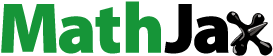
Abstract
Herein, we report the synthesis of different novel sets of coumarin-6-sulfonamide derivatives bearing different functionalities (4a, b, 8a–d, 11a–d, 13a, b, and 15a–c), and in vitro evaluation of their growth inhibitory activity towards the proliferation of three cancer cell lines; HepG2 (hepatocellular carcinoma), MCF-7 (breast cancer), and Caco-2 (colon cancer). HepG2 cells were the most sensitive cells to the influence of the target coumarins. Compounds 13a and 15a emerged as the most active members against HepG2 cells (IC50 = 3.48 ± 0.28 and 5.03 ± 0.39 µM, respectively). Compounds 13a and 15a were able to induce apoptosis in HepG2 cells, as assured by the upregulation of the Bax and downregulation of the Bcl-2, besides boosting caspase-3 levels. Besides, compound 13a induced a significant increase in the percentage of cells at Pre-G1 by 6.4-folds, with concurrent significant arrest in the G2-M phase by 5.4-folds compared to control. Also, 13a displayed significant increase in the percentage of annexin V-FITC positive apoptotic cells from 1.75–13.76%. Moreover, QSAR models were established to explore the structural requirements controlling the anti-proliferative activities.
Introduction
Cancer is a very complex, widespread and lethal disease that affects approximately 14 million people every year. In 2018, the American Cancer Society predict that the new diagnosed cancer cases will reach to 1,735,350 and cancer death 595,690 cancer deaths in the United StatesCitation1. Accordingly, the discovery of new anti-cancer agents with promising bioactivity and high therapeutic index is still an urgent need and a major challenge for researchers.
It is well established that natural and synthetic coumarin derivatives have attracted a great deal of interest due to their variety of biological and pharmacological properties, such as their anti-inflammatoryCitation2, antibacterialCitation3, antiviralCitation4, and anti-cancer activitiesCitation5. In the current medical era, much attention has been extensively paid to modify and update coumarin-based drug leads from the point of view of drug design and medicinal chemistry to fulfill more effective and safe anti-cancer agentsCitation6–8.
The promising biological profile of coumarins as anti-cancer agents, and their easy synthetic modifications paved the way for design and synthesis of various coumarin-based derivatives with diverse activities against cancer. In this context, many coumarin-based molecules were widely reported to possess anti-cancer activity through binding to different targets and diverse pharmacological mechanisms, to name just a few, selective estrogen receptor modulationCitation9–11 (as CHF4227, ), MEK1 inhibitionCitation12–14 (as G8935, ), steroid sulfatase inhibitionCitation15–17 (as Irosustat, ), CDC25 phosphatases inhibitionCitation18–20 (as SV37, ), tubulin polymerization inhibitionCitation21–23, aromatase inhibitionCitation24,Citation25, COX-2 inhibitionCitation26, and apoptosis inductionCitation27–31.
Figure 1. Structure of some reported coumarins and sulfonamides with effective anti-cancer activities.
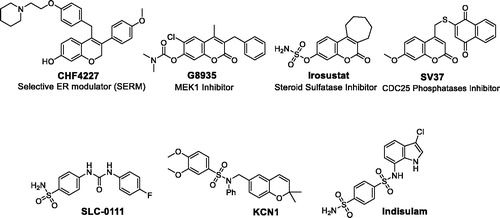
On the other hand, sulfonamide derivatives constitute another important class of organic compounds that displayed interesting biological activities including anti-carbonic anhydrase and anti-cancer activitiesCitation32,Citation33. It is noteworthy to mention that there is a continuing interest in the synthesis of coumarin sulfonamide derivatives as potent anti-cancer agentsCitation34–37. SLC-0111, , an ureido benzenesulfonamide derivative, is currently in phase I/II clinical trials as anti-cancer drug. SLC-0111 II is characterized by its selectivity towards inhibition of the transmembrane isoforms hCA IX/XII (over the cytosolic isoforms hCA I/II). SLC-0111 was also able to block human breast cancer invasion, delay tumor growth and diminish the cancer stem cell population in vivoCitation38–40.
KCN1, , a novel synthetic sulfonamide, is a HIF pathway inhibitor with in vitro and in vivo anti-pancreatic cancer activities and preclinical pharmacology. KCN1 specifically inhibited HIF reporter gene activity in several glioma cell lines at the nanomolar level. Also, KCN1 effectively inhibited the growth of subcutaneous malignant glioma tumor xenografts with low side effects on the hostCitation41,Citation42. Moreover, Indisulam (N-(-3-chloro-7-indolyl)-1,4-benzenedisulfonamide), , is an orally active sulfonamide anti-tumor agent that possesses anti-cancer properties through down‐regulation of various cell‐cycle checkpoint molecules, thereby blocking the phosphorylation of retinoblastoma protein and inducing p53 and p21. Pre-clinical and clinical studies have established synergy of Indisulam with nucleoside analogs as well as topoisomerase inhibitorsCitation43–47.
Based on the aforementioned findings, herein we report the synthesis of novel sets of coumarin sulfonamide derivatives (4a, b, 8a–d, 11a–d, 13a, b and 15a–c) and their in vitro growth inhibitory activity towards the proliferation of three cancer cell lines; HepG2 (hepatocellular carcinoma), MCF-7 (breast cancer), and Caco-2 (colon cancer). Additionally, the synthesized coumarin sulfonamides were further examined regarding their potential apoptotic induction and their effects on cell cycle progression in the Hep-G2 cancer cells to acquire a perception for the mechanism of their anti-cancer activity.
