Abstract
A novel copper (II) complex of Schiff base prepared through condensation between 2-formyl-17-deoxyestrone and d-glucosamine was synthesized and characterized. Fluorescence spectroscopy was conducted to assess their binding ability with CT-DNA. The results showed that the copper (II) complex could bind to DNA with a weak intercalative mode. The interaction between the copper (II) complex and DNA was also investigated by gel electrophoresis. Interestingly, we found that the complex could cleave plasmid DNA (pUC19) to nicked and linear forms through an oxidative mechanism without the use of exogenous agents.
Introduction
Investigations on the interaction between transition metal complexes and DNA has attracted much interest due to the potential uses of these metal complexes as chemotherapeutic drugs and tools in molecular biology [Citation1–5]. Many kinds of metal complexes were synthesized in order to study their abilities of recognition and cleavage toward DNA [Citation6–13]. Among them, Schiff base metal complex is a kind of attractive reagents due to their special activities in pharmacology and physiology. People paid a great interest in the synthesis, structures and DNA cleavage abilities of Schiff base metal complexes in recent years [Citation14–21]. However, in most cases, the cleavage reactions of DNA catalyzed by Schiff base metal complexes must be initiated by exogenous agents such as H2O2, ascorbic acid, mercaptopropionic acid or light, which limited their in vitro applications. Therefore, self-activating systems that require no further activation to induce the cleavage of DNA were desirable. Recently, it has been reported that the Schiff base metal complexes containing hydroxyl-rich ligands were extremely efficient catalysts in promoting the cleavage of plasmid DNA in the absence of oxygen or additional reductives [Citation22–24].
d-Glucosamine is a natural product with polyhydroxyl and amino groups. It could kill tumor cells with high selectivity [Citation25]. To our best knowledge, the clinical and endocrinological applications of estrogenic steroids and its derivatives have evoked widespread interest recently [Citation26,Citation27]. In this paper, we designed and synthesized a novel copper (II) complex with Schiff base derived from estrone and d-glucosamine. The complex was then used as artificial nuclease to bind DNA and catalyzed the cleavage of DNA. The preliminary mechanism of the catalyzed cleavage process was also discussed.
Materials and methods
17-Deoxyestrone (2) and 3-O-methoxy-17-deoxyestrone (3) were prepared according to the literature [Citation28]. All other reagents were purchased from commercial sources and used without further purification. Melting points were determined using a micro-melting point apparatus without any corrections. Elemental analyses were performed using a Carlo-Elba1106 elemental analytical instrument. IR spectra were recorded on a Shimadzu FTIR-4200 spectrometer as KBr pellets in the range of 4000–400 cm− 1. HRMS spectral data were recorded on Bruker Daltonics Bio TOF. 1H NMR spectral data were measured on a Varian INOVA-400 spectrometer and chemical shifts in ppm are reported relative to internal Me4Si. Fluorescence spectra were recorded on a LS55 spectrofluorometer. Electrophoresis grade agarose and plasmid DNA (pUC19) were purchased from Takara Biotechnology Company.
Preparation of 2-formyl-3-O-methoxy-17-deoxyestrone (4)
Phosphorus oxychloride (5.55 mL, 58.7 mmol) was added to N, N-dimethylformamide (5.5 mL) at 0°C. After stirring for 0.5 h, 3-O-methoxy-17-deoxyestrone (3) (1.50 g, 5. 55 mol) was added and the resulting mixture was heated carefully under 80°C for 8 h. The mixture was then cooled to room temperature, poured into crushed ice, and stirred overnight. The resulting precipitate was collected via vacuum filtration. The crude product was purified by column chromatography (petroleum ether/ diethyl ether, 15:1) to provide 1.25 g of the title product (4) as a pale yellow solid. Yield 73%; m.p: 175–176°C. IR (KBr, cm− 1): 2923, 2864, 1671, 1566, 1496, 1269. 1H NMR (CDCl3, δ, ppm): 0.74 (s, 3H), 1.10–1.93 (m, 13H), 2.16–2.28 (m, 2H), 2.76–2.88 (m, 2H), 3.84 (s, 3H), 6.67 (s, 1H), 7.76 (s, 1H), 10.39 (s, 1H). Anal. Calcd. for C20H26O2: C, 80.50; H, 8.78. Found: C, 80.62; H, 8.56%. HRMS of C20H26O2: 299.2011[M+H]+, found: 299.1857[M+H]+.
