Abstract
Finding a new type of cholinesterase inhibitor that would overcome the brain availability and pharmacokinetic parameters or hepatotoxic liability has been a focus of investigations dealing with the treatment of Alzheimer’s disease. Isothiocyanates have not been previously investigated as potential cholinesterase inhibitors. These compounds can be naturally produced from their glucosinolate precursors, secondary metabolites widely distributed in our daily Brassica vegetables. Among 11 tested compounds, phenyl isothiocyanate and its derivatives showed the most promising inhibitory activity. 2-Methoxyphenyl ITC showed best inhibition on acetylcholinesterase with IC50 of 0.57 mM, while 3-methoxyphenyl ITC showed the best inhibition on butyrylcholinesterase having 49.2% at 1.14 mM. Assessment of the antioxidant efficacy using different methods led to a similar conclusion. The anti-inflammatory activity was also tested using human COX-2 enzyme, ranking phenyl isothiocyanate, and 3-methoxyphenyl isothiocyanate as most active, with ∼99% inhibition at 50 μM.
Introduction
Alzheimer’s disease is the most common neurodegenerative disorder in western societies, mostly affecting elderly population. The most prominent symptom includes decrease in cognitive function, which in turn leads to changes in the behavioural patterns of an individual. Both acetylcholinesterase and butyrylcholinesterase still represent the only pharmacotherapy able to affect the increase of the acetylcholine neurotransmitter in the brainCitation1–3. Essential oils, containing small lipophilic molecules, such as terpenes, terpenoids, phenylpropanoids, and others, were increasingly studied lately since they can readily cross the blood-brain barrierCitation4. Interestingly, there are no reports whatsoever on the so called “mustard oils”, the major constituents of which are isothiocyanates (ITCs), known for their diversified and generally marked bioactivityCitation5. Mustard oils stand as bio-markers of Brassica vegetables which encompass many species used in daily diets (cabbage, horseradish, cauliflower, turnip, radish, cress, etc.).
Oxidative stress is most commonly associated with the development of many diseases, including neurodegenerative disordersCitation6. Different studies have mentioned ITCs as antioxidants, particularly in relation with induction of phase II enzymes, though a limited number of them have shown direct antioxidant behaviourCitation7. The antioxidant potentials of volatile methylsulfanylalkyl ITCs such as erucin and raphasatin were revealed by effective quenching of hydrogen peroxide and organic hydroperoxidesCitation8,Citation9 which are commonly classified as reactive oxygen species (ROS) and are important contributors to oxidative stress in vivo.
Antioxidant and anti-inflammatory effects of ITCs are likely mediated via the activation of Nrf2 and inhibition of NF-κBCitation10,Citation11. The anti-inflammatory properties of allyl ITC and other plant-derived ITCs have been previously reportedCitation10,Citation12,Citation13. After oral administration, allyl ITC is distributed in the tissues as well as in the brain of rats and miceCitation14. Additionally, it was reported that ITCs protect the blood-brain barrier from oxidative stress-induced injuryCitation15. Overproduction of PGE-2 and increased COX-2 activity are frequently observed in a variety of inflammation-associated disorders, such as Alzheimer's disease, Parkinson's disease, certain kinds of cancers, and cardiovascular problemsCitation10,Citation16,Citation17.
In the present study, 11 commercially available ITCs were tested for their cholinesterase inhibitory (using Ellman’s method), and antioxidant (using DPPH, ORAC, Briggs-Rauscher, Rancimat, and FRAP method) activities as well as anti-inflammatory activity via PGE-2 synthesis suppression through COX-2 inhibition.
Materials and methods
All reagents and solvents used were of analytical grade. Isothiocyanates 1–11 were purchased from Sigma-Aldrich GmbH (Steinheim, Germany). Acetylcholinesterase (AChE, from Electrophorus electricus – electric eel, type V-S), butyrylcholinesterase (BChE, from equine serum), acetylthiocholine iodide (ATChI), butyrylthiocholine iodide (BTChI), and 5,5-dithiobis(2-nitrobenzoic acid) (DTNB, Ellman’s reagent), were also purchased from Sigma-Aldrich. The human COX-2 inhibitor screening assay kit was purchased from Cayman Chemical (Item № 701080, Ann Arbor, MI). Indomethacin (purity 99%) was obtained from Fluka (Buchs, Switzerland). Absorbance and fluorescence measurements were performed on a Synergy HTX S1LFA multi-mode microplate reader (BioTek Instruments, Inc., Winooski, VT).
