Abstract
Free radical damage has been associated with a growing number of diseases and conditions, such as autoimmune diseases, neurodegenerative disorders and multiple types of cancer. Some dehydroamino acids and corresponding peptides can function as radical scavengers. In this study the in vitro effects on rat liver lipid peroxidation levels of fourteen N-substituted dehydroamino acid derivatives and α-tocopherol were investigated. α-Tocopherol is a powerful antioxidant that is beneficial in the treatment of many free radical related diseases. The results indicated that all the compounds showed very good inhibitory effect on the lipid peroxidation compound with α-tocopherol at 1 mM concentrations and the inhibition rate was in the range of 70–79 % with the exception of compound 5. At 0.1 mM concentrations compounds 1, 2 and 9 were found more active than α-tocopherol. The results confirmed that molecules such as dehydroamino acids which have reactive double bonds can act as a guard in vitro against oxidants.
Introduction
The implication of oxidative stress in the pathogenesis of many chronic diseases such as cancer, atherosclerosis, and several neurodegenerative disorders has led to the commonly accepted point of view that antioxidants could be beneficial and prevent these diseases [Citation1]. Oxidative stress may occur either due to the over production of reactive oxygen species (ROS) or to decrease of cellular antioxidant levels. Free radicals and ROS generated in tissues and sub-cellular compartments are efficiently scavenged by the antioxidant defense system, which constitutes antioxidant enzymes [Citation2], such as superoxide dismutase, catalase, glutathione peroxidase, and glutathione reductase. Oxidative stress is defined as the imbalance between oxidants and anti-oxidants in favor of the oxidants potentially leading to damage. When there is an excessive production of ROS or impaired antioxidant defense mechanisms, oxidative stress occurs. Hence, any excess ROS must be continuously inactivated in order to maintain normal cell function either by antioxidant enzymes or using synthetic antioxidant supplement.
Dehydroamino acids represent a significant class of compound as they are key intermediates in amino acid and peptide synthesis and are found in variety of naturally occurring antibiotics [Citation3–5]. They also play an important role at the active site of some enzymes and show free radical scavenging activity [Citation6–9]. Dehydroalanine derivatives are useful also for protecting the skin, the mucous membranes and the hair against oxidative stress [Citation10]. Wagner et al [Citation11] showed that dehydroalanyl residue can capture the 5’-deoxyadenosyl radical generated from S-adenosylmethionine by pyruvate formate-lyase-activating enzyme. N-(acylaryl)-dehydroalanine derivatives have shown inhibitory activity towards oxygen radicals [Citation12,Citation13]. Captodative substituted olefins are radical scavengers which react with free radicals to form stabilized radical adducts. One of those compounds, N-(paramethoxyphenylacetyl) dehydroalanine, may react and scavenge both superoxide anion (O2−) and alkoxyl radicals (RO·), and in this way prevent the appearance of their mediated biological effects. The inhibitory effect of this compound [Citation14] implies that O2− is scavenged more efficiently than RO·. N-Acyl dehydroalanines have shown free radical scavenging activity. They react with and scavenge mainly oxygen-derived free radicals such as the O2− and the hydroxyl radical (HO·) and they protect from radiation toxicity and inhibit radiation carcinogenesis in mice [Citation14].
Molecules, which contain Michael acceptor functionalities such as olefins or acetylenes, protect against the toxicity of oxidants and electrophiles indirectly, i.e., by inducing phase 2 cytoprotective enzymes. This may be beneficial to antioxidant functions [Citation15–17]. The presence of Michael acceptor groups at critical positions was found essential not only for anti-inflammatory activity, but also for inhibition of proliferation, promotion of differentiation, and induction of apoptosis in various cell lines in terpenoid (isoprene) type compounds [Citation18–21]. The reactivity of N-substituted dehydroalanines with hydroxyl radical is evident from their inhibition of hydroxyl radical adduct formation. ESR spin trapping studies of the species formed by reaction of the dehydroalanine derivatives with the hydroxyl radical was reported by Sipe et al [Citation22]. Earlier we have shown that N-acetyl dehydroalanine derivatives had strong effect on the Lipid Peroxidation [Citation23]. Therefore, it was of interest to investigate antioxidant ability of N-substituted dehydroamino acid derivatives. This study has surveyed the in vitro effects on rat liver lipid peroxidation levels of fourteen N-substituted dehydroalanine derivatives ( and ). These compounds were previously synthesized by Ferreira et al [Citation24–26] using β-elimination and Michael addition reactions.
