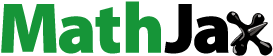
Abstract
Four human (h) carbonic anhydrase isoforms (CA, EC 4.2.1.1), hCA I, II, IV, and VII, were investigated for their activation profile with piperazines belonging to various classes, such as N-aryl-, N-alkyl-, N-acyl-piperazines as well as 2,4-disubstituted derivatives. As the activation mechanism involves participation of the activator in the proton shuttling between the zinc-coordinated water molecule and the external milieu, these derivatives possessing diverse basicity and different scaffolds were appropriate for being investigated as CA activators (CAAs). Most of these derivatives showed CA activating properties against hCA I, II, and VII (cytosolic isoforms) but were devoid of activity against the membrane-associated hCA IV. For hCA I, the KAs were in the range of 32.6–131 µM; for hCA II of 16.2–116 µM, and for hCA VII of 17.1–131 µM. The structure-activity relationship was intricate and not easy to rationalize, but the most effective activators were 1-(2-piperidinyl)-piperazine (KA of 16.2 µM for hCA II), 2-benzyl-piperazine (KA of 17.1 µM for hCA VII), and 1-(3-benzylpiperazin-1-yl)propan-1-one (KA of 32.6 µM for hCA I). As CAAs may have interesting pharmacologic applications in cognition and for artificial tissue engineering, investigation of new classes of activators may be crucial for this relatively new research field.
1. Introduction
Carbonic anhydrases (CAs, EC 4.2.1.1) are widespread metalloenzymes involved in the equilibration of carbon dioxide and bicarbonate, with formation of a protonCitation1–5. This process can be described schematically by considering EquationEquations (1(1)
(1) and Equation2)
(2)
(2) , the first being the interconversion step between CO2 and bicarbonate, and the second one, which is rate-determining for the entire catalytic cycle, regenerates the nucleophilic, zinc hydroxide species of the enzymeCitation6–9:
(1)
(1)
(2)
(2)
For this step to occur efficiently, a proton transfer reaction must take place from the Zn(II)-bound water molecule to the external medium (EquationEquation (2))(2)
(2) . Generally, this process is assisted by active site amino acid residues acting as proton shuttles, for example, His residues placed in the middle or at the entrance of the active site cavityCitation9. In many human (h) CA isoforms, such as hCA II, IV, IX, XII, etc., this role of proton shuttle is played by His64Citation9, but the possibility that a cluster of His residues (comprising residues 3, 4, 10, 15, and 64, hCA I numbering system) perform the shuttling has also been contemplatedCitation10, which may explain the fact that isoforms in which the cluster is present, such as hCA II and IX are among the most effective catalysts known in NatureCitation1,Citation10.
(3)
(3)
Enzyme–activator complexes
It has been shown mainly by one of our groupsCitation10 that endogenous compounds able to participate in proton shuttling processes, in a similar manner to His64, act as CA activators (CAAs), by a mechanism described in EquationEquation (3)(3)
(3) . The activator (A in EquationEquation (3)
(3)
(3) ) binds within the enzyme active site with formation of enzyme–activator complexesCitation7, in which the activator molecule participates to the rate-determining step of the catalytic cycle, i.e. the proton shuttling from the water molecule coordinated to zinc to the external medium. Kinetic data in the presence of CAAs demonstrated that the activator does not influence KM (the affinity for the substrate) and has an effect only on Kcat of the enzyme-catalyzed reaction, both for the esterase and CO2 hydrase activities of various CA isoformsCitation10–15. X-ray crystallography of CA–activator complexes, such as the histamine, noradrenaline, l-/d-His, l-/d-Phe or d-Trp bound to hCA I and hCA II confirmed that the activators bind indeed at the entrance of the active site, not far away from His64 (which is present in two conformation, the “in” conformation – which is at around 6 Å from Zn(II), and the conformation pointing towards the exit of the active site, the “out” conformation – at >8 Å from Zn(II) as shown in )Citation11. As seen from , the activator binding site is indeed far away from the metal ion, in the middle part of the active site, extending towards the rim of the cavity, in a region also occupied by His64 with its two different (in and out) orientations ()Citation10,Citation12–15.