Preparation of 2-formyl-17-deoxyestrone (5)
At − 78°C, to a solution of 2-formyl-3-O-methoxy-17-deoxyestrone (4) (1.20 g, 4.03 mmol) in dry CH2Cl2 (50 mL) was slowly added a solution of BBr3 (0.25 mL) in dry CH2Cl2 (15 mL). The cooling bath was removed after 1.5 h and the reaction mixture was stirred under room temperature for 12 h. The mixture was quenched with ice water (10 mL). The aqueous phase was extracted with CH2Cl2 (3 × 30 mL). The combined organic phases were subsequently washed with brine and dried. The solvent was removed under reduced pressure, and the residue was purified by column chromatography (petroleum ether/ diethyl ether, 15:1) to provide 0.98 g of the title product (5) as a white solid. Yield 88%; m.p: 104–105 °C. IR (KBr, cm− 1): 3428, 2927, 2857, 1648, 1566, 1487, 1238. 1H NMR (CDCl3, δ, ppm): 0.70 (s, 3H), 1.10–1.93 (m, 13H), 2.16–2.28 (m, 2H), 2.76–2.87 (m, 2H), 6.70 (s, 1H), 7.43 (s, 1H), 9.81 (s, 1H), 10.78 (s, 1H). Anal. Calcd. for C19H24O2: C, 80.24; H, 8.51. Found: C, 80.42; H, 8.42%. HRMS of C19H24O2: 285.1855[M+H]+, found: 285.1315[M+H]+.
Preparation of Schiff base compound (6)
To a solution of d-glucosamine (0.200 g, 0.56 mmol) in methanol (5 mL) was added a solution of 2-formyl-17-deoxyestrone (5) (0.150 g, 0.87 mmol) in methanol (5 mL) and sodium bicarbonate (0.07 g, 0.80 mmol) under stirring. The solution was stirred at room temperature for 24 h and then concentrated in vacuo. The resulting precipitate was filtered off, washed with cold methanol, and dried. Recrystallization from ethanol provided 0.16 g of the title product (6) as a yellow solid. Yield 65%; m.p: 240–241°C. IR (KBr, cm− 1): 3425, 2923, 2852, 1633, 1573, 1083. 1H NMR(DMSO-d6, δ, ppm): 0.75 (s, 3H), 1.28–1.81 (m, 13H), 2.11–2.28 (m, 2H), 2.48 (s, 4H), 2.76–2.88 (m, 2H), 3.13–3.35 (m, 4H), 3.68–3.71 (d, 2H), 4.65–4.67 (d, 1H), 6.54 (s, 1H), 7.33 (s, 1H), 8.31 (s, 1H). Anal. Calcd. for C25H35NO6: C, 67.39; H, 7.92; N, 3.14. Found: C, 67.72; H, 7.53; N, 3.25%. HRMS of C25H35NO6: 446.2543[M+H]+, found: 446.2542[M+H]+.
