Abstract
Cholinesterases are divided into two classes based on differences in their substrate specificity and tissue distribution: acetylcholinesterase (AChE) and butyrylcholinesterase (BChE). These enzymes may be inhibited by several compounds, such as antidepressants. The antidepressants paroxetine, imipramine, clomipramine and sertraline inhibited both venom AChE as well as human serum BChE in a concentration-dependent manner but had no effect on AChE in the rat brain striatum. The IC50 of venom calculated for imipramine was 0.3 mM, paroxetine 0.38 mM, clomipramine 0.34 mM and sertraline 0.35 mM. Analysis of kinetic data indicated that the inhibition caused by sertraline and paroxetine was mixed, i.e. Km values increased and Vmax decreased in a concentration dependent manner. Imipramine and clomipramine exhibited competitive inhibition, i.e. Km values increased and Vmax remained constant. The present results suggest that these therapeutic agents used for depression can also be considered as inhibitors of snake venom and human serum cholinesterase.
Introduction
Cholinesterases are divided into two classes based on differences in their substrate specificity; acetylcholinesterase or “true cholinesterase” (AChE; acetylcholine acetyl hydrolase, E.C.3.1.1.7) and butyrylcholinesterase (BChE; acyl choline acylhydrolase, E.C.3.1.1.8). BChE is also known as pseudo cholinesterase, non specific cholinesterase or simply cholinesterase. AChE hydrolyses acetylcholine faster than other cholinesterases and is very less active on butyrylcholine [Citation1,2]. The hydrolysis of the neurotransmitter acetylcholine (ACh) by AChE in the nervous system is known to be one of the most efficient enzyme catalytic reactions. Ligand-binding studies suggest that the active centre of cholinesterase is composed of a cationic esteratic subsite containing the active serine, an anionic site which accommodate the choline moiety of ACh and a peripheral anionic site [Citation3,4]
In mammals, AChE is abundant in the brain, muscles and erythrocyte membranes, whereas BChE has higher activity in the liver, intestine, heart, kidney and lung [Citation5–7]. The physiologic function of human BChE is still unknown; but it can take the place of AChE in acetylcholine (ACh) degradation when AChE is inhibited or absent [Citation8]. AChE and BChE share 65% amino acid sequence homology and have a similar molecular form and their substrate hydrolysis is carried out by the “catalytic triad” of Ser, His and Glu in the active centre of both types of enzymes [Citation9]. The 3-D structure of the enzyme showed the long and narrow active site gorge is about 20 A° deep and includes two sites of ligand interaction: an acylation site at the base of the gorge with the catalytic triad and a peripheral site in its mouth. Some ligands can bind specifically to the acylation or to the peripheral site, and ternary complexes with different ligands bound to each site can be formed [Citation10–12.
Elapidae snake is found in several parts of the world [Citation13]. Bungarus venom contains 747000 Ellman's units per g of dry venom of AChE-like activity, being one of the richest venoms in this activity [Citation14]. It is non toxic to mice even at very high doses, and does not reinforce the toxicity of other venom components, thus venom enzyme provides an excellent model for analyzing catalytic mechanism of AChE [Citation15]. In the literature there are reports indicating that antidepressants are inhibitors of cholinesterases from different sources [Citation16,17]. The kinetic and specific fluorescent probe propidium study indicate that the tricyclic antidepressants drugs (TCA) amitriptyline and nortriptyline interact at the peripheral anionic site of Electrophorus electricus AChE [Citation18].
In the present study three different classes of antidepressants were selected. Imipramine and clomipramine are tricyclic antidepressants. During depression, it occurs a decrease in the amount of the chemicals noradrenaline and serotonin released from nerve cells in the brain. The release of these chemicals results in a mood exciting effect. Imipramine and clomipramine both pharmacologically act by stopping these chemicals from going back into the nerve cells. Paroxetine (a phenylpiperidine derivative) and sertraline are selective serotonin reuptake inhibitor (SSRIs), commonly used to treat the symptoms of depression [Citation19].
The interaction of antidepressants with the cholinergic system is poorly related in the literature. It is known that, krait venom and rat brain striatum are rich source of AChE and human serum have high amount of BChE. In view of this, we selected three different rich sources of cholinesterase to study the interaction of these enzymes with the commonly used antidepressants, imipramine, clomipramine, paroxetine and sertraline.
