Abstract
Fatty acid synthase (FAS) is a very significant lipogenic enzyme participating in energy metabolism in vivo and has been reported as a potential new therapeutic target for cancer treatment. The extracts from sixteen Aceraceae were prepared to assay their inhibitory activities against duck liver FAS and their correlated antitumor bioactivity. Their inhibition of FAS was composed of a reversible fast-binding inhibition, by which 0.41 μg/mL of the A. campestre extract inhibits 50% FAS activity, and an irreversible slow-binding inhibition with inactivation rate constants, kobs, ranging between 1.5 × 10− 3 and 10.6 × 10− 3 min− 1. Three Aceraceae extracts were selected from their smaller IC50 values to study different type of inhibitions against the three substrates in the FAS overall reaction. As compared with other reported FAS inhibitors including EGCG with regard to inhibition constant and IC50 value, the extracts appeared to be more efficient inhibitors, and exhibited a considerable inhibition against the growth of five types of cancer cells (China patent application number 200610088901.6), which may be related to the inhibition of lipogenesis in these cells.
Introduction
The de novo synthesis of fatty acids is essential for all organisms. Animal fatty acid synthase (E.C. 2.3. l.85, FAS) which plays an important role in fatty acid synthesis, one of the most complex cellular multienzymes, comprises two identical, 260–270kDa subunits juxtapositioned head to tail, each containing an acyl carrier protein and seven enzymatic active sites, including acetyl transacylase, malonyl transacylase, β-ketoacyl synthase, β-ketoacyl reductase, β–hydroxyacyl dehydratase, enoyl reductase, and thioesterase [Citation1]. Animal FAS catalyses the de novo synthesis of fatty acids from acetyl-CoA and malonyl-CoA in the presence of NADPH through the reaction which elongates the acetyl group by C2 units derived from malonyl-CoA in a stepwise and sequential manner [Citation2]. The overall architecture of mammalian FAS has been revealed by 4.5 Å resolution x-ray crystallography at intermediate resolution [Citation3].The amino acid sequence of human FAS has 79% and 63% identity with those of the rat and fowl enzymes, respectively [Citation4]. Traditional fowl FAS is a good model for the kinetic study of animal FAS.
It was reported that FAS is selectively highly expressed in certain human cancer cells, including carcinoma of the breast, prostate, colon, ovary, endometrium and lung Citation5-14. On the basis of much research, FAS has been suggested as a potential new therapeutic target for the treatment of cancer Citation15-16 and may lead to new chemotherapeutic methods which kill cancer cells but are non-toxic to normal cells Citation17-18. Inhibitors of FAS such as cerulenin, synthesized C75 [Citation19], thiolactomycin and its structural analogues [Citation20], and some natural polyphenols Citation21-24 are known for their effects on some human cancers. However, efficient FAS inhibitors, especially prepared from natural plants are still scarce and novel [Citation25].
The Acer genus (Aceraceae) consists of approx. 200 species widely distributed in the northern hemisphere with a distribution centre in China Citation26-27. They are horticulturally important and widely planted for the brilliant autumn colours of their leaves. Most of them has been used as Chinese folk medicine [Citation28]. Acer mono leaves [Citation29], Acer palmatum leaves [Citation30], Acer albopurpurascens [Citation31] and Acer truncatum Bunge show a high antioxidant effect [Citation32]. It was found that compared with extracts from control samples either Acer rubrum, Acer saccharum, or Acer saccharinum extracts increased methaemoglobin formation [Citation33]. Acer mono leaves showed significant hepatoprotective activities against H2O2-induced toxicity in primary cultures of rat hepatocytes[Citation34]. Acer nikoense leaves exhibited inhibitory effects on the release of beta-hexosaminidase in RBL-2H3 cells [Citation35]. Furthermore, Acer okamotoanum Nakai leaves was found to potently inhibit HIV-1 integrase [Citation36].
Recently, we have demonstrated that the extract of the leaves of Acer truncatum Bunge appeared to be an efficient inhibitor of FAS, and exhibited considerable inhibition of the growth of four types of cancer cells [Citation37]. In this paper, we report the potent inhibitory effects on FAS of the ethyl acetate extracts of sixteen species of Acer Linn plants which were collected from Beijing Arboretum, China, and the investigations on their inhibition kinetics as well as antitumor activity experiments.
