Abstract
A series of aldose reductase (ALR2) inhibitors based on pyridothiadiazine were prepared and evaluated for their activities in ALR2 inhibition, DPPH scavenging, and MDA inhibition. Comparison studies were carried out between analogs having either hydroxyl or methoxy groups substituted on the N2-benzyl side chains of the compounds. Most of the hydroxy-substituted compounds were found to be more potent compared to their methoxy-substituted analogs with respect to DPPH inhibition (>93%) and MDA inhibition (>73%). However, ALR2 inhibitory activity was found to be affected by the electron-withdrawing substituent at the C7 position in addition to the effect of the N2-substituted benzyl group. These results provide an array of multifunctional ALR2 inhibitors possessing capacities both for ALR2 inhibition and as antioxidants.
Introduction
Diabetes mellitus (DM), a life-threatening disease distributed worldwide, is associated with various complications such as retinopathy, nephropathy, cataract, neuropathy and strokeCitation1,Citation2. Diabetic complications triggered by hyperglycemia have been proposed to involve three major pathways: protein kinase C (PKC) isoform activation, increased glucose flux via the aldose reductase pathway and higher production of glucose-derived advanced glycation end products (AGE). As the starting event of DM development is hyperglycemia, mechanisms such as glucose-stimulated free radical production and hyperglycemia-induced overproduction of mitochondrial superoxide have also been studiedCitation3–5. Under hyperglycemic conditions, the polyol metabolic pathway is activated () and leads to the accumulation of sorbitol. Aldose reductase (E.C.1.1.1.21, ALR2), as the first and rate-determining enzyme, catalyzes the reduction of excess glucose to sorbitol in the presence of co-factor NADPH. In turn, sorbitol dehydrogenase converts sorbitol to fructose through an NAD+-dependent reaction. Sorbitol accumulation causes osmotic stress, which results in tissue damage. Furthermore, the increase in the NADH/NAD+ ratio promotes the activation of PKC isoform that are believed to act as mediators for biochemical and functional alterations induced by hyperglycemia. Moreover, the activity of the NADPH-requiring enzyme glutathione (GSH) reductase decreases with the depletion of NADPH cofactor. The decrease in GSH level is associated with a concomitant rise in toxic lipid peroxidation products like malondialdehyde (MDA) and trans-4-hydroxy-2-nonenal (HNE). Therefore, the inhibition of ALR2 has received considerable attention as a means for the prevention and treatment of diabetic complications. To this purpose, a large number of ALR2 inhibitors (ARIs) have been developed in the past few decades ()Citation6–9. Unfortunately, few of these have advanced to the later stages of clinical trials. At present, epalrestat is only the drug available on the market for treatment of diabetic complications. Therefore, the development of more ARIs suitable for use to prevent DM complications is still needed.
It has been suggested that the discovery of ARIs possessing antioxidant activity is very desirable when designing drug candidates that are effective against diabetic complications, since increased oxidative stress is a widely accepted participant in the development and progression of DM and its complications3,Citation10–13. A phenolic group is commonly involved in many natural and synthetic products exhibiting antioxidant activityCitation14–16. Previously, we have designed a series of ARIs based on the scaffold of pyrido[2,3-e][1,2,4]thiadiazine-1,1-dioxideCitation17,Citation18. The present study is focused on the introduction of phenolic groups into the framework in order to obtain ARIs with antioxidant functionality.
Results and discussion
The key intermediate compounds 1 in the synthesis were obtained by starting from 2-amino-pyridines, and the procedure has been previously reportedCitation17.
The introduction of a benzyl group as a side chain at the N2-position of esters 1a–c was achieved by an alkylation reaction with benzyl bromide as appropriate to achieve the corresponding methyl esters 2a–c, 4a–c and 7, which were then treated with different methods to form the final carboxylic acidsCitation18–20. The procedures are depicted in Schemes 1–3, respectively.
Scheme 2. Reagents and conditions: (i) K2CO3, CH3CN, 70 °C; (ii) NaOHaq., THF, then HClaq. and (iii) K2CO3, thiophenol, NMP, 150 °C.
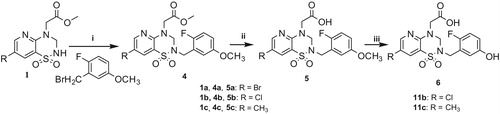
Scheme 3. Reagents and conditions: (i) K2CO3, CH3CN, 70 °C; (ii) AlCl3, CH2Cl2, 40 °C and (iii) NaOHaq., THF, then HCaq.