Materials and methods
Chemistry
Melting points were determined on Electrothermal IA 9000 apparatus and were uncorrected. Elemental microanalyses were performed on Elementar, Vario EL, at the Micro-analytical Laboratory, National Research Centre, Dokki, Cairo. The 1H NMR and 13C NMR spectra were recorded with a BrukerAvance 400 MHz spectrometer at Turku University, Finland and JEOL ACA 500 NMR spectrometer, at the National Research Centre, Dokki, Cairo, Egypt. The mass spectra were performed on Mass Spectrometer Finnigan MAT SSQ-7000 and GCMS-QP 1000EX Shimadzu Gas Chromatography MS Spectrometer at Faculty of Science, Cairo University, Egypt. The reactions were followed by TLC (silica gel, aluminum sheets 60 F254, Merck) using chloroform/methanol (9.5:0.5 v/v) as eluent.
Synthesis of coumarin-6-sulfonyl chloride 2
Compound 2-oxo-2H-chromene-6-sulfonyl chloride 2 was obtained from a reaction of coumarin with chlorosulfonic acidCitation48.
Synthesis of coumarin-6-sulfonamide derivatives 4a,b
A solution of 2-oxo-2H-chromene-6-sulfonyl chloride 2 (0.5 g, 2 mmol) and the appropriate sulfanilamide 3a or sulfapyridine 3b (2 mmol) in absolute ethanol (25 ml) was refluxed for 12 h. The obtained solid was filtered, dried, and recrystallized from ethanol to give compounds 4a, b.
2-Oxo-N-(4-sulfamoylphenyl)-2H-chromene-6-sulfonamide (4a)
Brown crystals, mp 266–268 °C, yield (43%). 1H NMR (500 MHz, DMSO-d6) δ ppm: 6.47 (d, H, J = 9.5 Hz, H3 of coumarin), 6.94 (d, 2H, J = 8.2 Hz, Ar), 7.31 (d, 1H, J = 8.5 Hz, H8 of coumarin), 7.61 (d, 2H, J = 8.4 Hz, Ar), 7.71–7.71 (m, 3H, NH2 and H7 of coumarin), 7.95 (d, 1H, J = 10 Hz, H4 of coumarin), 8.11 (s, 1H, H5 of coumarin), 10.95 (s, 1H, NH); 13C NMR (100 MHz, DMSO-d6); δ ppm: 116.32(2C), 116.90, 118.40, 118.84, 125.96, 125.97, 127.87 (2C), 129.81 (2C), 137.55, 144.94 (C4 of coumarin), 153.88 (C9 of coumarin), 160.40 (C=O of coumarin); MS m/z [%]: 380 [9.21], 92 [100]. Analysis for C15H12N2O6S2 (380), Calcd.: % C, 47.36; H, 3.18; N, 7.36; O, 25.24; S, 16.86. Found: % C, 47.28; H, 3.22; N, 7.42; O, 25.18; S, 16.78.
2-Oxo-N-(4-(N-(pyridin-2-yl)sulfamoyl)phenyl)-2H-chromene-6-sulfonamide (4b)
White crystals, mp 237–239 °C, yield (40%). 1H NMR (400 MHz, DMSO-d6) δ ppm: 6.62 (d, 1H, J = 9.6 Hz, H3 of coumarin), 6.83 (t, 1H, J = 12.4 Hz, H3 of pyridine), 7.08 (d, 1H, J = 8.8 Hz, H5 of pyridine), 7.24 (d, 2H, J = 8.8 Hz, Ar), 7.55 (d, 1H, J = 8.8 Hz, H8 of coumarin), 7.67 (t, 1H, J = 8.8 Hz, H4 of pyridine), 7.75 (d, 2H, J = 8.8 Hz, Ar), 7.94–7.96 (m, 2H, H2 of pyridine and H7 of coumarin), 8.15 (d, 1H, J = 10 Hz, H4 of coumarin), 8.28 (s, 1H, H5 of coumarin), 11.05 (s, 1H, NH, D2O exchangeable), 11.92 (s, 1H, NH, D2O exchangeable); 13 C NMR (100 MHz, DMSO); δ ppm: 114.23 (C5 of pyridine), 118.42 (2C), 118.99 (2C), 119.49, 128.29, 128.66, 128.55, 129.88, 129.96, 135.38, 137.18, 140.90, 141.01, 141.29, (Ar, pyridine and coumarin), 143.76 (C4 of coumarin), 153.57 (C=N of pyridine), 156.51 (C9 of coumarin), 159.54 (C=O of coumarin); Analysis for C20H15N3O6S2 (457), Calcd.: % C, 52.51; H, 3.30; N, 9.19; O, 20.98; S, 14.02. Found: % C, 52.58; H, 3.40; N, 9.22; O, 21.01; S, 14.09.
Synthesis of N-(4-acetylphenyl)-2-oxo-2H-chromene-6-sulfonamide (6)
Compound 6 was prepared according to the literatures proceduresCitation49.
Synthesis of schiff base coumarin sulfonamides 8a-d
To a mixture of compound 6 (0.25 g, 0.729 mmol) and the appropriate phenylhydrazine 7a, 2,4-dinitrophenylhydrazine 7b, 2,4,6-trichlorophenylhydrazine 7c or p-toluenesulfonyl hydrazide 7d (0.911 mmol) in absolute ethanol (30 ml), three drops of glacial acetic acid was added and the reaction mixture was refluxed for 8 h. After cooling, the precipitate was filtered, washed with water, purified via crystallization from ethanol to give compounds 8a–d, respectively.
