Abstract
Cyanidin-3-galactoside, a natural anthocyanin, was investigated for its α-glucosidase inhibitory activity. The IC50 value of cyanidin-3-galactoside was 0.50 ± 0.05 mM against intestinal sucrase. A low dose of cyanidin-3-galactoside showed a synergistic inhibition on intestinal α-glucosidase (maltase and sucrase) when combined with acarbose. A kinetic analysis showed that cyanidin-3-galactoside gave a mixed type inhibition against intestinal sucrase. The results indicated that cyanidin-3-galactoside was an α-glucosidase inhibitor and could be used in combination with acarbose for treatment of diabetes.
Introduction
Within the last decade, many studies have focused on the potential biological activities or health effects of anthocyanins in animals and humans. Anthocyanins are the largest group of water-soluble pigments in the plant kingdom. They are widely distributed in the human diet through crops, beans, fruits, vegetables, and red wine [Citation1]. Consumption of anthocyanins is associated with a reduced risk of several degenerative diseases such as atheroscelerosis [Citation2], cardiovascular disease [Citation3], cancer [Citation4], and diabetes [Citation5]. Inhibition of α-glucosidase is one of the therapeutic approaches for carbohydrate absorption, by delaying digestion of disaccharides to absorbable monosaccharides. This leads to a reduction in glucose absorption and, subsequently, the rise of postprandial hyperglycemia is attenuated [Citation6]. The agents that reduce postprandial hyperglycemia have a key role in the treatment of Type 2 diabetes and pre-diabetic states. Furthermore, such agents have a potential to reduce the progression of diabetes as well as micro- and macro-vascular complications [Citation7]. Recent studies revealed that α-glucosidase inhibitor improved postprandial hyperglycemia and subsequently reduced the risk of development of type 2 diabetes in patients with impaired glucose tolerance [Citation8].
Cyanidin-3-galactoside () a natural anthocyanin, is widely found in various fruits such as red apple peels, blueberries, cranberries [Citation9]. Yan et al. reported that cyanidin-3-galactoside, isolated from cranberry fruit, showed antioxidant activity as well as vitamin E by evaluating compounds for 1,1-diphenyl-2-picrylhydrazyl radical-scavenging activity and ability to inhibit low-density lipoprotein oxidation in vitro [Citation10]. Recent studies demonstrated that cyanidin-3-galactoside stimulated glucose-induced insulin secretion from rodent pancreatic β-cells [Citation11]. In addition, the natural anthocyanins from a number of plants and fruits are well known as the effective inhibitors of intestinal α-glucosidase activity. To the best our knowledge, intestinal α-glucosidase inhibition of cyanidin-3-galactoside has never been reported and the type of its inhibition is not yet understood. The aim of the study was to evaluate α-glucosidase inhibitory activity of cyanidin-3-galactoside and its kinetic inhibitions. Moreover, the study was also investigated the synergistic inhibition of cyanidin-3-galactoside and acarbose on α-glucosidase.
Materials and methods
Reagents
Rat intestinal acetone powder, glucose oxidase kits were purchased from Sigma Chemical Co.Ltd. (St. Louis, MO). Acarbose was obtained from Bayer, Germany. Cyanidin-3-galactoside chloride was purchased from Chromadex, USA. All others chemicals used were of analytical grade.
α-Glucosidase assay
The assessment of the α-glucosidase inhibitory activity was slightly modified according to reported methods [Citation12]. Briefly, 100 mg of rat intestinal acetone powder (Sigma Chemical, St Louis, MO) was homogenized in 3 mL of 0.9% NaCl solution. After centrifugation (12,000 g × 30 min), 10 μL of the supernatant (maltase = 0.68 units/mg protein, sucrase = 0.10 units/mg protein) was incubated with 70 μL of substrate solution (37 mM maltose, 54 mM sucrose), and 20 μL of cyanidin-3-galactoside at various concentrations in 0.1 M phosphate buffer pH 6.9 at 37°C for 30 min (maltase assay) and 60 min (sucrase assay). The mixtures were suspended in boiling water for 10 min to stop the reaction. The concentrations of glucose released from the reaction mixtures were determined by the glucose oxidase method.