Materials and methods
Materials
Acetylthiocholine iodide, DTNB [5,5′-dithiobis(2-nitro-benzoic acid)], ethopropazine, imipramine, were purchased from Sigma Chemical Co. (St.Louis, MO,USA). Sertraline, clomipramine and paroxetine were obtained from Galena (Campinas, SP, Brazil).
Animals
Male Wistar 3 to 6-month-old rats with a body weight of 300–400 g were kept in separate animal cages, on a 12 hr light/dark cycle, at a temperature of 22°C, with free access to food and water. All animal procedures were approved by the Institutional Commission of the Federal University of Santa Maria.
Human serum
Human serum was obtained from healthy volunteers, 20–28 years old, from both sexes from the University Hospital from Santa Maria, with written consent. The Human Ethics Committee of the Federal University of Santa Maria approved the protocol (Protocol number: 23081.006134/2006-81). None of the volunteers had a recurrent or past history of psychiatric illness, any significant medical disorder nor history of drug, cigarette or alcohol abuse. None of them had been taking any medication for at least one month. 6 mL of blood was collected from all participants in vaccutainer tubes, centrifuged at 3000 rpm for 10 min and the serum was collected and used for the enzyme assays.
Venom
Mature Krait (Bungarus sindanus) snakes (1–1.5 meter length) of both sexes were captured from wild by snake man with the help of specific capturing stick. The snakes were kept in separate animal cages, with free access to soil and water. Venom of two to three drops (300–350 microliters) was squeezed out manually from each snake, mixed, lyophilized immediately and stored at − 20°C for further use.
Cholinesterase assay
AChE and BChE activities were determined by the method of Ellman [Citation20] modified by Rocha [Citation21] using a Hitachi 2001 spectrophotometer. Hydrolysis rates (V) were measured at various acetylthiocholine (S) concentrations (0.01–1 mM) in 1 mL assay mixture with 62 mM phosphate buffer, pH 7.5, and 0.2 mM DTNB at 25°C. Twenty microliters of human serum (350 μg of protein), snake venom (2 μg of protein) and 50 μL of rat brain striatum (75 μg of protein) were added to the reaction mixture and pre-incubated for 30 min at 37°C. The experiments were performed separately with each source of AChE. The hydrolysis was monitored by the formation of the thiolate dianion of DTNB at 412 nm for 2–3 min (intervals of 10 s). For AChE activity, 0.06 mM ethopropazine (specific inhibitor of BChE) was used to inhibit the activity of BChE in snake venom as well as in rat brain striatum. All samples were run in duplicate or triplicate.
Protein determination
The protein content of the enzyme preparation was assayed by the method of Bradford [Citation22] using bovine serum albumin as a standard.
Kinetic determinations
The interaction of antidepressants and cholinesterase was determined using the Lineweaver–Burk [Citation23] double reciprocal plot, by plotting 1/V against 1/S analysed over a range of acetylthiocholine concentrations (0.01–1 mM) in the absence and in the presence of imipramine (0.3–0.5 mM), paroxetine (0.3–0.7 mM), clomipramine (0.3–0.7 mM), and sertraline (0.3 − 0.7 mM). Michaelis constants (Km) were determined by two different plots of 1/V vs. 1/S and V vs. V/S [Citation24,25] The values of inhibition constant (Ki) were obtained using Cornish-Bowden plots of S/V vs. [I]. IC50, was estimated at fixed substrate concentration (0.5 mM), according to the Dixon and Webb [Citation26] plot using 1/V vs. [I].
Statistical analysis
Statistical analysis was performed using one-way ANOVA, which was followed by post-hoc analysis (Duncan multiple range test). The difference was considered to be significant for P < 0.05.
Results
To compare the inhibitory potency of the different class of antidepressant against different sources of cholinesterase, 500 μM concentration of antidepressants imipramine, paroxetine, clomipramine and sertraline () were observed to modify the activity of snake venom as well as human serum cholinesterase () when using 0.4 mM substrate (a saturated concentration) in 1 ml assay mixture. In contrast, these antidepressants had no effect on rat brain striatum AChE () in the same conditions. The rate constants (Km) and Vmax for ACh hydrolysis versus substrate concentration were measured in the absence and the presence of antidepressants. Analysis of kinetic data indicated that the inhibition caused by paroxetine () and sertraline () in snake venom AChE was mixed, where the Km values increased and the Vmax decreased in a concentration dependent manner. Imipramine () and clomipramine () exhibited competitive inhibition, where the Km values increased and the Vmax remained the same. The concentration of antidepressants that inhibits 50% of enzymatic activity, IC50, was estimated at fixed substrate concentration (0.5 mM), the values of imipramine range from 0.3–0.5 mM while paroxetine, clomipramine and sertraline range from 0.3 to 0.7 mM. The concentrations required to inhibit 50% of AChE activity calculated by using the Dixon and Webb [Citation25] for venom AChE are depicted in . The values of inhibition constant (Ki) calculated by using Cornish-Bowden plots of S/V vs. [I] are also listed in .
