Abstract
Aldose reductase (AR) inhibitors have vital importance in the treatment and prevention of diabetic complications. In this study, rat kidney AR was purified 19.34-fold with a yield of 3.49% and a specific activity of 0.88 U/mg using DE-52 Cellulose anion exchange chromatography, gel filtration chromatography and 2′5′ ADP Sepharose-4B affinity chromatography, respectively. After purification, the in vitro inhibition effects of some phenolic acids (tannic acid, chlorogenic acid, sinapic acid, protocatechuic acid, 4-hydroxybenzoic acid, p-coumaric acid, ferulic acid, vanillic acid, syringic acid, α-resorcylic acid, 3-hydroxybenzoic acid and gallic acid) were investigated on purified enzyme. We determined IC50, Ki values and inhibition types of these phenolic acids. As a result, tannic and chlorogenic acid had a strong inhibition effect. On the other hand, gallic acid had a weak inhibition effect. In this study, all phenolic acids except for chlorogenic acid and p-coumaric acid showed non-competitive inhibition effects on rat kidney AR.
Introduction
Diabetes mellitus is a group of metabolic diseases characterized by high blood sugar resulting from partial or complete lack of insulin secretion. The chronic hyperglycemia of diabetes is associated with long-term diabetic complications such as retinopathy, neuropathy, cataracts, nephropathy and cardiovascular complications. Currently, diabetes is an increasingly important health problem all over the world due to the frequency of diabetes and diabetic complications. According to recent data from the International Diabetes Federation, in 2013, there were approximately 382 million people globally suffering from diabetes and this number is expected to reach 592 million an increase of 55% by the year 2030Citation1. Among all diseases, diabetes-induced death ranks eighth in the worldCitation2. It has been reported that 5.1 million people all over the world died from diabetes and its complications in 2013Citation1. In addition to being a chronic disease that threatens human health, diabetes is quite a costly disease for both individuals and countriesCitation1. To reduce the burden of diabetes on the individual and society, early diagnosis and the appropriate treatment of diabetes and its complications should be effected. Therefore, studies about the prevention of diabetes and its complications are very important.
Various biochemical pathways are activated under hyperglycemic conditions. Among these, the polyol pathway is the most widely studied and the most promising in order to explain the mechanisms of diabetic complicationsCitation3. When there are excessive glucose levels, they are metabolized via the polyol pathway. This pathway involves two main enzymatic steps: the first and key enzyme aldose reductase (AR), which reduces glucose to sorbitol by using NADPH as cofactor, and the second enzyme, sorbitol dehydrogenase (SDH), converts sorbitol to fructose with NAD+ as a cofactorCitation4 ().
In cases of diabetic complications such as retinopathy, neuropathy and nephropathy, the speed of the polyol pathway significantly increases. As a result of this, accumulation of sorbitol and its metabolites occurs in several cells including nerves, retina and kidneys due to the poor penetration across membranes and inefficient metabolism of sorbitol. Thus, both oxidative stress and osmotic stress increase in the cells depending on the development of diabetic complications. High activity of AR causes an increase in the consumption of NADPH and this causes the decrease of the activities of glutathione reductase and nitric oxide synthase. Therefore, high activity of AR causes a reduction of the intracellular GSH and NO levels. As a result, aldose reductase enzyme activity reduces the cellular antioxidant capacityCitation5–7. Meanwhile, the oxidation of sorbitol to fructose by SDH leads to oxidative stress. This is because its co-factor NAD+ is converted to NADH in the process, and NADH is the substrate for NADH oxidase to generate reactive oxygen species (ROS)Citation8. Fructose, which is generated from the polyol pathway, is metabolized fructose-3-phosphate (F3P) and 3-deoxyglucosone (3DG). F3P and 3DG are more potent nonenzymatic glycation agentsCitation9,Citation10. Thus, the flux of glucose through the polyol pathway would increase the advanced glycation end products (AGE) formation. It is known that AGE leads to several noxious diseases such as Alzheimer’s disease, atherosclerosis, diabetes, heart failure and cancer associated with oxidative stressCitation11 (). As a result, studies on the determination of the aldose reductase inhibitors are gaining importance every day. Inhibitors of AR have been used in therapeutic applications in diabetic complicationsCitation7. Some well-known AR inhibitors are epalrestat, tolrestat, zenarestat, sorbinil, zopolrestat, ponalrestat, lidorestat, fidarestat, ranirestat, quercetin, resveratrol and ADN-158Citation3. The use of these inhibitors has remained limited due to the undesirable side effectsCitation12,Citation13. Therefore, the identification of new aldose reductase inhibitors and the inhibition of aldose reductase by natural chemicals are very important. In particular, studies using phenolic compounds as aldose reductase inhibitors are very popularCitation14–17. In recent years, there have been many studies on the isolation of phenolic compounds from traditional plants and their effects on glucose metabolism, particularly the polyol pathwayCitation18–23.