2-Oxo-N-(4–(1-(2-phenylhydrazono)ethyl)phenyl)-2H-chromene-6-sulfonamide (8a)
Brown crystals, mp 178–180 °C, yield (66%). 1H NMR (500 MHz, DMSO-d6) δ ppm: 2.19 (s, 3H, CH3), 6.47 (d, 1H, J = 12 Hz, H3 of coumarin), 6.87–7.83 (m, 11H, NH, Ar, and H8 of coumarin), 7.95 (dd, 1H, J = 6 and 10.5 Hz, H7 of coumarin), 8.04 (d, 1H, J = 2.4 Hz, H4 of coumarin), 8.23 (s, 1H, H5 of coumarin), 11.09 (s, 1H, NH); 13C NMR (125 MHz, DMSO); δ ppm: 13.10 (CH3), 113.21 (2C), 113.25, 118.59, 119.25, 120.47, 122.66, 126.50, 128.18, 128.29, 129.32 (2C), 129.40, 130.33 (2C), 132.62, 135.93, 135.95, 143.81 (C4 of coumarin), 154.54 (C=N), 156.31 (C9 of coumarin), 160.02 (C=O of coumarin); HRMS (ESI): m/z calculated for C23H19N3O4S [M + H]+, 434.1169; found, 434.1163.
N-(4–(1-(2–(2,4-dinitrophenyl)hydrazono)ethyl)phenyl)-2-oxo-2H-chromene-6-sulfonamide (8b)
Red crystals, mp 269–270 °C, yield (60%). 1H NMR (500 MHz, DMSO-d6) δ ppm: 2.36 (s, 3H, CH3), 5.72 (s, 1H, NH), 6.61 (d, 1H, J = 6.50 Hz, H3 of coumarin), 7.21(d, 2H, J = 11 Hz, Ar), 7.57–7.85 (m, 3H, Ar, and H8 of coumarin), 7.96 (dd, 1H, J = 2.5 and 2.5 Hz, H7 of coumarin), 8.03 (d, 1H, J = 12 Hz, H4 of coumarin), 8.19 (d, 1H, J = 12 Hz, Ar), 8.29 (s, 1H, H5 of coumarin), 8.36 (d, 1H, J = 12 Hz, NO2-CH, Ar), 8.87 (s, 1H, H3 of 2,4-(NO2)2C6H3), 11.06 (s, H, NH); 13 C NMR (125 MHz, DMSO-d6); δ ppm: 13.83 (CH3), 118.26, 118.29, 118.38, 118.42, 118.60, 119.41, 119.50, 120.01, 127.26, 128.18, 128.29, 130.00, 130.05, 130.31, 132.60, 135.88, 138.33, 142.38, 143.86 (C4 of coumarin), 154.37 (C=N), 156.34 (C9 of coumarin), 159.56 (C=O of coumarin); Analysis for C23H17N5O8S (523), Calcd.: % C, 52.77; H, 3.27; N, 13.38; O, 24.45; S, 6.13. Found: % C, 52.84; H, 3.20; N, 13.32; O, 24.40; S, 6.18.
2-Oxo-N-(4–(1-(2–(2,4,6-trichlorophenyl)hydrazono)ethyl)phenyl)-2H-chromene-6-sulfonamide (8c)
White crystals, mp 259–260 °C, yield (58%). 1H NMR (500 MHz, DMSO-d6) δ ppm: 2.21 (s, 3H, CH3), 6.55 (d, 1H, J = 12 Hz, H3 of coumarin), 7.11 (d, 2H, J = 10.5 Hz, Ar), 7.54–7.60 (m, 3H, Ar, and H8 of coumarin), 7.64 (s, 2H, H3 and H5 of 2,4,6-(Cl)3C6H2), 7.92 (dd, 1H, J = 2 and 2 Hz, H7 of coumarin), 8.04 (s, 1H, NH), 8.16 (d, 1H, J = 12.5 Hz, H4 of coumarin), 8.22 (s, 1H, H5 of coumarin), 10.51 (s, 1H, NH); 13 C NMR (125 MHz, DMSO-d6); δ ppm: 12.98 (CH3), 118.23, 118.29, 118.36, 119.39, 120.21 (2C), 126.78 (2C), 128.06, 128.17, 128.86, 129.14 (2C), 130.03, 134.92, 135.86, 137.80, 139.14, 143.85 (C4 of coumarin), 145.64 (C=N), 156.33 (C9 of coumarin), 159.54 (C=O of coumarin); Analysis for C23H16Cl3N3O4S (534), Calcd.: % C, 51.46; H, 3.00; Cl, 19.81; N, 7.83; O, 11.92; S, 5.97. Found: % C, 51.49; H, 2.96; Cl, 19.85; N, 7.85 O, 11.87; S, 5.92.
2-Oxo-N-(4–(1-(2-tosylhydrazono)ethyl)phenyl)-2H-chromene-6-sulfonamide (8d)
White crystals, mp 163–165 °C, yield (76%). 1H NMR (400 MHz, DMSO-d6) δ ppm: 2.03 (s, 3H, CH3), 2.31 (s, 3H, CH3 - tosyl), 6.60 (d, 1H, J = 9.6 Hz, H3 of coumarin), 7.11 (d, 2H, J = 8.4 Hz, Ar), 7.39 (d, 2H, J = 8.4 Hz, Ar-tosyl), 7.50–7.56 (m, 3H, Ar, and H8 of coumarin), 7.78 (d, 2H, J = 8.4 Hz, Ar-tosyl), 7.91 (dd, 1H, J = 2 and 2 Hz, H7 of coumarin), 8.17 (d, 1H, J = 9.6 Hz, H4 of coumarin), 8.18 (s, 1H, H5 of coumarin), 10.44 (s, 1H, NH), 10.56 (s, 1H, NH); 13 C NMR (100 MHz, DMSO); δ ppm: 14.47 (CH3), 21.47 (CH3), 118.30, 118.45, 118.53, 119.42, 119.75, 125,77, 127.50, 128.04 (2C), 128.18, 129.91, 129.97 (2C), 133.45, 135.70, 136.57, 138.90, 143.79, 143.83 (C4 of coumarin), 152.92 (C=N), 156.37 (C9 of coumarin), 159.56 (C=O of coumarin); Analysis for C24H21N3O6S2 (511), Calcd.: % C, 56.35; H, 4.14; N, 8.21; O, 18.77; S, 12.54. Found: % C, 56.40; H, 4.08; N, 8.16; O, 18.71; S, 12.50.