Scheme 2. The methyl esters of N-substituted dehydrodipeptide (8, 9), of β-substituted dehydroalanine (10–13) and of N,N-disubstituted dehydroaminobutyric (14).
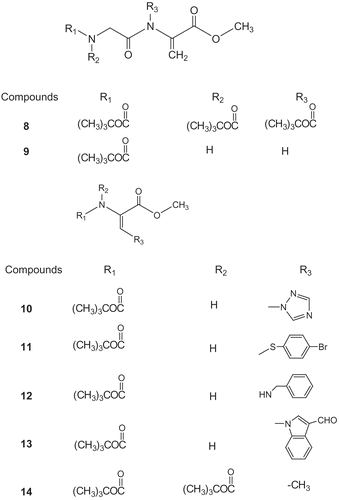
It has been claimed that α-tocopherol is the most important lipid-soluble antioxidant, and it protects cell membranes from oxidation by reacting with lipid radicals produced in the lipid peroxidation chain reaction [Citation27]. This removes the free radical intermediates and prevents the oxidation reaction from continuing. These findings have prompted us to investigate comparative effect of N-substituted dehydroamino acids and α-tocopherol on rat liver lipid peroxidation.
Materials and methods
Chemicals
α-Tocopherol, thiobarbituric acid (TBA), malondialdehyde (MDA), ascorbic acid, FeCl2, H3PO4, butanol were purchased from Sigma Chemical Co. (St Louis, MO, USA).
Synthesis of N-substituted dehydroamino acids
These compounds were previously synthesized and characterized by Ferreira et al [Citation24–26] using β-elimination and Michael addition reactions ( & ).
Assay of lipid peroxidation
The effect of crude extract on rat liver homogenate induced with FeCl2-ascorbic acid and lipid peroxidation (LP) was determined by the method of modified Mihara et al [Citation28]. Wistar rats (200–225 g) were fed with standard laboratory rat chow and tap water ad libitum. The animals were starved for 24 h prior to sacrifice and then killed by decapitation under anesthesia. The study was carried out in accordance with the Guide for the Care and Use of Laboratory Animals. The livers were removed immediately and washed in ice-cold distilled water, and homogenized immediately with Teflon homogenizer in ice. LP was measured spectrophotometrically by estimation of thiobarbituric acid reactant substances (TBARS). Amounts of TBARS were expressed in terms of μmol malondialdehyde (MDA)/g tissue. A typical optimized assay mixture contained 0.5 mL of liver homogenate, 0.1 mL 0.1 M (buffer capacity t= 0.010) of Tris-HCl buffer (pH 7.2), 0.05 mL of 0.1 mM ascorbic acid, 0.05 mL of 4mM FeCl2 and 0.05 mL of various concentration of N-substituted dehydroalanine derivative, or α-tocopherol. This mixture was incubated for 1 h at 37ºC. After incubation, 3.0 mL of H3PO4 and 1 mL of 0.6% TBA were added and shaken vigorously. The mixture was boiled for 30 min. After cooling, butanol was added and the mixture was shaken vigorously. The butanol phase was separated by centrifugation at 3000 rpm for 10 min. The absorbance of the supernatant was read at 532 nm against a blank, which contained all reagents except liver homogenate. α-Tocopherol was used as positive control. Lipid peroxidation inhibitory activity (%) is expressed as follows:
Lipid peroxidation inhibitory activity (%):
Where, Acontrol is the absorbance of the control, Asample is the absorbance of the sample and Ablank is the absorbance of the blank, to which the sample and the free radical generating system (Fe+2/ascorbate) were not added.
Statistical analysis
All data are the average of duplicate analyses. The data were recorded as mean ± standard deviation and analyzed by SPSS (version 11.0 for Windows 98 SPSS Inc.). One-way analysis of variance was performed by ANOVA procedures. Significant differences between means were determined by Duncan’s multiple range tests. Values of p < 0.05 were regarded as significant.