Figure 1. Chemical structure of paroxetine (A), sertraline (B), imipramine (C) and clomipramine (D).
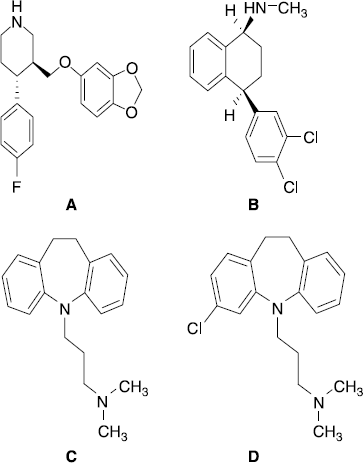
Figure 2. Acetylcholinesterase (AChE) and butrylcholinesterase (BChE) experiments in the presence of 0.5 mM of antidepressants (Clom; clomipramine, Sert; sertraline, Paro; paroxetine and Imip; imipramine). Hydrolysis rates were measured at 412 nm by using 0.4 mM substrate in 1 mL assay solutions with 62 mM phosphate buffer (pH 7.5) and 1 mM DTNB [5,5-dithiobis(2-nitronenzoic acid)]. Enzyme was preincubated for 30 min before 0.4 mM substrate addition. For AChE activity, 0.06 mM ethopropazine (specific inhibitor of BChE) was used to inhibit the activity of BChE in snake venom. All experiments were repeated at least three times and similar results were obtained. For snake venom AChE *P < 0.05 and for human serum BChE *P < 0.038. Significantly different from control.
![Figure 2. Acetylcholinesterase (AChE) and butrylcholinesterase (BChE) experiments in the presence of 0.5 mM of antidepressants (Clom; clomipramine, Sert; sertraline, Paro; paroxetine and Imip; imipramine). Hydrolysis rates were measured at 412 nm by using 0.4 mM substrate in 1 mL assay solutions with 62 mM phosphate buffer (pH 7.5) and 1 mM DTNB [5,5-dithiobis(2-nitronenzoic acid)]. Enzyme was preincubated for 30 min before 0.4 mM substrate addition. For AChE activity, 0.06 mM ethopropazine (specific inhibitor of BChE) was used to inhibit the activity of BChE in snake venom. All experiments were repeated at least three times and similar results were obtained. For snake venom AChE *P < 0.05 and for human serum BChE *P < 0.038. Significantly different from control.](/cms/asset/34ef5041-a603-4477-b995-ffbca0956622/ienz_a_281057_f0002_b.gif)
Figure 3. The graphs show rat brain striatum acetylcholinesterase experiments in the presence of 0.5 mM different antidepressants (Clom; clomipramine, Sert; sertraline, Paro; paroxetine and Imip; imipramine). Hydrolysis rates were measured at 412 nm by using 0.4 mM substrate in 1 mL assay solutions with 62 mM phosphate buffer (pH 7.5), and 1 mM DTNB [5,5-dithiobis(2-nitronenzoic acid)]. Enzyme was preincubated for 30 min before 0.4 mM substrate addition. 0.06 mM ethopropazine (specific inhibitor of BChE) was used to inhibit BChE activity. All experiments were repeated at least three times and similar results were obtained.
![Figure 3. The graphs show rat brain striatum acetylcholinesterase experiments in the presence of 0.5 mM different antidepressants (Clom; clomipramine, Sert; sertraline, Paro; paroxetine and Imip; imipramine). Hydrolysis rates were measured at 412 nm by using 0.4 mM substrate in 1 mL assay solutions with 62 mM phosphate buffer (pH 7.5), and 1 mM DTNB [5,5-dithiobis(2-nitronenzoic acid)]. Enzyme was preincubated for 30 min before 0.4 mM substrate addition. 0.06 mM ethopropazine (specific inhibitor of BChE) was used to inhibit BChE activity. All experiments were repeated at least three times and similar results were obtained.](/cms/asset/ce06af75-422c-4ba3-a95c-eb195f1c5e05/ienz_a_281057_f0003_b.gif)
Figure 4. Kinetic analysis of inhibition of snake venom acetylcholinesterase by Paroxetine (A) Sertraline (B) Imipramine (C) and Clomipramine (D).
