Abstract
A methanolic extract of dried leaves of Polygala japonica Houtt (Polygalaceae) significantly attenuated nitric oxide production in lipopolysaccharide-simulated BV2 microglia. Five anthraquinones chrysophanol (1), emodin (2), aloe-emodin (3), emodin 8-O-β-D-glucopyranoside (4) and trihydroxy anthraquinone (5), and four flavonoids kaempferol (6), chrysoeriol (7), kaempferol 3-gentiobioside (8) and isorhamnetin (9) were isolated from the methanolic extract using bioactivity-guided fractionation. Among them, compounds 1–4, 6 and 7 showed significant inhibitory effect on lipopolysaccharide-induced nitric oxide production in BV2 microglia at the concentrations ranging from 1.0 to 100.0 μM.
Introduction
Nitric oxide (NO), precisely excessive NO, has been reported to be involved in a number of neurodegenerative diseases [Citation1]. Thus we tried to find compounds modulating NO production from natural products using lipopolysaccharide (LPS)-stimulated BV2 microglia [Citation2]. In this screening system, the methanolic extract of the leaves of Polygala japonica Houtt (Polygalaceae) significantly inhibited LPS-induced NO production. P. japonica is a perennial herbaceous plant native to some Asian countries. In Korea, the whole plant of P. japonica has been used to treat pharyngitis, insomnia, depression, cuts and bruises [Citation3]. This plant has been known to be rich in triterpenoid saponins [Citation4]. Several flavonoid glycosides, xanthones and cerebrosides have been also reported to be isolated from this plant [Citation5,Citation6].
In the present study, bioassay-guided fractionation of the extract of P. japonica led to the isolation of five anthraquinones (1–5) and four flavonoids (6–9). We describe the isolation and the identification of these compounds and the evaluation of their effect on NO production in LPS-stimulated BV2 cells.
Methods and materials
Plant material
The dried whole plant of P. japonica was purchased from Kyungdong traditional herbal market (Seoul, Korea) and authenticated by Dr. Jong Hee Park, professor of Pusan National University. A voucher specimen (KD-034) has been deposited at the Herbarium of the Medicinal Plant Garden, College of Pharmacy, Seoul National University.
Isolation of NO production inhibitory compounds
The plant material (12.6 kg) was ground and extracted with 80% methanol at room temperature. The methanol extract was concentrated in vacuo to give a crude extract (2.7 kg). The methanolic extract was then suspended in H2O and partitioned successively with n-hexane, CHCl3, ethylacetate (EtOAc) and n-butanol. The n-hexane fraction (263.6 g) was subjected to silica gel column chromatography (CC) to yield 13 fractions (H1 - H13). Compound 1 was isolated from H2 by recrystallization with methanol. Compounds 2 and 3 were isolated from H4 and H9, respectively, by CC over silica gel and Sephadex LH-20. In addition, the EtOAc fraction (87 g) was fractionated into 8 fractions (E1 - E8) by silica gel CC. Compounds 4, 6 and 9 were isolated from E2 by sequentially using silica gel CC and ODS reverse phase CC. E5 was subjected to silica gel CC and Sephadex LH-20 to yield compounds 5 and 7. Compound 8 was isolated from E3 using silica gel CC followed by ODS reverse phase CC.
Cell line cultures
BV2 mouse microglia cell line originally developed by Dr. Bocchini at University of Perugia (Perugia, Italy) [Citation7] was generously provided by Dr. Sun-yeou Kim at Kyunghee University (Suwon, Korea). The cell line was maintained in DMEM containing 10% FBS with penicillin (100 IU/mL) and streptomycin (10 mg/mL) at 37°C in a humidified atmosphere of 95% air-5% CO2.