Figure 2. The relationship between oxidative stress and diabetic complications of the polyol pathway: Accelerated flux polyol pathway plays a critical role in the development of diabetic complications. Cataract is one of the diabetic complications. It is known that sorbitol accumulates in tissues and causes an increase in the osmotic pressure. Thus, water enters into the cells and swelling takes place, which can cause a cataract. Also, AR competes with glutathione reductase (GR) for their co-factor NADPH. The activity of GR is decreased when the activity of AR increased and this leading to a decrease in GSH level. Increased NADH causes NADH oxidase (NOx) to produce ROS. Fructose-3-phosphate (F-3-P) and 3-deoxyglucosone (3-DG), metabolites of fructose, increase AGE and binding of advanced glycation end product (AGE) to receptor of AGE (RAGE) increase oxidative stressCitation4.
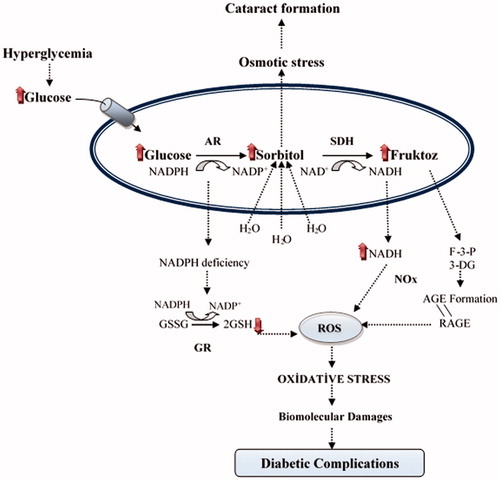
In the present study, we aimed to purify aldose reductase enzyme from rat kidney and to investigate the in vitro inhibitory effects of a series phenolic acids (). These phenolic acids are used as antioxidant food additives, prodrugs or drugs. Tannic acid, a plant polyphenol, is also used as a food additive. Its safe dosage ranges from 10 to 400 µg, depending on the type of food to which it is addedCitation24. In addition, tannic acid has been shown to have antimutagenic, anticarcinogenic and antioxidant activities by Gülçin et al.Citation25 Chlorogenic acid is a natural phenolic compound that is the ester of cinnamic acids, such as caffeic acid, ferulic and p-coumaric acids. Also, it is used as food additive. For example, green or raw coffee is a major source of chlorogenic acid in natureCitation26. Recently, it has been determined that chlorogenic acid has many health benefits such as reduction of the relative risk of cardiovascular disease, diabetes type 2, Alzheimer’s disease, and antibacterial and anti-inflammatory activitiesCitation27–29. Sinapic acid (3,5-Dimethoxy-4-hydroxycinnamic acid) is one of the important bioactive compounds. It is found in the plant kingdom in various fruits, vegetables, cereal grains, oilseed crops, some spices and medicinal plantsCitation30. Sinapic acid shows antimicrobialCitation31, anti-inflammatoryCitation32, anticancerCitation33 and anti-anxiety activitiesCitation34. Protocatechuic acid (3,4-dihydroxybenzoic acid) is a type of natural phenolic acid. Protocatechuic acid is present in most edible plants used in folk medicine. It is a compound widely found in the human diet. In particular, it presents in bran and grain brown rice (Oryza sativa L.) and onion (Allium cepa L.). It has been reported that protocatechuic acid has antioxidant, antibacterial, anticancer, antiulcer, antidiabetic, antiaging, antifibrotic, antiviral, anti-inflammatory, analgesic, antiatherosclerotic, cardiac, hepatoprotective, neurological and nephro protective activitiesCitation35. 4-Hydroxybenzoic acid is commonly used as an additive and preservative in food processing, pharmaceuticals, beverages and cosmetic productsCitation36. p-Coumaric acid (trans-4-Hydroxycinnamic acid) exists widely in fruits, such as apples and pears, and in vegetables and plant products, such as beans, potatoes, tomatoes and tea. p-Coumaric has antioxidant properties. It has been reported that it reduces the risk of stomach cancer by reducing the formation of carcinogenic nitrosaminesCitation37. Ferulic acid (4-Hydroxy-3-methoxycinnamic acid) is widely distributed in the plant kingdom and might be found in high concentrations in foods such as navy bean, corn bran, wheat bran, eggplant, artichokes and beets. Ferulic acid has hepatic, neuro and photoprotective effects, and antimicrobial and anti-inflammatory activities. It is useful in the treatments of cancer, diabetes and lung and cardiovascular diseasesCitation38. Vanillic acid (4-hydroxy-3-methoxybenzoic acid) is used as a food additive and is one of the main natural phenols in argan oil. Also, it is found in wine and