Materials and methods
Preparation of FAS and substrates
Acetyl-CoA and malonyl-CoA were purchased from Sigma, NADPH was from Fluka. All other reagents were local products of analytical grade. The FAS from duck liver was purified with an improved method [Citation38]. The preparation was homogeneous on polyacrylamide gel electrophoresis in the presence and absence of SDS. FAS with specific activity of 150 U/mg was stored in 0.1 M potassium phosphate buffer of pH 7.0 containing 10 mM DTT and 1.0 mM EDTA, and then during all the following experiments, 1.0 mM DTT and 1.0 mM EDTA were added to the reaction solutions to protect the essential thiol groups of FAS and to remove metal ions which could inactivate FAS.
Assay of FAS activity
The assay for FAS activity, described [Citation39] previously, was performed with a Shimadzu UV2550 double wavelength/double beam spectrophotometer at 37°C by following the decrease of NADPH at 340 nm. The reaction system contains 0.1 M potassium phosphate buffer, pH 7.0; 1.0 mM EDTA; 1.0 mM DTT; 3μM acetyl-CoA; 10μM malonyl-CoA; 32μM NADPH and duck liver FAS 10μg in a total volume of 2.0 mL. Fast-binding inhibition was determined by adding the inhibitor (the extracts from each species) to the reaction system before FAS initiated the reaction. After the enzyme solutions were mixed with various concentrations of the extracts, aliquots were taken to measure the remaining activity at the indicated time intervals to follow slow-binding inactivation.
Preparation of the extracts from the leaves of Acer Linn Plants
The leaves of Acer Linn Plants were collected from Beijing Arboretum in October 2005 and identified by Professor Chen Yuting of Beijing University of Traditional Chinese Medicine. After appropriate treatment, the cleaned and dried leaves were cut into small pieces and mixed with 70% ethanol in the ratio of 1 to 20 (w/v) followed by magnetic stirring for 24 h at room temperature. A brown concentrated solution was obtained on removal of ethanol under reduced pressure which was then mixed with an equal volume of petroleum ether to remove coloring matter. The extraction was repeated three times to give a residual solution free of lipid which was then extracted thrice with EtOAc of equal volume. The pooled EtOAc extract was evaporated under reduced pressure to yield a brown residue which was further dried in a vacuum at a temperature less than 50°C.
Measurement of inhibition of FAS
IC50 values were obtained from the % inhibition-concentration curves for the inhibitors. Inhibition of FAS by different concentrations of inhibitor solution was measured and repeated three times. Reversible inhibition was measured by adding 5μL sample of extracts to the reaction system followed by 10 μg FAS to start the reaction in a total volume of 2 mL. The remaining activity of FAS was assayed as ai, and the control activity with 5μL solvent instead of extract was assayed as ao. The inhibition is also termed fast-binding inhibition and the inhibition extent was calculated by the formula: If = (1 − ai/ao) × 100%. This inhibition is caused by the non-covalent fast combination of inhibitor with enzyme and is usually reversible.
The time course of inactivation was determined by taking aliquots to measure residual activity at the indicated time intervals after the enzyme solution was mixed with the inhibitors in 0.1 M potassium phosphate buffer, pH 7.0. The time-dependent inhibition course is often an irreversible process to form a covalent bond between the inhibitor and enzyme known as slow-binding inactivation. The vehicles without inhibitors were used as control in these experiments. FAS activity in the control remained unchanged for 4 h. The apparent first-order inactivation rate constant, kobs, was obtained from the semilogarithmic plot of the inactivation time course. The time course indicates the actual inhibitory effect of the inhibitor, i.e., the more rapid the loss of enzyme activity, the more potent the inhibitior.
The experiments with human cancer cell lines
The inhibitory effects of the extracts on cancer cell growth were investigated using human esophageal cancer cell line (CAES-17), gastric cancer cell line (BGC-823), breast cancer cell line (MCF-7), and the liver cancer cell line (BEL-7402) as well as the human prostate cancer cell line (PC-3M). They were all purchased from the Institute of Medicament Research of Chinese Academy of Medical Sciences. The above various types of cancer cells were seeded in RPMI-1640 containing 10% fetal bovine serum, 100 μg/mL penicillin, and 100 μg/mL streptomysin on a 96-well plate with 2 × 105 cells in each well and incubated at 37°C for 24 h in a CO2 incubator. The plant extracts were then dissolved in dimethylsulfoxide at a preset concentration and added to the cell culture; the control with 200 μL solvent instead of extract was also assayed. The incubation proceeded for an additional 24 h. Cell viability was determined by the MTT [3-(4,5-dimethylthiazol-2-yl)-2,5-diphenyl-2H-tetra- zolium bromide] assay [Citation40]. The extent of inhibition for each extract concentration is an average value of three independent experiments. The above experiments were carried out with extracts from Acer tataricum, Acer platanoides, Acer capillipes, Acer campestre, and Acer pseudoplatanus.