Antioxidant activity
Assessment of the antioxidant activity was carried out using five methods: scavenging ability of 2,2-diphenyl-1-picrylhydrazyl radical (DPPH˙), Oxygen Radical Absorbance Capacity (ORAC), Briggs–Rauscher (BR) oscillating reaction, Rancimat assay and Ferric Reducing/Antioxidant Power (FRAP) method.
DPPH assay
DPPH scavenging ability of the samples was measured according to a recently reported procedureCitation18. The results for free radical scavenging activities of the samples are expressed as IC50 values (where possible) and inhibition percentages of DPPH radical (% inhibition).
ORAC assay
ORAC assay was performed according to the slightly modified procedure described by Prior et al.Citation19. The volumes were modified so as to perform in 96-well microplates and the reaction was followed during 80 min. The results are expressed as mM of Trolox Equivalents (mM TE).
Briggs–Rauscher assay
ITCs ability to stop the Briggs–Rauscher (BR) oscillations was assayed as described previouslyCitation20. BR assay is based on the fact that the addition of antioxidant in oscillating BR system (visually detectable as fast colour change between blue → yellow → colourless) results in immediate quenching of the oscillations (visually = no colour). The quenching time linearly depends on the type and concentration of the added antioxidant. The oscillations in the BR assays were followed spectrophotometrically at 620 nm and the results are expressed as the inhibition time (in seconds).
Rancimat assay (oxidation stability testing)
Fish oil was investigated using a Rancimat 743 (Metrohm, Herisau, Switzerland) instrument in order to monitor the progress of accelerated oxidation. The fish oil samples (3 g) were exposed to a temperature of 120 °C (ΔT = 1.4 °C) and a constant air flow of 20 L/h. The conductivity was measured as a function of time and the results are expressed as Protection Factor (PF), calculated according to the equation PF = sample induction time/control induction timeCitation21.
FRAP assay
The reducing potential of ITCs was measured as described by Benzie and StrainCitation22. In this assay, antioxidants are evaluated as reducing agents of Fe3+ to Fe2+, which undergoes chelation by 2,4,6-tris(2-pyridyl)-s-triazine (TPTZ) to form a Fe2+-TPTZ complex absorbing at 593 nm.
Acetylcholinesterase/butyrylcholinesterase inhibitory activity
AChE/BChE inhibitory activity measurements were carried out by a slightly modified Ellman assay as described before for AChE inhibitory activityCitation23. A typical run consisted of 180 μL of phosphate buffer (0.1 M, pH 8), 10 μL of DTNB (at a final concentration of 0.3 mM prepared in 0.1 M phosphate buffer pH 7 with 0.12 M sodium bicarbonate added for stability), 10 μL of sample solution (dissolved in EtOH), and 10 μL of AChE/BChE solution (with final concentration 0.03 U/mL). Reactants were mixed in a cuvette and reaction was initialised by adding 10 μL of acetylthiocholine iodide/butyrylthiocholine iodide (ATChI/BTChI, to reach a final concentration of 0.5 mM). As negative control, EtOH was used instead of sample solution. Non-enzymatic hydrolysis was also monitored by measurement of two blank runs for each run. In short: in the first blank, the AChE/BChE, respectively, was replaced by equivalent buffer amount and in second blank, the ATChI/BTChI, respectively, was replaced by equivalent buffer amount. All spectrophotometric measurements were performed at 405 nm and at room temperature for 6 min periods. The results are expressed as percentage inhibition of enzyme activity.
Anti-inflammatory activity
To analyse the inhibition of the COX-2 enzyme activity mediated by the selected ITCs, the COX-2 (human) inhibitor screening assay kit from Cayman was used. In summary, the COX-2 enzyme was incubated with the samples (having final concentration in the assay of 50 μM, in 96% ethanol) for 10 min at 37 °C and subsequently the reaction was initiated by adding arachidonic acid and incubating the mixture for 2 min at 37 °C. The background tubes correspond to inactivated COX-2 enzyme obtained after keeping the tubes containing enzymes in boiling water for 3 min along with sample solvent. Enzyme catalysis was blocked by adding saturated stannous chloride solution. Prostaglandin (PGF2α) release was quantified using ELISA at 412 nm. Indomethacin solution (having final concentration in the assay of 10 μM, in 96% ethanol) was used as a positive control.