vinegar. Vanillic acid has been associated with a variety of pharmacologic activities such as inhibiting snake venom activity, carcinogenesis, apoptosis and inflammationCitation39. Syringic acid shows strong antioxidant, antiproliferative anti-endotoxic, anti-cancer activity and hepatoprotective activityCitation40,Citation41. α-Resorcylic acid (3,5-dihydroxybenzoic acid) is found in peanuts (Arachis hypogaea), chickpeas (Cicer arietinum), red sandalwood (Pterocarpus santalinus) and hill raspberry (Rubus niveus). Studies show that α-resorcylic acid has nematicidal, antioxidant, adipocytes lipolysis inhibitory and polyphenoloxidase (PPO) inhibitory activityCitation42. 3-hydroxybenzoic acid is found in common plants such as grapefruit (Citrus paradisi), olive oil (Olea europaea) and medlar fruit (Mespilus germanica). It has glucosylating activityCitation43. Gallic acid is an endogenous plant polyphenol. It is found abundantly in tea, grapes, berries and other fruits as well as in wine. Gallic acid is a powerful antioxidant and it has anti-inflammatory, antimutagenic and anticancer propertiesCitation44.
Experimental
Chemistry
Tannic acid, chlorogenic acid, sinapic acid, salicylic acid, protocatechuic acid, 4-hydroxybenzoic acid, p-coumaric acid, ferulic acid, vanillic acid, syringic acid, α-resorcylic acid, 3- hydroxybenzoic acid, gallic acid, protein assay reagents, NADPH, DL-glyceraldehyde and 2′5′-ADP Sepharose 4B were obtained from Sigma-Aldrich Co. (Sigma- Aldrich Chemie GmbH Export Department Eschenstrasse 5, 82024 Taufkirchen, Germany). All other chemicals were analytical grade and obtained from Merck (Merck KGaA Frankfurter strasse 250, D 64293 Darmstadt, Germany).
Homogenate preparation and ammonium sulphate precipitation
Approximately 15 g of rat liver was homogenized in 45 ml of 10 mM Na-phosphate buffer (pH 7.4). The homogenate was centrifuged 13.500 × g for 60 min. Supernatant was used for following studies. The supernatant suspension was precipitated with ammonium sulphate. The precipitation intervals were 0%–70% for AR enzyme. The precipitate was collected by centrifugation at 13.500 × g for 30 min and redissolved in a 10 mM Na-phosphate buffer (pH 7.4). The solution was dialyzed against 10 mM Na-phosphate buffer (pH 7.4) containing 5 mM 2-mercaptoethanol.
Aldose reductase activity assay
Aldose reductase activity was assayed by following the absorbance decrease of NADPH at 340 nm spectrophotometrically. About 1 ml total volume of the enzymatic reaction mix contained 0.8 M Na-phosphate buffer (pH =5.5), 4.7 mM DL-glyceraldehyde, 0.11 mM NADPH and enzyme solutionCitation45.
Purification of aldose reductase from rat kidney
The dialyzed enzyme solution was loaded onto the DE-52 Cellulose anion exchange column previously equilibrated with 10 mM Na-phosphate buffer (pH 7.4). The enzyme did not interact with anion exchange column and eluted with the same buffer. Eluted fractions were collected, and the enzyme activity was checked at 340 nm. The fractions with the enzyme activity were pooled and mixed with glycerol. Then this enzyme solution was loaded onto the Sephadex G-100 column equilibrated with 10 mM Na-phosphate buffer (pH 7.4). Fractions were analyzed for both protein amount (280 nm) and enzyme activity (340 nm). Fractions from the Sephadex G-100 was loaded onto the 2′5′-ADP Sepharose 4B affinity column equilibrated with 10 mM Na-phosphate buffer (pH 7.4), subsequently. The column was washed with 10 mM Na-phosphate buffer (pH 7.4) and then elution was performed with linear gradient of 0.2–2.5 mM NaCl. The enzyme activity was checked at 340 nm in collected fractions, and the tubes with enzyme activity were combined. All purification procedures were performed at 4 °C. SDS polyacrylamide gel electrophoresis was done to the control of enzyme purity.
Protein determination
During the purification steps, quantitative protein determination was done by measuring the absorbance at 595 nm according to Bradford, using bovine serum albumin as a standardCitation46.