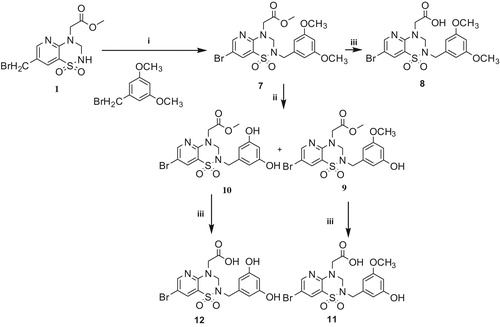
Compounds 2a–c were demethylated to form the final acids 3a–c by treatment with BBr3.
The preparation of compounds 6a–c proceeded from 4a–c, which involved two steps as they were first converted into 5a–c by hydrolysis with aqueous sodium hydroxide (Scheme 2). Compounds 11 and 12 were obtained by demethylation with AlCl3 (Scheme 3).
The synthesized compounds were evaluated for their ALR2 inhibition, scavenging effects on the DPPH free radical, and MDA inhibition. The results are summarized in . Previously reported compoundsCitation17 were also included and named as 13a–c in for comparison.
Table 1. Biological evaluation of pyridothiadiazine derivatives.
ALR2 inhibitory activity
The synthetic work led to a series of compounds containing diversified groups located on the C7-position of the core and the N2-benzyl ring as shown in . All the compounds could be categorized according to substituents at the N2-benzyl ring in two types: the first type included 5a–c and 6b–c, which contained both an electron-donating group and fluoro, an electron-withdrawing group, at the N2-benzyl ring. The second type included those with only the electron-donating group at the N2-benzyl ring, namely compounds 13a–c, 8, 11 and 12. Of all the compounds, 5a–c and 6b–c in the first type showed a more potent inhibitory activity against ALR2 with IC50 values ranging from 1.3 to 2.86 μM. The compounds in the second type bearing only electron-donating substituents at the N2-benzyl ring, namely 13b–c, 3a–c, 8, 11 and 12, displayed less inhibitory activity against ALR2. These results suggest that electron-donating substituents at the N2-benzyl ring retained ALR2 inhibition activity, and the electron-withdrawing substituents at the N2-benzyl ring improved the activity of ARIs based on the pyrido[2,3-e]-[1,2,4]-thiadiazine 1,1-dioxide scaffold. This is consistent with a previous studyCitation17. In addition, in comparing the series 3a–c with the parent compounds 13a–c, an interesting inhibition profile was observed. The C7-halogen, N2-4-hydroxyl derivatives 3a and 3b showed a higher activity than 13a and 13b, respectively. In contrast, the C7- methyl, N2-4-hydroxyl derivatives 3c appeared to be less active than 13c. These findings indicated that the introduction of a phenolic group does not necessarily increase inhibitory activity against ALR2. This is further evidenced by the fact that compound 11 is more active than compound 8 but less active than compound 12, resulting in the rank order 11 >12 >8. In the case of compounds 13b and 3b, the introduction of a hydroxyl group increased the activity, which is likely due to the enhanced binding behavior between 3b and ALR2.
DPPH radical scavenging activity
The free radical scavenging potential of all the compounds were assessed in vitro using a model reaction with the stable free radical 2,2-diphenyl-1-picrylhydrazyl (DPPH). Results are summarized in . While their free radical scavenging activity was less than that of Trolox, the positive control for antioxidant potency, compounds 3a,b, 6b, 8, 11 and 12 showed good to excellent DPPH radical scavenging activity, and compound 3b was the most active achieving 93.2% activity at a concentration of 100 μM, which approached that of Trolox (95%).
Structure-activity relationship studies were based on three comparisons. Among all the compounds in , the compounds containing a phenolic hydroxyl group showed higher free radical scavenging activity compared to their parent analogs, revealing that the phenolic subunit is a structural determinant of DPPH free radical scavenging activity in the present study. This is further confirmed by the effect of the number of the hydroxyl group in compounds 8, 11, 12 on the DPPH radical scavenging activity in which the hydroxyl group-containing products 11, 12 formed from 9, and 10, respectively showed a much greater free radical scavenging activity (21.3 and 49.8%, respectively) compared with their parent compound 8 (11.5%), and compound 12 containing two hydroxyl groups showed more than twice the activity of compound 11 containing one hydroxyl group. Of all the hydroxyl contained compounds, with respect to the effect of a C7-substituent at the core only, the C7-chloro substitution undoubtedly had a more remarkable influence on activity than that of C7-bromo and C7-methyl, when comparing 3b with 3a and 3c, and 6b with 6c.
