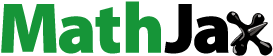
Abstract
On account of their significance as apoptosis inducing agents, merging indole and 3-hydrazinoindolin-2-one scaffolds is a logic tactic for designing pro-apoptotic agents. Consequently, 27 hybrids (6a–r, 9a–f and 11a–c) were synthesised and evaluated for their cytotoxicity against MCF-7, HepG-2 and HCT-116 cancer cell lines. SAR studies unravelled that N-propylindole derivatives were the most active compounds such as 6n (MCF-7; IC50=1.04 µM), which displayed a significant decrease of cell population in the G2/M phase and significant increase in the early and late apoptosis by 19-folds in Annexin-V-FTIC assay. Also, 6n increased the expression of caspase-3, caspase-9, cytochrome C and Bax and decreased the expression of Bcl-2. Moreover, compounds 6i, 6j, 6n and 6q generated ROS by significant increase in the level of SOD and depletion of the levels of CAT and GSH-Px in MCF-7.
Introduction
Apoptosis, programmed cell death, is considered as an essential mechanism by the body to get rid of unwanted cells. Therefore, triggering apoptosis in cancer cells will lead to automatic death and increase the relief from cancer proliferation. Thoroughly understanding the mechanism of apoptosis reveals that it is affected by the expression of caspases, Bcl-2 family proteins, including either anti-apoptotic or pro-apoptotic members. Induction of apoptosis is considered as one of the most successful strategies to target cancerCitation1.
Indole is a well-known interesting scaffold to generate anticancer agents through induction of apoptosis. Indole-3-carbinol (I3C) (), a naturally occurring compound found in family Cruciferae, displayed a remarkable activity against different types of cancer cells including breast, colon, leukaemia and prostate. This activity was justified by its ability to induce apoptosis through arresting G1/S phase of cell cycleCitation2,Citation3. Interestingly, under acidic conditions, indole-3-carbinol is dimerised into 3,3′-diindolylmethane (DIM) which exhibited superior activity as apoptosis inducing agent over indole-3-carbinolCitation2,Citation3. Moreover, Phemindole () overcame the poor bioavailability of DIM and illustrated apoptotic activity against triple negative breast cancer cells (MDA-MB-231) through reactive oxygen species (ROS) mediated mitochondrial-dependent apoptosisCitation4. Modifications for both I3C and DIM took place to get more active compounds. For example, OSU-A9, N-substituted derivative of I3C, was 100 times more potent than the parent compound (the range of IC50 of OSU-A9 against MDA-MB-231, MCF-7 and SKBR3 is 1.2–1.8 µM and IC50 of IC3 = 200 µM) with more acid stability against both breast and prostate cancer cells ()Citation5,Citation6. In addition, 1,1-Bis(3'-indolyl)-1-(p-hydroxyphenyl)methane (I), a derivative of Phemindole, was found to promote apoptosis in both pancreatic and colon cancer cells in vivo as well as in vitroCitation7,Citation8.
On the other hand, several 3-hydrazinoindolin-2-one derivatives proved their anticancer activity through induction of apoptosis in various cancer cellsCitation9. For instance, compounds IIa–c () showed cytotoxic activity against HepG2 cancer cells with IC50 range (1.0–2.4 µM). The apoptosis in HepG2 cells by compounds IIa–c was investigated through increasing the expression of Bax, a pro-apoptotic protein, and decreasing the expression of Bcl-2, an anti-apototic protein, accompanied by high levels of caspase-3Citation10. Moreover, compound III, a water-soluble oxindole derivative (), possessed EC50 = 0.14 µM against human colorectal cells; HCT-116. It had an inhibitory activity against tubulin polymerisation (IC50 = 0.19 µM) through induction of apoptosisCitation11. Furthermore, compound IV had the ability to activate caspase 3/7 and promote apoptosis in Panc1 cellsCitation12. The use of 3-hydrazinoindolin-2-one as apoptosis inducer was augmented by designing bis-isatin hydrazones connected with linker as in compound V (). It showed cytotoxic activity against two cancer cell lines, namely MCF-7 and HCT-116 with an IC50 of 1.84 µM and 3.31 µM, respectively. The pro-apoptotic activity of this compound was assured by cell cycle disruption and Annexin-V FTIC analysisCitation13.
Inspired by these findings and as a part of our ongoing efforts towards developing novel and potent anticancer agents based on the indoline-2-one moietyCitation14, we herein report the design and synthesis of new three different sets of indole-indolin-2-one hybrids 6a–r, 9a–f and 11a–c (), with the prime goal of developing potent anticancer agents with potential pro-apoptotic activity. First, methylenehydrazono (HC=N–N=) linker was selected to conjugate the indole and indolin-2-one moieties. Then, three strategies were applied to develop the three hybrids series. For the first series 6a–r, indole-3-carboxaldehyde or a variety of N-alkylated indole derivatives (N-methyl, N-propyl or N-benzyl) was conjugated with 5-/7-substituted indolin-2-one moieties. In the second series 9a–f, indole-3-carboxaldehyde was hybridised with a variety of N-alkylated indolin-2-one derivatives (N-methyl, N-propyl or N-benzyl). Regarding the third series, it was designed so as to maintain the pharmacophoric N-propyl-indole moiety while exploring the chemical variation at the N-position of the indolin-2-one moiety, in an attempt to optimise the obtained cytotoxicity results from the first and the second series and to carry out further elaboration of the indole-indolin-2-one hybrid scaffolds to explore a valuable SAR.
All compounds will be subjected to cytotoxic activity. According to the results, the most active hybrid will be further investigated regarding its effects on cell cycle progression and potential apoptotic effect in the MCF-7 cells, to acquire perception of the mechanism of the anti-proliferative activity of the synthesised hybrids. As inhibition of apoptotic markers (as Bcl-2, Bax, Caspase 3 and 9 and cytochrome C) leads to the accumulation of ROS and results in oxidative stressCitation15, some oxidative stress parameters will be taken into consideration in our biological study.
Materials and methods
Chemistry
Melting points were measured with a Stuart melting point apparatus and were uncorrected. Infrared (IR) Spectra were recorded as KBr disks using Schimadzu FT-IR 8400S spectrophotometer (Shimadzu, Kyoto, Japan). Mass spectral data are given by GCMS-QP1000 EX spectrometer at 70 eV. NMR spectra were recorded on a Varian Mercury NMR spectrometer. 1H spectrum was run at 400 MHz and 13C spectrum was run at 100 MHz in deuterated dimethylsulphoxide (DMSO-d6). Chemical shifts are expressed in values (ppm) using the solvent peak as internal standard. All coupling constant (J) values are given in Hertz. The abbreviations used are as follows: s, singlet; d, doublet; m, multiplet. Elemental analyses were carried out at the Regional Center for Microbiology and Biotechnology, Al-Azhar University, Cairo, Egypt. Analytical thin layer chromatography (TLC) on silica gel plates containing UV indicator was employed routinely to follow the course of reactions and to check the purity of products.
Synthesis of 1H-indole-3-carbaldehyde
Compound 2 was prepared according to the literature procedureCitation16.
Synthesis of 3-hydrazonoindolin-2-ones 4a–f
To a stirred solution of isatins 3a–f (5 mmol) in methyl alcohol (10 ml), 99% hydrazine hydrate (1.25 ml, 25 mmol) was added. The reaction mixture was refluxed for 1 h, and then the formed precipitate was filtered off, washed with cold methanol, dried and recrystallised from glacial acetic acid to furnish hydrazones 4a–fCitation17.
Synthesis of N-substituted-1H-indole-3-carbaldehyde derivatives 5a–c
Compounds 5a–c were prepared according to the literature procedureCitation18.
General procedure for the synthesis of target compounds 6a–r
A mixture of 3-hydrazonoindolin-2-ones 4a–f (1 mmol) and 1H-indole-3-carbaldehyde 2 or the appropriate N-substituted-1H-indole-3-carbaldehyde 5a–c (1 mmol), was refluxed in absolute ethyl alcohol in the presence of a catalytic amount of glacial acetic acid for 3 h, and then cooled to room temperature. The solid formed was collected by filtration, dried and crystallised from dioxane to produce compounds 6a–r, respectively.
3-(((1H-indol-3-yl)methylene)hydrazono)indolin-2-one (6a)
Yield 80%, m.p. 262–263 °C; IR (KBr, ν cm−1): 3409 (NH), 1697 (C=O); 1H NMR (DMSO-d6) δ (ppm): 6.89 (d, 1H, Ar-H, J = 8.0 Hz), 7.05 (t, 1H, Ar-H, J = 7.6 Hz), 7.27–7.38 (m, 3H, Ar-H), 7.53–7.55 (m, 1H, Ar-H), 8.21 (s, 1H, -CH=N-), 8.28–8.30 (m, 1H, Ar-H), 8.37 (d, 1H, Ar-H, J = 7.6 Hz), 8.94 (s, 1H, H-2 indole), 10.74 (s, 1H, NH isatin, D2O exchangeable), 12.14 (s, 1H, NH indole, D2O exchangeable); 13C NMR (DMSO-d6) δ ppm: 111.04, 112.66, 113.13, 117.60, 121.70, 122.36, 122.47, 123.89, 124.96, 128.11, 133.16, 136.84, 138.03, 144.70, 149.58, 162.28, 165.82 (C=O); MS m/z [%]: 288 [M+, 31.53] 116 [100]; Anal. calcd. For C17H12N4O: C, 70.82; H, 4.20; N, 19.43; Found C, 71.04; H, 4.27; N, 19.70.
3-(((1H-indol-3-yl)methylene)hydrazono)-5-chloroindolin-2-one (6b)
Yield 75%, m.p. > 300 °C; IR (KBr, ν cm−1): 3427 (NH), 1703 (C=O); 1H NMR (DMSO-d6) δ (ppm): 6.90 (d, 1H, Ar-H, J = 8.0 Hz), 7.26–7.33 (m, 2H, Ar-H), 7.41 (dd, 1H, Ar-H, J = 8.4, 2.4 Hz), 7.55 (d, 1H, Ar-H, J = 7.6 Hz), 8.26–8.29 (m, 2H, –CH=N– and Ar-H), 8.50 (d, 1H, Ar-H, J = 2.4 Hz), 9.00 (s, 1H, H-2 indole), 10.87 (s, 1H, NH isatin, D2O exchangeable), 12.23 (s, 1H, NH indole, D2O exchangeable); 13C NMR (DMSO-d6) δ ppm: 111.17, 112.76, 113.37, 117.99, 121.60, 122.35, 124.15, 124.82, 126.66, 127.31, 132.18, 138.10, 138.17, 144.32, 148.07, 164.08, 164.15 (C=O); Anal. calcd. For C17H11ClN4O: C, 63.26; H, 3.44, N, 17.36; Found C, 63.43; H, 3.49; N, 17.61.
3-(((1H-indol-3-yl)methylene)hydrazono)-5-bromoindolin-2-one (6c)
Yield 75%, m.p. > 300 °C; IR (KBr, ν cm−1): 3381 (NH), 1695 (C=O); 1H NMR (DMSO-d6) δ (ppm): 6.87 (d, 1H, Ar-H, J = 8.0 Hz), 7.30–7.55 (m, 4H, Ar-H), 8.25–8.28 (m, 2H, –CH=N– and Ar-H), 8.68 (s, 1H, Ar-H), 9.00 (s, 1H, H-2 indole), 10.88 (s, 1H, NH isatin, D2O exchangeable), 12.24 (s, 1H, NH indole, D2O exchangeable); 13C NMR (DMSO-d6) δ ppm: 112.82, 112.95, 113.34, 113.71, 119.25, 121.67, 122.34, 124.13, 124.81, 130.47, 135.09, 137.99, 138.15, 143.59, 148.78, 163.88, 165.47 (C=O); MS m/z [%]: 369 [(M + 2)+, 1.21], 367 [M+, 1.66], 116 [100]; Anal. calcd. For C17H11BrN4O: C, 55.61; H, 3.02; N, 15.26; Found C, 55.89; H, 2.98; N, 15.44.