SDS–polyacrylamide gel electrophoresis
Enzyme purity was controlled according to Laemmli’s procedure. The procedure includes two different acrylamide concentrations as 3% and 8% for running and stacking gel, respectivelyCitation47. The experiment was done as our previous studiesCitation4,Citation48. SDS-PAGE gel was stained with silver reagent. The electrophoretic pattern was photographed ().
In vitro inhibition studies
In inhibition studies, aldose reductase activity was assayed by following the change in absorbance at 340 nm, spectrophotometrically. One millilitre of the reaction mix contained 0.2 M Na-phosphate buffer (pH = 6.2), 10 mM DL-glyceraldehyde, 0.5 mM NADPH and enzyme solutionCitation45. AR activity was measured in the presence of different concentrations of phenolic acids. A control sample without inhibitor was taken as 100%, and for each inhibitor, an Activity%-[Inhibitor] graphs were drawn. IC50 values were determined from Activity%-[Inhibitor] graphs. For determination of the Ki constant, three different phenolic acid concentrations were used, and DL-glyceraldehyde was also used as a substrate at five different concentrations. Ki constant obtained from the Lineweaver-Burk graph (1/V-1/[S]), and all inhibition type was found for all phenolic acids. Analysis of data obtained was made by t-test and they are given as X ± SD.
Results and discussion
It is well known that polyol pathway activity increases in cases of hyperglycemia. Aldose reductase, the first and rate-limiting enzyme in the polyol pathway, has been implicated in the aetiology of complications in diabetes such as neuropathy, nephropathy, retinopathy and cataractogenesisCitation49. Thus, aldose reductase inhibitors are potential therapeutic candidates in the treatment and prevention of diabetic complicationsCitation50,Citation51. Therefore, discovery of new and reliable aldose reductase inhibitors is required to improve the quality of life of diabetic patients by preventing complications.
In light of the above information, in the current study, one of the major goals was to investigate the in vitro inhibition effects of some phenolic acids on rat kidney aldose reductase enzyme activity. For this purpose, aldose reductase enzyme was purified from rat kidney using simple and rapid chromatographic methods. Up to now, aldose reductase has been purified from many different sources with different yields and purification folds by using distinct chromatographic techniques. For example, Wermuth et al.Citation52 purified AR from human brains using DEAE-cellulose, Sephadex G-100 and blue sepharose chromatography techniques. In two other studies, human erythrocyte and human placenta AR enzymes were purified using ion-exchange chromatography, chromatofocusing, affinity chromatography, sephadex gel filtrationCitation53 and red sepharose affinity chromatography, chromatofocusing and high performance liquid chromatography on a size-exclusion columnCitation54, respectively. In a previous study, we isolated AR with 19.34-fold from rat kidney, a yield of 3.49% and a specific activity of 0.88 U/mg using DE-52 Cellulose anion exchange chromatography, gel filtration chromatography and 2′5′ ADP Sepharose 4B affinity chromatography, respectively (). Additionally, purity of the enzyme was controlled using SDS-PAGE. The purified enzyme had a single band at around 39 kDa (). Our purification results indicate proximity with the results in the literatureCitation55. After the purification step, the inhibitory effects of some phenolic acids (tannic acid, chlorogenic acid, sinapic acid, protocatechuic acid, 4-hydroxybenzoic acid, p-coumaric acid, ferulic acid, vanillic acid, syringic acid, α-resorcylic acid, 3-hydroxybenzoic acid and gallic acid) were investigated in vitro.
Table 1. Summary of purification of aldose reductase from rat kidney.
To describe inhibitory effects, researchers often list an IC50 value; however, a more suitable measure is the Ki constant. Both the IC50 and Ki parameters of some phenolic acids were determined in this study from Activity%-[Inhibitor] graphs and Lineweaver-Burk graphs (1/V-1/[S]), respectively. The IC50 values were found by Activity%/[Inhibitor] graphs to be 0.5 µM, 5.47 µM, 0.033 mM, 0.048 mM, 0.05 mM, 0.057 mM, 0.069 mM, 0.086 mM, 0.095 mM, 0.11 mM, 0.15 mM and 0.176 mM for tannic acid, chlorogenic acid, sinapic acid, protocatechuic acid, 4-hydroxybenzoic acid, p-coumaric acid, ferulic acid, vanillic acid, syringic acid, α-resorcylic acid, 3-hydroxybenzoic acid and gallic acid, respectively. According to the results, the order of the inhibitors is as follows: tannic acid > chlorogenic acid > sinapic acid > protocatechuic acid> 4-hydroxybenzoic acid > p-coumaric acid > ferulic acid > vanillic acid > syringic acid> α-resorcylic acid > and 3-hydroxybenzoic acid > gallic acid (). For determination of the Ki constants, three different phenolic acid concentrations were used for each compound, and DL-glyceraldehyde was also used as a substrate at five different concentrations. Ki constants were obtained from the Lineweaver-Burk graph (1/V-1/[S]), and inhibition type was found for each phenolic acid.