Results
Fast-binding inhibition of FAS by extracts of sixteen species of Acer
The IC50 (the inhibitor concentration inhibiting 50% of the activity of FAS) values for extracts from sixteen species of Acer indicated their fast binding inhibitory capacities (see ).The IC50 values were obtained from the % inhibition-concentration curves for the inhibitors (). The results showed that Acer tataricum, Acer platanoides, Acer capillipes, Acer pseudoplatanus, Acer negundo and Acer campestre possessed highest inhibitory activities, and that Acer campestre was the most potent inhibitor with an IC50 value of 0.41 μg/mL, 86-fold less than that of Acer negundo ‘Vureomarginatum’.
Table I. The reversible inhibition activity of extracts of Aceraceous plants.
Figure 1 Fast-binding inhibition of FAS by the extracts of above six species of Aceraceous plants:(A) Acer tataricum, (B) Acer platanoides,(C) Acer capillipes,(D) Acer pseudoplatanus, (E) Acer negundo, (F) Acer campestre. (•) the overall reaction, (♦) ketoacyl reduction reaction, and (▴) enoyl reduction reaction. The reaction system contains 0.1 M potassium phosphate buffer, pH 7.0; 1.0 mM EDTA; 1.0 mM DTT; 3μM acetyl-CoA; 10μM malonyl-CoA; 32μM NADPH and FAS 20 μg in a total volume of 2.0 mL, 37°C, by following the decrease of NADPH at 340 nm within 1.5 min.
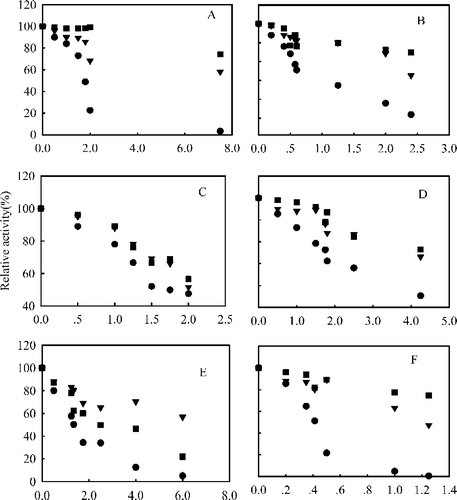
The extracts of the above six species of aceraceous plants were further used for measurement of their IC50 values for the FAS ketoacyl and enoyl reduction reactions (). As shown in , compared to the considerable inhibition against the FAS overall reaction, the inhibition of the enoyl reduction reaction was not obvious for both Acer tataricum and platanoides, but for all Acer extracts the FAS ketoacyl reduction reaction appeared to be inhibited with further increasing concentrations of extracts. It is worthy to note that with increasing concentration of extracts of Acer capillipes and Acer pseudoplatanus the inhibition of the enoyl- and the ketoacyl reduction reactions were enhanced almost to the same extent (,C, D). Moreover, the inhibition by Acer negundo of the ketoacyl reduction reaction became actually not as much as that of the enoyl reduction reaction. The IC50 values of these six species of Acer for the FAS overall reaction and ketoacyl reduction reaction are listed in .
Table II. IC50 values of extracts of six species of Aceraceous plants for the FAS overall reaction and ketoacyl reduction reaction, and kobs (min−1) values.
The inhibition time courses of FAS by Acer extracts
The time courses of inhibition of the FAS overall reaction are shown in . They are biphasic. In the first three minutes FAS activity rapidly decreased by 20–40%, which is attributed to fast and reversible binding to the enzyme before the time-dependent irreversible step which further decreased FAS activity. The semilogarithmic plot of relative activity (ln R.A.) versus time (insert of ) exhibited two phases and the line, initially appeared concave, but later became linear which gave the inactivation rate constant, kobs, equal to the fitted slope of the plots. The kobs values are listed in . Although the IC50 value for the FAS overall reaction of A. tataricum approached those of A. capillipes and A. pseudoplatanus, the smaller kobs value demonstrated its weak slow-binding irreversible inhibition of FAS.