3-(((1H-indol-3-yl)methylene)hydrazono)-5-methoxyindolin-2-one (6d)
Yield 83%, m.p. 291–293 °C; IR (KBr, ν cm−1): 3395 (NH), 1711 (C=O); 1H NMR (DMSO-d6) δ (ppm): 3.78 (s, 3H, OCH3), 6.80 (d, 1H, Ar-H, J = 8.4 Hz), 6.95 (dd, 1H, Ar-H, J = 8.4, 2.4 Hz), 7.19 (t, 1H, Ar-H, J = 8.0 Hz), 7.27 (t, 1H, Ar-H, J = 8.4 Hz), 7.53 (d, 1H, Ar-H, J = 8.4 Hz), 8.08 (d, 1H, Ar-H, J = 2.8 Hz), 8.21 (s, 1H, -CH=N-), 8.35 (d, 1H, Ar-H, J = 8.4 Hz), 8.95 (s, 1H, H-2 indole), 10.53 (s, 1H, NH isatin, D2O exchangeable), 12.16 (s, 1H, NH indole, D2O exchangeable); 13C NMR (DMSO-d6) δ ppm: 55.98 (OCH3), 111.76, 112.76, 112.77, 113.23, 117.92, 119.83, 121.86, 122.06, 124.02, 124.91, 137.23, 138.09, 138.50, 150.07, 155.07, 162.78, 166.01 (C=O); MS m/z [%]: 318 [M+, 1.32]; Anal. calcd. For C18H14N4O2: C, 67.92; H, 4.43; N, 17.60; Found C, 68.17; H, 4.52; N, 17.89.
3-(((1-Methyl-1H-indol-3-yl)methylene)hydrazono)indolin-2-one (6e)
Yield 78%, m.p. 255–257 °C; IR (KBr, ν cm−1): 3386 (NH), 1701 (C=O); 1H NMR (DMSO-d6) δ (ppm): 3.90 (s, 3H, CH3), 6.88 (d, 1H, Ar-H, J = 8.0 Hz), 7.04 (t, 1H, Ar-H, J = 7.6 Hz), 7.33–7.38 (m, 3H, Ar-H), 7.60–7.62 (m, 1H, Ar-H), 8.21 (s, 1H, -CH=N-), 8.27–8.29 (m, 1H, Ar-H), 8.36 (d, 1H, Ar-H, J = 7.6 Hz), 8.92 (s, 1H, H-2 indole), 10.73 (s, 1H, NH isatin, D2O exchangeable), 13C NMR (DMSO-d6) δ ppm: 33.77 (CH3), 111.05, 111.59 (2C), 117.63, 121.77, 122.49, 122.68, 123.94, 125.49, 128.16, 133.17, 138.63, 139.85, 144.74, 149.58, 161.76, 165.83 (C=O); Anal. calcd. For C18H14N4O: C, 71.51; H, 4.67; N, 18.53; Found C, 71.68; H, 4.52; N, 18.81.
5-Chloro-3-(((1-methyl-1H-indol-3-yl)methylene)hydrazono)indolin-2-one (6f)
Yield 80%, m.p. 285–287 °C; IR (KBr, ν cm−1): 3414 (NH), 1708 (C=O); 1H NMR (DMSO-d6) δ (ppm): 3.92 (s, 3H, CH3), 6.88 (d, 1H, Ar-H, J = 8.0 Hz), 7.31–7.54 (m, 4H, Ar-H), 8.23–8.28 (m, 2H, -CH=N- and Ar-H), 8.63 (s, 1H, Ar-H), 8.99 (s, 1H, H-2 indole), 10.73 (s, 1H, NH isatin, D2O exchangeable); 13C NMR (DMSO-d6) δ ppm: 33.87 (CH3), 111.68, 111.86, 112.51, 118.75, 121.70, 122.65, 124.19, 125.31, 126.08, 127.59, 132.40, 138.79, 140.96, 143.29, 148.78, 163.23, 165.58 (C=O); Anal. calcd. For C18H13ClN4O: C, 64.20; H, 3.89; N, 16.64; Found C, 64.43; H, 3.93; N, 16.87.
5-Bromo-3-(((Z)-(1-methyl-1H-indol-3-yl)methylene)hydrazono)indolin-2-one (6g)
Yield 77%, m.p. 295–297 °C; IR (KBr, ν cm−1): 3390 (NH), 1695 (C=O); 1H NMR (DMSO-d6) δ (ppm): 3.91 (s, 3H, CH3), 6.86 (d, 1H, Ar-H, J = 7.6 Hz), 7.33–7.38 (m, 2H, Ar-H), 7.54 (d, 1H, Ar-H, J = 7.2 Hz), 7.63 (d, 1H, Ar-H, J = 7.2 Hz), 8.24–8.29 (m, 2H, -CH=N- and Ar-H), 8.66 (s, 1H, Ar-H), 8.97 (s, 1H, H-2 indole), 10.87 (s, 1H, NH isatin, D2O exchangeable); 13C NMR (DMSO-d6) δ ppm: 33.84 (N-CH3), 111.71, 111.79, 112.92, 113.69, 119.22, 121.75, 122.64, 124.16, 125.23, 130.43, 135.06, 138.75, 141.07, 143.56, 148.70, 163.38, 165.45 (C=O); Anal. calcd. For C18H13BrN4O: C, 56.71; H, 3.44, N, 14.70; Found C, 57.02; H, 3.48; N, 14.96.
5-Methoxy-3-(((1-methyl-1H-indol-3-yl)methylene)hydrazono)indolin-2-one (6h)
Yield 82%, m.p. 281–283 °C; IR (KBr, ν cm−1): 3427 (NH), 1705 (C=O); 1H NMR (DMSO-d6) δ (ppm): 3.79 (s, 3H, OCH3), 3.92 (s, 3H, CH3), 6.79 (d, 1H, Ar-H, J = 8.0 Hz), 6.93 (dd, 1H, Ar-H, J = 8.0, 2.4 Hz), 7.21 (t, 1H, Ar-H, J = 8.0 Hz), 7.32 (t, 1H, Ar-H, J = 8.0 Hz), 7.55 (d, 1H, Ar-H, J = 8.4 Hz), 8.06 (d, 1H, Ar-H, J = 2.4 Hz), 8.19 (s, 1H, -CH=N-), 8.34 (d, 1H, Ar-H, J = 8.4 Hz), 8.96 (s, 1H, H-2 indole), 10.49 (s, 1H, NH isatin, D2O exchangeable); 13C NMR (DMSO-d6) δ ppm: 33.74 (N-CH3), 55.92 (OCH3), 111.64 (2 C), 111.71, 112.68, 117.87, 119.77, 121.94, 122.43, 124.01, 125.29, 138.46, 138.65, 140.35, 149.99, 155.02, 162.30, 165.97 (C=O); MS m/z [%]: 332 [M+, 67.77], 289 [100]; Anal. calcd. For C19H16N4O2: C, 68.66; H, 4.85, N, 16.86; Found C, 68.91; H, 4.92; N, 17.09.
3-(((1-Propyl-1H-indol-3-yl)methylene)hydrazono)indolin-2-one (6i)
Yield 75%, m.p. 118–120 °C; IR (KBr, ν cm−1): 3415 (NH), 1698 (C=O); 1H NMR (DMSO-d6) δ (ppm): 0.85 (t, 3H, –CH2–CH2–CH3, J = 7.2 Hz), 1.81 (sextet, 2H, –CH2–CH2–CH3, J = 7.2 Hz), 4.24 (t, 2H, –CH2–CH2–CH3, J = 7.2 Hz), 6.88 (d, 1H, Ar-H, J = 8.0 Hz), 7.04 (t, 1H, Ar-H, J = 8.0 Hz), 7.33–7.38 (m, 3H, Ar-H), 7.66–7.68 (m, 1H, Ar-H), 8.26 (s, 1H, -CH=N-), 8.28–8.30 (m, 1H, Ar-H), 8.36 (d, 1H, Ar-H, J = 7.6 Hz), 8.92 (s, 1H, H-2 indole), 10.73 (s, 1H, NH isatin, D2O exchangeable); 13C NMR (DMSO-d6) δ ppm: 11.53 (–CH2–CH2–CH3), 23.28 (–CH2–CH2–CH3), 48.26 (–CH2–CH2–CH3), 111.05, 111.70, 111.74, 117.64, 121.93, 122.49, 122.62, 123.92, 125.56, 128.15, 133.18, 138.01, 139.08, 144.70, 149.61, 161.80, 165.83 (C=O); Anal. calcd. For C20H18N4O: C, 72.71; H, 5.49, N, 16.96; Found C, 72.88; H, 5.56; N, 17.24.
5-Chloro-3-(((1-propyl-1H-indol-3-yl)methylene)hydrazono)indolin-2-one (6j)
Yield 80%, m.p. 141–142 °C; IR (KBr, ν cm−1): 3406 (NH), 1712 (C=O); 1H NMR (DMSO-d6) δ (ppm): 0.85, 1.02 (2t, 3H, –CH2–CH2–CH3, J = 7.6 Hz), 1.80, 3.40 (2sextet, 2H, –CH2–CH2–CH3, J = 7.2 Hz), 4.25, 4.31 (2t, 2H, –CH2–CH2–CH3, J = 7.2 Hz), 6.83, 6.90 (2d, 1H, Ar-H, J = 8.4 Hz), 7.14, 7.41 (2dd, 1H, Ar-H, J = 8.4, 2.0 Hz), 7.29–7.38 (m, 3H, Ar-H), 7.60, 7.69 (2d, 1H, Ar-H, J = 8.0 Hz), 8.29, 8.30 (2 s, 1H, -CH=N-), 8.48 (d, 1H, Ar-H, J = 2.4 Hz), 8.97, 8.99 (2 s, 1H, H-2 indole), 10.78, 10.87 (2 s, 1H, NH isatin, D2O exchangeable); 13C NMR (DMSO-d6) δ ppm: 11.45, 11.51 (–CH2–CH2–CH3), 23.15, 23.24 (–CH2–CH2–CH3), 48.28, 48.33 (–CH2–CH2–CH3), 111.82, 111.84, 111.95, 112.49, 117.40, 117.52, 118.74, 121.51, 121.64, 121.89, 122.58, 122.85, 123.91, 124.15, 124.53, 125.15, 125.40, 125.51, 126.10, 126.80, 127.60, 132.38, 137.52, 137.62, 138.15, 140.11, 143.27, 148.84, 163.08, 163.22, 165.60, 184.89; MS m/z [%]: 366 [(M + 2)+, 24.47], 364 [M+, 69.06], 143 [100]; Anal. calcd. For C20H17ClN4O: C, 65.84; H, 4.70; N, 15.36; Found C, 66.09; H, 4.74; N, 15.53.