2–(1-(4–(2-Oxo-2H-chromene-6-sulfonamido)phenyl)ethylidene)hydrazine carbothioamide (9)
A mixture of N-(4-acetylphenyl)-2-oxo-2H-chromene-6-sulfonamide 6 (0.5 g, 1.45 mmol), and thiosemicarbazide (1.45 mmol) in absolute ethanol (20 ml) and catalytic amount of acetic acid was refluxed for 6 h. The obtained solid was filtered, dried, and washed with hot ethanol to afford compound 9.
Light yellow crystals, mp 253–254 °C, yield (34%). 1H NMR (400 MHz, DMSO-d6) δ ppm: 2.18 (s, 3H, CH3), 6.58 (d, 1H, J = 4.4 Hz, H3 of coumarin), 7.25 (d, 2H, J = 6.8 Hz, Ar), 7.55 (d, 1H, J = 2.4 Hz, H8 of coumarin), 7.79–7.84 (m, 4H, NH2, Ar), 7.94 (dd, 1H, J = 6 and 1.6 Hz, H7 of coumarin), 8.16 (d, 1H, J = 10 Hz, H4 of coumarin), 8.29 (d, 1H, J = 2.4 Hz, H5 of coumarin), 10.13 (s, 1H, NH), 10.60 (s, 1H, NH); 13 C NMR (100 MHz, DMSO-d6); δ ppm: 14.17 (CH3), 113.57, 118.30, 118.34, 119.43 (2C), 119.69, 128.14 (2C), 130.01, 133.82, 135.86, 138.72, 143.82 (C4 of coumarin), 147.53 (C=N), 156.36 (C9 of coumarin), 159.54 (C=O of coumarin), 179.22 (C = S), Analysis for C18H16N4O4S2 (416), Calcd.: % C, 51.91; H, 3.87; N, 13.45; O, 15.37; S, 15.40. Found: %C, 51.79; H, 3.82; N, 13.31; O, 15.42; S, 15.47.
General procedures for preparation of target compounds (11a–d and 13a, b)
A mixture of thiosemicarbazone 9 (10 mmol) and the appropriate α-halocarbonyl compounds 10a–d, phenacyl bromide 12a or coumarin-3-acetylbromide 12 b (10 mmol) in dioxane (25 ml) containing catalytic amount of triethylamine was heated under reflux for 8 h and then cooled. The solution was poured onto water-ice and concentrated hydrochloric acid. The solid produced was collected by filtration and crystallized from ethanol to furnish compounds 11a–d and 13a, b, respectively.
N-(4–(1-(2–(4-methylthiazol-2-yl)hydrazono)ethyl)phenyl)-2-oxo-2H-chromene-6-sulfonamide (11a)
Brown crystals, mp 257–259 °C, yield (78%). 1H NMR (500 MHz, DMSO-d6) δ = 2.15 (s, 3H, CH3), 2.45 (s, 3H, CH3-thiazole), 5.99 (s, 1H, CH thiazole), 6.57 (d, 1H, J = 9.6 Hz, H3 of coumarin), 7.19 (d, 2H, J = 8.6 Hz, Ar), 7.52 (d, 1H, J = 8.6 Hz, H8 of coumarin), 7.80 (d, 2H, J = 8.6 Hz, Ar), 7.91 (dd, 1H, J = 9.5 and 2 Hz, H7 of coumarin), 8.12 (d, 1H, J = 10 Hz, H4 of coumarin), 8.27 (s, 1H, H5 of coumarin), 10.55 (s, 1H, NH, D2O exchangeable), 11.01 (s, 1H, NH, D2O exchangeable); 13 C NMR (125 MHz, DMSO); δ ppm: 13.83 (CH3), 14.55 (CH3-thiazole), 118.26, 118.42, 118.60, 119.41, 119.50, 120.01, 127.26, 128.18, 130.31, 132.60, 135.88, 138.33, 142.38 (Ar, C5-thiazole and coumarin), 143.86 (C4 of coumarin), 154.33 (C=N), 154.37 (C4-thiazole), 156.34 (C9 of coumarin), 159.56 (C=O of coumarin), 168.66 (S-C=N): Analysis for C21H18N4O4S2 (454), Calcd.: % C, 55.49; H, 3.99; N, 12.33; O, 14.08; S, 14.11. Found: % C, 55.40; H, 3.96; N, 12.36; O, 14.05; S, 14.14.
Ethyl 4-methyl-2–(2-(1–(4-(2-oxo-2H-chromene-6-sulfonamido)phenyl) ethylidene)hydrazinyl)thiazole-5-carboxylate (11b)
Yellow crystals, mp 180–182 °C, yield (53%). 1H NMR (500 MHz, DMSO-d6) δ ppm: 1.21 (t, 3H, J = 11.48 Hz, CH3), 2.19 (s, 3H, CH3), 2.41 (s, 3H, CH3-thiazole), 4.14 (q, 2H, J = 6.65 Hz, OCH2), 6.56 (d, 1H, J = 9.2 Hz, H3 of coumarin), 7.14 (d, 2H, J = 8.4 Hz, Ar), 7.53 (d, 1H, J = 8.6 Hz, H8 of coumarin), 7.63 (d, 2H, J = 8.4 Hz, Ar), 7.91 (dd, 1H, J = 9.6 and 5 Hz, H7 of coumarin), 8.14 (d, 1H, J = 9.6 Hz, H4 of coumarin), 8.22 (s, 1H, H5 of coumarin), 10.67 (s, 1H, NH), 11.04 (s, 1H, NH); 13 C NMR (125 MHz, DMSO-d6); δ ppm: 14.62 (CH3), 14.87 (CH3), 26.93 (CH3-ester), 60.69 (OCH2), 112.87, 118.34, 118.67, 119.48, 120.01, 127.51, 128.25, 130.13 (2C), 130.37, 135.91 (Ar, C5-thiazole and coumarin), 143.87 (C4 of coumarin), 152.41 (C=N), 156.41 (C4-thiazole), 156.53 (C9 of coumarin), 159.48 (C=O of coumarin), 169.66 (S-C=N), 185.45 (C=O); HRMS (ESI): m/z calculated for C24H22N4O4S2 [M + H]+, 527.1054; found, 527.1052.