Evaluation of NO production inhibitory effect in LPS-stimulated BV2 microglia
Test fractions and compounds were dissolved in DMSO (final concentration in cultures < 0.1%). To remove any trace of phenol red, the cell cultures were washed and the medium was replaced with phenol red-free DMEM. Then BV2 microglia cells (2 × 105 cells/well in 96 well plates) were treated with test samples for 1 h before exposure to 100 ng/mL of LPS. After 24 h incubation, nitrite in culture media was measured to assess NO production in BV2 cells using Griess reagent. In 96 well plate, 100 μL of sample aliquots were mixed with 100 μL of Griess reagent (1% sulfanilamide, 0.1% naphtylethylenediamine dihydrochloride, 2% phosphoric acid) and incubated at room temperature for 15 min. The absorbance (abs) at 550 nm was measured on a microplate reader. The concentration was determined using nitrite standard curve [Citation8]. After 100 μL of sample aliquots were collected for Griess assay, MTT (0.2 mg/mL) was directly added to cultures, followed by incubation at 37°C for 3 h. The supernatant was then aspirated and 100 μL of DMSO was added to dissolve the formazan. The absorbance (abs) at 540 nm was measured using a microplate reader. Data were expressed as percent cell viability relative to control cultures [Citation8].
Statistical analysis
All data were expressed as mean ± standard deviation (SD). The evaluation of statistical significance was determined by an “one-way ANOVA” test using computerized statistical package. The data were considered to be statistically significant if the probability had a value of 0.05 or less.
Results and discussion
In last decades, evidence has accumulated suggesting excessive NO production may play a role in neurodegenerative diseases. Increased generation of NO after cerebral ischemia has been reported by measurements of brain nitrite in mice [Citation9]. Moreover, the immunohistochemical and biochemical analysis demonstrated the increment of nitrotyrosine in the brains of Alzehimer's disease or Parkinson's disease patients [Citation10,Citation11]. Taken together, high concentrations of NO and reactive nitric oxide species (RNOS), derivatives of NO, can oxidize, nitrate, nitrosate or nitrosylate at critical residues of biomolecules such as DNA, fatty acids, mitochondrial enzyme and key amino acids in proteins Citation12–14. Thus we tried to find compounds modulating NO production from natural products using LPS-stimulated BV2 microglia. In this screening system, the methanolic extract of P. japonica significantly inhibited LPS-induced NO production. The methanolic extract was then suspended in H2O and partitioned successively with n-hexane, CHCl3, EtOAc and n-butanol. As shown in , all the tested fractions effectively inhibited LPS-induced NO production in BV2 cells. Among these fractions, the EtOAc fraction which showed the most significant NO production inhibitory effect and the n-hexane fraction, the most abundant fraction, were used for the isolation of active compounds to yield five anthraquinones (1–5) such as chrysophanol (1), emodin (2), aloe-emodin (3), emodin 8-O-β-D-glucopyranoside (4) and trihydroxy anthraquinone (5), and four flavonoids including kaempferol (6), chrysoeriol (7), kaempferol 3-gentiobioside (8) and isorhamnetin (9). The chemical structures of these compounds were determined by comparison of their spectroscopic data with those previously reported Citation15–18 (). NO production inhibitory effect of these compounds was evaluated at the concentrations ranging from 1.0 μM to 100.0 μM. As shown in , it was found that compounds 1–4, 6, and 7 have significant inhibitory effects on LPS-induced NO production in BV2 cells. To verify whether reduced cell numbers by the cytotoxicity of these compounds resulted in decreased of NO production, cell viability was measured employing MTT assay. The isolated anthraquinones (1–5) showed slight toxicity at the concentration of 100.0 μM. However, the effect of these compounds, except compound 5 (data not shown), on NO production exceeded their cytotoxicity. Among the isolated flavonoids (6–9), compounds 6 and 7 showed potent inhibitory activity against NO production without cytotoxicity.
Figure 1. Effect of the methanolic extract and the fractions of P. Japonica on LPS-induced NO production in BV2 microglia. BV2 cells were washed with phenol red-free DMEM and incubated with test samples (100 μg/mL) for 1 h. The cultures were then stimulated by 100 n/mL of LPS for 24 h. After incubation, NO production was measured by the Griess reaction and sodium nitrite was used as the standard. NO production (NP) of control and LPS-treated cultures were 3.9 ± 0.1 and 17.7 ± 0.5 μM, respectively. The solid bar shows relative NO production (%) which was calculated as (NP of sample treated – NP of control)/(NP of LPS-treated – NP of control) × 100 (%). LPS-stimulated value differs significantly from control at a level of p < 0.001. Results differ significantly from the LPS-treated, *p < 0.05, **p < 0.01 and ***p < 0.001, respectively. The hatched bar shows the cell viability relative to control cultures.