Preparation of Schiff base copper (II) complex (7)
The Schiff base (0.096 g, 0.27 mmol) was dissolved in methanol (30 mL) at room temperature and cupric acetate (0.018 g, 0.13 mmol) was then added with stirring. The solution was stirred at room temperature overnight and then concentrated in vacuum. The resulting precipitate was filtered off, washed with methanol. Recrystallization from methanol provided 0.10 g of the title complex (7) as a green solid. Yield 71%; m.p: 220°C (decomposition). IR (KBr, cm− 1): 3412, 2926, 2865, 1623, 1573, 1042. Anal. Calcd. for C25H35CuNO7: C, 57.18; H, 6.72; N, 2.67. Found: C, 57.55; H, 6.43; N, 2.54%. HRMS of C25H35CuNO7: 525.1788[M+H]+, found: 525.2063[M+H]+
Fluorescence quenching experiments
Fluorescence quenching experiments were conducted by adding solution of Schiff base copper(II) complex (7) to the samples containing 32 μg/mL EB, 4 μg/mL DNA at different concentrations (0–15.4 μM). All the samples were excited at 497 nm. Ethidium bromide displacement assay was recorded on a Hitachi FL4500 spectrofluorimeter. Excitation of the sample was carried out on 3 mL quartz cuvette with 497 nm excitation light and emission was measured at 620 nm. The buffer designated 0.01 M Tris–HCl (pH 7.4). Ethidium bromide was dissolved in the buffer in the concentration of 32 μg/mL. 10 μL highly polymerized calf thymus type I DNA (1 mg/mL of nucleotide concentration in the above buffer) was added to provide a concentration of 4 μg/mL and increasing the fluorescence to measurement maxima. The test agent in aqueous solution (depending on the compound) was added in microlitre portions to reduce the fluorescence of DNA-ethidium complex.
Plasmid DNA cleavage experiments
Plasmid DNA (pUC 19) cleavage activity of Schiff base copper (II) complex (7) was monitored by using agarose gel electrophoresis. In a typical experiment, supercoiled DNA (pUC 19) (25 μg/mL, 5 μL) in Tris–HCl (100 mM, pH 7.4) was treated with different concentrations of Schiff base copper(II) complex, followed by dilution with the Tris–HCl buffer to a total volume of 17.5 μL. The samples were then incubated at 37°C for different times, and loaded on a 0.7% agarose gel containing 1.0 μg/mL ethidium bromide. Electrophoresis was carried out at 40 V for 30 min in TAE buffer and run in duplicate. Bands were visualized by UV light and photographed followed by the estimation of the intensity of the DNA bands using a Gel Documentation System.
Tbars-method
The reaction mixture, in a total volume of 2.0 mL, contained the following reagents at the final concentration stated: complex 7 (0.5 mM); Tris–HCl buffer (0.01 mM, pH 7.4) and CT-DNA (40 μg/ mL). The reaction mixture was incubated at 40 °C for 34 h. TBA (0.2 mL of 6% (w/v)) was then added. The whole was heated at 100°C, cooled, and the absorbance of 532 nm was determined.
Results and discussion
Preparation of the Schiff base copper (II) complex
The synthetic route for the novel Schiff base copper (II) complex (7) is shown in Scheme . Subsequent selective formylation of 3-O-methoxy-17-deoxyestrone (3) via a Vilsmeir procedure could give 2-formyl-3-O-methoxy-17-deoxyestrone (4) 4 was then deprotected by boron tribromide in − 78°C to give 2-formyl-17-deoxyestrone (5) Final condensation between 5 and d-glucosamine readily afforded the Schiff base ligand (6) as a yellow solid. The ligand was allowed to react with cupric (II) acetate hydrate in methanol overnight to give Schiff base copper (II) complex (7) as a green solid. All the structures of new compounds were confirmed by IR, HNMR, elemental analyses and HRMS.
DNA binding and cleavage studies
Fluorescence spectroscopy
The binding ability of the Schiff base copper (II) complex (7) to CT (calf thymus) DNA was studied by fluorescence spectroscopy with the use of ethidium bromide (EB). EB has weak fluorescence, but its emission intensity in the presence of DNA could be greatly enhanced because of its strong intercalation between the adjacent DNA base pairs. It was previously reported that this enhanced fluorescence could be quenched, at least partly by the addition of a second molecule [Citation29,Citation30]. The emission spectra of EB bound to DNA in the absence and the presence of the complex were given in . The addition of the complex to DNA pretreated with EB caused appreciable decrease in the emission intensity, which indicated that the DNA-bound EB fluorophore is partially replaced by the complex.
