinase linking glycolysis to neuronal inhibition. J Neurosci 2004; 24: 7614–7622
- Walikonis RS, Jensen ON, Mann M, Provance DW, Jr, Mercer JA, Kennedy MB. Identification of proteins in the postsynaptic density fraction by mass spectrometry. J Neurosci 2000; 20: 4069–4080
- Daum G, Keller K, Lange K. Association of glycolytic enzymes with the cytoplasmic side of the plasma membrane of glioma cells. Biochim Biophys Acta 1988; 939: 277–281
- Mercer RW, Dunham PB. Membrane-bound ATP fuels the Na/K pump. Studies on membrane-bound glycolytic enzymes on inside-out vesicles from human red cell membranes. J Gen Physiol 1981; 78: 547–568
- Fahien LA. A study of the reaction of glyceraldehyde with glyceraldehyde 3-phosphate dehydrogenase. J Biol Chem 1996; 241: 4115–4123
- Seidler NW, Yeargans GS. Effects of thermal denaturation on protein glycation. Life Sci 2001; 71: 1789–1799
- Laverty DM, Fennema O. Effect of anaesthetics and dichlorodifluoromethane on the viability of the cells of Escherichia coli and the activities of some of its enzymes. Microbios 1985; 44: 7–20
- Laverty DM, Fennema O. Effects of anesthetics and dichlorodifluoromethane on the activities of glyceraldehyde-phosphate dehydrogenase and pectin methylesterase. Biochem Pharmacol 1985; 34: 2839–2846
- Furfine CS, Velick SF. The acyl-enzyme intermediate and the kinetic mechanism of the glyceraldhyde 3-phosphate dehydrogenase reaction. J Biol Chem 1965; 240: 844–855
- Smith CM, Velick SF. The glyceraldehyde 3-phosphate dehydrogenases of liver and muscle. Cooperative interactions and conditions for functional reversibility. J Biol Chem 1972; 247: 273–284
- Schwendimann B, Ingbar D, Bernhard SA. On the function of half-site reactivity: Intersubunit. NAD+-dependent activation of acyl-glcyeraldehyde 3-phosphate dehydrogenase reduction by NADH. J Mol Biol 1976; 108: 123–138
- Osborne HH, Hollaway MR. An investigation of the nicotinamide-adenine dinucleotide-induced ’tightening’ of the structure of glyceraldehyde 3-phosphate dehydrogenase. Biochem J 1976; 152: 255–259
- Leslie AG, Wonacott AJ. Structural evidence for ligand-induced sequential conformational changes in glyceraldehyde 3-phosphate dehydrogenase. J Mol Biol 1984; 178: 743–772
- Bell JE, Dalziel K. Conformational changes of glyceraldehyde-3-phosphate dehydrogenase induced by the binding of NAD. A unified model for positive and negative cooperativity. Biochim Biophys Acta 1975; 410: 243–251
- Osborne HH, Hollaway MR. The investigation of substrate-induced changes in subunit interactions in glyceraldehyde 3-phosphate dehydrogenases by measurement of the kinetics and thermodynamics of subunit exchange. Biochem J 1975; 15: 37–45
- Mercer WD, Winn SI, Watson HC. Twinning in crystals of human skeletal muscle D-glyceraldehyde-3-phosphate deyhdrogenase. J Mol Biol 1976; 104: 277–283
- Gyulai L, Dora E, Kovach AG. NAD/NADH: Redox state changes on cat brain cortex during stimulation and hypercapnia. Am J Physiol 1982; 243: H619–H627
- Statler KD, Janesko KL, Melick JA, Clark RS, Jenkins LW, Kochanek PM. Hyperglycolysis is exacerbated after traumatic brain injury with fentanyl vs. isoflurane anesthesia in rats. Brain Res 2003; 994: 37–43
- Janin J, Chothia C. Role of hydrophobicity in the binding of coenzymes. Biochemistry 1978; 17: 2943–2948
- Nishikawa K, MacIver MB. Agent-selective effects of volatile anesthetics on GABAA receptor-mediated synaptic inhibition in hippocampal interneurons. Anesthesiology 2001; 94: 340–347