Figure 2 Kinetic time courses of inhibition of the overall reaction in the presence of the extracts of six species of Acer:(○) Acer platanoides, (▴) Acer pseudoplatanus, (*) Acer tataricum,( × ) Acer campestre, (♦) Acer truncatum, and (▪) Acer capillipes. The insert is a plot of ln R.A.(relative activity) versus time calculated from Figure1 data (Acer platanoides). The FAS solution (0.6μM) was mixed with the extract (0.5 μg/mL) and the aliquots were assayed for remaining activity at the indicated time intervals.
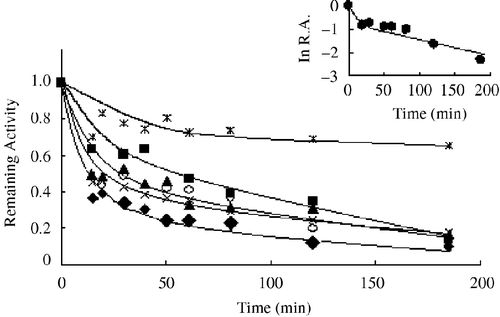
The inhibition pattern studies
Considering the smaller IC50 value representing a higher fast binding inhibitory capacity, A. Platanoids and A. Campestre were selected for studying the inhibition patterns against the FAS overall reaction in the presence of three fixed extract concentrations and NADPH, Malonyl-CoA, and Acetyl-CoA as the variable substrates, () and for estimating the respective kinetic parameters which were calculated by secondary plots on the basis of and listed in . It can bee seen from that the extract of Acer platanoides was a competitive inhibitor of acetyl-CoA, but for malonyl-CoA nearly noncompetitive while for NADPH the extract appeared to be a mixed type of inhibitor (). The extract of Acer campestre is nearly a noncompetitive inhibitor of acetyl-CoA (), whereas it was a mixed type inhibitor against malonyl-CoA and NADPH ().
Figure 3 Lineweaver-Burk plot of the inhibition of the overall reaction of FAS by A. platanoides extracts. The concentration of extract in the reaction system was 0 (0), 0. 25 μg/mL (1), 0.5 μg/mL (2), and 0.75 μg/mL (3). The FAS concentration was 0.012μM, and the fixed concentrations of NADPH and Malonyl-CoA were 32 and 10μM, respectively. The reaction rate was the concentration of NADPH per min consumed in the overall reaction.
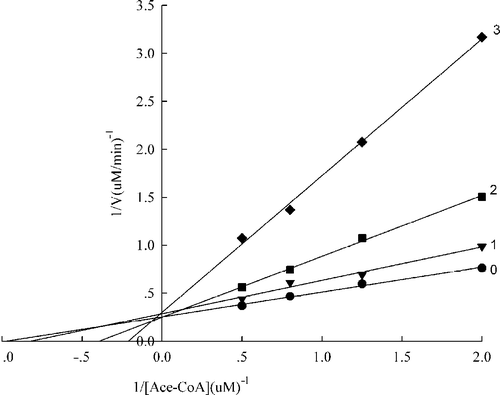
Figure 4 Lineweaver-Burk plot of the inhibition of the overall reaction of FAS by A. platanoides extract. The concentration of extract in the reaction system was 0 (0), 0. 25 μg/mL (1), 0.5 μg/mL (2), and 0.75 μg/mL (3). The FAS concentration was 0.012μM, and the fixed concentrations of Malonyl-CoA and Acetyl-CoA were 10 and 2.5μM, respectively. The reaction rate was the concentration of NADPH per min consumed in the overall reaction.