N-(4–(1-(2–(5-((4-chlorophenyl)diazenyl)-4-methylthiazol-2-yl)hydrazono)ethyl) phenyl)-2-oxo-2H-chromene-6-sulfonamide (11c)
Red crystals, mp 250–252 °C, yield (88%). 1H NMR (500 MHz, DMSO-d6) δ ppm: 2.41 (s, 3H, CH3), 2.57(s, 3H, CH3-thiazole), 6.62 (d, 1H, J = 12 Hz, H3 of coumarin), 7.21 (d, 2H, J = 10.5 Hz, Ar), 7.35–7.39 (m, 4H, Ar), 7.59 (d, 1H, J = 8.6 Hz, H8 of coumarin), 7.85 (d, 2H, J = 8.8 Hz, Ar), 7.97 (dd, 1H, J = 3 and 3 Hz, H7 of coumarin), 8.19 (d, 1H, J = 12 Hz, H4 of coumarin); 8.30 (s, 1H, H5 of coumarin), 10.65 (br, 2H, 2NH,); 13 C NMR (125 MHz, DMSO) δ ppm: 14.55 (CH3), 15.50 (CH3), 118.35, 118.38, 118.64, 119.47 (2C), 119.98, 123.52, 124.53, 128.22, 128.54, 129.64 (2C), 129.99, 130.04, 130.31, 133.07, 135.92, 139.30, 140.32 (Ar, C5-thiazole and coumarin), 143.84 (C4 of coumarin), 147.89 (C4-thiazole), 151.37 (C=N), 156.42 (C9 of coumarin), 159.54 (C=O of coumarin), 171.80 (S-C=N); Analysis for C27H21ClN6O4S2 (592), Calcd.: % C, 54.68; H, 3.57; Cl, 5.98; N, 14.17; O, 10.79; S, 10.81. Found: % C, 54.61; H, 3.52; N, 14.20; O, 10.71; S, 10.70.
N-(4–(1-(2–(4-methyl-5-(p-tolyldiazenyl)thiazol-2yl)hydrazono)ethyl)phenyl)-2-oxo-2H-chromene-6-sulfonamide (11d)
Red crystals, mp 258–259 °C, yield (83%). 1H NMR (500 MHz, DMSO-d6) δ ppm: 2.25 (s, 3H, CH3), 2.41 (s, 3H, CH3), 2.56 (s, 3H, CH3-thiazole), 6.62 (d, 1H, J = 12 Hz, H3 of coumarin), 7.13 (d, 2H, J = 10 Hz, Ar), 7.20–7.25 (m, 4H, Ar), 7.59 (d, 1H, J = 11 Hz, H8 of coumarin), 7.85 (d, 2H, J = 10.5 Hz, Ar), 7.97 (dd, 1H, J = 3 and 3 Hz, H7 of coumarin), 8.19 (d, 1H, J = 12 Hz, H4 of coumarin), 8.29 (s, 1H, H5 of coumarin), 10.47 (s, 1H, NH), 10.77 (s, 1H, NH); 13 C NMR (125 MHz, DMSO); δ ppm: 15.48 (CH3), 16.94 (CH3), 20.85 (CH3), 114.68, 118.39, 118.60, 119.47 (2C), 128.23, 128.28, 128.52, 129.99, 130.04, 130.20, 130.33, 131.65, 133.26, 135.63, 135.83, 137.96, 140.10, 141.61, 142.35 (Ar, thiazole and coumarin), 143.83 (C4 of coumarin), 151.15 (C=N), 156.44 (C9 of coumarin), 159.54 (C=O of coumarin), 171.79 (S-C=N); Analysis for C28H24N6O4S2 (572), Calcd.: % C, 58.73; H, 4.22; N, 14.68; O, 11.18; S, 11.20. Found: % C, 58.82; H, 4.14; N, 14.77; O, 11.19; S, 11.16.
2-Oxo-N-(4–(1-(2–(4-phenylthiazol-2-yl)hydrazono)ethyl)phenyl)-2H-chromene-6-sulfonamide (13a)
Yellow crystals, mp 170–171 °C, yield (80%). 1H NMR (500 MHz, DMSO-d6) δ ppm: 2.19 (3H, s, CH3), 6.56 (d, 1H, J = 5.75 Hz, H3 of coumarin), 7.16 (d, 2H, J = 8.8 Hz, Ar), 7.27–7.36 (m, 5H, Ar), 7.54 (d, 1H, J = 8.65 Hz, H8 of coumarin), 7.61 (d, 2H, J = 8.4 Hz, Ar), 7.94 (dd, 1H, J = 2.4 and 8 Hz, H7 of coumarin), 8.15(d, 1H, J = 8 Hz, H4 of coumarin), 8.20 (s, 1H-thiazole), 8.29 (d, 1H, J = 2.5 Hz, H5 of coumarin), 10.59 (s, 1H, NH), 10.99 (s, 1H, NH); 13C NMR (125 MHz, DMSO-d6); δ ppm: 14.29 (CH3), 104.44 (C4-thiazole), 118.29, 118.58, 119.43, 120.11, 125.96, 128.20, 128.29, 129.06 (2C), 130.05, 130.33, 132.61, 134.20, 135.21, 135.61, 135.86, 138.26, 142.35 (Ar, and coumarin), 143.86 (C4 of coumarin), 146.27 (C5-thiazole), 156.36 (C=N), 156.50 (C9 of coumarin), 159.56 (C=O of coumarin), 170.21 (S-C=N);. MS m/z [%]: 516 [93], 132 [100]; Analysis for C26H20N4O4S2 (516), Calcd.: % C, 60.45; H, 3.90; N, 10.85; O, 12.39; S, 12.41 Found: % C, 60.39; H, 3.88; N, 10.87; O, 12.33; S, 12.36.