Measurements of the kinetics constant
In order to investigate the type of inhibition, the enzyme kinetic analysis was performed according to the above reaction. Maintaining the quantity of sucrase constant at 0.10 units/mg protein, and cyanidin-3-galactoside (from 0.1 to 1.0 mM) were measured in various concentrations (20, 37, 56, 74, 84 mM) of sucrose. The type of inhibitions was calculated on the basis of Lineweaver–Burk by reciprocally plotted data (substrate concentration on horizontal axis and velocity on vertical axis). For calculation of Ki and Ki′, slope and interception from a Lineweaver–Burk plot were replotted vs. [I] which gave the secondary plots and Ki and Ki′ constants which are the dissociation constants of the inhibitor containing complexes EI and ESI, respectively [Citation13].
Combined effect of cyanidin-3-galactoside and acarbose
The various concentrations of acarbose (0.05 -50 μM) were combined with or without the cyanidin-3-galactoside (1 μM). The reaction was performed according to the above assay. Results were expressed as a percentage inhibition of the corresponding control values.
Data analysis
Data was expressed as mean ± S.E.M. (n = 3). The IC50 values were determined from plots of log concentration of inhibitor concentration versus percentage inhibition curves. The type of inhibition and Ki and Ki′ constants were calculated on the basis of Lineweaver–Burk and replot of its slopes as the non-linear regression using the Sigma Plot 8.0 software (IL, USA). Statistical analysis was performed by Student's t-test (P < 0.01).
Results and discussion
This is the first study to investigate the inhibitory effect of cyanidin-3-galactoside and its type of inhibition on α-glucosidase. We also examined the synergistic inhibition of cyanidin-3-galactoside and acarbose. Firstly, cyanidin-3-galactoside (1 mM) was screened for α-glucosidase inhibition by using maltose and sucrose as a substrate. The results showed that cyanidin-3-galactoside significantly inhibited maltase and sucrase by 35% and 64%, respectively, suggesting that cyanidin-3-galactoside had more potent α-glucosidase inhibitory activity against intestinal sucrase than that of maltase. We then performed dose-response experiments for further investigating the inhibitory effect of cyanidin-3-galactoside on intestinal sucrase inhibition. Cyanidin-3-galactoside markedly inhibited intestinal sucrase in concentration-dependent manner (data not shown). The IC50 value of cyanidin-3-galactoside was 0.50 ± 0.05 mM. However, it was less potent activity than that of acarbose (IC50 = 0.02 ± 0.02 mM). Kay et al. proposed the pathway for the formation of anthocyanin metabolites in human urine and serum post-consumption that intestinal β-galactosidase or lactase-phlorizin hydrolase (LPH) hydrolyzes cyanidin-3-galactoside to cyanidin which could be then passively transported [Citation14]. In contrast, it is clearly that cyanidin-3-galactoside could inhibit α-glucosidase (sucrase). However, the molecular interaction of cyanidin-3-galactoside on specific binding site on intestinal sucrase is also unknown. It can be assumed that cyanidin-3-galactoside contains hydroxyl groups in its molecular structure which can form hydrogen bonds with the polar groups (amide, guanidine, peptide, amino and carboxyl groups) in active site of protein by covalent and/or non-covalent interaction. The occurrence of these interactions would change the enzyme molecular configuration, hydrophilic and hydrophobic properties, resulting in an impact on the enzyme activities. The intestinal sucrase inhibitory effects of cyanidin-3-galactoside derivatives using X-ray crystallography to evaluate the binding activity are needed to further investigation. Additionally, the study revealed that metal ions inhibited α-glucosidase by the decline in the percent of the α-helix structure within the second structure of protein molecule. This phenomenon directly affects to increase the hydrophilic and decrease the hydrophobic properties of α-glucosidase, lead to failure of the formation of active center, resulting that enzyme was inhibited [Citation15]. Based on the data mentioned above, it is possible that the inhibitory effect of cyanidin-3-galactoside on α-glucosidase (sucrase) might demonstrate the same phenomenon on the α-helix region within the second structure of the protein. Further study is needed to characterize the effect of cyanidin-3-galactoside on hydrophilic and hydrophobic properties of intestinal sucrase.