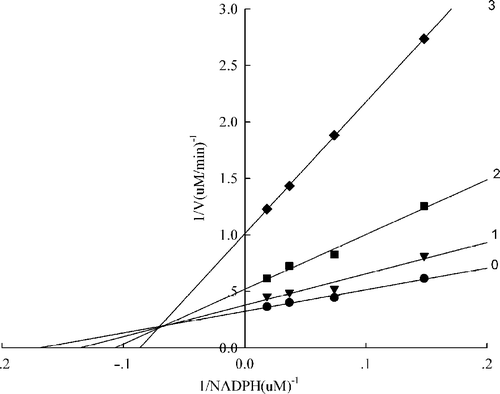
Figure 5 Lineweaver-Burk plot of the inhibition of the overall reaction of FAS by A. platanoides extract. The concentration of extract in the reaction system was 0 (0), 0. 25 μg/mL (1), 0.5 μg/mL (2), and 0.75 μg/mL (3). The FAS concentration was 0.012μM, and the fixed concentrations of NADPH and Acetyl-CoA were 32 and 2.5μM, respectively. The reaction rate was the concentration of NADPH per min consumed in the overall reaction.
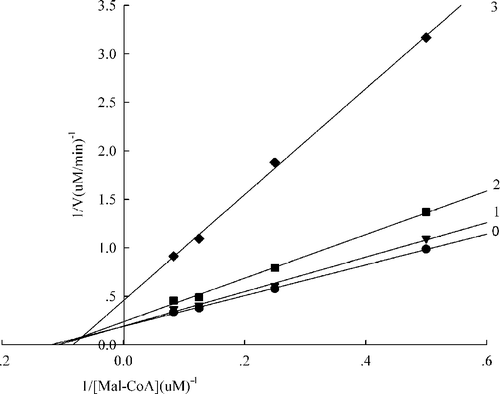
Figure 6 Lineweaver-Burk plot of the inhibition of the overall reaction of FAS by A. campestre extract. The concentration of extract in the reaction system was 0 (0), 0. 2 μg/mL (1), 0.4 μg/mL (2), and 0.5 μg/mL (3). The FAS concentration was 0.012μM, and the fixed concentrations of NADPH and Malonyl-CoA were 32 and 10μM, respectively. The reaction rate was the concentration of NADPH per min consumed in the overall reaction.
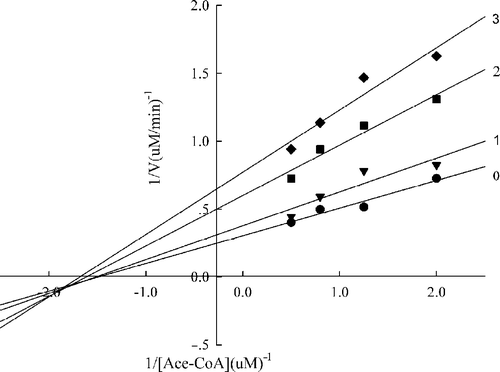
Figure 7 Lineweaver-Burk plot of the inhibition of the overall reaction of FAS by A. campestre extracts.The concentration of extracts in the reaction system was 0 (0), 0. 2 μg/mL (1), 0.4 μg/mL (2), and 0.5 μg/mL (3). The FAS concentration was 0.012μM, and the fixed concentrations of NADPH and Acetyl-CoA were 32 and 2.5μM, respectively. The reaction rate was the concentration of NADPH per min consumed in the overall reaction.
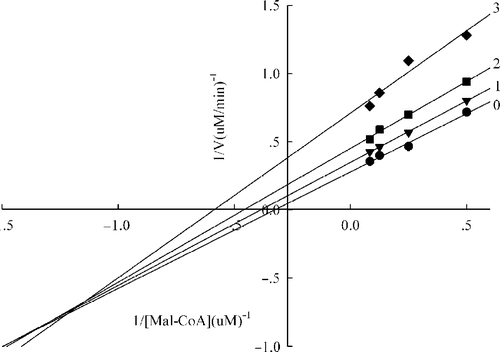
Figure 8 Lineweaver-Burk plot of the inhibition of the overall reaction of FAS by extracts.The concentration of extracts in the reaction system was 0 (0), 0. 2 μg/mL (1), 0.4 μg/mL (2), and 0.5 μg/mL (3). The FAS concentration was 0.012μM, and the fixed concentrations of Malonyl-CoA and Acetyl-CoA were 10 and 2.5μM, respectively. The reaction rate was the concentration of NADPH per min consumed in the overall reaction.
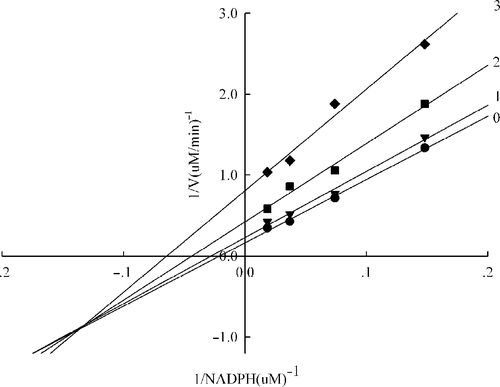
Table III. The inhibition types for the extracts from Aceraceous plants against the FAS overall reaction.