2-Oxo-N-(4–(1-(2–(4-(2-oxo-2H-chromen-3-yl)thiazol-2-yl)hydrazono)ethyl)phenyl)-2H-chromene-6-sulfonamide (13b)
Yellow crystals, mp 201–202 °C, yield (63%). 1H NMR (500 MHz, DMSO-d6) δ ppm: 2.16 (s, 3H, CH3), 6.58 (d, 1H, J = 9.3 Hz, H3 of coumarin), 7.20 (d, 2H, J = 8.1 Hz, Ar), 7.34–8.80 (m, 7H, 8H coumarin and Ar), 7.97 (dd, 1H, J = 8.6 and 9.5 Hz, H7 of coumarin), 8.11 (s, 1H, H4 of coumarin), 8.15 (d, 1H, J = 9.5 Hz, H4 of coumarin), 8.28 (d, 1H, J = 8.6 Hz, H5 of coumarin), 8.52 (s, 1H-thiazole), 10.60 (s, 1H, NH); 10.99 (s, 1H, NH); 13 C NMR (125 MHz, DMSO-d6); δ ppm: 14.32 (CH3), 111.46 (C4-thiazole), 116.36, 118.30, 118.43, 118.58 (2C), 119.50, 120.05, 125.20, 127.17, 128.29, 129.20 (2C), 129.98, 130.33 (2C), 132.61, 134.03, 135.61, 135.84, 142.35, 143.80 (C4 of coumarin), 152.75 (C=N), 156.36 (C5-thiazole), 156.50 (2C9 of coumarin), 159.51 (2C=O of coumarin), 169.67 (S-C=N); Analysis for C29H20N4O6S2 (584), Calcd.: % C, 59.58; H, 3.45; N, 9.58; O, 16.42; S, 10.97. Found: % C, 59.52; H, 3.48; N, 9.56; O, 16.36; S, 10.94.
General procedures for synthesis of thiazolidinones (15a–c)
In 50 ml round-bottom flask, thiosemicarbazone 9 (10 mmol) was dissolved in 15 ml acetic acid, followed by addition of anhydrous sodium acetate (30 mmol) under magnetic stirring and warming. After 20 min, bromoacetic acid 14a, 2-bromopropanoic acid 14b or maleic anhydride 14c (15 mmol) was added and the reaction mixture was maintained under reflux for 8 h. After cooling, the precipitate was filtered, washed with water, dried, and recrystallized from ethanol to give compounds 15a–c, respectively.
2-Oxo-N-(4–(1-(2–(5-oxo-4,5-dihydrothiazol-2-yl)hydrazono)ethyl)phenyl)-2H-chromene-6-sulfonamide (15a)
Yellow crystals, mp 191–192 °C, yield (64%). 1H NMR (400 MHz, CDCl3) δ ppm: 2.23 (s, 3H, CH3), 3.8 (s, 2H, CH2, thiazolidinone), 6.62 (d, 1H, J = 10.8 Hz, H3 of coumarin), 7.25 (d, 2H, J = 12 Hz, Ar), 7.55 (d, 1H, J = 8.4 Hz, H8 of coumarin); 7.85 (d, 2H, J = 8.4 Hz, Ar), 7.95 (dd, 1H, J = 2.4 and 2 Hz, H7 of coumarin), 8.16 (d, 1H, J = 10 Hz, H4 of coumarin), 8.29 (s, 1H, H5 of coumarin), 10.66 (s, 1H, NH), 10.97 (s, 1H, NH); 13 C NMR (100 MHz, CDCl3); δ ppm: 15.33 (CH3), 56.30 (CH2), 118.39, 118.64 (2C), 119.72, 127.93, 128.26, 129.99 (2C), 130.30, 132.66, 135.87, 142.35, 143.79 (C4 of coumarin), 152.56 (C=N), 156.50 (C9 of coumarin), 159.48 (C=O of coumarin), 166.33 (S-C=N), 196.90 (C=O); Analysis for C20H16N4O5S2 (456), Calcd.: % C, 52.62; H, 3.53; N, 12.27; O, 17.52; S, 14.05. Found: % C, 52.58; H, 3.50; N, 12.19; O, 17.50; S, 14.00.