Results
The results indicated that all the compounds studied have a noticeable effect on the inhibition of lipid peroxidation (LP) at the 1mM concentration. They are summarized in . At a concentration of 1mM all the tested compounds inhibited LP between 70–79 %, with the exception of compound 5 (methyl ester of N-tert-butoxycarbonyldehydroalanine) that showed 40 % inhibition at the same concentration. At a concentration of 0.1mM while compounds 1, 2 and 9 showed higher activity than α-tocopherol, compounds 3, 7, 8, 13 and 14 showed moderate inhibition activity between 13–20 % and finally compounds 6, 10 and 11 showed low inhibition activity between 4–9%. It is noticeable that the activities of the tested compounds at 1mM concentration are much higher than the standard α-tocopherol.
Table 1. Anti-Lipid Peroxidation Effects of synthesized compoundsa.
Interesting results were obtained from compounds 4, 5 and 12 that showed pro-oxidant activity at concentration of 0.1 mM. Pro-oxidants are chemicals that induce oxidative stress, either through creating reactive oxygen species or inhibiting antioxidant systems. This result is not surprising because some antioxidant compounds may show pro-oxidant activity such as α-tocopherol which is a classical lipid-soluble antioxidant and well known scavenger of free radicals [Citation29].
On the other hand all the compounds were tested using DPPH radical scavenging activity and superoxide (O2.-) radical scavenging activity assays. No significant results were obtained with these tests. All the compounds had no effect on DPPH radical scavenging activity that was tested by measuring the dehydroalanine derivatives ability to bleach the stable radical 2,2-diphenyl-1-picrylhydrazyl (DPPH) according to the modified method of Blois [Citation30]. The superoxide radical scavenging activity was tested by measuring the capacity of dehydroalanines to scavenge superoxide anion which was determined spectrophotometrically on the basis of inhibition of cytochrome c reduction according to the modified method of McCord et al [Citation31]. Because compounds were inactive for scavenging DPPH and O2.- radical the data is not shown.
Discussion
Dehydroamino acids are essential intermediates in the design of peptides and some antibiotics [Citation32]. Olefins such as dehydroalanines have been shown to inactivate free radicals by forming stabilized free radical adducts. It has been shown that N-substituted dehydroalanines react with and scavenge oxygen radicals. Also they inhibit lipid peroxidation with a different degree of efficacy [Citation7].
Lipid peroxidation can be characterized as the oxidative deterioration of lipids containing any number of carbon-carbon double bonds. The process whereby free radicals take electrons from the lipids of cell membranes, resulting in cell damage and increased production of free radicals. In a peroxide-free lipid system, the peroxidation sequence starts by the attack of a ROS to a hydrogen atom that has a high mobility. This attack generates simply free radicals from polyunsaturated fatty acids. ·OH is the most efficient ROS to do that attack [Citation33,Citation34]. This peroxidation process is inhibited by compounds such as tocopherols, mannitol and formate. The presence of a double bond in the fatty acid weakens the C-H bonds on the carbon atom adjacent to the double bond and so makes H removal easier. The carbon radical tends to be stabilized by a molecular rearrangement to form a conjugated diene. Although we have good information there is still speculation as to the role of the dehydro units in biologically active compounds. Certainly, they have an influence as a conformational constraint due to their sp2 hybridized carbon structure, however, they could well have the ability to add on nucleophiles via Michael type conjugative addition, as recently proven in a set of model experiments [Citation35]. In dehydroalanine type compounds, free radicals are most probably attacked to the carbon-carbon double bond and the resulting radical adduct is formed easily by the effect of the groups next to the dehydro unit. N,N-diprotected dehydroalanine derivatives were found excellent substrates of Michael addition reactions by Ferreira et al [Citation25,Citation26,Citation36,Citation37] but the mono-protected derivatives were found relatively poorer Michael acceptors than the other tested compounds [Citation25,Citation26]. There was no significant correlation found between the antioxidant activity results and the Michael acceptor properties of the compounds. This could be due to more complex chemical structure of the tested compounds than the compounds we worked earlier in our studies [Citation23].
All the compounds were effective only in LP experiment allowing the conclusion that these dehydroalanine derivatives are able to scavenge OH· radical rather than DPPH or superoxide radicals. This result is similar to our earlier findings [Citation23] in which some N-acyl dehydroalanine derivatives showed a strong inhibitory effect on lipid peroxidation at 10−3M and 10−4M concentrations (76–90%), while they had very little or no effect on DPPH radical.
The results established that molecules such as dehydroamino acids which are reactive Michael acceptors can be active in in vitro against the oxidants.
Acknowledgments
Declaration of interest: The authors report no conflicts of interest.