Results and discussion
Structures of isothiocyanates (ITCs) tested on different biological activities are shown in .
Figure 1. Structures of the isothiocyanates (ITCs) used in the current study: 1 – phenyl ITC, 2 – benzyl ITC, 3 – 2-phenylethyl ITC, 4 – 4-methylsulfanylphenyl ITC, 5 – 4-methylphenyl ITC, 6 – 4-methoxyphenyl ITC, 7 – 2-methoxyphenyl ITC, 8 – 3-methoxyphenyl ITC, 9 – allyl ITC, 10 – isopropyl ITC and 11 – 3-(methylsulfanyl)propyl ITC.
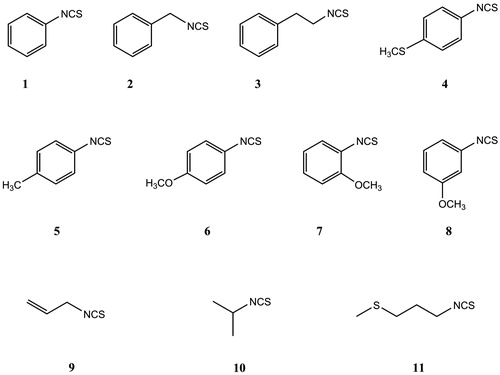
Antioxidant activity
Free radical scavenging activity
The free radical scavenging potential of ITCs was determined by DPPH, ORAC, and BR antioxidant assays (). For practical purposes, the concentrations of the investigated compounds tested by DPPH method are given as stock solutions and should be divided by a factor of 21 to obtain the final concentration in the system (), and are discussed as such in the text ahead. The half-maximal DPPH radical scavenging concentration (IC50) was calculated for phenyl ITC (1), 3-methoxyphenyl ITC (8), 4-methoxyphenyl ITC (6), 4-methylphenyl ITC (5), and 2-methoxyphenyl ITC (7) having IC50 of 1.08, 1.16, 1.25, 1.45 and 3.90 mM, respectively. 4-Methylsulfanylphenyl ITC (4) had to be tested at lower concentration due to turbidity problems, with 1.19 mM (final concentration) showing 9.9% of DPPH inhibition. Montaut et al.Citation24 reported 4-hydroxybenzyl ITC to have IC50 of 9.43 mM. This compound, having OH group as a strongly activating substituent on the aromatic ring, showed a much weaker activity than phenyl ITC (1). 3-Methoxyphenyl ITC (8), 4-methoxyphenyl ITC (6), 4-methylphenyl ITC (5), and 2-methoxyphenyl ITC (7), bearing a substituent on the aryl ring, also exhibited a decrease in the DPPH activity, thus indicating a negative effect of substitution. When the ITC group was not directly attached on the aryl ring like in benzyl ITC (2) and 2-phenylethyl ITC (3), the free radical scavenging ability also rapidly decreased.
Table 1. Antioxidant properties of isothiocyanates (ITCs).
Benati et al.Citation25–27 investigated the attack of aryl radicals on ITCs, highlighting the variation of electrophilicity of the radical depending on substituents. Thus, electron-withdrawing substituents would favour the nucleophilic S-addition of the ITC, hence increasing the overall efficiency of the cascade reaction. In connection with the results of Benati et al.Citation25–27, one may hypothesise that the S-atom of ITCs reacts with the DPPH radical, to produce an α-(arylsulfanyl)imidoyl radical that can gain stabilisation through interference with one of the phenyl rings of DPPH (). However, it was observed that aryl ITCs (5–8) bearing electron-donating groups showed a decrease in free radical scavenging ability when compared to “group free” phenyl ITC (1).