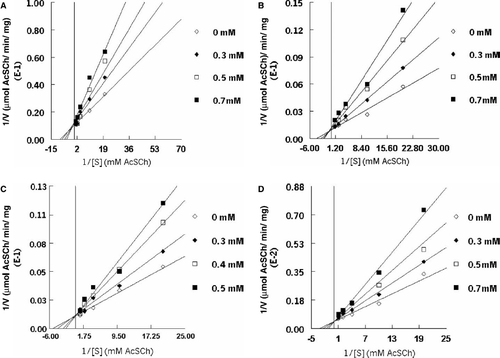
Table I. Ki and IC50 values for venom acetylcholinesterase.
Discussion
The major role of AChE is the rapid hydrolysis of acetylcholine after it release at the cholinergic synapses and a large number of drugs inhibit AChE activity prolonging the synaptic action of acetylcholine. Recently, we verified the inhibition of AChE from krait (Bungarus sindanus) venom and BChE from human serum by malathion, carbofuran, paraquat [Citation27] and tacrine [Citation28]. The three commonly prescribed compounds donepezil, rivastigmine and galantamine are used for the treatment of Alzheimer's disease. All these compound inhibit AChE while rivastigmine additionally inhibit BChE [Citation29,30]. Furthermore, fasciculin, BW284C51, propidium, and decamethonium are potent reversible inhibitors of AChE. These compounds prefer to bind at the peripheral site of the enzyme [Citation14].
The antidepressants drugs which exhibit anticholinesterase properties have great medical application [Citation31]. In the present study, different classes of antidepressants () inhibited cholinesterase in Bungarus sindanus venom as well as human serum () but had no effect on rat brain striatum AChE (). It may be due to different forms in which cholinesterase is found: ChEs may be in globular soluble forms, but can also be anchored to the neuronal membrane. These two main types of cholinesterasic forms may exhibit distinct hydrolytic profiles, and this fact may be the basis of the differences observed. The inhibitory potency of the antidepressants was imipramine > clomipramine > sertraline > depending on the nature of the enzyme source as well as the structure of compounds, which resulted in the diverse interactions with cholinesterase (BChE–serum or AChE–snake venom and rat brain striatum), mainly for primary and tertiary enzyme structure peculiarities. In the present study competitive inhibition was observed with venom AChE for imipramine () and clomipramine (), with different inhibition constant (), in this case the Km increased and the Vmax was unchanged. The kinetic analysis indicated a mixed inhibition for paroxetine () and sertraline (), it was clearly observed that the km values increased and Vmax decreased with increase of inhibitor concentration. Cholinesterase has two sites for substrate binding an acylation site or A-site and peripheral binding site or P-site [Citation32]. Recent literature from NMR spectroscopy and molecular dynamic study with some antidepressants revealed that these compounds prefer conformations in which the side chain is folded over the phenyl rings in a “scorpion-like fashion [Citation33] due to which conformation of the active site of cholinesterase is effected by occupation of peripheral anionic site, it is possible that sertraline and paroxetine act in a scorpion-like fashion, in order to bind with peripheral site or P-site and block the entry of substrate toward the active site of the enzyme. This is supported by the study of Harel [Citation34] which demonstrated that the crystal structure of tacrine (an inhibitor of AChE with a structure similar to that of sertraline) in AChE binds at the bottom of the active site gorge. In cholinesterase the substrate momentarily binds to the p-site and forms the ES-complex at the active site, due to its low affinity with the p-site, this fact contributes to catalytic processes by insuring that all substrate molecules which transiently bind to the p-site will proceed toward the A- site. The presence of any toxicant at peripheral site would block the access of substrate toward the active site [Citation35]. Imipramine () and clomipramine () compete with substrate to bind at the active site. As these antidepressants inhibited venom AChE competitively, only a binary (ChE.Drug or ChE.S) complex could be formed. The complex ChE.S liberates the product at a higher rate, while Vmax remains unchanged. We propose the following scheme for such type of interaction.
Paroxetine () and sertraline () bind other site than the active site i.e peripheral binding site or P-site. So, we propose the following scheme for the interactions between the sertraline and paroxetine with snake venom AChE.