5-Bromo-3-(((1-propyl-1H-indol-3-yl)methylene)hydrazono)indolin-2-one (6k)
Yield 83%, m.p. 126–128 °C; IR (KBr, ν cm−1): 3381 (NH), 1697 (C=O); 1H NMR (DMSO-d6) δ (ppm): 0.88 (t, 3H, –CH2–CH2–CH3, J = 7.2 Hz), 1.84 (sextet, 2H, –CH2–CH2–CH3, J = 7.2 Hz), 4.27 (t, 2H, –CH2–CH2–CH3, J = 7.2 Hz), 6.86 (d, 1H, Ar-H, J = 8.0 Hz), 7.31–7.36 (m, 2H, Ar-H), 7.54 (d, 1H, Ar-H, J = 8.0 Hz), 7.69 (d, 1H, Ar-H, J = 8.0 Hz), 8.29–8.31 (m, 2H, -CH=N- and Ar-H), 8.66 (s, 1H, Ar-H), 8.97 (s, 1H, H-2 indole), 10.87 (s, 1H, NH isatin, D2O exchangeable); 13C NMR (DMSO-d6) δ ppm: 11.05 (–CH2–CH2–CH3), 23.22 (–CH2–CH2–CH3), 48.32 (–CH2–CH2–CH3), 111.82, 111.90, 112.98, 113.69, 119.22, 121.89, 122.61, 124.17, 125.32, 130.42, 135.12, 138.15, 140.32, 143.59, 148.77, 163.41, 165.44 (C=O); Anal. calcd. For C20H17BrN4O: C, 58.69; H, 4.19; N, 13.69; Found C, 58.95; H, 4.26; N, 14.02.
5-Methoxy-3-(((1-propyl-1H-indol-3-yl)methylene)hydrazono)indolin-2-one (6l)
Yield 80%, m.p. 122–124 °C; IR (KBr, ν cm−1): 3361 (NH), 1696 (C=O); 1H NMR (DMSO-d6) δ (ppm): 0.86 (t, 3H, –CH2–CH2–CH3, J = 7.2 Hz), 1.81 (sextet, 2H, –CH2–CH2–CH3, J = 7.2 Hz), 3.78 (s, 3H, OCH3), 4.24 (t, 2H, –CH2–CH2–CH3, J = 7.2 Hz), 6.81 (d, 1H, Ar-H, J = 8.0 Hz), 6.94 (d, 1H, Ar-H, J = 8.0 Hz), 7.22 (t, 1H, Ar-H, J = 8.0 Hz), 7.35 (t, 1H, Ar-H, J = 8.0 Hz), 7.55 (d, 1H, Ar-H, J = 8.4 Hz), 8.09 (s, 1H, Ar-H), 8.22 (s, 1H, -CH=N-), 8.33 (d, 1H, Ar-H, J = 8.4 Hz), 8.97 (s, 1H, H-2 indole), 10.81 (s, 1H, NH isatin, D2O exchangeable); 13C NMR (DMSO-d6) δ ppm: 11.51 (–CH2–CH2–CH3), 23.25 (–CH2–CH2–CH3), 48.27 (–CH2–CH2–CH3), 55.96 (OCH3), 111.76, 111.80 (2C), 112.74, 117.90, 119.83, 122.11, 122.32, 124.03, 125.43, 138.06, 138.51, 139.53, 150.07, 155.07, 162.32, 166.00; Anal. calcd. For C21H20N4O2: C, 69.98; H, 5.59; N, 15.55; Found C, 76.21; H, 5.66; N, 15.80.
5-Fluoro-3-(((1-propyl-1H-indol-3-yl)methylene)hydrazono)indolin-2-one (6m)
Yield 75%, m.p. 135–137 °C; IR (KBr, ν cm−1): 3420 (NH), 1702 (C=O); 1H NMR (DMSO-d6) δ (ppm): 0.86 (t, 3H, –CH2–CH2–CH3, J = 7.6 Hz), 1.83 (sextet, 2H, –CH2–CH2–CH3, J = 7.2 Hz), 4.23 (t, 2H, –CH2–CH2–CH3, J = 7.2 Hz), 6.98–7.02 (m, 1H, Ar-H), 7.31–7.36 (m, 2H, Ar-H), 7.42 (dd, 1H, Ar-H, J = 8.0, 2.4 Hz), 7.59 (d, 1H, Ar-H, J = 8.0 Hz), 8.27–8.30 (m, 2H, -CH=N- and Ar-H), 8.45 (d, 1H, Ar-H, J = 2.4 Hz), 8.96 (s, 1H, H-2 indole), 10.83 (s, 1H, NH isatin, D2O exchangeable); MS m/z [%]: 348 [M+, 25.60], 143 [100]; 13C NMR (DMSO-d6) δ ppm: 11.49 (–CH2–CH2–CH3), 23.26 (–CH2–CH2–CH3), 48.25 (–CH2–CH2–CH3), 111.13, 111.41, 112.55, 113.96, 117.90, 119.08, 121.47, 122.35, 124.03, 125.52, 132.78, 136.62, 138.97, 143.07, 149.21, 161.83, 165.90; Anal. calcd. For C20H17FN4O: C, 68.95; H, 4.92; N, 16.08; Found C, 69.18; H, 4.97; N, 16.24.
7-Fluoro-3-(((1-propyl-1H-indol-3-yl)methylene)hydrazono)indolin-2-one (6n)
Yield 79%, m.p. 129–131 °C; IR (KBr, ν cm−1): 3418 (NH), 1705 (C=O); 1H NMR (DMSO-d6) δ (ppm): 0.85 (t, 3H, –CH2–CH2–CH3, J = 7.6 Hz), 1.81 (sextet, 2H, –CH2–CH2–CH3, J = 7.2 Hz), 4.24 (t, 2H, –CH2–CH2–CH3, J = 7.2 Hz), 7.09–7.12 (m, 1H, Ar-H), 7.29–7.376 (m, 3H, Ar-H), 7.67–7.69 (m, 1H, Ar-H), 8.23–8.30 (m, 3H, –CH=N– and 2 Ar-H), 8.95 (s, 1H, H-2 indole), 11.27 (s, 1H, NH isatin, D2O exchangeable); 13C NMR (DMSO-d6) δ ppm: 11.52 (–CH2–CH2–CH3), 23.29 (–CH2–CH2–CH3), 48.23 (–CH2–CH2–CH3), 111.13, 111.41, 112.98, 116.87, 119.16, 121.23, 123.85, 125.48, 127.04, 131.23, 135.47, 138.56, 142.61, 147.08, 152.32, 162.72, 166.04; Anal. calcd. For C20H17FN4O: C, 68.95; H, 4.92; N, 16.08; Found C, 69.12; H, 4.87; N, 16.31.
3-(((1-Benzyl-1H-indol-3-yl)methylene)hydrazono)indolin-2-one (6o)
Yield 75%, m.p. 261–263 °C; IR (KBr, ν cm−1): 3422 (NH), 1697 (C=O); 1H NMR (DMSO-d6) δ (ppm): 5.55 (s, 2H, benzylic CH2), 6.88 (d, 1H, Ar-H, J = 8.0 Hz), 7.04 (t, 1H, Ar-H, J = 7.2 Hz), 7.27–7.38 (m, 8H, Ar-H), 7.61 (dd, 1H, Ar-H, J = 6.8, 2.0 Hz), 8.30 (dd, 1H, Ar-H, J = 6.8, 2.0 Hz), 8.34 (d, 1H, Ar-H, J = 8.0 Hz), 8.39 (s, 1H, -CH=N-), 8.94 (s, 1H, H-2 indole), 10.74 (s, 1H, NH isatin, D2O exchangeable); 13C NMR (DMSO-d6) δ ppm: 50.21 (benzylic CH2), 110.41, 111.07, 112.04, 112.15, 117.53, 117.90, 121.80, 122.50, 122.75, 124.06, 127.48, 127.77, 128.16, 129.19, 133.26, 137.40, 137.88, 139.15, 144.77, 149.75, 161.51, 163.22, 165.77 (C=O); Anal. calcd. For C24H18N4O: C, 76.17; H, 4.79; N, 14.81; Found C, 76.29; H, 4.87; N, 14.04.
3-(((1-Benzyl-1H-indol-3-yl)methylene)hydrazono)-5-chloroindolin-2-one (6p)
Yield 80%, m.p. 218–220 °C; IR (KBr, ν cm−1): 3395 (NH), 1703 (C=O); 1H NMR (DMSO-d6) δ (ppm): 5.57 (s, 2H, benzylic CH2), 6.90 (d, 1H, Ar-H, J = 8.4 Hz), 7.14 (dd, 1H, Ar-H, J = 8.4, 2.0 Hz), 7.27–7.36 (m, 6H, Ar-H), 7.42 (dd, 1H, Ar-H, J = 8.4, 2.0 Hz), 7.64–7.66 (m, 1H, Ar-H), 8.29–8.31 (m, 1H, Ar-H), 8.42 (s, 1H, -CH=N-), 8.46 (d, 1H, Ar-H, J = 2.0 Hz), 8.99 (s, 1H, H-2 indole), 10.88 (s, 1H, NH isatin, D2O exchangeable); 13C NMR (DMSO-d6) δ ppm: 49.61, 50.31, 111.19, 111.29, 111.85, 112.25, 112.30, 112.53, 117.41, 118.03, 118.70, 119.67, 120.19, 121.92, 122.47, 122.71, 123.71, 124.30, 124.54, 125.52, 125.57, 126.10, 126.13, 126.34, 126.38, 126.81, 127.59, 127.65, 127.71, 127.82, 128.27, 128.50, 129.00, 129.22, 132.48, 136.74, 137.27, 137.62, 138.05, 138.36, 138.42, 140.14, 143.35, 149.06, 163.02, 163.08, 163.19, 165.57; Anal. calcd. For C24H17ClN4O: C, 69.82; H, 4.15; N, 13.57; Found C, 70.01; H, 4.21; N, 13.74.
3-(((1-Benzyl-1H-indol-3-yl)methylene)hydrazono)-5-bromoindolin-2-one (6q)
Yield 78%, m.p. 210–213 °C; IR (KBr, ν cm−1): 3428 (NH), 1705 (C=O); 1H NMR (DMSO-d6) δ (ppm): 5.56 (s, 2H, benzylic CH2), 6.81 (d, 1H, Ar-H, J = 8.0 Hz), 7.31–7.42 (m, 8H, Ar-H), 7.55 (d, 1H, Ar-H, J = 8.0 Hz), 7.65 (d, 1H, Ar-H, J = 7.6 Hz), 8.29–8.31 (m, 1H, Ar-H), 8.42 (s, 1H, -CH=N-), 8.64 (s, 1H, Ar-H), 9.00 (s, 1H, H-2 indole), 10.88 (s, 1H, NH isatin, D2O exchangeable); MS m/z [%]: 459 [(M + 2)+, 5.93], 457 [M+, 6.83], 116 [100]; 13C NMR (DMSO-d6) δ ppm: 50.32, 112.36, 113.03, 113.82, 120.17, 121.99, 122.77, 124.33, 125.00, 125.36, 127.84, 128.30, 129.24, 129.61, 130.52, 135.23, 137.28, 138.01, 140.32, 143.71, 149.03, 162.94, 163.22, 165.46; Anal. calcd. For C24H17BrN4O: C, 63.03; H, 3.75; N, 12.25; Found C, 63.26; H, 3.78; N, 12.51.