Figure 1. Complex of hCA II (green, ribbon diagram, Zn(II) ion as violet sphere) with L-adrenaline, in magenta, PDB file 2HKKCitation14 and d-Trp, in yellow, PDB file 3EFICitation13b in the activator binding sites A and B, respectively. His64, in red, is shown both in its “in” and “out” conformations. The two structures were superimposed.
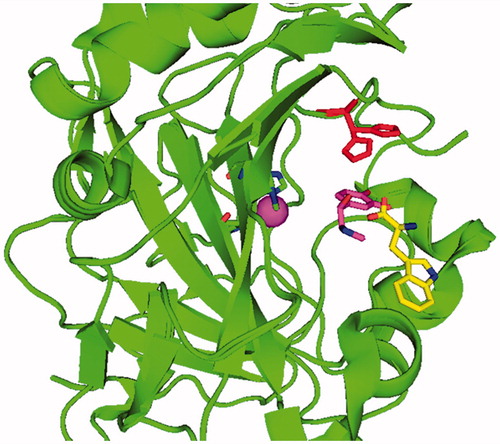
Figure 2. 4-Aminoethyl-piperazine. A: histamine; B: piperazine; C: used as leads for the CAAs investigated in this paper.
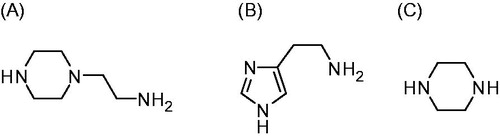
There are, in fact, two slightly different activator binding sites in α-CAs. Most activators except d-TrpCitation13b bind in the activator binding site A (shown for l-adrenaline in ), whereas d-Trp is bound in an outer binding site compared to the other activators, denominated by the activator binding site B ()Citation10,Citation12–15.
There are thirteen catalytically active mammalian CAs, CA I-VA, VB, VI, VII, IX, XII-XVCitation1–3. Apart from CA XV, which is not found in primatesCitation1, the remaining ones, CA I-XIV, are found in humans together with the murine (m) CA XV, they were investigated for their interaction with many activators, such as amino acids and aminesCitation16–19. Among them, 4-aminoethyl-piperazine A, which is structurally similar to histamine B (the first CAA investigated in detail, ) was an effective, low micromolar activator for several CA isoforms, but this was the only piperazine derivative investigated so far for such an activityCitation16–19. Thus, in this paper, we report an activation study against four CA isoforms, hCA I, II, IV, and VII, with a library of piperazines incorporating a variety of scaffolds ().
2. Experimental
2.1. Chemistry
Compounds 1–24 were either commercially, highest purity available derivatives from Sigma–Aldrich (Milan, Italy) and were used without further purification, or were prepared as described in the literatureCitation20.
2.2. Carbonic anhydrase assay
A stopped-flow methodCitation21 has been used for assaying the CA catalysed CO2 hydration activity with Phenol red as indicator, working at the absorbance maximum of 557 nm, following the initial rates of the CA-catalyzed CO2 hydration reaction for 10–100 s. For each activator, at least six traces of the initial 5–10% of the reaction have been used for determining the initial velocity. The uncatalyzed rates were determined in the same manner and subtracted from the total observed rates. Stock solutions of activator (0.1 mM) were prepared in distilled-deionized water and dilutions up to 0.1 nM were done thereafter with the assay buffer. The activation constant (KA), defined similarly with the inhibition constant KI, was obtained by considering the classical Michaelis–Menten equation (EquationEquation (4)(4)
(4) ), which has been fitted by non-linear least squares by using PRISM 3:
(4)
(4)
where [A]f is the free concentration of activator.