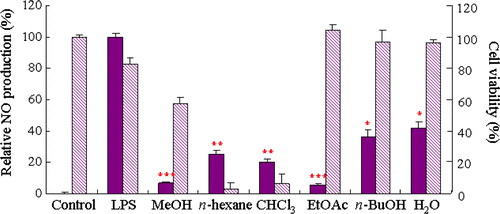
Table I. Effect of the isolated compounds from the leaves of P. Japonica on LPS-induced NO production in BV2 microglia.
Acknowledgements
This research was supported by a grant PF06200-00 from Plant Diversity Research Center of the 21st Century Frontier Research Program funded by the Ministry of Science and Technology, Korea.
Declaration of interest: The authors report no conflicts of interest. The authors alone are responsible for the content and writing of the paper.
References
- JR Heales, JP Bolanos, VS Stewart, PS Brroke, JM Land, and JB Clarak. (1999). Nitric oxide, mitochondrial and neurological disease. Biochim Biophys Acta 1410:215–228.
- SH Kim, SH Sung, SY Choi, YK Chung, J Kim, and YC Kim. (2005). Idesolide: A new spiro compound from Idesia polycarpa. Org Lett 7:3275–3277.
- BS Jung, and MK Shin. (1990). 798. Hyang-Yak-Dae-Sa-Jeon. Young Rim Sa Co., Seoul
- H Wang, J Gao, D Zhu, and D Yu. (2007). Quality evaluation of Polygala japonica through simultaneous determination of six bioactive triterpenoid saponins by HPLC-ELSD. J Pharm Biomed Anal 43:1552–1556.
- TZ Li, WD Zhang, GJ Yang, WY Liu, RH Liu, C Zhang, and HS Chen. (2007). New flavonol glycosides and new xanthone from Polygala japonica. J Asian Nat Prod Res 8:401–409.
- W Zhang, T Li, R Liu, G Yang, and H Chen. (2006). A new cerebroside from Polygala japonica. Fitoterapia 77:336–337.
- E Blasi, R Barluzzi, V Bocchibi, R Mazzolla, and F Bistoni. (1990). Immortalization of murine microglial cells by a v-raf/v-myc carrying retrovirus. J Neuroimmunol 27:229–237.
- SH Kim, YP Jang, SH Sung, and YC Kim. (2007). Inhibitory activity of phenolic glycosides from the fruits of Idesia polycarpa on lipopolysaccharide-induced nitric oxide production in BV2 microglia. Planta Med 73:167–169.
- M Grandati, C Verrecchia, ML Revaud, M Alliz, RG Boulu, and M Plotkine. (1997). Calcium-independent NOS activity and nitrites/nitrates production in transient focal cerebral ischemia in mice. Br J Pharmacol 122:625–630.
- P Fernández-Vizarra, AP Fernández, S Castro-Blanco, JM Encinas, J Serrano, ML Bentura, P Muñoz, R Martínez-Murillo, and J Rodrigo. (2004). Expression of nitric oxide system in clinically evaluated cases of Alzheimer's disease. Neurobiol Dis 15:287–305.
- PF Good, A Hsu, DP Werner, DO Perl, and CW Olanow. (1998). Protein nitration in Parkinson's disease. J Neuropathol Exp Neurol 57:338–342.
- D Wink, and JB Mitchell. (1998). Chemical biology of nitric oxide; insights into regulatory, cytotoxic and cytoprotective mechanisms of nitric oxide. Free Radic Biol Med 25:434–456.
- N Hogg, and B Kalyanarama. (1999). Nitric oxide and lipid peroxidation. Biochim Biophys Acta 1411:378–384.
- MP Murphy. (1999). Nitric oxide and cell death. Biochim Biophys Acta 1411:401–414.
- PK Arawal, MC Bansal, LY Foo, KR Markham, LJ Porter, and RS Thakur. Carbon-13 NMR of flavonoids. Amsterdam: Elsevier, (1989).
- JS Choi, SK Lee, CK Sung, and JH Jung. (1996). Phytochemical study on Aloe vera. Arch Pharm Res 19:163–167.
- M Coskun, T Satake, K Hori, Y Saik, and M Tanker. (1989). Anthraquinone glucosides from Rhamnus libanoticus. Phytochemistiry 29:2018–2020.
- CN Lin, MI Chung, and CM Lu. (1990). Anthraquinone from Rhamnus formosana. Phytochemistry 29:3903–3905.