Table 2. IC50, Ki values and inhibition types for phenolic acids used in this study.
Tannic acid has been identified in the literature as a potent inhibitor of human placental aldose reductase and the inhibition type of tannic acid was found as mixed typeCitation56. In our study, the tannic acid showed a potent inhibition effect for rat kidney aldose reductase but its inhibition type is a non-competitive. Ki value for tannic acid was found to be 0.598 µM. According to these results, we can say that the enzyme obtained from different sources may cause different inhibition results.
Chlorogenic acid has an antidiabetic effect in diabetic animal models and it has an inhibitory effect on aldose reductase enzyme as identified in literatureCitation57. However, there is no information about the inhibition type and the inhibition mechanism. Hence, in this study, we have identified Ki value and inhibition type of chlorogenic acid for rat kidney aldose reductase. Chlorogenic acid showed mixed type inhibition. Ki and Ki' values were found to be 1.563 µM and 28.05 µM, respectively.
Sinapic acid, p-coumaric acid and ferulic acid are natural hydroxycinnamic acid derivatives. The literature has shown that these hydroxycinnamic acid derivatives have an inhibition effect on aldose reductase enzyme activityCitation18 but inhibition mechanisms were not reported. In this study, we investigated the inhibition types of sinapic acid, p-coumaric acid and ferulic acid on rat kidney aldose reductase. Sinapic acid and ferulic acid showed non-competitive inhibition while p-coumaric acid exhibited uncompetitive inhibition. Ki values of Sinapic acid, p-coumaric acid and ferulic acid were found to be 0.0434 mM, 0.0531 mM and 0.0725 mM for rat kidney aldose reductase, respectively.
Protocatechuic acid, α-resorcylic acid and Vanillic acid are types of dihydroxybenzoic acid derivatives. It is clear from earlier studies that protocatechuic acidCitation58 and vanillic acidCitation18 have antiglycative effects and an inhibition effect on aldose reductase enzyme activity. However, their inhibition mechanisms are not conclusive. In addition, the inhibitory effect of α-resorcylic acid on aldose reductase enzyme activity is not known in the literature. In the present study, we determined Ki values and inhibition type of protocatechuic acid and vanillic acid and α-resorcylic acid for rat kidney aldose reductase. They exhibited non-competitive inhibition, and Ki values were found to be 0.0463 mM, 0.103 mM and 0.127 mM, respectively.
Gallic acid and syringic acid are trihydroxybenzoic acids. It has been determined that they showed an inhibitory effect on aldose reductase enzyme activity in many studiesCitation18. However, their inhibition mechanisms were not disclosed. In this study, we determined inhibition types and Ki values of these trihydroxybenzoic acids. Both of them exhibited non-competitive inhibition. Ki values of gallic acid and syringic acid were found to be 0.219 mM and 0.107 mM, respectively.
4-Hydroxybenzoic acid and 3-Hydroxybenzoic acid are monohydroxybenzoic acids. In the literature, it has been determined that 4-hydroxybenzoic acid has an inhibitory effect on aldose reductase enzyme activityCitation18 but the effect of 3-hydroxybenzoic acid on aldose reductase enzyme is unknown. We determined inhibition types of this monohydroxybenzoic acid as non-competitive, and Ki values of 4-hydroxybenzoic acid and 3-hydroxybenzoic acid were found to be 0.0522 mM and 0.176 mM, respectively.
The inhibition can be determined as a result of IC50 and Ki studies. If IC50 and Ki values of an inhibitor are smaller, its inhibition effect will be higher. According to this, tannic acid (Ki of 0.598 µM) and chlorogenic acid (Ki of 1.563 µM, Ki'of 28.05 µM) have a strong inhibition effect. Gallic acid (Ki of 0.219 mM) also had a weak inhibition effect on rat kidney aldose reductase enzyme compared to other phenolic acids in the present study (). Many -OH groups in the structure of tannic acid may cause potent inhibitory effects compared to the structures of the other phenolic compounds. In this study, all phenolic acids except chlorogenic acid and p-coumaric acid showed non-competitive inhibition on AR enzyme activity (). A non-competitive inhibitor shows its inhibitory effect by decreasing the turnover rate or catalytic activity of the enzyme. Considering the structural similarity between the used compounds, it can be seen corelation among their inhibition effects. Chlorogenic acid exhibited mixed-type inhibition while p-coumaric acid showed uncompetitive inhibition. According to this result, chlorogenic acid leads to inhibition by binding to the free enzyme or enzyme substrate complex and p-coumaric acid causes inhibition by only binding to the enzyme-substrate complex. Therefore, to suggest a specific binding mechanism is difficult for these phenolic compounds. It is planned more comprehensive studies on this mechanism.