Inhibition of lipid peroxidation
To further confirm the antioxidant potential of the newly synthesized compounds described above, the inhibitory effect of the compounds on hydroxyl radical dependent lipoperoxidation induced in rat brain homogenate by the oxidant system Fe(III)/ascorbic acid was also examined. The level of MDA is an indicator of lipid peroxidation in cellular membranes. In membranes, relative antioxidant reactivity is probably different from that in a homogeneous system, because of the role played by additional factors such as the subcellular location of the antioxidants and radicals, and to some extent, the differing activities between aqueous and lipophilic compartments. The antioxidation results from the DPPH radical scavenging experiment were perfectly replicated in this assessment demonstrating suppression of lipid peroxidation with the compounds. As shown in , the tested compounds inhibited the production of MDA and the thiobarbituric acid reactive substances (TBARS), and reduced the index of lipid peroxidation, with varying degrees of efficacy. Compounds 3a,b, 10b, 11b, 11 and 12 showed appreciable antioxidant properties, and were more potent than the other tested compounds. In particular, the inhibitory activity of the most active compound 3b was 73.9% with an IC50 value of 124.4 μM, further demonstrating the importance of the hydroxyl substituents in the derivatives for their antioxidant activity. This is consistent with the results that were determined by the DPPH method. Antioxidants are known to act at different levels in the cellular oxidative pathway, showing multiple mechanisms of action. The degree of efficacy in inhibiting the level of MDA indicated the capacity of the compound to scavenge free radicals, or prevent the formation of free radicals, or both.
Molecular docking
To understand the mechanistic details in the inhibition of ALR2 and the importance of the hydroxyl group on the N2-benzyl ring, molecular docking of compounds 13b and 3b, a pair of structural analogs with 3b possessing good activities both in ALR2 inhibition and antioxidant activity, were performed on a human ALR2 complex with NADP+ and IDD594 (PDB code = 1US0). Docking results showed that compounds 13b and 3b bound well to the active site of ALR2 (). The carbonyl group of 13b was inserted deeply into the anion binding site by a hydrogen bond interacting with the carbonyl oxygen atom and the side chains of residues Trp111 (3.12 Å). However, in the case of 3b, in addition to the hydrogen bond between the carboxylic oxygen atom and Tyr48 (3.11 Å), an extra hydrogen bond (2.48 Å) was formed between the oxygen atom of the hydroxyl group on the N-benzyl ring with the residues of Thr113 (). These hydrogen bonding interactions deeply embedded compound 3b in the anion bonding pocket, resulting in higher binding activity compared to 13b.
Figure 3. Molecular docking of compound 13b (A & B) and 3b (C & D). (A & C) Docking of compounds 13b or 3b into the ALR2 active site. The protein structure is shown in ribbon and tube representation with selected residues labeled and shown in line representation; ligand 13b (or 3b) and NADPH are shown as stick models. The docked pose of 13b and 3b are shown in yellow. Hydrogen bonds are shown as green dashed lines. (B & D) Surface representation of protein active site residues in the docking of 13b or 3b into the active site of ALR2.
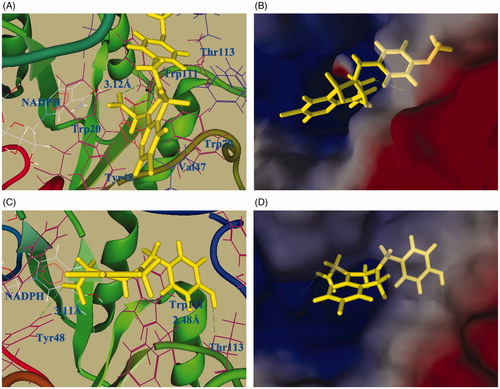
Conclusions
The developed pyridothiadiazine derivatives having the electron withdrawing group at the C7 position and a hydroxyl group on the N2-benzyl ring were active in ALR2 inhibition. The hydroxyl group on the N2-benzyl ring was more effective than a methoxy group for ARI activity. Furthermore, compounds 3a–b and 10 showed appreciable antioxidant properties in the tests both of DPPH scavenging and MDA inhibition. In addition, the increase in the number of the hydroxyl group on the N2-benzyl ring significantly promoted the DPPH scavenging activity and MDA inhibition of compounds.