Figure 1. Fluorescence spectra of EB bound to CT-DNA in the absence and presence of the Schiff base copper (II) complex (7.). [EB] = 32 μg/mL, [DNA] = 4 μg/mL, [complex 7] = 0, 1.4, 2.8, 4.2, 5.6, 7.0, 8.4, 9.8, 11.2, 12.6, 14.0, 15.4 μM, respectively; λex = 497 nm. The arrow shows the intensity changes on increasing the complex concentration.
![Figure 1. Fluorescence spectra of EB bound to CT-DNA in the absence and presence of the Schiff base copper (II) complex (7.). [EB] = 32 μg/mL, [DNA] = 4 μg/mL, [complex 7] = 0, 1.4, 2.8, 4.2, 5.6, 7.0, 8.4, 9.8, 11.2, 12.6, 14.0, 15.4 μM, respectively; λex = 497 nm. The arrow shows the intensity changes on increasing the complex concentration.](/cms/asset/a3fe9e3c-2905-4d1b-8b2d-6a4cae1c500e/ienz_a_291722_f0002_b.gif)
According to the classical Stern–Volmer equation [Citation31]:
Where F0 and F are the fluorescence intensities in the absence and presence of the Schiff base copper (II) complex (7), respectively, K is a linear Stern–Volmer quenching constant, [Q] is the concentration of complex. The fluorescence quenching curve of EB bound to DNA by the complex were shown in . The K value for the complex is estimated as 2.4 × 104 M− 1, which suggested that the binding of the Schiff base copper (II) complex (7) with DNA was a weak intercalative mode.
Cleavage of plasmid DNA
Besides the above methods, interaction between the copper (II) complex (7) and DNA was also investigated by the cleavage assay of plasmid DNA (pUC 19). The cleavage of the plasmid DNA was analyzed by monitoring the conversion of supercoiled circular DNA (form I) to nicked DNA (Form II) and linear DNA (Form III). The amounts of strand scission were assessed by agarose gel electrophoresis. The cleavage of DNA by different concentrations of complex was initially studied for 48 hours. The amount of nicked DNA (Form II) observed in agarose gel electrophoresis diagram increased in accord with the change trend of the concentration of complex in the reaction system (). Increasing the concentration of the complex in the order of 0.1, 0.3 and 0.5 mM resulted in 46%, 64%, and 75% of nicked DNA, respectively. Further increase of the concentration of the complex led to the appearance of linear DNA (5% of Form III, , Lane 5).
Figure 2. Effect of concentration of the Schiff base copper (II) complex (7.) on the cleavage of pUC19 DNA (7.0 μg/mL) in a Tris–HCl buffer (100 mM, pH 7.4) containing DMF (8%) and at 37°C for 48 h. (A) Agarose gel electrophoresis diagram: Lane 1, DNA control; Lanes 2–5, [complex 7] = 0.1, 0.3, 0.5 and 0.7 mM. (B) Quantitation of % plasmid relaxation (Form II% and Form III %) relative to plasmid DNA per lane.
![Figure 2. Effect of concentration of the Schiff base copper (II) complex (7.) on the cleavage of pUC19 DNA (7.0 μg/mL) in a Tris–HCl buffer (100 mM, pH 7.4) containing DMF (8%) and at 37°C for 48 h. (A) Agarose gel electrophoresis diagram: Lane 1, DNA control; Lanes 2–5, [complex 7] = 0.1, 0.3, 0.5 and 0.7 mM. (B) Quantitation of % plasmid relaxation (Form II% and Form III %) relative to plasmid DNA per lane.](/cms/asset/1c7045ef-4ecf-45a4-b075-56070ea49c29/ienz_a_291722_f0003_b.gif)
illustrates the influence of reaction time on the cleavage of the plasmid DNA. An increase in the intensity of Form II was observed associated with the increase of reaction time. Amount of nicked DNA (Form II) was observed when the reaction time was 2, 4, 6, 8, 12 and 24 hours respectively. Supercoiled circular DNA (form I) almost disappeared completely after 48 h, and 96% nicked DNA and 3% linear DNA (Form III) were found (, Lane 7).