3-(((1-Benzyl-1H-indol-3-yl)methylene)hydrazono)-5-methoxyindolin-2-one (6r)
Yield 76%, m.p. 222–224 °C; IR (KBr, ν cm−1): 3408 (NH), 1705 (C=O); 1H NMR (DMSO-d6) δ (ppm): 3.75 (s, 3H, OCH3), 5.55 (s, 2H, benzylic CH2), 6.80 (d, 1H, Ar-H, J = 8.4 Hz), 6.95 (dd, 1H, Ar-H, J = 8.4, 2.4 Hz), 7.24–7.34 (m, 7H, Ar-H), 7.63 (d, 1H, Ar-H, J = 8.0 Hz), 8.03 (d, 1H, Ar-H, J = 2.8 Hz), 8.37–8.39 (m, 2H, –CH=N– and Ar-H), 8.95 (s, 1H, H-2 indole), 10.54 (s, 1H, NH isatin, D2O exchangeable); 13C NMR (DMSO-d6) δ ppm: 50.21 (benzylic CH2), 55.94 (–OCH3), 111.97, 112.12, 112.22, 112.73, 117.81, 119.92, 122.10, 122.46, 124.17, 125.55, 127.81 (2C), 128.24, 129.20 (2C), 137.36, 137.94, 138.54, 139.61, 150.21, 155.04, 162.12, 165.91 (C=O); Anal. calcd. For C25H20N4O2: C, 73.51; H, 4.94; N, 13.72; Found C, 73.84; H, 4.98; N, 13.96.
Synthesis of N-substituted-indoline-2,3-dione 7a–f
Compounds 7a–f were prepared according to the literature procedureCitation19,Citation20.
Synthesis of N-substituted-3-hydrazonoindolin-2-ones 8a–f
Following the same procedures descried above for the preparation of hydrazones 4a–f.
Compounds 8a, e, fCitation20 and 8cCitation21 are previously reported.
5-Chloro-3-hydrazono-1-methylindolin-2-on (8b)
Yield 65%, m.p. 159–161 °C; IR (KBr, ν cm−1): 3304 (NH2), 1698 (C=O); 1H NMR (DMSO-d6) δ (ppm): 3.12, 3.16 (2 s, 3H, N-CH3), 6.97, 7.01 (2d, 1H, H-7 isatin, J = 8.4 Hz), 7.21 (dd, 1H, H-6 isatin, J = 8.4, 2.4 Hz), 7.31, 8.06 (2d, 1H, H-4 isatin, J = 2.4 Hz), 9.87, 10.57 (2d, 2H, NH2, D2O exchangeable, J = 14.4 Hz); 13C NMR (DMSO-d6) δ ppm: 25.69 (N-CH3), 26.13 (N-CH3), 109.87, 110.58, 117.13, 117.64, 122.31, 123.56, 124.61, 126.25, 126.44, 126.63, 126.67, 128.16, 138.94, 140.67, 161.09, 164.68 (C=O); Anal. calcd. For C9H8ClN3O: C, 51.57; H, 3.85; N, 20.05; Found C, 51.69; H, 3.87; N, 19.93.
5-Chloro-3-hydrazono-1-propylindolin-2-one (8d)
Yield 72%, m.p. 133–135 °C; IR (KBr, ν cm−1): 3310 (NH2), 1703 (C=O); 1H NMR (DMSO-d6) δ (ppm): 0.80 (t, 3H, -CH2-CH2-CH3, J = 7.2 Hz), 1.51 (sextet, 2H, -CH2-CH2-CH3, J = 7.2 Hz), 3.60 (t, 2H, -CH2-CH2-CH3, J = 7.2 Hz), 7.05 (d, 1H, H-7 isatin, J = 8.4 Hz), 7.18 (dd, 1H, H-6 isatin, J = 8.4, 2.0 Hz), 7.32 (d, 1H, H-4 isatin, J = 2.0 Hz), 9.89, 10.61 (2d, 2H, NH2, D2O exchangeable, J = 14.4 Hz); 13C NMR (DMSO-d6) δ ppm: 11.55, 11.57 (–CH2–CH2–CH3), 21.00, 21.09 (–CH2–CH2–CH3), 40.65, 40.91 (–CH2–CH2–CH3), 110.03, 110.74, 117.24, 117.74, 122.45, 123.66, 124.53, 126.12, 126.34, 126.52, 126.64, 128.11, 138.25, 140.01, 161.07 (C=O), 164.66 (C=O); Anal. calcd. For C11H12ClN3O: C, 55.59; H, 5.09; N, 17.68; Found C, 55.43; H, 5.14; N, 17.80.
General procedure for the synthesis of target compounds 9a–f
Following the same procedures descried above for the preparation of compounds 6a–r.
3-(((1H-indol-3-yl)methylene)hydrazono)-1-methylindolin-2-one (9a)
Yield 70%, m.p. 283–285 °C; IR (KBr, ν cm−1): 3410 (NH), 1698 (C=O); 1H NMR (DMSO-d6) δ (ppm): 3.19 (s, 3H, N-CH3), 7.08 (d, 1H, Ar-H, J = 8.0 Hz), 7.13 (t, 1H, Ar-H, J = 7.6 Hz), 7.28–7.33 (m, 2H, Ar-H), 7.44 (t, 1H, Ar-H, J = 8.0 Hz), 7.52–7.55 (m, 1H, Ar-H), 8.22 (s, 1H, -CH=N-), 8.28–8.30 (m, 1H, Ar-H), 8.42 (d, 1H, Ar-H, J = 7.2 Hz), 8.97 (s, 1H, H-2 indole), 12.17 (s, 1H, NH indole, D2O exchangeable); 13C NMR (DMSO-d6) δ ppm: 26.41 (N-CH3), 109.68, 112.66, 113.15, 116.88, 121.70, 122.42, 123.00, 123.93, 124.95, 127.79, 133.07, 137.06, 138.04, 145.75, 148.89, 162.72, 164.41 (C=O); MS m/z [%]: 302 [M+, 20.95], 273 [100]; Anal. calcd. For C18H14N4O: C, 71.51; H, 4.67; N, 18.53; Found C, 71.67; H, 4.72; N, 18.79.
3-(((1H-indol-3-yl)methylene)hydrazono)-5-chloro-1-methylindolin-2-one (9b)
Yield 65%, m.p. > 300 °C; IR (KBr, ν cm−1): 3387 (NH), 1697 (C=O); 1H NMR (DMSO-d6) δ (ppm): 3.19 (s, 3H, N-CH3), 7.11 (d, 1H, Ar-H, J = 8.4 Hz), 7.26–7.33 (m, 2H, Ar-H), 7.51 (dd, 1H, Ar-H, J = 8.4, 2.4 Hz), 7.55 (d, 1H, Ar-H, J = 7.6 Hz), 8.27–8.28 (m, 2H, -CH=N- and Ar-H), 8.53 (d, 1H, Ar-H, J = 2.0 Hz), 9.01 (s, 1H, H-2 indole), 12.25 (s, 1H, NH indole, D2O exchangeable); MS m/z [%]:438 [(M + 2)+, 8.1], 336 [M+, 21.32], 116 [100]; 13C NMR (DMSO-d6) δ ppm: 26.63, 111.28, 112.85, 113.47, 118.68, 121.85, 122.43, 124.30, 124.91, 125.13, 127.44, 132.24, 138.26 (2C), 144.57, 147.93, 164.13, 164.15; Anal. calcd. For C18H13ClN4O: C, 64.20; H, 3.89; N, 16.64; Found C, 64.43; H, 3.94; N, 16.87.
3-(((1H-indol-3-yl)methylene)hydrazono)-1-propylindolin-2-one (9c)
Yield 64%, m.p. 274–275 °C; IR (KBr, ν cm−1): 3438 (NH), 1704 (C=O); 1H NMR (DMSO-d6) δ (ppm): 0.87 (t, 3H, –CH2–CH2–CH3, J = 7.2 Hz), 1.59 (sextet, 2H, –CH2–CH2–CH3, J = 7.2 Hz), 3.68 (t, 2H, –CH2–CH2–CH3, J = 7.2 Hz), 7.12–7.15 (m, 2H, Ar-H), 7.28–7.33 (m, 2H, Ar-H), 7.42 (t, 1H, Ar-H, J = 7.6 Hz), 7.52–7.55 (m, 1H, Ar-H), 8.23 (s, 1H, -CH=N-), 8.28–8.30 (m, 1H, Ar-H), 8.43 (d, 1H, Ar-H, J = 7.2 Hz), 8.96 (s, 1H, H-2 indole), 12.17 (s, 1H, NH indole, D2O exchangeable); 13C NMR (DMSO-d6) δ ppm: 11.61 (–CH2–CH2–CH3), 20.86 (–CH2–CH2–CH3), 41.25 (–CH2–CH2–CH3), 109.87, 112.68, 113.16, 116.93, 121.70, 122.43, 122.88, 123.94, 124.95, 127.97, 133.09, 137.08, 138.05, 145.13, 148.77, 162.66, 164.41 (C=O); MS m/z [%]: 330 [M+, 12.07], 273 [100]; Anal. calcd. For C20H18N4O: C, 72.71; H, 5.49; N, 16.96; Found C, 72.98; H, 5.53; N, 17.23.
3-(((1H-indol-3-yl)methylene)hydrazono)-5-chloro-1-propylindolin-2-one (9d)
Yield 75%, m.p. 295–297 °C; IR (KBr, ν cm−1): 3415 (NH), 1702 (C=O); 1H NMR (DMSO-d6) δ (ppm): 0.86 (t, 3H, –CH2–CH2–CH3, J = 7.2 Hz), 1.57 (sextet, 2H, –CH2–CH2–CH3, J = 7.2 Hz), 3.68 (t, 2H, –CH2–CH2–CH3, J = 7.2 Hz), 7.18 (d, 1H, Ar-H, J = 8.4 Hz), 7.26–7.34 (m, 2H, Ar-H), 7.50 (dd, 1H, Ar-H, J = 8.4, 2.0 Hz), 7.56 (d, 1H, Ar-H, J = 8.4 Hz), 8.27–8.29 (m, 2H, -CH=N- and Ar-H), 8.55 (d, 1H, Ar-H, J = 2.4 Hz), 9.02 (s, 1H, H-2 indole), 12.26 (s, 1H, NH indole, D2O exchangeable); 13C NMR (DMSO-d6) δ ppm: 11.61, 20.83, 41.47, 111.46, 112.82, 113.43, 118.12, 121.63, 122.40, 124.20, 124.87, 126.61, 126.93, 127.52, 132.25, 138.23, 143.76, 148.31, 164.07, 164.08; Anal. calcd. For C20H17ClN4O: C, 65.84; H, 4.70; N, 15.36; Found C, 66.01; H, 4.78; N, 15.49.
3-(((1H-indol-3-yl)methylene)hydrazono)-1-benzylindolin-2-one (9e)
Yield 70%, m.p. 243–245 °C; IR (KBr, ν cm−1): 3419 (NH), 1702 (C=O); 1H NMR (DMSO-d6) δ (ppm): 4.98 (s, 2H, benzylic CH2), 6.99 (d, 1H, Ar-H, J = 8.0 Hz), 7.11 (t, 1H, Ar-H, J = 7.6 Hz), 7.24–7.39 (m, 8H, Ar-H), 7.54–7.56 (m, 1H, Ar-H), 8.23 (s, 1H, -CH=N-), 8.28–8.30 (m, 1H, Ar-H), 8.45 (d, 1H, Ar-H, J = 7.2 Hz), 9.00 (s, 1H, H-2 indole), 12.19 (s, 1H, NH indole, D2O exchangeable); 13C NMR (DMSO-d6) δ ppm: 43.12 (benzylic CH2), 110.27, 112.71, 113.19, 117.10, 121.72, 122.48, 123.19, 123.97, 124.97, 127.69 (2C), 127.92, 127.98, 129.17 (2C), 132.97, 136.73, 137.29, 138.08, 144.69, 148.59, 162.94, 164.58 (C=O); MS m/z [%]: 378 [M+, 5.01] 91 [100]; Anal. calcd. For C24H18N4O: C, 76.17; H, 4.79; N, 14.81; Found C, 76.44; H, 4.85; N, 15.06.