3-(Methylsulfanyl)propyl ITC (11), allyl ITC (9), and isopropyl ITC (10) were also tested and they were found to be very inefficient scavengers at 4.76 mM having 12.6, 10.1 and 4.2%, respectively. Montaut et al.Citation24 reported 4-(methylsulfanyl)butyl ITC (erucin) to be also very ineffective, having IC50 of 57.1 mM. 4-(Methylsulfanyl)but-3-enyl ITC (raphasatin) was reported to have a high reactivity toward the DPPH radical, presumably due to hydrogen atom abstraction either in allylic position, or in alpha to the –NCS group or bothCitation9. 4-(Methylsulfinyl)butyl ITC (sulforaphane) also showed scavenging activity of DPPH radical with IC50 of 3.8 mMCitation28.
Alkyl ITCs bearing a sulfanyl group (like erucin, raphasatin and ibervirin 11) in the side-chain and arylalkyl ITCs (like 2 and 3) are expected to react with radicals by H-atom transfer from the methylene group neighbouring the S-atom or the phenyl ringCitation7, contrary to the cases described by Benati et al.Citation25,Citation26, for which such methylene groups are missing. When tested by the DPPH method, ITCs 2, 3 and 11 showed very insignificant antiradical activity. Similarly, ITC 3 was reported by Sarkar et al.Citation29 to be a very inefficient free radical scavenger.
ORAC assay is a method that combines both inhibition percentage and inhibition time of the reactive species action by antioxidants into a single quantityCitation30. It reflects classical radical chain breaking antioxidant activity by H atom transfer and it measures antioxidant inhibition of peroxyl radical induced oxidationsCitation31. Although Valgimigli and IoriCitation7 reported that ITCs do not express chain-breaking activity, our study produced contrasting results. Samples were tested using ORAC assay at several concentrations (results not shown) similar to those of DPPH, but they had to be diluted due to the high sensitivity of the ORAC method. Results are presented in with all the compounds measured at the final concentration of 1.56 µM. According to the present results the highest activity of 32 mM TE was detected for 4-methylsulfanylphenyl ITC (4). The activity of this compound was not detected by DPPH method due to turbidity problems, while the ORAC method circumvents turbidity problems since it is based on measurement of fluorescence. Direct connection of the –NCS group to the aromatic ring resulted in good activity of the ITCs, as previously observed, while in the case of compounds 2 and 3 a significant decrease in the activity was detected. The lowest activity was found for 2-methoxyphenyl ITC (7) and isopropyl ITC (10), as well as for arylalkyl ITCs 2 and 3. Among methoxyphenyl ITCs (6, 7 and 8) the highest free radical scavenging activity was detected for 3-methoxyphenyl ITC (8) while among alkyl ITCs bearing a sulfanyl group, a remarkable activity was observed for 3-(methylsulfanyl)propyl ITC (11) (20.3 mM TE).
The Briggs–Rauscher assay gave more or less no activity for any of the ITCs at the highest tested concentration except for 4-methoxyphenyl ITC (6) that prolonged oxidation for 9180 s at final concentration of 0.81 mM. However, the ortho- (7) and meta- (8) regioisomers of 6 did not exhibit activity at four times higher concentration. Chen et al.Citation32 reported comparable observations when investigating cyclohexanedione isomers: the 1,3-dione showed inhibition time, whereas the 1,4-dione did not.
Reducing activity
ITCs were also tested using FRAP assay, which is presumed to estimate the “total antioxidant power”. The relevant chemical reaction of the FRAP method represents the “total reducing power” as it involves a single electron reaction between Fe(TPTZ)2 (III) and any species able to reduce it to Fe(TPTZ)2 (II), making this species an antioxidantCitation31. In our study, none of the ITCs tested showed any reductive capacity.
Oxidative stability testing
The antioxidant activity of ITCs was also tested by means of a Rancimat apparatus, using a lipophilic system at 120 °C with an air flow rate of 20 L/h. The results are expressed as protection factors (PF), taking PF = 1 for the control sample. The best activity was found for phenyl ITC (1) with PF = 2.5 at 3.23 mM. For ITCs in which the –NCS group is not directly attached to the aromatic ring, the activity is decreasing (compared to 1) as seen for compounds 2 (PF = 1.5) and 3 (PF = 1.3) at the same concentration. Some of the ITCs – either alkyl or aryl – exhibited activities close to that of 2, i.e. allyl ITC (9), 3-methoxyphenyl ITC (8), 2-methoxyphenyl ITC (7), with PF = 1.7, and 3-(methylsulfanyl)propyl ITC (11) with PF = 1.6 at 3.23 mM. 4-Methylsulfanylphenyl ITC (4), 4-methylphenyl ITC (5), 4-methoxyphenyl ITC (6), and isopropyl ITC (10) did not show any protective effect.