Figure 3. Effect of reaction time on the cleavage of pUC19 DNA (7.0 μg/mL) with the Schiff base copper (II) complex (7.) (0.7 mM) in a Tris–HCl buffer (100 mM, pH 7.4) containing DMF (8%) at 37°C. (A) Agarose gel electrophoresis diagrams: Lane 1, DNA control, 48 h; Lanes 2–7, 2 h, 4 h, 6 h, 8 h, 24 h, 48 h; (B) Quantitation of % plasmid relaxation (Form II and Form III %) relative to plasmid DNA per lane.
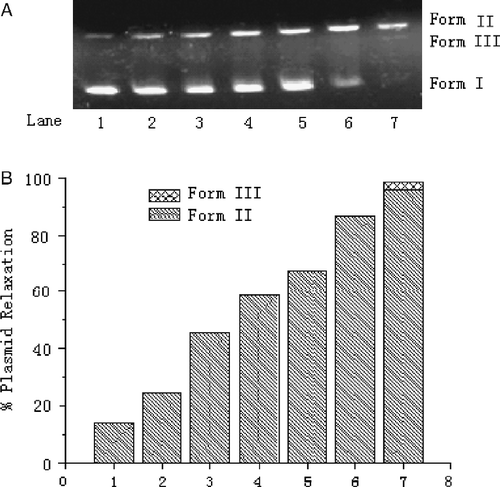
The preliminary mechanism of the DNA cleavage by the Schiff base copper (II) complex (7) was studied by using a series of scavengers that could inhibit the reactive oxygen species. Plasmid pUC19 DNA was incubated with complex in the presence of sodium azide, DMSO and tert-butylalchol respectively, and the results were shown in . Among these scavengers, t-butyl alcohol and NaN3 presented observable inhibition on the DNA cleavage reaction. However, DMSO did not show any inhibit ability against DNA cleavage. Therefore, DNA cleavage promoted by the complex might occur through an oxidative mechanism instead of a hydrolytic mechanism. In order to clarify the cleavage mechanism, we used TBARS-method [Citation32] to detect hydroxyl radical, and an absorbance of 532 nm was observed obviously. This result indicated that hydroxyl radical and singlet oxygen was involved in DNA cleavage process.
Figure 4. Effect of radical scavengers on the cleavage reaction of pUC 19 DNA (7.0 μg/mL) with the Schiff base copper (II) complex (7.) (0.7 mM) in Tris–HCl buffer (100 mM, pH 7.4) containing DMF(8%) at 37°C for 40 h. Agarose gel electrophoresis diagram: Lane 1, DNA control; Lane 2, DNA+complex 7; Lane 3, DNA+complex 7+DMSO (2.5 mM); Lane 4, DNA+complex 7+t-butyl alcohol (2.5 mM); Lane 5, DNA+complex 7+NaN3.
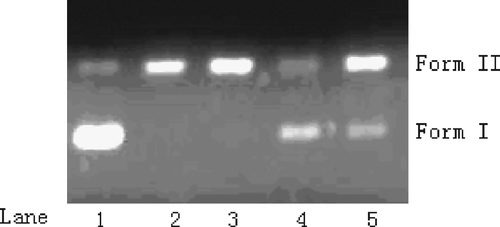
In conclusions, this work described the synthesis, characterization, DNA binding and cleavage abilities of a new copper (II) complex (7) using Schiff base, which was derived from estrone and d-glucosamine, as ligand. The results showed that the Schiff base copper (II) complex with hydroxyl-rich ligand is capable of binding DNA by an intercalative mode, and it could also cleave DNA efficiently without the use of any exogenous agents. The DNA cleavage promoted by the Schiff base copper (II) complex might occur through an oxidative mechanism.