Figure 5. A: Lineweaver–Burk graph and inhibition mechanism of tannic acid using three different tannic acid concentrations for determination of Ki and inhibition type. B: Lineweaver–Burk graph and inhibition mechanism of chlorogenic acid using three different chlorogenic acid concentrations for determination of Ki and inhibition type. C: Lineweaver–Burk graph and inhibition mechanism of p-coumaric acid using three different p-coumaric acid concentrations for determination of Ki and inhibition type.
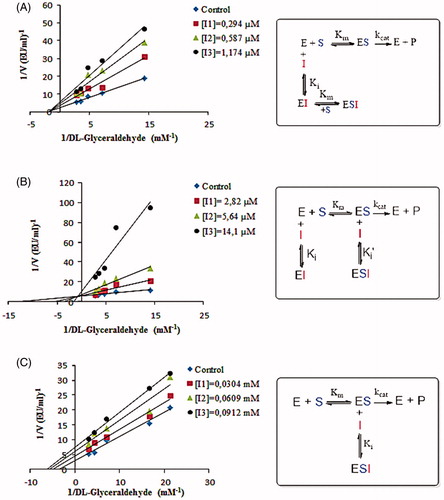
Conclusions
In conclusion, some enzymes in the glucose metabolism are drug-targets. It is well known that the polyol pathway is also a small and vital metabolic route of the glucose metabolism. This is because a lot of metabolic disorders, especially diabetic complications, are associated with this pathway. Therefore, discovery of new and natural aldose reductase inhibitors is required to improve the quality of life of diabetic patients by preventing diabetic complications. In this study, we examined the in vitro inhibition effects of some phenolic acids on AR enzyme activity and we showed that some phenolic acids could be potent inhibitors of AR. We believe that these results may be helpful to elucidate the inhibition mechanism of these phenolic acids. Moreover, we consider that these results may be important in identifying new AR inhibitors and may also provide more effective and better therapeutic agents for diabetic complications in the future
Disclosure statement
The authors report no conflicts of interest. The authors alone are responsible for the content and writing of this article.
References
- International Diabetes Federation. Diabetes Atlas. 6th ed.; 2013. Available from: http://www.idf.org/diabetesatlas.
- World Health Organization. The top ten causes of death. Available from: http://www.who.int/mediacentre/factsheets/fs310/en/#.
- Reddy TN, Ravinder M, Bagul P, et al. Synthesis and biological evaluation of new epalrestat analogues as aldose reductase inhibitors(ARIs). Eur J Med Chem 2014;71:53–66.
- Alım Z, Beydemir Z. Effects of some anti-neoplastic drugs on sheep liver sorbitol dehydrogenase. Arch Physiol Biochem 2012;118:244–52.
- Cheng HM, Gonzalez RG. The effect of high glucose and oxidative stress on lens metabolism, aldose reductase, and senile cataractogenesis. Metab Clin Exp 1986;35:10–14.
- Koya D, Haneda M, Kikkawa R, et al. D-α-Tocopherol treatment prevents glomerular dysfunctions in diabetic rats through inhibition of proteinkinase C-diacylglycerol pathway. Biofactors 1998;7:69–76.
- Patel DK, Kumar R, Sairam K, Hemalatha S. Pharmacologically tested aldose reuctase inhibitors isolated from plant sources-A concise report. Chin J Nat Med 2012;10:388–40.
- Morre DM, Lenaz G, Morre DJ. Surface oxidase and oxidative stress propagation in aging. J Exp Biol 2000;203:1513–21.
- Brownlee M. Glycation products and the pathogenesis of diabetic complications. Diabetes Care 1992;15:1835–43.
- Hamada Y, Araki N, Horiuchi S, et al. Role of poliol pathway in nonenzymatic glycation. Nephrol Dial Transplant 1996;11:5–95.
- Israël M, Schwartz L. On the metabolic origin of cancer: substances that target tumor metabolism. Biomed Res 2011;22:130–64.
- Pfeifer MA, Schumer MP, Gelber DA. Aldose reductase inhibitors: the end of an era or the need for different trial designs? Diabetes 1997;46:82–9.
- Akileshwari C, Raghu G, Muthenna P, et al. Bioflavonoid ellagic acid inhibits aldose reductase: Implications for prevention of diabetic complications. J Funct Foods 2014;6:374–83.