Cholinesterase inhibition
To the best of our knowledge, the present paper reports the first investigation on cholinesterase (ChE) inhibitory activity of ITCs. The inhibition of acetylcholinesterase (AChE) and butyrylcholinesterase (BChE) was assessed for 6 aromatic, 2 arylaliphatic, and 3 aliphatic ITCs and results are reported in . Generally, aromatic and arylaliphatic ITCs showed better activity than aliphatic ITCs (except for ITC 4, due to the turbidity problems at higher concentrations). The best activity was shown by 3-methoxyphenyl ITC (8) with 61.4% of AChE inhibition at 1.14 mM. Para- (6) and ortho- (7) isomers of ITC 8 also showed relatively good activity with 30.4 and 57.0%, respectively, at the same concentration. The inhibitory activity of 4-methylphenyl ITC (5) on AChE was comparable to that of 7 and 8 with 58.4% at 1.14 mM. Electron donating groups (–OMe, –Me) seem to contribute to the increase of inhibitory activity when compared to phenyl ITC (1) which showed only 17.9% at the same concentration. In the case of arylaliphatic ITCs 2 and 3, the inhibitory activity was also relatively good with 37.2 and 48.1%, respectively, at 1.14 mM.
Table 2. Cholinesterase and COX-2 inhibition by isothiocyanates (ITCs).
In general, all tested compounds showed lower inhibitory activity towards BChE, except for compounds 1 and 11. As observed in the case of AChE, the inhibitory activity of the aromatic ITC 8 towards BChE was shown to be the best with 49.2% at 1.14 mM. Closely related aryl ITCs 1 and 5 also showed notable activity with 42.1 and 40.7%, respectively, at the same concentration. In comparison to 1, the inhibitory activity towards BChE is similar (5, 8) or inferior when substituents are present on the aromatic ring (6, 7), or when the –NCS group is not directly attached to the aromatic ring (2). Aliphatic ITCs 9–11 showed very weak activity or no inhibition at all tested concentrations on both enzymes.
Anti-inflammatory activity
Results for the inhibition of prostaglandin biosynthesis, by ITCs as determined using COX-2 assay, are given in and are expressed as % of inhibition. Indomethacin was used as a positive control having 98.9% inhibition at the final concentration of 10 μM. Among the 11 tested ITCs, phenyl ITC (1), 2-methoxyphenyl ITC (7), and 3-(methylsulfanyl)propyl ITC (11) exhibited significant inhibitory activity of COX-2 enzyme with 98.9, 99 and 48%, respectively, at final concentration of 50 μM. At the same concentration, ITCs 2, 6, and 8 did not show any inhibitory activity on prostaglandin production. On the other hand, ITCs 9, 3, 4, 5 and 10 showed moderate to low inhibitory activity having 19.5, 17.8, 15.5, 13.6 and 9.4%, respectively, at final concentration of 50 μM. The effective inhibitory activity of 1 is in accordance with previously reported results on suppression of PGE2 synthesisCitation11. Bhattacharya et al.Citation33 also reported that 9, the most common and most studied naturally occurring ITC, showed no expected inhibitory activity against COX-2. However, studies of in vitro ITC inhibitory activity on COX-2 enzyme are scarce. The data presented in indicate that inhibitory activity of the tested ITCs is quite specific. It seems that a slight change in the ITC structure can have a significant impact on its inhibitory potential, i.e. 7 showing strong inhibitory activity contrary to its regioisomers 6 and 8 which proved inactive at the tested concentration. The effective inhibition of prostaglandin synthesis by ITCs 1, 7 and 11 calls for further investigation of their effects on COX-2 protein and mRNA expression.