N-(4–(1-(2–(4-methyl-5-oxo-4,5-dihydrothiazol-2-yl)hydrazono)ethyl)phenyl)-2-oxo-2H-chromene-6-sulfonamide (15b)
Brown crystals, mp 180–182 °C, yield (50%). 1H NMR (400 MHz, CDCl3) δ ppm: 1.59 (d, 3H, J = 9 Hz, CH3 thiazolidinone), 2.27 (s, 3H, CH3), 3.96 (q, 1H, J = 9 Hz CH thiazolidinone), 5.23 (s, 1H, NH), 6.44 (d, 1H, J = 10 Hz, H3 of coumarin), 7.05 (d, 2H, J = 10.5 Hz, Ar), 7.30 (d, 1H, J = 10.5 Hz, H8 of coumarin), 7.61 (d, 1H, J = 12 Hz, H4 of coumarin), 7.69 (d, 2H, J = 10.5 Hz, Ar), 7.84 (dd, 1H, J = 5.5 and 3 Hz, H7 of coumarin), 7.95 (s, 1H, H5 of coumarin), 11.02 (s, 1H, NH); 13 C NMR (100 MHz, CDCl3); δ ppm: 13.70 (CH3), 18.17 (CH3), 41.47 (CH of thiazolidinone), 117.10, 117.49, 117.88, 119.85 (2C), 126.66, 127.03 (2C), 129.05, 134.25, 136.40, 140.69, 141.30 (C4 of coumarin), 148.51 (C=N), 155.60 (C9 of coumarin); 158.13 (C=O of coumarin), 172.21 (S-C=N), 180.55 (C=O); Analysis for C21H18N4O5S2 (470), Calcd.: % C, 53.61; H, 3.86; N, 11.91; O, 17.00; S, 13.63. Found: % C, 53.55; H, 3.83; N, 11.87; O, 16.98; S, 13.60.
2–(4-Oxo-2–(2-(1–(4-(2-oxo-2H-chromene-6-sulfonamido)phenyl)ethylidene) hydrazinyl)-4,5-dihydrothiazol-5-yl)acetic acid (15c)
White crystals, mp 193–194 °C, yield (40%). 1H NMR (400 MHz, CDCl3) δ ppm: 2.43 (s, 3H, CH3), 2.86 (t, 1H, J = 6.4 Hz CH thiazolidinone), 3.04 (d, 2H, J = 12.8 Hz CH2), 6.58 (d, 1H, J = 7.6 Hz, H3 of coumarin), 7.22 (d, 2H, J = 6.4 Hz, Ar); 7.53 (d, 1H, J = 7.2 Hz, H8 of coumarin), 7.82 (d, 2H, J = 6.4 Hz, Ar); 7.95 (dd, 1H, J =6.8 and 2 Hz, H7 of coumarin), 8.16 (d, 1H, J = 8 Hz, H4 of coumarin), 8.27 (s, 1H, H5 of coumarin), 10.99 (s, 1H, NH), 11.14 (s, 1H, NH), 13.21 (s, 1H, COOH); 13 C NMR (100 MHz, CDCl3); δ ppm: 16.36 (CH3), 38.04 (CH2), 42.56 (CH of thiazolidinone), 118.42, 118.59 (2C), 119.51, 128.29, 129.99 (2C), 130.32 (2C), 132.62, 135.63, 142.37, 143.37 (C4 of coumarin), 152.24 (C=N), 156.56 (C9 of coumarin), 159.51 (C=O of coumarin), 165.86 (S-C=N), 172.48 (C=O of COOH), 196.92 (C=O); Analysis for C22H18N4O7S2 (514), Calcd.: % C, 51.35; H, 3.53; N, 10.89; O, 21.77; S, 12.46. Found: % C, 51.30; H, 3.51; N, 10.86; O, 21.74; S, 12.44.
Biological evaluation
In vitro anti-proliferative activity
HepG2 liver cancer, MCF-7 breast cancer and Caco-2 cancer cell lines were obtained from the National Cancer Institute (Cairo, Egypt). Caco-2 cells were grown in DMEM while HepG2 and MCF-7 were grown in RPMI-1640. Media were supplemented with 10% heat-inactivated FBS, 50 units/mL of penicillin and 50 g/mL of streptomycin and maintained at 37 °C in a humidified atmosphere containing 5% CO2. The cells were maintained as a “monolayer culture” by serial subculturing. Cytotoxicity was determined using the sulforhodamine B (SRB) method as previously described by Skehan et al.Citation50 Exponentially growing cells were collected using 0.25% trypsin-EDTA and seeded in 96-well plates at 1000–2000 cells/well in supplemented DMEM medium. After 24 h, cells were incubated for 72 h with various concentrations of the tested compounds as well as doxorubicin as the reference compound. Following 72 h of treatment, the cells were fixed with 10% trichloroacetic acid for 1 h at 4 °C. Wells were stained for 10 min at room temperature with 0.4% SRB dissolved in 1% acetic acid. The plates were air dried for 24 h, and the dye was solubilized with Tris–HCl for 5 min on a shaker at 1600 rpm. The optical density (OD) of each well was measured spectrophotometrically at 564 nm with an ELISA microplate reader (ChroMate-4300, FL, USA). The IC50 values were calculated according to the equation for Boltzmann sigmoidal concentrationeresponse curve using the nonlinear regression models (GraphPad, Prism Version 5). The results reported are means of at least three separate experiments. Significant differences were analyzed by one-way ANOVA wherein the differences were considered to be significant at p < .05.
ELISA immunoassay
The levels of the apoptotic markers (Bax, caspase-3) and the anti-apoptotic marker (Bcl-2) were examined using ELISA colorimetric kits per the manufacturer’s protocol and referring to reported instructionsCitation51,Citation52.
Cell cycle analysis
The HepG2 cells were treated with compound 13a for 24 h (at IC50 concentration), then cells were washed twice with ice-cold phosphate-buffered saline (PBS). Subsequently, the treated cells were collected by centrifugation, fixed in ice-cold 70% (v/v) ethanol, washed with PBS, re-suspended with 0.1 mg/mL RNase, stained with 40 mg/mL PI, and analyzed by flow cytometry using FACS Calibur (Becton Dickinson, BD). The cell cycle distributions were calculated using CellQuest software (Becton Dickinson)Citation53.
Annexin V–FITC apoptosis assay
Phosphatidylserine externalization was measured using Annexin V-FITC/PI apoptosis detection kit (BD Biosciences, San Jose, CA) according to the manufacturer's instructions, as reported earlierCitation53. HepG2 cells were treated with 13a at defined concentrations for 24 h.