Working at substrate concentrations considerably lower than KM ([S] ≪ KM), and considering that [A]f can be represented in the form of the total concentration of the enzyme ([E]t) and activator ([A]t), the obtained competitive steady-state equation for determining the activation constant is given by EquationEquation (5)(5)
(5) Citation22–26:
(5)
(5)
where v0 represents the initial velocity of the enzyme-catalyzed reaction in the absence of an activator. All CA isozymes used in the experiments were purified recombinant proteins obtained as reported earlier by our groupCitation22–26.
3. Results and discussion
3.1. Chemistry
Piperazines 1–24 () were chosen to be investigated as CAAs as they contain the endocyclic NH group able to participate in proton shuttling processes between the zinc-coordinated water from the CA active site and the external medium, in a similar manner to 4-aminoethyl-piperazine A, and histamine B, which were considered as lead compounds. Furthermore, in contrast to A and B, piperazines 1–24 do not possess the aminoethyl moiety present in the two leads, but the pKa of the NH (or NR) groups from the heterocyclic ring is influenced by the diverse substitution patterns present in them. Indeed, both electron withdrawing as well as electron donating moieties are present in these compounds which may lead to a different basicity of the moieties able to shuttle protons between the enzyme active site and the reaction medium (). The unsubstituted piperazine (compound C) was also tested for comparison.
3.2. CA activation
Activation data against four physiologically relevant hCA isoforms, hCA I, II, IV, and VII, are shown in . Indeed, hCA I, II, and IV are involved in a multitude of eye diseasesCitation1,Citation27, and their inhibition is pharmacologically used for the treatment of glaucomaCitation27, edemaCitation28, obesityCitation29, and hypoxic tumorsCitation30, whereas more recently, some of these isoforms were also validated as drug targets for neuropathic painCitation31, cerebral ischemiaCitation32, and arthritisCitation33. Thus, modulators, potentially with selective action, both for inhibiting and activating these enzymes, are of great pharmacological interest. In fact, recently CAAs were shown to potentiate cognition through the phosphorylation of the extracellular signal-regulated kinase in the cortex and the hippocampus of model animalsCitation34, being thus of great interest for memory therapy. Furthermore, Muller’s group showed that CAAs potentiate the initial steps of bone formation in models of artificial mineralization processesCitation35.
Table 1. CA activation of isoforms hCA I, II, and VII (cytosolic) and IV (membrane-associated) with compounds 1-24, by a stopped-flow CO2 hydrase assayCitation21.
The activation of the four CA isoforms mentioned above with the piperazine derivatives 1–24 and two standard activators (compounds A and B) shown in allowed us to delineate the following structure-activity relationship (SAR):
Although unsubstituted piperazine C was inactive as a CAA (KAs > 150 µM against all investigated enzymes), the substituted-piperazines 1–24 showed CA activating properties against hCA I (except compounds 1, 10, 11, and 15, which had KAs > 150 µM) with activation constants ranging between 32.6 and 131 µM, being thus moderate – weak activators. Indeed, the leads A and B were much more potent, low micromolar activators of this isoform, with KAs of 2.1–7.4 µM ()Citation18. The best hCA I activators in the series of investigated compounds were 4, 19, 21, and 24 (KAs of 32.6–48.1 µM), and they belong to variously substituted piperazines. Small variations on the core structure of these compounds generally led to a diminution of the activity. For example, 19, the best hCA I activator, carries a propionyl group on the piperazine ring and a benzyl moiety in the 3 position. Its deacylated analog, 18, was almost two times a less effective hCA I activator, with a KA of 73.7 µM, compared to 19.