Declaration of interest
This work was supported by the National Natural Science Foundation of China (Grant No. 21272025), the Research Fund for the Doctoral Program of Higher Education of China (Grant No. 20111101110042) and the Science and Technology Commission of Beijing, China (Grant No. Z131100004013003).
Supplementary material available online
IENZ_1178638_Supplementary_Material.pdf
Download PDF (467.3 KB)References
- Nathan D. Long-term complications of diabetes mellitus. N Engl J Med 1993;328:1676–85
- Alexiou P, Pegklidou K, Chatzopoulou M, et al. Aldose reductase enzyme and its implication to major health problems of the 21(st) century. Curr Med Chem 2009;16:734–52
- Maritim AC, Sanders RA, Watkins JB III. Diabetes, oxidative stress, and antioxidants: a review. J Biochem Mol Toxicol 2003;17:24–38
- Nishikawa T, Edelstein D, Du X, et al. Normalizing mitochondrial superoxide production blocks three pathways of hyperglycaemic damage. Nature 2000;404:787–90
- Yamagishi SI, Edelstein D, Du XI, Brownlee M. Hyperglycemia potentiates collagen-induced platelet activation through mitochondrial superoxide overproduction. Diabetes 2001;50:1491–4
- Kikkawa R, Hatanaka I, Yasuda H, et al. Effect of a new aldose reductase inhibitor, (E)-3-carboxymethyl-5-[(2E)-methyl-3-phenylpropenylidene]rhodanine (ONO-2235) on peripheral nerve disorders in streptozotocin-diabetic rats. Diabetologia 1983;24:290–2
- Ao S, Shingu Y, Kikuchi C, et al. Characterization of a novel aldose reductase inhibitor, FR74366, and its effects on diabetic cataract and neuropathy in the rat. Metab Clin Exp 1991;40:77–87
- Van Zandt MC, Jones ML, Gunn DE, et al. Discovery of 3-[(4,5,7-trifluorobenzothiazol-2-yl)methyl]indole-N-acetic acid (lidorestat) and congeners as highly potent and selective inhibitors of aldose reductase for treatment of chronic diabetic complications. J Med Chem 2005;48:3141–52
- La Motta C, Sartini S, Salerno S, et al. Acetic acid aldose reductase inhibitors bearing a five-membered heterocyclic core with potent topical activity in a visual impairment rat model. J Med Chem 2008;51:3182–93
- Nishikawa T, Araki E. Impact of mitochondrial ROS production in the pathogenesis of diabetes mellitus and its complications. Antioxid Redox Signal 2007;9:343–53
- Reddy VP, Zhu X, Perry G, Smith MA. Oxidative stress in diabetes and Alzheimer's disease. J Alzheimers Dis 2009;16:763–74
- Nwose EU, Jelinek HF, Richards RS, Kerr PG. Erythrocyte oxidative stress in clinical management of diabetes and its cardiovascular complications. Br J Biomed Sci 2007;64:35–43
- Stefek M, Snirc V, Djoubissie P, et al. Carboxymethylated pyridoindole antioxidants as aldose reductase inhibitors: synthesis, activity, partitioning, and molecular modeling. Bioorg Med Chem 2008;16:4908–20
- Li S, Zhang D, Yang L, Li Y. Study on the total synthesis of velbanamine: chemoselective dioxygenation of alkenes with PIFA via a stop-and-flow strategy. Beilstein J Org Chem 2013;9:983–90
- Przybylski P, Huczynski A, Pyta K. Biological properties of schiff bases and azo derivatives of phenols. Curr Org Chem 2009;13:124–48
- Isemura M, Timmermann BN. Plant polyphenols and health benefits. Curr Pharm Design 2013;19:6051–2
- Chen X, Yang Y, Ma B, et al. Design and synthesis of potent and selective aldose reductase inhibitors based on pyridyl-thiadiazine scaffold. Eur J Med Chem 2011;46:1536–44
- Liu Y, Yi-Lin L, Karin M, et al. Thyroid receptor ligands. 1. Agonist ligands selective for the thyroid receptor beta1. J Med Chem 2003;46:1580–8
- Li M, Han X, Yu B. Facile synthesis of flavonoid 7-O-glycosides. J Org Chem 2003;68:6842–5
- Max VD, Christine ML, Dong VM. Rhodium-phosphoramidite catalyzed alkene hydroacylation: mechanism and octaketide natural product synthesis. J Am Chem Soc 2012;134:15022–32