Effects of five species of Acer extracts on growth of various cancer cell lines
Effects of extracts of five species of Acer on cancer cells growth were investigated using CAES-17, BGC-823, MCF-7, BEL-7402, and PC-3M cells. Treatment with serial dilutions of Acer extracts at 5–80 μg/mL exhibited significant growth inhibition on all these cells, and the extent of inhibition exceeded 50% when the extract concentration reached 60 μg/mL () In addition, the IC50 of Acer tataricum and Acer capillipes for MCF-7 and PC-3M cells was only 9 and 11 μg/mL, respectively.
Table IV. The IC50 of the extracts of five species of Acer against various cancer cells.
Discussion
In the present work we have shown that extracts from sixteen species of Acer leaves inhibit the overall reaction and ketoacyl reduction of FAS effectively and significantly inhibited growth of cancer cells. These enzyme inhibition involved both reversible and irreversible inhibition. Especially for the fast-binding inhibition, the IC50 values of the extracts of six species (Table II) for both the overall reaction and ketoacyl reduction reaction of FAS clearly demonstrate that the inhibitory effects of the extract remarkably exceed those of polyphenols like NDGA [Citation41].
It can be seen that the IC50 of A. capillipes for ketoacyl reduction reaction at 2.07 μg/mL is close to that for the FAS overall reaction (1.5 μg/mL) implying that inhibition of the FAS overall reaction is mainly ascribed to the inhibition of the ketoacyl reduction reaction, which is also supported by . However for other Acer species the several fold discrepancy between the IC50 values for the ketoacyl reduction reaction and for the FAS overall reaction implied that inhibition of ketoacyl reduction is not the predominant reason for FAS inactivation and other intermediate step-reactions of FAS are also somewhat inhibited. As summarized in Table III for the overall reaction of FAS, there are different types of inhibition between the extracts using the three substrates (Table III). Extracts show inhibitiory effect on many steps of the FAS overall reaction, including acetyl transacylation and malonyl transacylation and β-ketoacyl reduction.
The synthesized C75 and cerulenin are the main well-known inhibitors of FAS. C75 showed only irreversible inhibition against FAS without obvious reversible inhibition [Citation11]. The reported IC50 value of cerulenin for FAS was 20 μg/mL [Citation42] which is larger than that for any of the 15 species of Acer listed in Table 1, except for A. negundo ‘Vureomarginatum’.
In recent years gallated catechins such as EGCG, ECG have become known for their inhibitory effects on FAS and have been extensively studied Citation43-45. The IC50 of EGCG and ECG for the FAS overall reaction are 24 and 18 μg/mL while for the ketoacyl reduction reaction their values are 46 and 30 μg/mL [Citation46], respectively, obviously larger than those for the six species of Acer shown in Table II. The extracts as compared with EGCG in the values of their inhibition constants (ki) (Table III) were demonstrated to be much more potent on FAS. The ki values of A. platanoides for acetyl-CoA, malonyl-CoA, and for NADPH are several hundredfold smaller than those of EGCG, showing A. platanoides's much more potent fast-binding inhibitory capacity. Overall, for the FAS overall reaction the aceraceous plants possess more inhibitory capacity than these catechins.
It was reported that some Chinese medicinal herbs such as Loranthus Parasiticus and green tea have inhibitory effects on FAS Citation46-47. The IC50 values of their extracts were 0.48 and 12.2 μg/mL, respectively. In comparison with reported medicinal herbs, the average value of IC50 for the aceraceous plants in Table II which are comparable in fast-binding inhibitory capacity, 1.20 μg/mL, is remarkably less than that for the above herbal inhibitors.
Wang and Tian reported that EGCG acts on the binding site of NADPH in β-ketoacyl reductase, and is a specific inhibitor of ketoacyl reductase [Citation48].