The physiologically dominant cytosolic isoform hCA II was more sensitive to activation with piperazines 1–24 investigated here compared to hCA I (). Thus, only 22 was inactive (KA > 150 µM), and the range of the activation constants for the remaining derivatives was of 16.2–116 µM. A number of compounds showed KAs in the range of 16.2–50.1 µM: for example, 2, 4, 14, 19, 21, 23, and 24. They belong to various chemical classes and incorporated different substituents, which demonstrates that it might be possible to design much more efficient CAAs incorporating this interesting ring. However, the simple lead compound A was a much more potent hCA II activator compared to the other piperazines investigated here, whereas histamine B was a very inefficient hCA II activator with a KA of 125 µM (). Amazingly, the best hCA II activator was 14, which has two potential piperidine rings that may participate in the proton shuttling processes.
Surprisingly, the membrane-bound isoform hCA has not activated significantly by any of the piperazines investigated here, although the leads A and B showed medium potency efficacy with KAs of 24.9–25.3 µM.
The brain cytosolic isoform hCA VII was not activated by piperazines 5, 7, and 16 (KA > 150 µM), whereas the remaining derivatives showed a profile of medium – weak activator, with KAs in the range of 17.1–131 µM (). The best hCA VII activators were 2, 17, 18, 20, and 22 (KAs in the range of 17.1–48.5 µM). For this isoform, the SAR of the couple 18/19 is completely different compared to what is mentioned above for the activation of hCA I. In this case, the deacetylatyed derivative 18 was 4.9 times a better hCA VII activator compared to the acetylated one 19. Thus, small changes in the scaffold lead to a very different activation profile in this series of piperazines and their derivatives.
4. Conclusions
We report here an activation study of four physiologically and pharmacologically relevant CA isoforms, hCA I, II, IV, and VII with a rather large series of piperazines and their derivatives. The compounds were included in order to investigate whether the fine tuning of the basicity correlated with the various shapes of these molecules may lead to efficient activators, considering the fact that the only piperazine investigated till now as activator (4-(2-aminoethy)l-piperazine) showed such interesting properties. hCA I was activated by most of the investigated derivatives, with activation constants of 32.6–131 µM; hCA II with activation constants of 16.2–116 µM, whereas the membrane-bound isoform hCA IV was not activated by the investigated piperidines. The brain-associated cytosolic isoform hCA VII was activated with KAs in the range of 17.1–131 µM. The structure-activity relationship was intricate and not easy to rationalize for each isoform, but the most effective activators were 1-(2-piperidinyl)-piperazine 14 (KA of 16.2 µM for hCA II), 2-benzyl-piperazine 18 (KA of 16.2 µM for hCA VII), and 1-(3-benzylpiperazin-1-yl)propan-1-one 19 (KA of 32.6 µM for hCA I). As CAAs may have interesting pharmacologic applications in cognition and for artificial tissue engineering, investigation of new classes of activators as the ones reported here may be crucial for this relatively new research field.
Disclosure statement
The authors do not declare conflict of interest.
Additional information
Funding
References
- Supuran CT. Structure and function of carbonic anhydrases. Biochem J 2016;473:2023–32.
- Supuran CT. How many carbonic anhydrase inhibition mechanisms exist? J Enzyme Inhib Med Chem 2016;31:345–60.
- Supuran CT. Carbonic anhydrases: novel therapeutic applications for inhibitors and activators. Nat Rev Drug Discov 2008;7:168–81.
- Domsic JF, McKenna R. Sequestration of carbon dioxide by the hydrophobic pocket of the carbonic anhydrases. Biochim Biophys Acta 2010;1804:326–31.
- (a) Supuran CT. Carbonic anhydrases: from biomedical applications of the inhibitors and activators to biotechnological use for CO2 capture. J Enzyme Inhib Med Chem 2013;28:229–30.(b) Fabrizi F, Mincione F, Somma T, et al. A new approach to antiglaucoma drugs: carbonic anhydrase inhibitors with or without NO donating moieties. Mechanism of Action and Preliminary Pharmacology. J Enzyme Inhib Med Chem 2012;27:138–47.