As the findings mentioned above, cyanidin-3-galactoside was moderate effective to be α-glucosidase inhibitor, it was of interest to establish whether this compound and acarbose might interact synergistically or the additively on α-glucosidase. The assay was then performed in the solutions containing acarbose alone or in mixture with a low dose of cyanidin-3-galactoside (1 μM) which had no inhibitory activity. The effect of cyanidin-3-galactoside together with acarbose on intestinal sucrase inhibition is shown in . The addition of cyanidin-3-galactoside to the assay system with low dose of acarbose (3.12 and 6.25 μM), the percentage inhibition was significantly increased when compared with acarbose alone. The percentage inhibition of mixtures was greater than that summing effect of acarbose alone by 12% and 20%, respectively. These findings indicated that the combination of cyanidin-3-galactoside and acarbose produced the synergistic inhibition. As shown in , the addition of cyanidin-3-galactoside to the assay system with low dose of acarbose (0.19 and 0.05 μM) markedly increased the percentage maltase inhibition by 8% and 5%, respectively, when compared with acarbose alone. However, the assay system with high dose of acarbose, cyanidin-3-galactoside did not differ significant in increasing the percentage maltase and sucrase inhibition. As results above, the combining two inhibitors in low dose could produce more effective inhibition of maltose and sucrose absorption, leading to reduction of postprandial hyperglycemia and hyperinsulinemia in type 2 diabetes. In order to investigate this effect, In vivo experiments should be further studied for the combination between cyanidin-3-galactoside and acarbose on lowering blood glucose in type 2 diabetes. In addition, the most common adverse effect of acarbose is a gastrointestinal disturbance, which is induced by producing gas with fermentation of the unabsorbed carbohydrates bowel Citation16–18. Acarbose has rarely been associated with systemic adverse effect, but in some case acute severe hepatotoxicity has been reported Citation19–21. These adverse effects tend to increase with higher doses. Our findings suggested that cyanidin-3-galactoside improves the effect with decreasing dosage of acarbose, resulting to diminish the adverse effect.
Figure 2. The percentage enzyme inhibition of acarbose and its combination with cyanidin-3-galactoside(Cya3Gal) on intestinal α-glucosidase (sucrase). Results were expressed as mean ± S.E.M., n = 3. * P < 0.01 compared to acarbose alone.
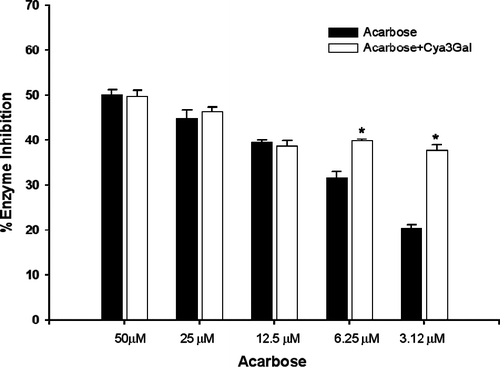
Figure 3. The percentage enzyme inhibition of acarbose and its combination with cyanidin-3-galactoside(Cya3Gal) on intestinal α-glucosidase (maltase). Results were expressed as mean ± S.E.M., n = 3. * P < 0.01 compared to acarbose alone.