The IC50 values of extracts for the ketoacyl reduction reaction are within the range 1.21–7.56 μg/mL; but for the FAS overall reaction the IC50 values range from 0.60–1.81 μg/mL (Table II). This remarkable difference in IC50 values indicates that, except for A. capillipes, in contrast with EGCG, the inhibition of ketoacyl reduction is not the main reason for FAS inactivation. Inactivation of FAS could rather be attributed to the cooperative effect of the components of extracts on the intermediate step-reactions of FAS. Overall, Aceraceae extracts are more efficient inhibitors of FAS.
For the overall reaction of FAS there are different inhibition patterns (Table III) between the extracts of A. Platanoids and A. campestre using the three substrates. The extracts of Acer campestre and Acer platanoides show inhibitory effect on the intermediate steps of the FAS overall reaction, including acetyl transacylation, malonyl transacylation, β-ketoacyl reduction.
Up-regulation of FAS occurs early in tumor development and is further enhanced in more advanced tumors including prostate and breast carcinomas, ovarian and endometrium cancers [Citation49]. In addition, high FAS expression levels often predict a poor outcome for cancer patients. Recently, FAS hyperactivity was also found in squamous cell carcinoma of the lung and intestinal metaplasia of the stomach [Citation50]. Pharmacological inhibition of tumour-associated FAS hyperactivity is under investigation as a chemotherapeutic target. The above five types of cancer cells other than breast cancer and prostate cancer are non sex-steroid-related, but were similarly inhibited by the extracts (Table IV), which may be related to the inhibition of lipogenesis in these cells. Brusselmans [Citation51] et al investigated the EGCG inhibition of FAS in cultured prostate cancer cells and how this inhibition affected endogenous lipid synthesis, cell proliferation and cell viability. Their findings established EGCG as a potent natural inhibitor of FAS in intact cells and strengthen the molecular basis for the use of EGCG as a chemopreventive and therapeutic antineoplastic agent.
Historically, traditional herbal medicine has gained much practical experience for the control of tumors and understanding their physiological and biochemical mechanism is important for the application of the natural product in the cancer preventive field. We believe that these natural products represent a promising source of new therapies for tumors.
Abbreviations | ||
FAS: | = | fatty acid synthase |
EGCG: | = | (-)-epigallocatechin gallate |
DTT: | = | dithiothreitol |
EtOAc: | = | ethyl acetate |
NDGA: | = | nordihydroguaiaretic acid |
Acknowledgements
The present investigation was supported by the Scientific Research Common Program of Beijing Municipal Commission of Education of China No. KM200410025013 and National Natural Science Foundation of China No. 30572252.
References
- Wakil SJ, Stoops JK, Joshi VC. Ann Rev Biochem 1983; 52: 537–553
- Wakil SJ. Biochem 1989; 28(11)4523–4530
- Maier T, Jenni S, Ban N. Science 2006; 311: 1258–1262
- Jayakumar A, Tai MH, Huang WY, al-Feel W, Hsu M, Abu-Elheiga L, et al. Proc Natl Acad Sci USA 1995; 92(19)8695–8699
- Swinnen JV, Roskams T, Joniau S, Van Poppel H, Oyen R, Baert L, et al. Int J Cancer 2002; 98(1)19–22
- Wang Y, Kuhajda FP, Li JN, Pizer ES, Han WF, Sokoll LJ, et al. Cancer Lett 2001; 167(1)99–104