- (a) Supuran CT. Structure-based drug discovery of carbonic anhydrase inhibitors. J. Enzyme Inhib Med Chem 2012;27:759–72.(b) Scozzafava A, Menabuoni L, Mincione F, Supuran CT. Carbonic anhydrase inhibitors. A general approach for the preparation of water-soluble sulfonamides incorporating polyamino-polycarboxylate tails and of their metal complexes possessing long-lasting, topical intraocular pressure-lowering properties. J Med Chem 2002;45:1466–76.
- Supuran CT. Carbonic anhydrase activators. Part 4. A general mechanism of action for activators of isozymes I, II and III. Rev Roum Chim 1992;37:411–21.
- Alterio V, Di Fiore A, D'Ambrosio K, et al. Multiple binding modes of inhibitors to carbonic anhydrases: how to design specific drugs targeting 15 different isoforms? Chem Rev 2012;112:4421–68.
- Tu CK, Silverman DN, Forsman C, et al. Role of histidine 64 in the catalytic mechanism of human carbonic anhydrase II studied with a site-specific mutant. Biochemistry 1989;28:7913–8.
- Briganti F, Mangani S, Orioli P, et al. Carbonic anhydrase activators: X-ray crystallographic and spectroscopic investigations for the interaction of isozymes I and II with histamine. Biochemistry 1997;36:10384–92.
- Nair SK, Christianson DW. Unexpected pH-dependent conformation of His-64, the proton shuttle of carbonic anhydrase II. J Am Chem Soc 1991;113:9455–8.
- (a) Temperini C, Scozzafava A, Supuran CT. Carbonic anhydrase activation and the drug design. Curr Pharm Des 2008;14:708–15.(b) Temperini C, Scozzafava A, Vullo D, Supuran CT. Carbonic anhydrase activators. Activation of isozymes I, II, IV, VA, VII, and XIV with L- and D-histidine and crystallographic analysis of their adducts with isoform II: engineering proton-transfer processes within the active site of an enzyme. Chemistry 2006;12:7057–66.
- (a) Temperini C, Scozzafava A, Vullo D, Supuran CT. Carbonic anhydrase activators. Activation of isoforms I, II, IV, VA, VII, and XIV with l- and d-phenylalanine and crystallographic analysis of their adducts with isozyme II: stereospecific recognition within the active site of an enzyme and its consequences for the drug design. J Med Chem 2006;49:3019–27. (b) Temperini C, Innocenti A, Scozzafava A, Supuran CT. Carbonic anhydrase activators: kinetic and X-ray crystallographic study for the interaction of D- and L-tryptophan with the mammalian isoforms I–XIV. Bioorg Med Chem 2008;16:8373–8.
- Temperini C, Innocenti A, Scozzafava A, et al. Carbonic anhydrase activators: l - adrenaline plugs the active site entrance of isozyme II, activating better isoforms I, IV, VA, VII, and XIV. Bioorg Med Chem Lett 2007;17:628–35.
- (a) Temperini C, Scozzafava A, Puccetti L, Supuran CT. Carbonic anhydrase activators: X-ray crystal structure of the adduct of human isozyme II with L-histidine as a platform for the design of stronger activators. Bioorg Med Chem Lett 2005;15:5136–41. (b) Temperini C, Scozzafava A, Supuran CT. Carbonic anhydrase activators: the first X-ray crystallographic study of an adduct of isoform I. Bioorg Med Chem Lett 2006;16:5152–6.
- (a) Vullo D, Nishimori I, Innocenti A, et al. Carbonic anhydrase activators: an activation study of the human mitochondrial isoforms VA and VB with amino acids and amines. Bioorg Med Chem Lett 2007;17:1336–40.(b) Pastorekova S, Vullo D, Nishimori I, et al. Carbonic anhydrase activators: activation of the human tumor-associated isozymes IX and XII with amino acids and amines. Bioorg Med Chem 2008;16:3530–6.