References
- Suzen S. Topics in Heterocyclic Chemistry, Bioactive Heterocycles V, Antioxidant Activities of Synthetic Indole Derivatives and Possible Activity Mechanisms, Khan, M.T.H. (Ed.), 2007, Vol. 11 Page 145–178 Spinger-Verlag Berlin Heidelberg.
- Jayaraj R, Anand T, Lakshmana RPV. Activity and gene expression profile of certain antioxidant enzymes to microcystin-LR induced oxidative stress in mice. Toxicology 2006;220: 136–146.
- Bierbaum G, Gotz F, Peschel A, Kupke T, VandeKamp M, Sahl HG. The biosynthesis of the lantibiotics epidermin, gallidermin, Pep5 and epilancin K7. Antonie Van Leeuwenhoek 1996;69:119–127.
- Dunn AD, Corsi CM, Myers HE, Dunn JT. Tyrosine 130 is an important outer ring donor for thyroxine formation in thyroglobulin. J Biol Chem 1998;273:25223–25229.
- Paik SH, Chakicherla A, Hansen JN. Identification and characterization of the structural and transporter genes for, and the chemical and biological properties of, sublancin 168, a novel lantibiotic produced by Bacillus subtilis 168. J Biol Chem 1998;273:23134–23142.
- Ghoshal AK, Rushmore TH, Buc-Calderon P, Roberfroid M, Farber E. Prevention by free radical scavenger AD5 of prooxidant effects of choline deficiency. Free Radic Biol Med 1990; 8:3–7.
- Buc-Calderon P, Sipe HJ Jr, Flitter W, Mason RP, Roberfroid M. N-acyl dehydroalanines scavenge oxygen radicals and inhibit in vitro free radical mediated processes. Chem Biol Interact 1990;73: 77–88.
- Suzen S. The role of Dehydroalanines in Enzyme and Peptide Chemistry. J Fac Pharm Ankara Univ 1999;28:129–141.
- Stadtman ER, Levine RL. Free radical-mediated oxidation of free amino acids and amino acid residues in proteins. Amino acids 2003;25:207–218.
- Genard S, Galey JB, Hocquaux M. Dehydroalanine derivatives for protecting the skin, the mucous membranes and/or the hair from oxidative stress, cosmetic or dermatological compositions containing them. 1999, United States Patent 5871755.
- Wagner AF, Demand J, Schilling G, Pils T, Knappe J. A dehydroalanyl residue can capture the 5’-deoxyadenosyl radical generated from S-adenosylmethionine by pyruvate formate-lyase-activating enzyme. Biochem Biophys Res Commun 1999;254:306–10.
- Buc-Calderon P, Defrense MP, Barvais C, Roberfroid M. N-acyl dehydroalanines protect from radiation toxicity and inhibit radiation carcinogenesis in mice. Carcinogenesis 1989;10:1641–1644.
- Vo TK, Fischer SM, Slaga TJ. Effects of N-acyl dehydroalanines on phorbol ester-elicited tumor development and other events in mouse skin. Cancer Lett 1991;60:25–32.
- Buc-Calderon P, Roberfroid M. Inhibition of rat liver microsomal lipid peroxidation by N-acyldehydroalanines: an in vitro comparative study. Arch Biochem Biophys 1989;273:339–46.
- Wettasinghe M, Bolling B, Plhak L, Parkin K. Screening for Phase II Enzyme-inducing and Antioxidant Activities of Common Vegetables. J Food Sci 2000;67:2583–2588.
- Being-Sun W, Ming-Chun H, Chun-Ching W, Chia-Wen H. Piceatannol upregulates endothelial heme oxygenase-1 expression via novel protein kinase C and tyrosine kinase pathways. Pharm Res 2006;53:113–122.
- Dinkova-Kostova AT, Cheah J, Samouilov A, Zweier JL, Bozak RE, Hicks RJ, Talalay P. Phenolic Michael reaction acceptors: combined direct and indirect antioxidant defenses against electrophiles and oxidants. Med Chem 2007;3:261–8.
- Ito Y, Pandey P, Sporn MB, Datta R, Kharbanda S, Kufe D. The Novel Triterpenoid CDDO Induces Apoptosis and Differentiation of Human Osteosarcoma Cells by a Caspase-8 Dependent Mechanism. Mol Pharmacol 2001;59:1094–1099.