Both binary (ChE.Drug or ChE.S) and ternary (ChE.Drug.S) complexes could be formed. The complex ChE.Drug.S liberates the product at a lower rate, decreasing the Vmax in the case of the mixed inhibition type.
In conclusion, the commonly used antidepressants (imipramine, paroxetine, clomipramine and sertraline) inhibited AChE of Bungarus sindanus venom and human serum BChE. However, these compounds did not inhibit AChE activity from the rat brain striatum, which may be related to subtle differences in the primary structure of the enzymes. Furthermore, the different cholinesterasic forms may exhibit distinct hydrolytic profiles, and this fact may be the basis of the differences observed. The venom of the Bungarus genera is the richest source of AChE in nature [Citation14] and, of medical significance an excellent model for analyzing catalytic mechanism of AChE. In this vein, kinetic studies with antidepressants, as cholinesterase inhibitors, could be useful for novel neuropharmacological purposes.
Acknowledgements
We wish to thank the Academy of Sciences for the Developing World (TWAS) and Conselho Nacional de Desenvolvimento Científico e Tecnológico (CNPq) for the fellowship awarded to Mushtaq Ahmed (TWAS-CNPq/Brazil-Pakistan), and FINEP research grant “Rede Instituto Brasileiro de Neurociência (IBN-Net)” # 01.06.0842-00.
References
- A Chatonnet, and O Lockridge. (1989). Comparision of butyrylcholinestrase and acetylcholinestrase. J Biochem 260:625–634.
- M Ekholm. (2001). Predicting relative binding free energies as substrate and inhibitors of acetyl and butyrylcholinestrase. J Mol Structure 572:25–34.
- JP Changeux. (1966). Response of acetylcholinesterase from Torpedo marmorata to salts and curarizing drugs. Mol Pharmacol 2:369–392.
- DJ Epstein, HA Berman, and P Taylor. (1979). Ligand-induced conformational changes in acetylcholinesterase investigated with fluorescence phosphonates. Biochemistry 18:4749–4754.
- KR Dave, AR Syal, and SS Katyare. (2000). Tissue cholinesterases. A comparative study of their kinetic properties. Z Naturforsch 55:100–108.
- CA Prody, D Zevin-Sonkin, A Gnatt, O Goldberg, and H Soreq. (1987). Isolation and characterization of full lenght cDNA clones coding for cholinestrase from fetal human tissues. Proc Natl Acad Sci USA 84:3555–3559.
- DJ Ecobicon, and AM Corneau. (1973). Pseudocholinestarase of mammalian plasma: Physichemical properties and organophosphate inhibition in eleven species. Toxicol Appl Pharmacol 24:29–100.
- B Li, JA Stribley, A Ticu, W Xie, LM Schopfer, P Hammond, S Brimijoin, SH Hinrichs, and O Lockridge. (2000). Abundant tissue butyrylcholinesterase and its possible function in the acetylcholinesterase knockout mouse. J Neurochem 75:1320–1331.
- V Chaliani, D Derr, B Earls, E Schmell, and T August. (1989). Purification and partial amino acid sequence analysis of human erythrocyte acetylcholinesterase. FEBS Lett 247 (2):279–282.
- T Szegletes, WD Mallander, PJ Thomas, and TL Rosenberry. (1999). Substrate binding to the peripheral site of acetylcholinesterase initiates enzymatic catalysis: Substrate inhibition arises as a secondary effect. Biochemistry 38:122–133.
- G Benzi, and A Moretti. (1998). Is there a rationale for the use of acetylcholinesterase inhibitors in the therapy of Alzheimer's disease?. Eur J Pharmacol 346:1–13.
- Y Bourne, P Taylor, and P Marchot. (1995). Acetylcholinesterase inhibition by fasciculin, crystal structure of the complex. Cell 83:503–512.
- HS Bawaskar, and PH Bawaskar. (2004). Envenoming by the common krait (Bungarus caeruleus) and Asian cobra (Naja naja): Clinical manifestations and their management in a rural setting. Wilderness Environ Med 15 (4):257–266.
- Y Frobert, C Créminon, X Cousin, M Rémy, J Chatel, S Bon, C Bom, and J Grassi. (1997). Acetylcholinesterases from Elapidae snake venoms: Biochemical, immunological and enzymatic characterization. Biochim Biophys Acta 1339:253–267.