- (a) Nishimori I, Onishi S, Vullo D, et al. Carbonic anhydrase activators. The first activation study of the human secretory isoform VI. Bioorg Med Chem 2007;15:5351–7.(b) Parkkila S, Vullo D, Puccetti L, et al. Carbonic anhydrase activators: activation of isozyme XIII with amino acids and amines. Bioorg Med Chem Lett 2006;16:3955–9.
- (a) Vullo D, Innocenti A, Nishimori I, et al. Carbonic anhydrase activators: activation of the human isoforms VII (cytosolic) and XIV (transmembrane) with amino acids and amines. Bioorg Med Chem Lett 2007;17:4107–12.(b) Vullo D, Nishimori I, Scozzafava A, Supuran CT. Carbonic anhydrase activators: activation of the human cytosolic isozyme III and membrane-associated isoform IV with amino acids and amines. Bioorg Med Chem Lett 2008;18:4303–7.
- (a) Innocenti A, Hilvo M, Parkkila S, et al. Carbonic anhydrase activators. Activation of the membrane-associated isoform XV with amino acids and amines. Bioorg Med Chem Lett 2009; 19:3430–3.(b) Clare BW, Supuran CT. Carbonic anhydrase activators. 3: structure-activity correlations for a series of isozyme II activators. J Pharm Sci 1994;83:768–73.
- Guandalini L, Martino MV, Di Cesare Mannelli L, et al. Substituted piperazines as nootropic agents: 2- or 3-phenyl derivatives structurally related to the cognition-enhancer DM235. Bioorg Med Chem Lett 2015;25:1700–4.
- Khalifah RG. The carbon dioxide hydration activity of carbonic anhydrase. I. Stop-flow kinetic studies on the native human isoenzymes B and C. J Biol Chem 1971;246:2561–73.
- Supuran CT, Barboiu M, Luca C, et al. Carbonic anhydrase activators. Part 14. Synthesis of mono- and bis- pyridinium salt derivatives of 2-amino-5-(2-aminoethyl)- and 2-amino-5-(3-aminopropyl)-1,3,4-thiadiazole, and their interaction with isozyme II. Eur J Med Chem 1996;31:597–606.
- Ilies MA, Banciu MD, Ilies M, et al. Carbonic anhydrase activators. Part 17. Synthesis and activation study of a series of 1-(1,2,4-triazole-(1H)-3-yl)-2,4,6-trisubstituted-pyridinium salts against isozymes I, II, and IV. Eur J Med Chem 1997;32:911–8.
- Licsandru E, Tanc M, Kocsis I, et al. A class of carbonic anhydrase I - selective activators. J Enzyme Inhib Med Chem 2017;32:37–46.
- Angeli A, Vaiano F, Mari F, et al. Psychoactive substances belonging to the amphetamine class potently activate brain carbonic anhydrase isoforms VA, VB, VII, and XII. J Enzyme Inhib Med Chem 2017;32:1253–9.
- Akocak S, Lolak N, Vullo D, et al. Synthesis and biological evaluation of histamine Schiff bases as carbonic anhydrase I, II, IV, VII, and IX activators. J Enzyme Inhib Med Chem 2017;32:1305–12.
- (a) Masini E, Carta F, Scozzafava A, Supuran CT. Antiglaucoma carbonic anhydrase inhibitors: a patent review. Expert Opin Ther Pat 2013;23:705–16.(b) Puccetti L, Fasolis G, Vullo D, et al. Carbonic anhydrase inhibitors. Inhibition of cytosolic/tumor-associated carbonic anhydrase isozymes I, II, IX, and XII with Schiff's bases incorporating chromone and aromatic sulfonamide moieties, and their zinc complexes. Bioorg Med Chem Lett 2005;15:3096–101.(c) Scozzafava A, Menabuoni L, Mincione F, et al. Carbonic anhydrase inhibitors: perfluoroalkyl/aryl-substituted derivatives of aromatic/heterocyclic sulfonamides as topical intraocular pressure-lowering agents with prolonged duration of action. J Med Chem 2000;43:4542–51.(d) Supuran CT, Mincione F, Scozzafava A, et al. Carbonic anhydrase inhibitors–part 52. Metal complexes of heterocyclic sulfonamides: a new class of strong topical intraocular pressure-lowering agents in rabbits. Eur J Med Chem 1998;33:247–54.