- Konopleva M, Tsao T, Ruvolo P, Stiouf I, Estrov Z, Leysath CE, Zhao S, Harris D, Chang S, Jackson EC, Munsell M, Suh N, Gribble G, Honda T, Stratford May W, Sporn MB, Andreeff M. Novel triterpenoid CDDO-Me is a potent inducer of apoptosis and differentiation in acute myelogenous leukemia. Blood 2002;99:326–335.
- Pedersen IM, Kitada S, Schimmer A, Kim Y, Zapata JM, Charboneau L, Rassenti L, Andreeff M, Bennet F, Sporn MB, Liotta LD, Kipps TJ, Reed JC. The triterpenoid CDDO induces apoptosis in refractory CLL B cells. Blood 2002;100:2965–2972.
- Dinkova-Kostova AT, Liby KT, Stephenson KK, Holtzclaw WD, Gao X, Suh N, Williams C, Risingsong R, Honda T, Gribble GW, Sporn MB, Talalay P. Extremely potent triterpenoid inducers of the phase 2 response: Correlations of protection against oxidant and inflammatory stress. Proc Natl Acad Sci USA 2005;102:4584–4589.
- Sipe HJ, Buc-Calderon P, Roberfroid M, Mason RP. Identification of the free radical formed by addition of hydroxyl radical to dehydroalanine compounds. Chem Biol Interact 1993;86:93–102.
- Suzen S, Gurkok G, Coban T. Novel N-acyl dehydroalanine derivatives as antioxidants: Studies on rat liver lipid peroxidation levels and DPPH free radical scavenging activity J Enz Inhib Med Chem 2006;21:179–85.
- Ferreira PMT, Maia HLS, Monteiro LS, Sacramento J. High yielding synthesis of dehydroamino acid and dehydropeptide derivatives. Chem Soc Perkin Trans 1 1999; 3697–3703.
- Ferreira PMT, Maia HLS, Monteiro LS, Sacramento J, Sebatião J. Synthesis of beta-substituted alanines via Michael addition of nucleophiles to dehydroalanine derivatives J Chem Soc Perkin Trans. 1 2000;3317–3324.
- Ferreira PMT, Maia HLS, Monteiro LS, Sacramento J. Michael addition of thiols, carbon nucleophiles and amines to dehydroamino acid and dehydropeptide derivatives. J Chem Soc Perkin Trans. 1 2001;3167–3174.
- Traber MG, Atkinson J. Vitamin E, antioxidant and nothing more. Free Radic Biol Med 2007;43:4–15
- Mihara M, Uchiyama M, Fukuzawa K. Thiobarbituric acid value on fresh homogenate of rat as a parameter of lipid peroxidation in aging, CCl4 intoxication, and vitamin E deficiency. Biochem Med 1980;23:303–311.
- Kontush A, Finckh B, Karten B, Kohlschutter A, Beisiegel U. Antioxidant and prooxidant activity of alpha-tocopherol in human plasma and low density lipoprotein. J Lipid Res 1996;37:1436–1448.
- Blois MS. Antioxidant determination by the use of stable free radical. Nature 1958;181:1199–1200.
- McCord J, Fridowich I. An enzymic function for erythrocuprein. J Biol Chem 1969;243:6049–6055.
- Suzen S, Williams JM. Investigation of the Synthesis of some dehydroalanine Derivatives. Turk J Chem 2000;24:361–370.
- Caliskan-Ergun B, Sukuroglu M, Coban T, Banoglu E, Suzen S. Screening and evaluation of antioxidant activity of some pyridazine derivatives. J Enz Inhib Med Chem 2007;23(2):225–229.
- Suzen S, Bozkaya P, Coban T, Nebioglu D. Investigation of the in vitro antioxidant behaviour of some 2-phenylindole derivatives: discussion on possible antioxidant mechanisms and comparison with melatonin. J Enz Inhib Med Chem 2006;21(4):405–411.
- Abreu AS, Silva NO, Ferreira PMT, Queiroz, MJRP. Synthesis of novel amino acids and dehydroamino acids containing benzothiophene moiety. Eur J Org Chem 2003;1537–1545.
- Ferreira PMT, Maia HLS, Monteiro LS, Sacramento J. Synthesis of novel beta-substituted alpha,beta-dehydroamino acid derivatives. Tetrahedron Lett 2000b;41:7437–7441.
- Ferreira PMT, Monteiro LS. Synthesis and reactivity of beta-bromo-beta-substituted Dehydroalanines. Eur J Org Chem 2006;3226–3234.