3-(((1H-indol-3-yl)methylene)hydrazono)-1-benzyl-5-chloroindolin-2-one (9f)
Yield 73%, m.p. 264–266 °C; IR (KBr, ν cm−1): 3380 (NH), 1698 (C=O); 1H NMR (DMSO-d6) δ (ppm): 4.98 (s, 2H, benzylic CH2), 7.01 (d, 1H, Ar-H, J = 8.4 Hz), 7.24–7.34 (m, 7H, Ar-H), 7.44 (dd, 1H, Ar-H, J = 8.4, 2.0 Hz), 7.56 (d, 1H, Ar-H, J = 7.6 Hz), 8.28–8.29 (m, 2H, -CH=N- and Ar-H), 8.57 (d, 1H, Ar-H, J = 2.0 Hz), 9.05 (s, 1H, H-2 indole), 12.28 (s, 1H, NH indole, D2O exchangeable); 13C NMR (DMSO-d6) δ ppm: 43.24 (benzylic CH2), 111.73, 112.83, 113.42, 118.26, 121.61, 122.42, 124.21, 124.83, 126.93, 127.51 (2C), 127.66, 127.98, 129.19 (2C), 132.14, 136.43, 138.20, 138.34, 143.23, 147.79, 164.31, 164.32 (C=O); MS m/z [%]: 414 [(M + 2)+, 1.41], 412 [M+, 3.85], 91 [100]; Anal. calcd. For C24H17ClN4O: C, 69.82; H, 4.15; N, 13.57; Found C, 70.04; H, 4.18; N, 13.81
Synthesis of 3-(hydrazonomethyl)-1-propyl-1H-indole 10
To a stirred solution of 1-propyl-1H-indole-3-carbaldehyde 5b (1.9 g, 10 mmol) in ethanol (15 ml), 99% hydrazine hydrate (2.5 ml, 50 mmol) was added. The reaction mixture was refluxed for 2 h, and then the formed precipitate upon cooling was filtered off, washed with water, dried and recrystallised from methanol to afford hydrazone 10. Yield 68%, m.p. 127–129 °C; IR (KBr, ν cm−1): 3294 (NH2); 1H NMR (DMSO-d6) δ (ppm): 0.83 (t, 3H, –CH2–CH2–CH3, J = 7.6 Hz), 1.75 (sextet, 2H, –CH2–CH2–CH3, J = 7.6 Hz), 4.13 (t, 2H, –CH2–CH2–CH3, J = 7.2 Hz), 7.17–7.27 (m, 2H, Ar-H), 7.54 (d, 1H, Ar-H, J = 8.4 Hz), 7.92 (s, 1H, -CH=N-), 8.33 (d, 1H, Ar-H, J = 8.0 Hz), 8.85 (s, 1H, H-2 indole); 13C NMR (DMSO-d6) δ ppm: 11.56 (–CH2–CH2–CH3), 23.40 (–CH2–CH2–CH3), 47.86 (-CH2-CH2-CH3), 110.90, 111.60, 121.18, 122.75, 123.10, 125.70, 134.89, 137.55, 155.08; Anal. calcd. For C12H15N3: C, 71.61; H, 7.51; N, 20.88; Found C, 71.82; H, 7.43; N, 20.72.
5.1.10. General procedure for the synthesis of target compounds 11a–c
Following the same procedures descried above for the preparation of compounds 6a–r.
1-Methyl-3-(((1-propyl-1H-indol-3-yl)methylene)hydrazono)indolin-2-one (11a)
Yield 70%, m.p. 124–126 °C; IR (KBr, ν cm−1): 1711 (C=O); 1H NMR (DMSO-d6) δ (ppm): 0.84 (t, 3H, –CH2–CH2–CH3, J = 6.8 Hz), 1.80 (sextet, 2H, –CH2–CH2–CH3, J = 6.8 Hz), 3.19 (s, 3H, N-CH3), 4.24 (t, 2H, –CH2–CH2–CH3, J = 6.8 Hz), 7.08 (d, 1H, Ar-H, J = 8.0 Hz), 7.12 (t, 1H, Ar-H, J = 8.0 Hz), 7.32–7.36 (m, 1H, Ar-H), 7.44 (dt, 1H, Ar-H, J = 8.0, 1.0 Hz), 7.54 (d, 1H, Ar-H, J = 8.0 Hz), 7.66–7.68 (m, 1H, Ar-H), 8.27–8.34 (m, 2H, -CH=N- and Ar-H), 8.40 (d, 1H, Ar-H, J = 7.2 Hz), 8.95 (s, 1H, H-2 indole); MS m/z [%]: 344 [M+, 29.71], 143 [100]; 13C NMR (DMSO-d6) δ ppm: 11.52, 23.28, 26.43, 48.28, 109.69, 111.70, 111.77, 116.90, 121.94, 122.69, 123.01, 123.95, 125.58, 127.83, 133.09, 138.03, 139.27, 145.79, 148.92, 162.24, 164.43; Anal. calcd. For C21H20N4O: C, 73.23; H, 5.85; N, 16.27; Found C, 72.98; H, 5.94; N, 16.23.
1-Propyl-3-(((1-propyl-1H-indol-3-yl)methylene)hydrazono)indolin-2-one (11b)
Yield 58%, m.p. 96–98 °C; IR (KBr, ν cm−1): 1706 (C=O); 1H NMR (DMSO-d6) δ (ppm): 0.84 (t, 3H, –CH2–CH2–CH3, J = 7.2 Hz), 0.88 (t, 3H, –CH2–CH2–CH3, J = 7.2 Hz), 1.61 (sextet, 2H, –CH2–CH2–CH3, J = 7.2 Hz), 1.83 (sextet, 2H, –CH2–CH2–CH3, J = 7.2 Hz), 3.72 (t, 2H, –CH2–CH2–CH3, J = 7.2 Hz), 4.20 (t, 2H, –CH2–CH2–CH3, J = 7.2 Hz), 6.91 (d, 1H, Ar-H, J = 8.0 Hz), 7.15–7.19 (m, 2H, Ar-H), 7.37–7.52 (m, 3H, Ar-H), 8.25 (s, 1H, -CH=N-), 8.29–8.31 (m, 1H, Ar-H), 8.39 (d, 1H, Ar-H, J = 7.6 Hz), 8.94 (s, 1H, H-2 indole); 13C NMR (DMSO-d6) δ ppm: 11.63, 11.65, 19.02, 20.68, 41.47, 41.66, 116.94, 117.97, 118.01, 119.38, 119.76, 123.22, 126.24, 127.31, 133.87, 137.40, 137.92, 143.26, 144.35, 146.45, 152.76, 153.09, 162.52; MS m/z [%]: 372 [M+, 24.94], 315 [81.21], 143 [100]; Anal. calcd. For C23H24N4O: C, 74.17; H, 6.49; N, 15.04; Found C, 74.38; H, 6.56; N, 15.29.
1-Benzyl-3-(((1-propyl-1H-indol-3-yl)methylene)hydrazono)indolin-2-one (11c)
Yield 65%, m.p. 143–145 °C; IR (KBr, ν cm−1): 1710 (C=O); 1H NMR (DMSO-d6) δ (ppm): 0.86 (t, 3H, –CH2–CH2–CH3, J = 7.2 Hz), 1.82 (sextet, 2H, –CH2–CH2–CH3, J = 7.2 Hz), 4.25 (t, 2H, –CH2–CH2–CH3, J = 7.2 Hz), 4.98 (s, 2H, benzylic CH2), 6.99 (d, 1H, Ar-H, J = 7.6 Hz), 7.11 (t, 1H, Ar-H, J = 7.6 Hz), 7.24–7.39 (m, 8H, Ar-H), 7.67–7.69 (m, 1H, Ar-H), 8.29–8.31 (m, 2H, -CH=N- and Ar-H), 8.44 (d, 1H, Ar-H, J = 7.2 Hz), 8.98 (s, 1H, H-2 indole); 13C NMR (DMSO-d6) δ ppm: 11.54, 26.72, 43.17, 48.32, 112.65, 114.00, 114.97, 117.33, 118.01, 118.43, 119.41, 119.72, 123.16, 124.65, 127.31, 129.19, 135.95, 136.65, 137.39, 138.52, 143.26, 144.70, 147.38, 152.74, 153.07, 162.43, 164.61; MS m/z [%]: 420 [M+, 8.91], 91 [100]; Anal. calcd. For C27H24N4O: C, 77.12; H, 5.75; N, 13.32; Found C, 77.40; H, 5.79; N, 13.48.
Biological evaluation
Assessment of in vitro cytotoxic activity
HepG-2, HCT-116 and MCF-7 cancer cell lines were obtained from VACSERA Tissue Culture Unit. The cells were propagated in DMEM supplemented with 10% heat-inactivated foetal bovine serum, 1% L-glutamine, HEPES buffer and 50 µg/ml gentamycin. All cells were maintained at 37 °C in a humidified atmosphere with 5% CO2 and were subcultured two times a week. Cytotoxicity was determined following a reported procedureCitation22. The relation between surviving cells and drug concentration is plotted to get the survival curve of each tumour cell line after treatment with the specified compound. The 50% inhibitory concentration (IC50) was estimated from graphic plots of the dose response curve for each conc. using Graphpad Prism software (San Diego, CA). The data presented are the mean of at least three separate experiments.
In vitro anti-proliferative activity by NCI-USA
The anticancer assays were performed in accordance with the protocol of the Drug Evaluation Branch, NCI, Bethesda, MDCitation23–25. A 48 h drug exposure protocol was used and sulphorhodamine B (SRB) protein assayCitation26 was applied to estimate the cell viability and growth, as reported earlierCitation27,Citation28.
DNA-flow cytometry analysis
To determine the effect of compound 6n on the cell cycle distribution MCF-7 cell line; cell cycle analysis was performed using the CycleTEST™ PLUS DNA Reagent Kit (Becton Dickinson Immunocytometry Systems, San Jose, CA). Control cells with known DNA content (PBMCs) were used as a reference point for determining the DI (DNA Index) for the test samples. The cells were stained with propodium iodide stain following the procedure provided by the kit and then run on the DNA cytometer. Cell-cycle distribution was calculated using CELLQUEST software (Becton Dickinson Immunocytometry Systems, San Jose, CA).