Conclusion
Herein, we tested 11 ITCs for their biological activity (i.e. antioxidative, anti-cholinesterase, and anti-inflammatory). Generally, among the tested ITCs, it can be suggested that aryl ITCs show the most promising potential. These results can be used as a basis for future investigation of other phenyl ITC derivatives bearing electron-donating and -withdrawing groups in order to better understand the influence of diversified substituents towards different biological activities.
Acknowledgements
This research has been fully supported by the Croatian Science Foundation under the projects IP-2016-06-1316 “Plants as a source of bioactive sulphur compounds and their ability to hyperaccumulate metals” and IP-2014-09-6897 “Investigation of bioactive compounds from Dalmatian plants: their antioxidant, enzyme inhibition and health properties”.
Disclosure statement
No potential conflict of interest was reported by the authors.
Additional information
Funding
References
- Burčul F, Radan M, Politeo O, Blažević I. Cholinesterase-inhibitory activity of essential oils. In: Taylor JC, ed. Advances in chemistry research. Vol. 37. New York: Nova Science Publishers Inc.; 2017:1–71.
- Gulçin İ, Abbasova M, Taslimi P, et al. Synthesis and biological evaluation of aminomethyl and alkoxymethyl derivatives as carbonic anhydrase, acetylcholinesterase and butyrylcholinesterase inhibitors. J Enzym Inhib Med Chem 2017;32:1174–82.
- Akıncıoğlu A, Kocaman E, Akıncıoğlu H, et al. The synthesis of novel sulfamides derived from β-benzylphenethylamines as acetylcholinesterase, butyrylcholinesterase and carbonic anhydrase enzymes inhibitors. Bioorg Chem 2017;74:238–50.
- Ayaz M, Junaid M, Ullah F, et al. Comparative chemical profiling, cholinesterase inhibitions and anti-radicals properties of essential oils from Polygonum hydropiper L: a preliminary anti- Alzheimer's study. Lipids Health Dis 2015;14:141.
- Blažević I, Montaut S, Burčul F, Rollin P. Glucosinolates: novel sources and biological potential. In: Mérillon J-M, Ramawat GK, eds. Glucosinolates. Cham: Springer International Publishing; 2016:1–58.
- Öztaskın N, Taslimi P, Maraş A, et al. Novel antioxidant bromophenols with acetylcholinesterase, butyrylcholinesterase and carbonic anhydrase inhibitory actions. Bioorg Chem 2017;74:104–14.
- Valgimigli L, Iori R. Antioxidant and pro-oxidant capacities of ITCs. Environ Mol Mutagen 2009;50:222–37.
- Barillari J, Canistro D, Paolini M, et al. Direct antioxidant activity of purified glucoerucin, the dietary secondary metabolite contained in rocket (Eruca sativa Mill.) seeds and sprouts. J Agric Food Chem 2005;53:2475–82.
- Papi A, Orlandi M, Bartolini G, et al. Cytotoxic and antioxidant activity of 4-methylthio-3-butenyl isothiocyanate from Raphanus sativus L. (Kaiware Daikon) sprouts. J Agric Food Chem 2008;56:875–83.
- Subedi L, Venkatesan R, Kim S. Neuroprotective and anti-inflammatory activities of allyl isothiocyanate through attenuation of JNK/NF-κB/TNF-α signaling. Int J Mol Sci 2017;18:1–16.
- Prawan A, Saw CLL, Khor TO, et al. Anti-NF-κB and anti-inflammatory activities of synthetic isothiocyanates: effect of chemical structures and cellular signaling. Chem-Biol Interact 2009;179:202–11.
- Jaja-Chimedza A, Graf BL, Simmler C, et al. Biochemical characterization and anti-inflammatory properties of an isothiocyanate-enriched moringa (Moringa oleifera) seed extract. PLoS One 2017;12:e0182658.
- Wagner AE, Boesch-Saadatmandi C, Dose J, et al. Anti-inflammatory potential of allyl-isothiocyanate–role of Nrf2, NF-κB and microRNA-155. J Cell Mol Med 2012;16:836–43.
- Zhang Y. Allyl isothiocyanate as a cancer chemopreventive phytochemical. Mol Nutr Food Res 2010;54:127–35.
- Xiang J, Alesi GN, Zhou N, et al. Protective effects of isothiocyanates on blood-CSF barrier disruption induced by oxidative stress. Am J Physiol-Regul Integr Comp 2012;303:R1–7.