- Suryanarayana P, Kumar PA, Saraswat M, et al. Inhibition of aldose reductase by tannoid principles of Emblica officinalis: implications for the prevention of sugar cataract. Mol Vis 2004;10:148–54.
- Saraswat M, Muthenna P, Suryanarayana P, et al. Dietary sources of aldose reductase inhibitors: prospects for alleviating diabetic complications. Asia Pac J Clin Nutr 2008;17:558–65.
- Akileshwari C, Muthenna P, Nastasijevic B, et al. Inhibition of aldose reductase by Gentiana lutea extracts. Exp Diabetes Res 2012;2012:147965. doi: 10.1155/2012/147965.
- Muthenna P, Akileshwari C, Reddy GB. Ellagic acid, a new antiglycating agent: its inhibition of Nε-(carboxymethyl)lysine. Biochem J 2012;442:221–30.
- Chethan S, Dharmesh SM, Malleshi NG. Inhibition of aldose reductase from cataracted eye lenses by finger millet (Eleusine coracana) polyphenols. Bioorg Med Chem 2008;16:10085–90.
- Güvenç A, Okada Y, Akkol EK, et al. Investigations of anti-inflammatory, antinociceptive, antioxidant and aldose reductase inhibitory activities of phenolic compounds from Sideritis brevibracteata. Food Chem 2010;118:686–92.
- Jung HA, Nurul IMD, Kwon YS, et al. Extraction and identification of three major aldose reductase inhibitors from Artemisia montana. Food Chem Toxicol 2011;49:376–84.
- Dongare V, Kulkarni C, Kondawar M, et al. Inhibition of aldose reductase and anti-cataract action of trans-anethole isolated from Foeniculum vulgare Mill. fruits. Food Chem 2012;132:385–90.
- Ha TJ, Lee JH, Lee MH, et al. Isolation and identification of phenolic compounds from the seeds of Perilla frutescens (L.) and their inhibitory activities against α-glucosidase and aldose reductase. Food Chem 2012;135:1397–403.
- Mok SY, Lee S. Identification of flavonoids and flavonoid rhamnosides from Rhododendron mucronulatum for. Albiflorum and their inhibitory activities against aldose reductase. Food Chem 2013;136:969–74.
- Chen SC, Chung KT. Mutagenicity and antimutagenicity studies of tannic acid and its related compounds. Food Chem Toxicol 2000;38:1–5.
- Gülçin I, Huyut Z, Elmastas M, et al. Radical scavenging and antioxidant activity of tannic acid. Arab J Chem 2010;3:43–53.
- Farah A, Monteiro M, Donangelo CM, et al. Chlorogenic acids from green coffee extract are highly bioavailable in humans. J Nutr 2008;138:2309–15.
- Lindsay J, Laurin D, Verreault R, et al. Risk factors for Alzheimer’s disease: a prospective analysis from the Canadian Study of Health and Aging. Am J Epidemiol 2002;156:445–53.
- Salazar-Martinez E, Willett WC, Ascherio A, et al. Coffee consumption and risk for type 2 diabetes mellitus. Ann Intern Med 2004;140:1–8.
- Ranheim T, Halvorsen B. Coffee consumption and human health-beneficial or detrimental?-Mechanisms for effects of coffee consumption on different risk factors for cardiovascular disease and type 2 diabetes mellitus. Mol Nutr Food Res 2005;49:274–84.
- Niciforovic N, Abramovic H. Sinapic acid and its derivatives: natural sources and bioactivity. Compr Rev Food Sci F 2014;13:34–51.
- Engels C, Schieber A, Ganzle MG. Sinapic acid derivatives in defatted oriental mustard (Brassica juncea L.) seed meal extracts using UHPLC-DADESI- MSn and identification of compounds with antibacterial activity. Eur Food Res Technol 2012;234:535–42.
- Yun KJ, Koh DJ, Kim SH, et al. Anti-inflammatory effects of sinapic acid through the suppression of inducible nitric oxide synthase, cyclooxygase-2, and proinflammatory cytokines expressions via nuclear factor-κB inactivation. J Agric Food Chem 2008;56:10265–72.
- Hudson EA, Dinh PA, Kokubun T, et al. Characterization of potentially chemopreventive phenols in extracts of brown rice that inhibit the growth of human breast and colon cancer cells. Cancer Epidemiol Biomarkers Prev 2000;9:1163–70.
- Yoon BH, Jung JW, Lee JJ, et al. Anxiolytic-like effects of sinapic acid in mice. Life Sci 2007;81:234–40.