Annexin V-FITC apoptosis assay
Apoptotic cells were further analysed by Annexin V-FITC/DAPI assay (Cayman Chemical, Ann Arbor, MI). Briefly, MCF-7 cells were cultured to a monolayer then treated with compound 6n at the IC50 concentration (1.04 µM) as described earlier. Cells were then harvested through trypsinisation, and rinsed twice in PBS followed by binding buffer. Moreover, cells were re-suspended in 100 lL of binding buffer with the addition of 1 lL of FITC-Annexin V (Becton Dickinson BD PharmingenTM, Heidelberg, Germany) followed by an incubation period of 30 min at 4 °C. Cells were then rinsed in binding buffer and re-suspended in 150 lL of binding buffer with the addition of 1 lL of DAPI (1 lg/lL in PBS) (Invitrogen, Life Technologies, Darmstadt, Germany). Cells were then analysed using the flow cytometer BD FACS Canto II (BD Biosciences, San Jose, CA) and the results were interpreted with FlowJo7.6.4 software (Tree Star, FlowJo LLC, Ashland, OR)
RNA extraction, real-time PCR analysis and quantification of gene expression
The gene expression of caspase3, caspase8, caspase 9, Bax, Bcl-2 and cytochrome-C was assessed by total mRNA extraction from cells using RNeasy Mini Kit®, Up to 1 × 107 cells, depending on the cell line, are disrupted in Buffer RLT and homogenised & disrupted, Ethanol is then added to the lysate, creating conditions that promote selective binding of RNA to the RNeasy membrane. The sample is then applied to the RNeasy Mini spin column. Total RNA binds to the membrane, contaminants are efficiently was headway, and high-quality RNA is eluted in RNase-free water. Primer sequences for each gene were as follows: caspase-3 forward primer 5′-TGCATACTCCACAGCACCTGGTTA-3′ and reverse primer 5′-CATGGCACAAAGCGACTGGATGAA-3′; Caspase-8 forward primer 5′-TTTCACTGTGTTAGCCAGGGTGGTA-3′ and reverse primer 5′-CCTGTAATCCCAGCACTTTGGGAG-3′; Caspase-9 forward primer 5′-TCAGTGACGTCTGTGTTCAGGAGA-3′ and reverse primer 5′-TTGTTGATGATGAGGCAG TAGCCG-3′; Bcl-2 forward primer 5′-ATGACCAGACACTGACCATCCACT-3′ and reverse primer 5′-ATGTAGTGGTTCTCCTGGTGGCAA-3; Bax forward primer 5′-TCTACTTTGCCAGCAAACTGGTGC-3′ and reverse primer 5′-TGTCCAGCCCATGATGGTTCTGAT-3′; Cytochrome-C forward primer 5′-AGCTGGAGACGTTGAGAAGG-3′ and reverse primer 5′-ATCTTCGTGCCAGGGATGTA-3′; GAPDH was used as reference housekeeping gene with forward primer 5′-TTCCAGGACCAAGATCCCTCCAAA-3′ and reverse primer 5′-ATGGTGGTGAAGACACCAGTGAAC-3′.
DPPH-free radical scavenging activities
The samples were tested for the antioxidant activity by measuring their radical scavenging ability that was assessed by the stable 2,2′-diphenyl-1-picrylhydrazyl free radical (DPPH; purchased from Sigma; St. Louis, MO) scavenging method as modified by ElSheikh et al.Citation29. In details, 150 µl (DMSO solution; 1000 µg/ml final concentration) of each sample (or ascorbic acid as a reference antioxidant) was added to 850 µl pure methanol and 10 two-fold dilutions were made from this stock solution to give good dose-response curve then 2 ml of freshly prepared 0.13 mM DPPH solution in methanol was added to each tube. For control sample, 850 µl methanol and 150 µl dimethyl sulphoxide (DMSO) were added to 2 ml DPPH solution The sample solutions were vigorously shaken on a vortex for 1 min. and then the absorbance was measured at 516 nm in a UV/VIS spectrophotometer (Spectronic Spectrometer, Milton Roy Ltd, Ivyland, PA) after 30 min. The radical scavenging ability (RSA) was calculated according to the following equation:
where Ac is the absorbance of control DPPH sample without antioxidant; As is the absorbance of tested sample after 30 min. The concentration required for 50% radical scavenging ability (IC50) was calculated from the graphic plots.
Oxidative stress parameters
Total protein assay in MCF-7 cells
The total proteins were assayed in treated and non-treated MCF-7 cells according to Lowry et al.Citation30. Briefly, a solution containing 0.01% cupric sulphate, 0.01% NaK Tartarate, 2% sodium carbonate and 0.1 N sodium hydroxide was added to each sample and to each tube containing BSA and water (for the standard curve of the assay). Samples were vortexed and then allowed to incubate for 10 min at RT. Then, Folin–Ciocalten phenol reagent was added to each reaction tube to a final concentration of 0.1 N. Samples were vortexed and then allowed to incubate for 30 min at RT. The absorbance of each sample tube was then read at 500 nm. A standard curve was constructed and each sample protein concentration was then calculated by interpolating within the range of values provided by the standard curve. All Lowry protein assay reagents were obtained from Sigma-Aldrich (St. Louis, MO).
Superoxide dismutase (SOD) activity
SOD activity was done according to Kakkar et al.Citation31. SOD assay is based on the spectrophotometric assessment of the inhibition of nitro blue tetrazolium-NADH and phenazine methosulphate (PMS)-mediated formazan formation. Absorbance was measured at 560 nm. About 50% inhibition of formazan formation under the assay condition in 1 min is taken as one unit of enzyme activity/minute.
Catalase (CAT)activity
CAT was assayed spectrophotometrically using the method of Aebi et al.Citation32. Assay is based on the principle of measurement of decomposition of H2O2 by CAT measured at 240 nm.
Glutathione peroxidase (GSHPx) activity
The method of Paglia and ValentineCitation33 was used to measure GPx activity. Cell pellets were homogenised in stock buffer containing 55 mM potassium phosphate, 1 mM sodium azide and 1 mM EDTA. GPx activity was measured by adding 0.25 mM H2O2 in the presence of 1 mM GSH, 1 EU/ml glutathione reductase (GR) and 0.2 mM NADPH and measuring the change in absorbance at 340 nm for 5 min. GSH is oxidised by H2O2 to GSSG which is recycled by GR in presence of NADPH. Data were normalised per mg protein as determined by the Lowry protein assay.
Malondialdehyde (MDA) assay
MDA is the last marker of the lipid peroxidation pathway. This assay is according to the repercussion of MDA with thiobarbituric acid (TBA) that forms the MDATBA adduct that can be quantified calorimetricallyCitation34. Briefly, cells were collected by centrifugation and sonicated in ice-cold potassium chloride (1.15%) and centrifuged for 10 min at 3000 rpm. The resulting supernatant (1 ml) was added to 2 ml of thiobarbituric acid (TBA) reagent (15% TCA, 0.7% TBA and 0.25 N HCl) and heated at 100 °C for 15 min in a boiling bath. The sample was then placed in cold and centrifuged at 1500 rpm for 10 min. Absorbance of the supernatant was measured at 535 nm.
Carbonyl protein content assay
Protein carbonyl (PC) is the marker of protein oxidation. The carbonyl was identified by measuring the PC residues using dinitrophenylhydrazine (DNPH). Absorbance of the samples was measured at 370 nmCitation35.
Results and discussion
Chemistry
The proposed synthetic routes to prepare the target compounds are shown in Schemes 1–3. The synthesis of the N-substituted-indole-3-carbaldehyde derivatives 5a–c was accomplished through formylation of 1H-indole 1 with phosphorus oxychloride and DMF to give 1H-indole-3-carbaldehyde 2, then alkylation of the latter by methyl/propyl/benzyl bromide in DMF in the presence of potassium carbonate. While, refluxing isatins 3a–d with hydrazine hydrate in methanol afforded the 3-hydrazonoindolin-2-ones 4a–d. The first series of the target compounds 6a–r was obtained in good yields (75–83%) through condensation of hydrazones 4a–d with 1H-indole-3-carbaldehyde 2 or N-substituted-indole-3-carbaldehydes 5a–c in absolute ethyl alcohol in the presence of a catalytic amount of glacial acetic acid (Scheme 1).
Scheme 1. Synthesis of target compounds 6a–r; Reagents and conditions: (i) dry DMF/POCl3/NaOH/H2O/2 h, (ii) alkyl (or benzyl) bromide/THF/NaH, r.t. 12 h, (iii) CH3OH/NH2NH2.H2O/reflux 1 h, (iv) EtOH/AcOH (catalytic)/reflux 3 h and (v) Hydrazones 4a–d/EtOH/AcOH (catalytic)/reflux 3 h.

We next synthesised another series of hybrids (9a–f) to assess the impact of N-alkylation of the isatin moiety. Reaction of isatins 3a,b with methyl/propyl/benzyl bromide were carried out in a refluxing dry acetonitrile in the presence of potassium carbonate to furnish N-alkylated isatins 7a–f, respectively, which subsequently condensed with hydrazine hydrate in methanol to afford the hydrazones 8a–f. The target compounds 9a–f were obtained (64–75% yields) by reacting the hydrazone intermediates 8a–f with H-indole-3-carbaldehyde 2 in a refluxing ethanol in the presence of a catalytic amount of glacial acetic acid (Scheme 2). Trials to increase the yield, by using only glacial acetic acid as a solvent, failed as it is reported that it will give another productCitation36.
Scheme 2. Synthesis of target compounds 9a–f; Reagents and conditions: (i) methyl, propyl or benzyl bromide, dry acetonitrile, K2CO3, reflux 3 h, (ii) CH3OH/NH2NH2.H2O/reflux 1 h and (iii) EtOH/AcOH (catalytic)/reflux 3 h.

Scheme 3. Synthesis of target compounds 11a–c; Reagents and conditions: (i) EtOH/NH2NH2.H2O/reflux 2 h and (ii) EtOH/AcOH (catalytic)/reflux 3 h.

As a part of the SAR study, an additional series of derivatives (11a–c) was designed. To prepare such set of analogues (11a–c), the intermediate 10 was condensed with the appropriate N-substituted isatin 7a,c,e in ethanol to furnish the target compounds 11a–c, respectively, with 58–70% yields (Scheme 3).
The structures of the all synthesised compounds were confirmed under the basis of spectral and elemental analyses which were in full agreement with the proposed structures.
Biological evaluation
In vitro anti-proliferative activity assay
Anti-proliferative activity towards MCF-7, HepG-2 and HCT-116 cell lines
Anti-proliferative activity of the synthesised compounds was proved by performing cytotoxic activity assay against three different cell lines (MCF-7, HepG-2 and HCT-116) as reportedCitation13. The results showed various strength of activity as shown in . Scrutinising the results reveals that compounds 6 of the first series, which is characterised by free NH of the isatin group and diverse N-substituents of indole moiety, illustrated their activity with respect to the substituent on NH of indole moiety. For example, compounds 6a–h, with –NH and –N–CH3 on indole group, displayed no or weak cytotoxic activity against all three cell lines as they all possess IC50 values exceeding 24.55 µM. Grafting an N-propyl group on –NH of indole moiety yielded compounds with variant activity. For instance, compounds 6j and 6k with 5-Cl and 5-Br substituent on the isatin group exhibited moderate cytotoxic activity against the three cell lines ranging from 12.74 µM up to 25.87 µM. Exceptionally, compound 6i with N-propyl indole group and unsubstituted isatin moiety and compound 6n with N-propyl indole group and substituted 7-F isatin moiety, displayed significant cytotoxic activity against the three cell lines with IC50 values of 3.67 µM and 8.7 µM, respectively, against HCT-116 cell line and IC50 values of 13 µM and 3.97 µM, respectively, against HepG-2 cell line. Compounds 6i and 6n with the N-propyl indole group and unsubstituted indolin-2-one moiety or unsubstituted indolin-2-one moiety with the 7-F group, displayed strong cytotoxic activity against MCF-7 (IC50 = 3.58 µM and 1.04 µM) as shown in . Fortunately, introduction of benzyl group on indole moiety was fruitful in compound 6q (HCT-116; IC50 = 5.99 µM, HepG-2; IC50 = 3.81 µM). On the other hand, all other derivatives with different substitutions on indolin-2-one moiety possessed weak or no activity.
Table 1. Antitumor activity of compounds 6a–u, 9a–f, and 11a–c against HCT-116, HepG2, and MCF-7 cancer cell lines.