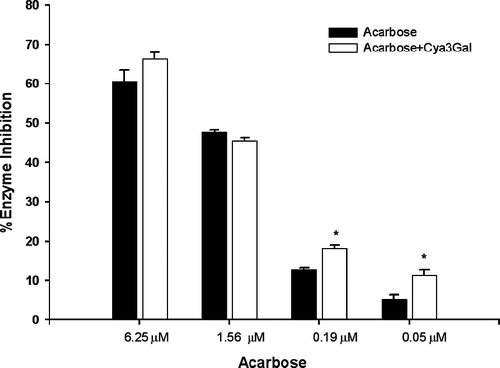
To determine the mechanism of inhibition, cyanidin-3-galactoside was used as an inhibitor in a study to elucidate the types and constants of inhibition. Possible interference by cyanidin-3-galactoside at each substrate-binding site was examined by holding the concentration of cyanidin-3-galactoside at a constant value, and measuring the effect of increasing one substrate concentration on the initial reaction rate. The kinetic parameters were calculated by using Lineweaver–Burk plot and the corresponding secondary plots. Lineweaver–Burk plots for intestinal sucrase inhibited by cyanidin-3-galactoside and replots of slope (s) and vertical axis intercept (i) versus the various concentrations of inhibitor were shown in . Lineweaver–Burk plot of cyanidin-3-galactoside generated straight lines (), which had different intersections for three concentrations of substrate. The lines for cyanidin-3-galactoside had a point of intersection in the second quadrant, indicating that the inhibition was of the mixed competitive and noncompetitive type. When plotting the slope or y-intercept obtained in Lineweaver–Burk processing of data versus the inhibitor concentration, a straight line was produced and the values for Ki and Ki′ dissociation constants could be obtained. From the secondary plot, increasing the inhibitor concentrations resulted in the increasing of slope and the vertical axis intercept. As shown in , The dissociation constant for cyanidin-3-galactoside binding with free enzyme (E), Ki of 0.40 mM, was obtained from the secondary plot of the slopes of these lines versus cyanidin-3-galactoside concentrations, while the dissociation constant for cyanidin-3-galactoside binding with enzyme-substrate complex (ES), Ki′ of 3.23 mM (). The possible binding mode of this compound was assumed that one inhibitor can bind either to active site of free enzyme or to the enzyme–substrate complex. When Ki and Ki′ dissociation constants were compared, it was found that the Ki value of cyanidin-3-galactoside was 8.0 times less than Ki′ value, suggesting that binding of cyanidin-3-galactoside to the ES form was stronger than the binding of cyanidin-3-galactoside to the enzyme alone, indicating that inhibition was competitive predominant over noncompetitive.
Figure 4. (A) The data were presented in a Lineweaver–Burk plot, 1/v against l/[S]. (B) Secondary replot of slope vs. [I] from a primary Lineweaver–Burk plot for the determination of Ki. The value of R2 and slope were 0.990 (C) Secondary replot of intercept vs. [I] from a primary Lineweaver–Burk plot for the determination of Ki′. The value of R2 and slope were 0.991.
![Figure 4. (A) The data were presented in a Lineweaver–Burk plot, 1/v against l/[S]. (B) Secondary replot of slope vs. [I] from a primary Lineweaver–Burk plot for the determination of Ki. The value of R2 and slope were 0.990 (C) Secondary replot of intercept vs. [I] from a primary Lineweaver–Burk plot for the determination of Ki′. The value of R2 and slope were 0.991.](/cms/asset/46fe159b-71c0-44e9-93d4-eb893b59b037/ienz_a_290860_f0004_b.gif)
In conclusion, cyanidin-3-galactoside demonstrated α-glucosidase (sucrase) inhibitory activity. With the combination to acarbose, cyanidin-3-galactoside produced the synergistic effect, indicating that such a combination may apply for clinical therapy and lead to dramatic dose reduction of the acarbose used.
Acknowledgements
This work was fully supported by a Government Research Budget, Thailand.
References
- RL Prior, and X Wu. (2006). Anthocyanins: Structural characteristics that result in unique metabolic patterns and biological activities. Free Radic Res 40:1014–1028.
- YC Chang, KX Huang, AC Huang, YC Ho, and CJ Wang. (2006). Hibiscus anthocyanins-rich extract inhibited LDL oxidation and oxLDL-mediated macrophages apoptosis. Food Chem Toxicol 44:1015–1023.