- Kakkar S, Bais S. A review on protocatechuic acid and its pharmacological potential. ISRN Pharmacol 2014;2014:952943. doi: http://dx.doi.org/10.1155/2014/952943.
- Er M, Degirmencioglu I, Tahtacı H. Novel olefinic-centered macroacyclic compounds involving tetrasubstituted 4-hydroxybenzoic acid fragments: synthesis, structural characterization and comparison of experimental and computational results. Spectrochimica Acta Part a: Mol Biomol Spectrosc 2015;139:68–74.
- Zang LY, Cosma G, Gardner H, et al. Effect of antioxidant protection by p-coumaric acid on low-density lipoprotein cholesterol oxidation. Am J Physiol Cell Physiol 2000;279:954–60.
- Paiva LB, Goldbeck R, Santos WD, et al. Ferulic acid and derivatives: molecules with potential application in the pharmaceutical field. Braz J Pharm Sci 2013;49:395–411.
- Gitzinger M, Kemmer C, Fluri DA, et al. The food additive vanillic acid controls transgene expression in mammalian cells and mice. Nucleic Acids Res 2012;40:5.
- Srinivasan S, Muthukumaran J, Muruganathan U, et al. Antihyperglycemic effect of syringic acid on attenuating the key enzymes of carbohydrate metabolism in experimental diabetic rats. Biomed Prev Nutr 2014;4:595–602.
- Muthukumaran J, Srinivasan S, Venkatesan RS, et al. Syringic acid, a novel natural phenolic acid, normalizes hyperglycemia with special reference to glycoprotein components in experimental diabetic rats. JAD 2013;2:304–9.
- Kwak SY, Noh JM, Lee S, et al. Effect of alpha-resorcylic acid–L-phenylalanine amide on collagen synthesis and matrix metalloproteinase expression in fibroblasts. Bioorg Med Chem Lett 2014;24:742–5.
- Khadem S, Marles RJ. Monocyclic phenolic acids; hydroxy- and polyhydroxybenzoic acids: occurrence and recent bioactivity studies. Molecules 2010;15:7985–8005.
- Verma S, Singh A, Mishra A. Gallic acid: molecular rival of cancer. Environ Toxicol Pharmacol 2013;35:473–85.
- Cerelli MJ, Curtis DL, Dunn JP, et al. Antiinflammatory and aldose reductase inhibitory activity of some tricyclic arylacetic acids. J Med Chem 1986;29:2347–51.
- Bradford MM. A rapid and sensitive method for the quantitation of microgram quantities of protein utilizing the principle of protein-dye binding. Anal Biochem 1976;72:248–51.
- Laemmli UK. Cleavage of structural proteins during the assembly of the head of bacteriophage T4. Nature 1970;227:680–5.
- Ekinci D, Beydemir S, Alim Z. Some drugs inhibit in vitro hydratase and esterase activities of human carbonic anhydrase-I and II. Pharmacol Rep 2007;59:580–7.
- Kato A, Yasuko H, Goto H, et al. Inhibitory effect of rhetsinine isolated from Evodia rutaecarpa on aldose reductase activity. Phytomedicine 2009;16:258–61.
- Ziegler D. Polyneuropathy in the diabetic patient-update on pathogenesis and management. Nephrol Dial Transplant 2004;19:2170–5.
- Chalk C, Benstead TJ, Moore F. Aldose reductase inhibitors for the treatment of diabetic polyneuropathy. Cochrane Database Syst Rev 2007;4:CD004572.
- Wermuth B, Bürgisser H, Bohren K, et al. Purification and characterization of human-brain aldose reductase. Eur J Biochem 1982;127:279–84.
- Das B, Srivastava SK. Purification and properties of aldose reductase and aldehyde reductase II from human erythrocyte. Arch Biochem Biophys 1985;238:670–9.
- Vander JDL, Stangebye LA, Hunsaker LA, et al. Purification of aldose reductase from human placenta and stabilization of the inhibitor binding site. Biochem Pharmacol 1988;37:1051–6.
- Moeckel G, Hallbach J, Guder WG. Purification of human and rat kidney aldose reductase. Enzyme Protein 1994;48:45–50.
- Sawada H, Hamatake M, Hara A, et al. Inhibition of human placenta aldose reductase by tannic acid. Chem Pharm Bull 1989;37:1662–4.
- Kim CS, Kim J, Lee YM, et al. Inhibitory effects of chlorogenic acid on aldose reductase activity in vitro and cataractogenesis in galactose-fed rats. Arch Pharm Res 2011;34:847–52.
- Lin CY, Tsai SJ, Huang CS, et al. Antiglycative Effects of protocatechuic acid in the kidneys of diabetic mice. J Agric Food Chem 2011;59:5117–24.