Unfortunately, changing the N-substitution from indole moiety to isatin moiety did not display any cytotoxic activity as in compounds 9a–f revealed by their high IC50 values as shown in . This gives insight that N-propyl substitution of the indole moiety is advantageous to anti-proliferative activity over substitution of the indolin-2-one group. Accordingly, a third series was designed (11a–c), sparing the N-propyl indole scaffold and substituting the indolin-2-one group with different substituents. Unfortunately, none of derivatives 11a–c proved to be a potent cytotoxic compound as they possess IC50 values on the three cell lines exceeding 27.7 µM.
NCI, USA anti-proliferative assay of 6i, 6j, 6n and 6q towards 60 cell lines
The structures of the active synthesised compounds were submitted to the National Cancer Institute (NCI) Developmental Therapeutic Program (www.dtp.nci.nih.gov). Four compounds 6i, 6j, 6n and 6q were selected to be screened for their anticancer activity in vitro. The compounds were screened at one dose primary anticancer assay towards a panel of 60 cancer cell lines (concentration 10−5 M). The tumour cell lines were taken from nine different organs (blood, colon, lung, brain, skin, ovary, kidney, prostate and breast). The data reported as mean-graph of the percent growth of the treated cells, and presented as percentage growth inhibition (GI%) caused by the test compounds ().
Table 2. Percentage growth inhibition (GI %) of in vitro subpanel tumour cell lines at 10 μM concentration for compounds 6i, 6j, 6n, and 6q.
Close examination of the obtained results, GI% values in , suggested that compounds 6j and 6n (N-propyl-indole derivatives combined with substituted indolin-2-one) are the most active analogues in this assay, showing broad spectrum activity toward numerous cell lines that belong to different tumour subpanels. Both compounds 6j and 6n displayed mean inhibition =19%, and possessed anti-proliferative activity against 44 and 39 cell lines, respectively, representing all subpanels (GI; 11–76%). Compound 6n showed potent growth inhibitory effect over leukaemia CCRF-CEM, HL-60(TB), K-562, MOLT-4, SR and breast cancer MCF7, T-47D with inhibition percent of 66%, 56%, 65%, 52%, 76%, 46% and 50%, respectively. Whereas, compound 6j showed potent growth inhibitory effect over non-small cell lung cancer EKVX, ovarian cancer IGROV1, renal cancer UO-31 and prostate cancer PC-3 with inhibition percent of 63%, 50%, 47% and 46%, respectively. All the leukaemia (except RPMI-8226) and breast cancer (except BT-549) cell lines were sensitive to all the tested compounds 6i, 6j, 6n and 6q with GI% range of 16–76% and 11–50%, respectively.
The susceptible cell lines to the tested compounds 6i, 6j, 6n and 6q with GI % ≥ 25 are presented in . Only leukaemia K-562, MOLT-4 and renal UO-31 cell lines were susceptible to all the tested compounds, with GI % ≥ 25. While compound 6q showed potent growth inhibition against ovarian cancer OVCAR-5 and renal cancer A498 (GI % = 72 and 85, respectively), it exerted lethal activity over breast cancer MDA-MB-468 with GI % = 143.
Cell-cycle analysis and apoptotic study
Compound 6n displayed a significant cytotoxic activity against MCF-7 cancer cell lines. Consequently, more biological studies were performed to explore its mechanistic study inside this cancer cell line. Cell-cycle analysis and apoptotic cell markers would be taken into consideration during this investigative study.
Cell-cycle analysis
Anti-proliferative agents abort cell growth by arresting its proliferation at certain well-known checkpointsCitation37. Upon treatment of cancer cells with anticancer agents, distinguish cells can be detected in various phases of cell cycle. Herein, MCF-7 cells were treated with compound 6n at its IC50 (1.04 µM). Analysing the obtained data, reveals that compound 6n produced a marked decrease of the cell population at G1 and S phases as compared to the control (55.3% and 27.56%, versus 64.82% and 26.85%, respectively). Whereas it caused significant decrease of cell population in the G2/M phase when compared with untreated control cells (17.13% versus 8.33%). These results prove that compound 6n exerts its cytotoxic effect in MCF-7 cells through arresting its proliferation in G2/M phase ().
Annexin-V FTIC apoptotic study
Annexin V-based flow cytometry analysis indicates either the cell death is achieved through programmed apoptosis or non-specific necrosisCitation38. Therefore, Annexin V-FTIC/DAPI dual staining assay () was carried out for compound 6n aiming to evaluate its apoptotic effect. In the current study, MCF-7 cells were treated with 6n.
Figure 6. Effect of compound 6n on the percentage of Annexin V-FITC positive staining in MCF-7 cells versus control (1% DMSO).

Scrutinising the results of Annexin-V FTIC assay revealed that 6n exhibited a marked increase in the percent of Annexin V-FITC positive apoptotic cells including both the early and late apoptotic phases, from 1.14% to 21.74% which implies 19-folds increase as compared with the control (p < .001). Moreover, only 2.45% of the cells showed non-specific necrosis. These findings are in accordance with the previous apoptosis studies observations that propound undoubtedly that compound 6n exhibits pro-apoptotic potential which contributes to its anti-proliferative activity.
Activation of proteolytic caspase cascade
Activation of the cysteine aspartic proteases, known as caspases, comprises a pivotal role in the self-automated cell death; apoptosisCitation39. Consequently, the effect of compound 6n on caspase 3, the executioner caspase, was evaluated aiming to investigate its pro-apoptotic effect. Moreover, its effect on caspase 8 and caspase 9 activations was determined in order to specify either apoptosis was induced through the intrinsic or extrinsic pathway. Results revealed that compound 6n amazingly induced the level of active caspase 3 by 76-folds in comparison with control cells. Exploring its effects on both caspase 8 and caspase 9 revealed that it potentiated the level of the former by only 1.98-folds, whereas it produced marked increments of the latter by 928-folds. These results strongly suggest that compound 6n induced apoptosis in MCF-7 cell line through the intrinsic rather than the extrinsic pathway ().
Table 3. Effect of compound 6n on the active caspases-3, -8 and -9 levels, and the expression levels of Bcl-2, Bax and cytochrome C, respectively, in MCF-7 cancer cells treated with the compound at its IC50 concentration.
Effects on mitochondrial apoptosis pathway (Bcl-2 family) proteins
Members of the Bcl-2 are chief regulators of the mitochondrial apoptotic pathwayCitation40. Among these, Bax and Bcl-2 precisely modulate this programmed process. Both proteins have opposing effects on apoptosis induction, where Bax possesses pro-apoptotic activity while Bcl-2 has anti-apoptotic effectCitation41,Citation42. Thus, the ratio between them is the determining factor for cell fate regulationCitation42. Accordingly, in the current study, MCF-7 cells were treated with the IC50 of compound 6n and its effect on the levels of both Bax and Bcl-2 were recorded in . Furthermore, the Bax/Bcl2 ratio was determined to give more profound insight of the pro-apoptotic activity of the molecule.
As indicated by the results in , compound 6n amazingly boosted the Bax level 379.8-folds as compared with the control. Whereas it only downregulated the Bcl2 level by 1.07-folds. A rather decisive value is the Bax/Bcl2 ratio which gives insight to the overall pro-apoptotic effect of our compound. The calculated value for the Bax/Bcl2 ratio in comparison with that of the reference compound is increased by 407-folds. Conclusively, the ability of compound 6n to upregulate Bax level, downregulate Bcl2 level while significantly boosting the Bax/Bcl2 ratio proves undoubtedly compound 6n pro-apoptotic activity.
Cytochrome C activation
Mitochondrial cytochrome C is released into the cytosol as a result of Bax/Bcl2 increments. Accordingly, it potentiates a cascade of caspases that finally triggers the executioner caspase: caspase 3Citation43,Citation44. From the previous findings, compound 6n proved to induce apoptosis in MCF-7 cell line through activation of the intrinsic rather than the extrinsic pathway as witnessed by the significant augmentation of caspase 9 level compared to caspase 8 level increments. Thus, we measured the level of cytochrome C to further emphasise the adoption of the intrinsic pathway. MCF-7 cells were treated with the IC50 of compound 6n and the level of cytochrome C was determined as compared with the control. Results revealed that cytochrome C level increased by 581-folds ().
Apoptotic effect through generation of ROS
DPPH free radical scavenging activities
In the current study, the antioxidant potential of the synthesised compounds 6i, 6j, 6n and 6q was tested using one of the most common antioxidant assays; the DPPH (2,2-diphenyl-1-picrylhydrazyl free radical) radical scavenging assay, and were compared with standard antioxidant (ascorbic acid). DPPH is a stable free radical that has the potential to accept an electron producing a stable molecule. Compounds that have the ability to act as electron donors result in reduction of this DPPH radical revealing their antioxidant potential. The principle of the assay depends on measuring the absorbance of the odd electrons of DPPH at 516 nm. In the presence of free radical scavengers, the absorbance decreases proportional to the decrease of the DPPH radical concentration. IC50 values that produce 50% inhibition of the DPPH radical are given in .
Table 4. Evaluation of antioxidant activity for compounds 6i, 6j, 6n, and 6q using DPPH radical scavenging activity.
The results reveal that all the tested compounds, 6i, 6j, 6n and 6q, exhibited much higher IC50 values compared with that of ascorbic acid (IC50 = 115.8 µg/ml, 43.9 µg/ml, 31.6 µg/ml and 48.1 µg/ml, versus 9.7 µg/ml, respectively). This confirms that they have low antioxidant potential which favours the induction of oxidative stress that triggers apoptosis in neoplastic cells.
Oxidative stress parameters
In the current study, we evaluated the activities of some free radical enzymes including SOD, CAT, GSHPx, lipid peroxide (LP) and PC in MCF-7 cells treated with our synthesised compounds (). Moreover, the level of total protein was evaluated as an indication of the cytotoxic effect of the tested compounds.
Table 5. Oxidative stress markers and antioxidants in control and MCF-7 treated cells with compounds 6i, 6j, 6n and 6q.
The results reveal that treatment of the MCF-7 cells with IC50 values of the compounds 6i, 6j, 6n and 6q elevated the level of SOD with concomitant depression of CAT and GSHPx as compared to the control cells. Additionally, the levels of ROS exemplified in LP were significantly elevated in comparison with the control cells. Moreover, the levels of PC were also elevated consequently ().
These results can thoroughly prove that the cytotoxic effect of the tested compounds 6i, 6j, 6n and 6q is partially exerted by disrupting the balance between free radical or ROS production and the antioxidant system. This is achieved through elevation of ROS (LP) levels evidenced by the significant increase in the level of SOD which triggers the production of ROS that in turn mediates apoptosis. ROS catabolising enzymes as CAT and GSH-Px levels were shown to be depleted in MCF-7 cells treated with the synthesised compounds as compared with the control which guarantees the consequent accumulation of ROS that eventually affects tumour cell killing due to protein oxidation which converts the protein to PC derivatives. In accordance, the elevated PC levels imply the protein oxidative damage as it is a reliable marker for protein damage. These results are consistent with the postulation that these compounds endeavour their cytotoxic activity through ROS generation. In accordance with these findings, the level of protein in the treated cells was lower than that of the control due to the oxidative damage of the protein by ROS over generation.
Conclusion
Hybridisation technique between indole and 3-hydrazinoindolin-2-one was successful to produce apoptosis inducing agents. According to this technique, 27 compounds were designed and synthesised to be investigated for their cytotoxic activity against three cancer cell lines. Compound 6n showed the best activity with IC50 = 1.04 µM against MCF-7 cancer cell line. This compound displayed a broad-spectrum activity against different cancer cell lines in 60-cell line panel test by NCI-USA. Deep apoptotic study on this compound revealed that this compound would disrupt G2/M phase in cell cycle and increase % of early and late apoptosis by 19-folds more than control in Annexin V-FTIC. Compound 6n exhibited an ability to increase the expression of some enzymes that affect the apoptosis as caspase 3, caspase 9, cytochrome c, Bax and decrease the expression of Bcl-2. Compound 6n showed the ability to induce apoptosis by generation of ROS and this shown in a further biological study on oxidative stress markers as superoxide dismutase (SOD), CAT and GSHPx.