Acknowledgements
This work was financially supported by the National Science Foundation of China (Nos. 20725206, 20732004 and 20572075), Program for New Century Excellent Talents in University, Specialized Research Fund for the Doctoral Program of Higher Education and Scientific Fund of Sichuan Province for Outstanding Young Scientist.
References
- DB Hall, RE Holmlin, and JK Barton. (1996). Oxidative DNA damage through long-range electron transfer. Nature 382:731–735.
- KE Erkkila, DT Odom, and JK Barton. (1999). Recognition and reaction of metallointercalators with DNA. Chem Rev 99:2777–2796.
- B Lippert. (2000). Multiplicity of metal ion binding patterns to nucleobases. Coord Chem Rev 200:487–516.
- A Sreedhara, and JA Cowan. (2001). Catalytic hydrolysis of DNA by metal ions and complexes. J Biol Inorg Chem 6:337–347.
- C Liu, M Wang, T Zhang, and H Sun. (2004). DNA hydrolysis promoted by di-and multi-nuclear metal complexes. Coord Chem Rev 248:147–156.
- DS Sigman, TW Bruice, A Mazumder, and CL Sutton. (1992). Targeted chemical nucleases. Acc Chem Res 26:98–104.
- JC Babrowaik, B Ward, and G Goodisman. (1989). Quantitative footprinting analysis using a DNA-cleaving metalloporphyrin complex. Biochemistry 28:3314–3322.
- CJ Burrows, and SE Rokita. (1994). Recognition of guanine structure in nucleic acids by nickel complexes. Acc Chem Res 27:295–301.
- G Pratvicl, J Bernadou, and B Mcunicr. (1998). DNA and RNA cleavage by metal complexes. Adv Inorg Chem 45:251–312.
- M Shionoya, T Ikeda, E Kimura, and M Shiro. (1994). Novel “multipoint” molecular recognition of nucleobases by a new Zinc(II) complex of acridine-pendant cyclen (cyclen = 1,4,7,10-Tetraazacyclododecane). J Am Chem Soc 116:3848–3859.
- DM Epstein, LL Chappell, H Khalili, RM Supkowski, WD Horrocks, and JR Morrow. (2000). Eu(III) macrocyclic complexes promote cleavage of and bind to models for the 5′-cap of mRNA. Effect of pendent group and a second metal ion. Inorg Chem 39:2130–2134.
- XY Wang, J Zhang, K Li, N Jiang, SY Chen, HH Lin, Y Huang, LJ Ma, and XQ Yu. (2006). Synthesis and DNA cleavage activities of mononuclear macrocyclic polyamine zinc(II), copper(II), cobalt(II) complexes which linked with uracil. Bioorg Med Chem 14:6745–6751.
- YG Fang, J Zhang, SY Cheng, N Jiang, HH Lin, Y Zhang, and XQ Yu. (2007). Chiral multinuclear macrocyclic polyamine complexes: Synthesis, characterization and their interaction with plasmid DNA. Bioorg Med Chem 15:696–701.
- AS Sitlani, EC Long, AM Pyle, and JK Barton. (1992). DNA photocleavage by phenanthrenequinone diimine complexes of rhodium(III): Shape-selective recognition and reaction. J Am Chem Soc 114:2303–2312.
- DC Crans, AD Keramidas, HH Lity, OP Anderson, MM Miller, LM Lemoine, SP Williams, M Vandenberg, AJ Rossomando, and LJ Sweet. (1997). Synthesis, structure, and biological activity of a new insulinomimetic peroxovanadium compound: Bisperoxovanadium imidazole monoanion. J Am Chem Soc 119:5447–5448.
- DJ Gravert, and JH Griffin. (1996). Steric and electronic effects, enantiospecificity and reactive orientation in DNA binding/cleaving by substituted derivatives of [MnIIIsalen]+. Inorg Chem 35:4837–4847.