- Vlad LD, Axiotis CA, Merino MJ. Mod Pathol 1999; 12: 70–75
- Piyathilake CJ, Frost AR, Manne U. Hum Pathol 2000; 31(9)23–28
- Innocenzi D, Alo PL, Balzani A, Balzani A, Sebastiani V, Silipo V, et al. J Cutan Pathol 2003; 30(1)23–28
- Visca P, Sebastiani V, Pizer ES, Botti C, De Carli P, Filippi S, et al. Anticancer Res 2003; 23(1A)335–339
- Kuhajda FP, Pizer ES, Li JN, Mani NS, Frehywot GL, Townsend CA, et al. Proc Natl Acad Sci USA 2000; 97(7)3450–3454
- Pizer ES, Wood FD, Heine HS, Romantsev FE, Pasternack GR, Kuhajda FP, et al. Cancer Res 1996; 56(12)1189–1193
- Pizer ES, Chrest FJ, Digiuseppe JA, Han WF. Cancer Res 1998; 58(20)4611–4615
- Kuhajda FP. Nutrition 2000; 16(3)202–208
- Pizer ES, Thupari J, Han WF. Cancer Res 2000; 60(2)213–218
- Menendez JA, Oza BP, Atlas E, Verma VA, Mehmi I, Lupu R. Oncogene 2004; 23(28)4945–4958
- Bagli E, Stefaniotou M, Morbidelli L, Ziche M, Psillas K, Murphy C, et al. Cancer Res 2004; 64: 7936–7939
- Vergote D, Cren OC, Chopin V, Toillon RA, Rolando C, Hondermarck H, et al. Breast Cancer Res 2002; 76(3)195–198
- Gabrielson EW, Pinn ML, Testa JR, Kuhajda FP. Clin Cancer Res 2001; 7(1)153–157
- Mcfsdden JM, Medghalchi SM, Thupari JN, Pinn ML, Vadlamudi A, Miller KI, et al. J Med Chem 2005; 48: 946–961
- Li BH, Tian WX. J Biochem (Tokyo) 2004; 135: 85–91
- Li BH, Tian WX. J Enz Inhib Med Chem 2003; 18: 349–356
- Wang X, Song KS, Guo QX, Tian WX. Biochem Pharm 2003; 66: 2039–2047
- Wang X, Tian WX. Biochem Biophys Res Commun 2001; 288: 1200–1206
- Tian WX, Li LC, Wu XD, Chen CC. Life Sci 2004; 74(19)2389–2399
- Ji SB, Saito N, Yokoi M, Shigihara A, Honda T. Phytochem 1992; 31: 665–668
- Ji SB, Yokoi M, Saito N, Ueda Y, Shigihara A, Honda T. Tech Bull Fac Hort Chiba Univ 1995; 49: 13
- Shi WSh, Lei X. China Trad Patent Med 2003; 25(11)948–950
- Yang H, Lee MK, Kim YC. J Agr Food Chem 2005; 53(10)4182–4186
- Kim HJ, Lee BC, Kim JH, Sim GS, Lee DH, Lee KE, et al. Arch Pharm Res 2005; 28(2)195–202
- Lee MH, Jiang CB, Juan SH, Lin RD, Hou WC. Fitoterapia 2006; 77: 109–105
- Wei Q, Ma XH. Food Sci 1998; 19(8)3–6
- Boyer JD, Breeden DC, Brown DL. Am J Veter Res 2002; 63(4)604–610
- Yang H, Sung SH, Kim YC. J Nat Prod 2005; 68(1)101–103
- Morikawa T, Tao J, Ueda K, Matsuda H, Yoshikawa M. Chem Pharm Bull 2003; 51(1)62–67
- Kim HJ, Woo ER, Shin CG, Park HJ. Nat Prod 1998; 61: 145–148
- Zhao WH, Zhang JF, Wang Zh, Zhang YX, Tian WX. J Enz Inhib Med Chem 2006; 21(5)589–596
- Tian WX, Wang YS, Hsu RY. Biochim Biophys Acta 1989; 998: 310–316
- Tian WX, Hsu RY, Wang YS. J Biol Chem 1985; 260(20)11375–11387
- Mosmann T. J Immunol Meth 1983; 65(1–2)55–63
- Li BH, Ma XF, Wang Y, Wang X, Tian WX. J Biochem 2005; 138(5)679–686
- Vance D, Gpldberg I, Mitsuhashi O. Biochem Biophys Res Commun 1972; 48: 649–655
- Zhang YM, Rock CO. J Biol Chem 2004; 279(30)994–1001
- Wang X, Song KSH, Guo QX, Tian WX. Biochem Pharm 2003; 66(10)2039–2047
- Du YT, Wang X, Wu XD, Tian WX. J Enz Inhib Med Chem 2005; 20(4)349–356
- Zhang R, Xiao WP, Wang X, Wu XD, Tian WX. Biotech Appl Biochem 2006; 43(1)1–7
- Wang Y, Zhang SY, Ma XF, Tian WX. J Enz Inhib Med Chem 2006; 21(1)87–93
- Wang X, Tian WX. Biochem Biophys Res Commun 2001; 288: 1200–1206
- Pizer ES, Pflug BR, Bova GS. Prostate 2001; 47(2)102–110
- Kusakabe T, Nashimoto A, Honma K, Suzuki T. Histopath 2002; 40(1)71–79
- Brusselmans K, De Schrijver E, Heyns W, Verhoeven G, Swinnen JV. Int J Cancer 2003; 106(6)856–862