IENZ_1421181_Supplementary_Material.pdf
Download PDF (1.8 MB)Disclosure statement
No potential conflict of interest was reported by the authors.
Additional information
Funding
References
- Elmore S. Apoptosis: a review of programmed cell death. Toxicol Pathol 2007;35:495–516.
- Aggarwal BB, Ichikawa H. Molecular targets and anticancer potential of indole-3-carbinol and its derivatives. Cell Cycle 2005;4:1201–15.
- Pfeifer BL, Fahrendorf T. Indole-3-carbinol: a glucosinolate derivative from cruciferous vegetables for prevention and complementary treatment of breast cancer. Deut Z Onk 2015;47:20–7.
- Chakraborty S, Ghosh S, Banerjee B, et al. Phemindole, a synthetic di-indole derivative maneuvers the store operated calcium entry (SOCE) to induce potent anti-carcinogenic activity in human triple negative breast cancer cells. Front Pharmacol 2016;7:114.
- Weng J-R, Tsai C-H, Kulp SK, et al. A potent indole-3-carbinol–derived antitumor agent with pleiotropic effects on multiple signaling pathways in prostate cancer cells. Cancer Res 2007;67:7815–24.
- Weng J-R, Tsai C-H, Omar H, et al. OSU-A9, a potent indole-3-carbinol derivative, suppresses breast tumor growth by targeting the Akt-NF-kB pathway and stress response signaling. Carcinogenesis 2009;30:1702–9.
- Kamal A, Srikanth Y, Ramaiah MJ, et al. Synthesis, anticancer activity and apoptosis inducing ability of bisindole linked pyrrolo [2, 1-c][1, 4] benzodiazepine conjugates. Bioorg Med Chem Lett 2012;22:571–8.
- Lee S-O, Abdelrahim M, Yoon K, et al. Inactivation of the orphan nuclear receptor TR3/Nur77 inhibits pancreatic cancer cell and tumor growth. Cancer Res 2010;70:6824–36.
- Ibrahim HS, Abou-Seri SM, Abdel-Aziz HA. 3-Hydrazinoindolin-2-one derivatives: chemical classification and investigation of their targets as anticancer agents. Eur J Med Chem 2016;122:366–81.
- Fares M, Eldehna WM, Abou‐Seri SM, et al. Design, synthesis and in vitro antiproliferative activity of novel isatin–quinazoline hybrids. Arch Der Pharm 2015;348:144–54.
- Sirisoma N, Pervin A, Drewe J, et al. Discovery of substituted N′-(2-oxoindolin-3-ylidene) benzohydrazides as new apoptosis inducers using a cell- and caspase-based HTS assay. Bioorg Med Chem Lett 2009;19:2710–3.
- Nasr T, Bondock S, Youns M. Anticancer activity of new coumarin substituted hydrazide–hydrazone derivatives. Eur J Med Chem 2014;76:539–48.
- Ibrahim HS, Abou-seri SM, Ismail NS, et al. Bis-isatin hydrazones with novel linkers: Synthesis and biological evaluation as cytotoxic agents. Eur J Med Chem 2016;108:415–22.
- (a) Eldehna WM, Almahli H, Al-Ansary GH, et al. Synthesis and in vitro anti-proliferative activity of some novel isatins conjugated with quinazoline/phthalazine hydrazines against triple-negative breast cancer MDA-MB-231 cells as apoptosis-inducing agents. J Enzyme Inhib Med Chem 2017;32:600–13. (b) Abdel-Aziz HA, Eldehna WM, Keeton AB, et al. Isatin-benzoazine molecular hybrids as potential antiproliferative agents: synthesis and in vitro pharmacological profiling. Drug Des Dev Ther 2017;11:2333–46. (c) Attia MI, Eldehna WM, Afifi SA, et al. New hydrazonoindolin-2-ones: synthesis, exploration of the possible anti-proliferative mechanism of action and encapsulation into PLGA microspheres. PloS One 2017;12:e0181241. (d) Eldehna WM, Abo-Ashour MF, Nocentini A, et al. Novel 4/3-((4-oxo-5-(2-oxoindolin-3-ylidene) thiazolidin-2-ylidene) amino) benzenesulfonamides: synthesis, carbonic anhydrase inhibitory activity, anticancer activity and molecular modelling studies. Eur J Med Chem 2017;139:250–62. (e) Eldehna WM, Fares M, Ibrahim HS, et al. Synthesis and cytotoxic activity of biphenylurea derivatives containing indolin-2-one moieties. Molecules 2016;21:762. (f) Eldehna WM, Fares M, Ibrahim HS, et al. Indoline ureas as potential antihepatocellular carcinoma agents targeting VEGFR-2: synthesis, in vitro biological evaluation and molecular docking. Eur J Med Chem 2015;100:89–97. (g) Abdel-Aziz HA, Ghabbour HA, Eldehna WM, et al. Synthesis, crystal structure, and biological activity of cis/trans amide rotomers of (Z)-N′-(2-Oxoindolin-3-ylidene)formohydrazide. J Chem 2014;2014:760434.
- (a) Hockenbery DM, Oltvai ZN, Yin X-M, et al. Bcl-2 functions in an antioxidant pathway to prevent apoptosis. Cell 1993;75:241–51. (b) Circu ML, Aw TY. Reactive oxygen species, cellular redox systems, and apoptosis. Free Radic Biol Med 2010;48:749–62.
- James P, Snyder NH. Indole‐3‐Aldehyde. Org Synth 1959;39:30.
- Eldehna WM, Altoukhy A, Mahrous H, Abdel-Aziz HA. Design, synthesis and QSAR study of certain isatin-pyridine hybrids as potential anti-proliferative agents. Eur J Med Chem 2015;90:684–94.
- Wang Y-T, Qin Y-J, Yang N, et al. Synthesis, biological evaluation, and molecular docking studies of novel 1-benzene acyl-2-(1-methylindol-3-yl)-benzimidazole derivatives as potential tubulin polymerization inhibitors. Eur J Med Chem 2015;99:125–37.
- Eldehna WM, Al-Ansary GH, Bua S, et al. Novel indolin-2-one-based sulfonamides as carbonic anhydrase inhibitors: synthesis, in vitro biological evaluation against carbonic anhydrases isoforms I, II, IV and VII and molecular docking studies. Eur J Med Chem 2017;127:521–30.
- El-Faham A, Hozzein WN, Wadaan MA, et al. Microwave synthesis, characterization, and antimicrobial activity of some novel isatin derivatives. J Chem 2015;2015:716987.
- Chawla HM, Gupta T. New chromogenic bis (isatin hydrazonyl) calix [4] arenes for dual recognition of fluoride and silver ions. Tetrahedron Lett 2013;54:1794–97.
- Mosmann T. Rapid colorimetric assay for cellular growth and survival: application to proliferation and cytotoxicity assays. J Immunol Methods 1983;65:55–63.
- Monks A, Scudiero D, Skehan P, et al. Feasibility of a high-flux anticancer drug screen using a diverse panel of cultured human tumor cell lines, JNCI. J Natl Cancer Inst 1991;83:757–66.
- Boyd MR, Paull KD. Some practical considerations and applications of the National Cancer Institute in vitro anticancer drug discovery screen. Drug Dev Res 1995;34:91–109.
- MR, Boyd In: B.A. Teicher, ed. Cancer Drug discovery and development: anticancer drug development guide: preclinical screening, clinical trials and approval, 2nd ed. Totowa, NJ: Humana Press; 2014: 41–62 (Chapter 1).
- Skehan P, Storeng R, Scudiero D, et al. New colorimetric cytotoxicity assay for anticancer-drug screening. JNCI, J Natl Cancer Inst 1990;82:1107–12.
- Eldehna WM, Abou-Seri SM, El Kerdawy AM, et al. Increasing the binding affinity of VEGFR-2 inhibitors by extending their hydrophobic interaction with the active site: design, synthesis and biological evaluation of 1-substituted-4-(4-methoxybenzyl) phthalazine derivatives. Eur J Med Chem 2016;113:50–62.
- Abou-Seri SM, Eldehna WM, Ali MM, El Ella DAA. 1-Piperazinylphthalazines as potential VEGFR-2 inhibitors and anticancer agents: synthesis and in vitro biological evaluation. Eur J Med Chem 2016;107:165–79.
- ElSheikh HH, Elaasser MM, Magdy HM, Abdel-Kareem SM. Antimicrobial, antitumor and antioxidant activities of certain marine fungi isolated from Alexandria. Afr J Mycol & Biotech 2014;19:13–22.
- Lowry OH, Rosebrough NJ, Farr AL, Randall RJ. Protein measurement with the Folin–phenol reagent. J Biol Chem 1951;193:265–75.
- Kakkar P, Das B, Viswanathan PN. A modified spectrophotometric assay of superoxide dismutase. Indian J Biochem Biophys 1984;21:130–32.
- Aebi H. Catalase in vitro. Methods Enzymol 1984;113:121–26.
- Paglia DE, Valentine WN. Studies on qualitative and quantitative characterization of erythrocytes glutathione peroxidase. J Lab Clin Med 1967;70:158–69.
- Buege JA, Aust SD. Microsomal lipid peroxidation. Methods Enzymol 1978; 52:302–10.
- Levine RL, Williams JA, Stadtman ER, Shacter E. Carbonyl assays for determination of oxidatively modified proteins. Methods Enzymol 1994;233:46–57.
- Ibrahim HS, Abdelhadi SR, Abdel-Aziz HA. Hydrolysis and hydrazinolysis of isatin-based ald-and ketazines. J Chem 2015;2015:826489.
- Chan KT, Meng FY, Li Q, et al. Cucurbitacin B induces apoptosis and S phase cell cycle arrest in BEL-7402 human hepatocellular carcinoma cells and is effective via oral administration. Cancer Lett 2010;294:118–24.
- Szumilak M, Szulawska-Mroczek A, Koprowska K, et al. Synthesis and in vitro biological evaluation of new polyamine conjugates as potential anticancer drugs. Eur J Med Chem 2010;45:5744–51.
- Wu Z-R, Liu J, Li J-Y, et al. Synthesis and biological evaluation of hydroxycinnamic acid hydrazide derivatives as inducer of caspase-3. Eur J Med Chem 2014;85:78–783.
- Tsujimoto Y. Cell death regulation by the Bcl-2 protein family in the mitochondria. J Cell Physiol 2003;195:158–67.
- Callagy GM, Webber MJ, Pharoah PD, Caldas C. Meta-analysis confirms BCL2 is an independent prognostic marker in breast cancer. BMC Cancer 2008;8:153
- McDonnell TJ, Korsmeyer SJ. Progression from lymphoid hyperplasia to high-grade malignant lymphoma in mice transgenic for the t (14; 18). Nature 1991;349:254.
- Zhu H, Zhang J, Xue N, et al. Novel combretastatin A-4 derivative XN0502 induces cell cycle arrest and apoptosis in A549 cells. Invest New Drugs 2010;28:493–501.
- Weir NM, Selvendiran K, Kutala VK, et al. Curcumin induces G2/M arrest and apoptosis in cisplatin-resistant human ovarian cancer cells by modulating Akt and p38 MAPK. Cancer Biol Ther 2007;61:78–184.