- (a) Carta F, Supuran CT. Diuretics with carbonic anhydrase inhibitory action: a patent and literature review (2005–2013). Expert Opin Ther Pat 2013;23:681–91.(b) Supuran CT. Carbonic anhydrase inhibitors: an editorial. Expert Opin Ther Pat 2013;23:677–9.
- (a) Scozzafava A, Supuran CT, Carta F. Antiobesity carbonic anhydrase inhibitors: a literature and patent review. Expert Opin Ther Pat 2013;23:725–35.(b) Carta F, Scozzafava A, Supuran CT. Sulfonamides: a patent review (2008–2012). Expert Opin Ther Pat 2012;22:747–58.
- (a) Neri D, Supuran CT. Interfering with pH regulation in tumours as a therapeutic strategy. Nat Rev Drug Discov 2011;10:767–77.(b) Monti SM, Supuran CTD, Simone G. Anticancer carbonic anhydrase inhibitors: a patent review (2008–2013). Expert Opin Ther Pat 2013;23:737–49.(c) Supuran CT. Carbonic anhydrase inhibition and the management of hypoxic tumors. Metabolites 2017;7:E48.(d) Abbate F, Winum JY, Potter BV, et al. Carbonic anhydrase inhibitors: X-ray crystallographic structure of the adduct of human isozyme II with EMATE, a dual inhibitor of carbonic anhydrases and steroid sulfatase. Bioorg Med Chem Lett 2004;14:231–4.
- (a) Carta F, Di Cesare Mannelli L, Pinard M, et al. A class of sulfonamide carbonic anhydrase inhibitors with neuropathic pain modulating effects. Bioorg Med Chem 2015;23:1828–40.(b) Supuran CT. Carbonic anhydrase inhibition and the management of neuropathic pain. Expert Rev Neurother 2016;16:961–8.
- Di Cesare Mannelli L, Micheli L, Carta F, et al. Carbonic anhydrase inhibition for the management of cerebral ischemia: in vivo evaluation of sulfonamide and coumarin inhibitors. J Enzyme Inhib Med Chem 2016; 31:894–9.
- (a) Margheri F, Ceruso M, Carta F, et al. Overexpression of the transmembrane carbonic anhydrase isoforms IX and XII in the inflamed synovium. J Enzyme Inhib Med Chem 2016;31:60–3.(b) Bua S, D, Cesare Mannelli L, Vullo D, et al. Design and synthesis of novel nonsteroidal anti-inflammatory drugs and carbonic anhydrase inhibitors hybrids (NSAIDs-CAIs) for the treatment of rheumatoid arthritis. J Med Chem 2017;60:1159–70.
- Canto de Souza L, Provensi G, Vullo D, et al. Carbonic anhydrase activation enhances object recognition memory in mice through phosphorylation of the extracellular signal-regulated kinase in the cortex and the hippocampus. Neuropharmacology 2017;118:148–56.
- (a) Wang X, Schröder HC, Schlossmacher U, et al. Modulation of the initial mineralization process of SaOS-2 cells by carbonic anhydrase activators and polyphosphate. Calcif Tissue Int 2014;94:495–509.(b) Müller WE, Schröder HC, Schlossmacher U, et al. Induction of carbonic anhydrase in SaOS-2 cells, exposed to bicarbonate and consequences for calcium phosphate crystal formation. Biomaterials 2013;34:8671–80.