- I Serraino, L Dugo, P Dugo, L Mondello, E Mazzon, G Dugo, AP Caputi, and S Cuzzocrea. (2003). Protective effects of cyanidin-3-O-glucoside from blackberry extract against peroxynitrite-induced endothelial dysfunction and vascular failure. Life Sci 73:1097–1114.
- PN Chen, SC Chu, HL Chiou, CL Chiang, SF Yang, and YS Hsieh. (2006). Cyanidin 3-glucoside and peonidin 3-glucoside inhibit tumor cell growth and induce apoptosis in vitro and suppress tumor growth in vivo. Nutr Cancer 53:232–243.
- D Ghosh, and T Konishi. (2007). Anthocyanins and anthocyanin-rich extracts: Role in diabetes and eye function. Asia Pac J Clin Nutr 16:200–208.
- GJ McDougall, D Stewart, GJ McDougall, and D Stewart. (2005). Biofactors 23:189–195.
- AD Baron. (1998). Postprandial hyperglycaemia and alpha-glucosidase inhibitors. Diabetes Res Clin Pract 40:S51–S55.
- JL Chiasson, RG Josse, R Gomis, M Hanefeld, A Karasik, and M Laakso. (2002). STOP-NIDDM trial research group. Acarbose for the prevention of Type 2 diabetes, hypertension and cardiovascular disease in subjects with impaired glucose tolerance: Facts and interpretations concerning the critical analysis of the STOP-NIDDM Trial data. Lancet 359:2072–2077.
- R Tsao, R Yang, JC Young, and H Zhu. (2003). Polyphenolic profiles in eight apple cultivars using high-performance liquid chromatography (HPLC). J Agric Food Chem 51:6347–6353.
- X Yan, BT Murphy, GB Hammond, JA Vinson, and CC Neto. (2002). Antioxidant activities and antitumor screening of extracts from cranberry fruit (Vaccinium macrocarpon). J Agric Food Chem 50:5844–5849.
- B Jayaprakasam, SK Vareed, LK Olson, MG Nair, B Jayaprakasam, SK Vareed, LK Olson, and MG Nair. (2005). J Agric Food Chem 53:28–31.
- M Toda, J Kawabata, and T Kasai. (2000). Alpha-glucosidase inhibitors from clove (Syzgium aromaticum). Biosci Biotechnol Biochem 64:294–298.
- L Kandra, A Zajacz, J Remenyik, and G Gyemant. (2005). Kinetic investigation of a new inhibitor for human salivary alpha-amylase. Biochem Biophys Res Commun 334:824–828.
- CD Kay, G Mazza, BJ Holub, and J Wang. (2004). Anthocyanin metabolites in human urine and serum. Br J Nutr 91:933–942.
- Y Wang, L Ma, Z Li, Z Du, Z Liu, J Qin, X Wang, Z Huang, L Gu, and AS Chen. (2004). Synergetic inhibition of metal ions and genistein on alpha-glucosidase. FEBS Lett 576:46–50.
- DE Kelley, P Bidot, Z Freedman, B Haag, D Podlecki, M Rendell, D Schimel, S Weiss, T Taylor, A Krol, and J Magner. (1998). Efficacy and safety of acarbose in insulin-treated patients with type 2 diabetes. Diabetes Care 21:2056–2061.
- H Kentrup, H Bongers, M Spengler, G Kusenbach, and H Skopnik. (1999). Efficacy and safety of acarbose in patients with cystic fibrosis and impaired glucose tolerance. Eur J Pediatr 158:455–459.
- JA Balfour, and D McTavish. (1993). Acarbose. An update of its pharmacology and therapeutic use in diabetes mellitus. Drugs 46:1025–1054.
- R Coniff, and A Krol. (1997). Acarbose: A review of US clinical experience. Clin Ther 19:16–26.
- M Carrascosa, F Pascual, and S Aresti. (1997). Acarbose-induced acute severe hepatotoxicity. Lancet 349:698–699.
- D Mennecier, ES Zafrani, D Dhumeaux, and A Mallat. (1999). Acarbose-induced acute hepatitis. Gastroenterol Clin Biol 23:1398–1399.