- VWW Yam, SWK Choi, KKW Lo, WF Dung, and RVC Kong. (1994). Photolytic cleavage of DNA by [Au3(dmmp)2]3+. J Chem Soc Chem Commun 2379–2380.
- LZ Li, C Zhao, T Xu, HW Ji, YH Yu, GQ Guo, and H Chao. (2005). Synthesis, crystal structure and nuclease activity of a Schiff base copper(II) complex. J Inorg Biochem 99:1076–1082.
- K Dhara, J Ratha, M Manassero, XY Wang, S Gao, and P Banerjee. (2007). Synthesis, crystal structure, magnetic property and oxidative DNA cleavage activity of an octanuclear copper(II) complex showing water–perchlorate helical network. J Inorg Biochem 101:95–103.
- S Routier, JL Bernier, JP Catteau, P Colson, C Houssier, C Rivalle, E Bisagni, and C Bailly. (1997). Synthesis, DNA binding, and cleaving properties of an ellipticine–salen copper conjugate. Bioconjugate Chem 8:789–792.
- S Dhar, M Nethaji, and AR Chakravarty. (2006). DNA cleavage on photoexposure at the d–d band in ternary copper(II) complexes using red-light laser. Inorg Chem 45:11043–11050.
- E Lamour, S Routier, JL Bernier, JP Catteau, C Bailly, and H Vezin. (1999). Oxidation of CuII to CuIII, free radical production, and DNA cleavage by hydroxy-salen-copper complexes. Isomeric effects studied by ESR and electrochemistry. J Am Chem Soc 121:1862–1869.
- C Sissi, F Mancin, M Gatos, M Palumbo, P Tecilla, and U Tonellato. (2005). Efficient plasmid DNA cleavage by a mononuclear copper(II) complex. Inorg Chem 44:2310–2317.
- SS Tonde, AS Kumbhar, SB Padhye, and RJ Butcher. (2006). Self-activating nuclease activity of copper (II) complexes of hydroxyl-rich ligands. J Inorg Biochem 100:51–57.
- Y Yamamura, S Kobayashi, and T Fukuda. (1984). Synthesis, and the adjuvant and tumor-suppressive activities of quinonyl muramy dipeptides. Bull Chem Soc Jpn 57:3182–3196.
- VS Pribluda, and SJ Green. (1998). A good estrogen. Science 280:983–987.
- A Ho, Y Kim, H Lee, K Cyrus, SH Baek, and KB Kim. (2006). SAR studies of 2-methoxyestradiol and development of its analogs as probes of anti-tumor mechanisms. Bioorg Med Chem Lett 16:3383–3387.
- P Lupon, F Canals, A Iglesias, and JC Ferrer. (1988). Photochemical behavior of DELTA.4-3-oxo, DELTA.5-7-oxo, and DELTA.1-3-oxo steroids in concentrated acid solution. J Org Chem 53:2193–2198.
- MD Kuwabara, C Yoon, TE Goyne, T Thederahn, and DS Sigman. (1986). Nuclease activity of 1,10-phenanthroline-copper ion: Reaction with CGCGAATTCGCG and its complexes with netropsin and EcoRI. Biochemistry 25:7401–7407.
- TB Thederahn, A Spassky, MD Kuwabara, and DS Sigman. (1990). Chemical nuclease activity of 5-phenyl-1,10-phenanthroline-copper ion detects intermediates in transcription initiation by E. Coli RNA polymerase. Biochem Biophys Res Commun 168:756–762.
- N Matsumoto, JZ Zhang, H Okawa, and S kida. (1989). Crystal structure and magnetic property of the binuclear manganese(III) complex [Mn(L)H2O]2(ClO4)2(L = N-(acetylaceto nylidene)-N′-(α-methylsalicylidene)- ethylenediamine). Inorg Chem Acta 160:153–157.
- B Halliwell, and JMC Gutteridge. (1981). Formation of a thiobarbituric-acid-reactive substance from deoxyribose in the presence of iron salts: The role of superoxide and hydroxyl radicals. FEBS Lett 128:347–352.