- Dinkova-Kostova AT, Kostov RV. Glucosinolates and isothiocyanates in health and disease. Trends Mol Med 2012;18:337–47.
- Karkoula E, Skantzari A, Melliou E, et al. Direct measurement of oleocanthal and oleacein levels in olive oil by quantitative 1H NMR. Establishment of a new index for the characterization of extra virgin olive oils. J Agric Food Chem 2012;60:11696–703.
- Katalinic V, Mozina Smole S, Generalic I, et al. Phenolic profile, antioxidant capacity, and antimicrobial activity of leaf extracts from six Vitis vinifera L. varieties. Int J Food Prop 2013;16:45–60.
- Prior RL, Hoang H, Gu L, et al. Assays for hydrophilic and lipophilic antioxidant capacity (oxygen radical absorbance capacity (ORACFL)) of plasma and other biological and food samples. J Agric Food Chem 2003;51:3273–9.
- Generalic Mekinic I, Blazevic I, Mudnic I, et al. Sea fennel (Crithmum maritimum L.): phytochemical profile, antioxidative, cholinesterase inhibitory and vasodilatory activity. J Food Sci Technol Mysore 2016;53:3104–12.
- Mastelić J, Jerković I, Blažević I, et al. Comparative study on the antioxidant and biological activities of carvacrol, thymol, and eugenol derivatives. J Agric Food Chem 2008;56:3989–96.
- Benzie IFF, Strain JJ. The ferric reducing ability of plasma (FRAP) as a measure of “antioxidant power”: the FRAP assay. Anal Biochem 1996;239:70–6.
- Mekinic IG, Burcul F, Blazevic I, et al. Antioxidative/acetylcholinesterase inhibitory activity of some Asteraceae plants. Nat Prod Commun 2013;8:471–4.
- Montaut S, Benson HJ, Kay M, et al. Probing the free-radical scavenging activity of the extract, the major glucosinolate and isothiocyanate of Eruca sativa Mill. and Lepidium densiflorum Schrad. seeds. J Food Compos Anal 2017;61:52–8.
- Benati L, Calestani G, Leardini R, et al. Cascade radical reactions via α-(arylsulfanyl)imidoyl radicals: competitive [4 + 2] and [4 + 1] radical annulations of alkynyl isothiocyanates with aryl radicals. J Org Chem 2003;68:3454–64.
- Benati L, Leardini R, Minozzi M, et al. Cascade radical reaction of 2-alkynyl-substituted aryl radicals with aryl isothiocyanates: a novel entry to benzothieno[2,3-b]quinolines through alpha-(arylsulfanyl)imidoyl radicals. J Org Chem 2000;65:8669–74.
- Leardini R, Nanni D, Pareschi P, et al. Alpha-(arylthio)imidoyl radicals: [3 + 2] radical annulation of aryl isothiocyanates with 2-cyano-substituted aryl radicals. J Org Chem 1997;62:8394–9.
- Yuan H, Yao S, You Y, et al. Antioxidant activity of isothiocyanate extracts from broccoli. Chin J Chem Eng 2010;18:312–21.
- Sarkar R, Mukherjee S, Biswas J, et al. Phenethyl isothiocyanate, by virtue of its antioxidant activity, inhibits invasiveness and metastatic potential of breast cancer cells: HIF-1α as a putative target. Free Radic Res 2016;50:84–100.
- Dávalos A, Gómez-Cordovés C, Bartolomé B. Extending applicability of the oxygen radical absorbance capacity (ORAC-fluorescein) assay. J Agric Food Chem 2004;52:48–54.
- Prior RL, Wu X, Schaich K. Standardized methods for the determination of antioxidant capacity and phenolics in foods and dietary supplements. J Agric Food Chem 2005;53:4290–302.
- Chen J, Hu L, Hu G, et al. An application of chemical oscillation: distinguishing two isomers between cyclohexane-1,3-dione and 1,4-cyclohexanedione. Electrochim Acta 2016;195:223–9.
- Bhattacharya A, Li Y, Shi Y, et al. Enhanced inhibition of urinary bladder cancer growth and muscle invasion by allyl isothiocyanate and celecoxib in combination. Carcinogenesis 2013;34:2593–9.