- Créminon Cousin X, J Grassi, K Méflah, G Cornu, B Saliou, S Bon, J Massoulié, and C Bon. (1996). Acetylcholinesterase from Bungarus venom: A monomeric species. FEBS Lett 387:196–200.
- CK Barcellos, MRC Schetinger, RD Dias, and JJF Sarkis. (1998). In vitro effect of central nervous system active drugs on the ATPase—ADPase activity and acetylcholinesterase activity from cerebral cortex of adult rats. Gen Pharmacol 31:563–567.
- TC Müller, JBT Rocha, VM Morsch, RT Neis, and MRC Schetinger. (2002). Antidepressants inhibit human acetylcholinesterase and butyrylcholinesterase activity. Biochim Biophys Acta 1587:92–98.
- N Nunes-Tavares, AN Matta, CMB Silva, GMN Araújo, SRW Louro, and A Hassón-Voloch. (2002). Inhibition of acetylcholinesterase from Electrophorus electricus by tricyclic antidepressants. Int J Biochem Cell Biol 34:1071–1079.
- M Bourin, P Chue, and C Guillon. (2001). Paroxetine. a review. CNS Drug Rev 7:25–47.
- GL Ellman, KD Courtney, JrV Andres, and RM Featherstone. (1961). A new and rapid colorimetric determination of acetylcholinesterase activity. Biochem Pharmacol 7:88–95.
- JBT Rocha, T Emanuelli, and ME Pereira. (1993). Effects of early under nutrition on kinetic parameters of brain acetylcholinesterase from adult rats. Acta Neurobiol Exp 53:431–437.
- MM Bradford. (1976). A rapid and sensitive method for the quantification of microgram quantities of protein utilizing the principle of protein dye binding. Anal Biochem 72:248–254.
- H Lineweaver, and D Burk. (1934). The determination of enzyme dissociation constants. J Am Chem Soc 56:658–666.
- BH Hofstee. (1952). On the evaluation of the constant Vm and constant Km enzyme reactions. Science 116:329–331.
- JE Dowd, and DS Riggs. (1965). A comparison of estimates of Michaelis menten kinetic constants from various linear transformations. J Biol Chem 240:863–869.
- M Dixon, EC Webb. Enzyme Kinetics. 2nd ed. London UK: Longman; (1964). 54.
- M Ahmed, JBT Rocha, CM Mazzanti, AL Morsch, D Cargnelutti, M Corrêa, V Loro, VM Morsch, and MRC Schetinger. (2007). Malathion, carbofuran and paraquat inhibit Bungarus sindanus (krait) venom acetylcholinesterase and human serum butyrylcholinesterase in vitro. Ecotoxicology 16 (4):363–369.
- M Ahmed, JBT Rocham, M Corrêa, CM Mazzanti, RF Zanin, AL Morsch, VM Morsch, and MRC Schetinger. (2006). Inhibition of two different cholinesterases by tacrine. Chem Biol Interact 162:165–171.
- DK Lahiri, JT Rogers, K Sambamurti, and NH Greig. (2004). Rationale for the development of cholinesterase inhibitors as anti-Alzheimer agents. Curr Pharm Des 10:3111–3119.
- JT O'Brien, and CG Ballard. (2001). Drugs for Alzheimer's disease. Br Med J 323:123–124.
- SH Snyder, and HI Yamamura. (1977). Antidepressants and the muscarinic acetylcholine receptor. Arch Gen Psychiatry 34:236–239.
- TL Rosenberry, JL Johnson, B Cusack, JL Thomas, S Emani, and KS Venkatasubban. (2005). Interaction between the peripheral site and the acylation site in acetylcholinesterase. Chem Biol Interact 157–158:181–189.
- S Marone, I Rozas, and DF Weaver. (1999). Theoretical structuralanalyses of tricyclic neuroactive drugs: Quantum pharmacologic descriptors for clustering anticonvulsant, antidepressant, and antipsychotic activities. J Mol Struct 467:25–30.
- M Harel, I Schalk, L Ehret-Sabatier, F Bouet, M Goeldner, C Hirth, P Axelsen, I Silman, and JL Sussman. (1993). Quaternary ligand binding to aromatic residues in the active-site gorge of acetylcholinesterase. Proc Natl Acad Sci USA 9031:9035.
- J Massoulié, L Pezzementi, S Bom, E Krejci, and EF Vallette. (1993). Molecular and cellular biology of cholinesterases. Prog Neurobiol Rev 41:31–91.