Results and discussion
Chemistry
The synthetic routes employed to prepare the new target derivatives are depicted in Schemes 1–4. The key intermediate 2-oxo-2H- chromene-6-sulfonyl chloride 2 was obtained from a reaction of coumarin 1 with chlorosulfonic acid, which subsequently reacted with sulfanilamide 3a and sulfapyridine 3 b in refluxing ethanol to furnish the corresponding target compounds 4a, b, respectively (Scheme 1).
Scheme 1. Synthesis of target compounds 4a, b; Reagents and conditions: (i) Heating 100 °C, 4 h; (ii) EtOH/reflux 12 h.
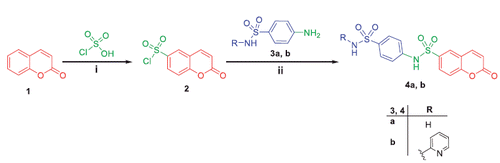
Scheme 2. Synthesis of target compounds 8a–d; Reagents and conditions: (i) Pyridine/stirring, rt 24h; (ii) EtOH/AcOH (catalytic)/reflux 8 h.
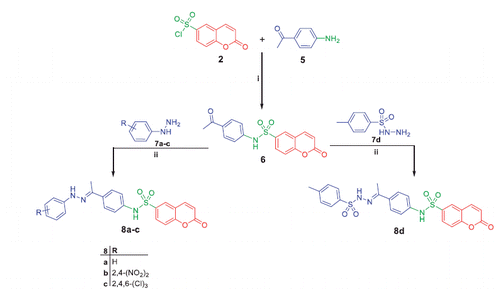
Scheme 3. Synthesis of target compounds 11a–d and 13a, b; Reagents and conditions: (i) EtOH/AcOH(catalytic)/reflux 6h (ii) Dioxane/TEA (catalytic)/reflux 8h.
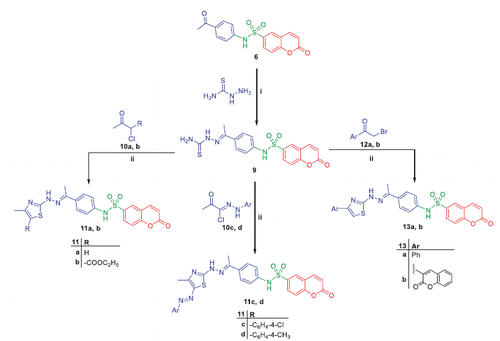
Scheme 4. Synthesis of target compounds 15a–c; Reagents and conditions: (i) AcOH/anhydrous AcONa/reflux 8h.
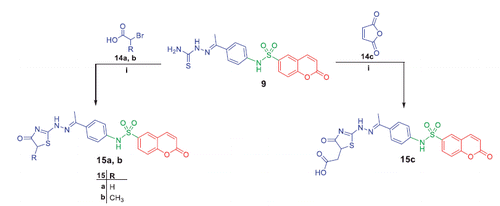
In Scheme 2, synthesis of N-(4-acetylphenyl)-2-oxo-2H-chromene-6-sulfonamide 6 was achieved via stirring compound 2 with 4-aminoacetophenone 5 in dichloromethane at room temperature. The later was refluxed with phenylhydrazine 7a, 2,4-dinitrophenylhydrazine 7b, 2,4,6-trichlorophenylhydrazine 7c and p-toluenesulfonyl hydrazide 7d in absolute ethanol to afford target coumarins 8a–d.
Refluxing of compound 6 with thiosemacarbazide in ethanol and catalytic amount of acetic acid yielded the key intermediate 9, which utilized for preparation of diverse derivatives. Treatment of intermediate 9 with the appropriate α-halocarbonyl compounds 10a–d in refluxing dioxane furnished the corresponding thiazole derivatives 11a–d. Additionally, refluxing of 9 with phenacyl bromide 12a and coumarin-3-acetylbromide 12b in dioxane afforded the corresponding thiazoles 13a, b, respectively (Scheme 3). Moreover, treatment of intermediate 9 with each of bromoacetic acid 14a, 2-bromopropanoic acid 14b and maleic anhydride 14c in acetic acid in the presence of anhydrous sodium acetate afforded the target thiazolidinone derivatives 15a–c, respectively (Scheme 4).
Postulated structures of the newly synthesized coumarin sulfonamides were in full agreement with their spectral and elemental analyses data (Supplementary material).
Biological evaluation
In vitro anti-proliferative activity
All the newly synthesized target coumarin sulfonamides were evaluated for their in vitro anti-proliferative activity against three human tumor cancer cell lines, HepG2 hepatocellular carcinoma, MCF-7 breast cancer, and Caco-2 colon cancer, using SRB colorimetric assay as described by Skehan et al.50 Doxorubicin was involved in the experiments as a reference cytotoxic compound. The results were expressed as growth inhibitory concentration (IC50) values which represent the compound concentrations required to produce a 50% inhibition of cell growth after 72 h of incubation compared to untreated controls. The results were summarized in .
Table 1. In vitro anti-proliferative activity of the target coumarin sulfonamide derivatives against HepG2, MCF-7 and Caco-2 cancer cell lines.
From the displayed results, it was obvious that several of the prepared coumarin sulfonamides possess excellent to modest growth inhibitory activity towards the tested cancer cell lines. Examinations of the anti-proliferative activity towards HepG2 cells revealed that it is the most sensitive cell line to the influence of the target coumarin sulfonamide derivatives. Compounds 13a and 15a emerged as the most active coumarins against HepG2 cells through this study with IC50 values of 3.48 ± 0.28 and 5.03 ± 0.39 µM, respectively. They displayed 1.5- and 1.1-fold increased potency than doxorubicin (IC50 = 5.43 ± 0.24 µM). Besides, compounds 4b and 15c with IC50 values of 8.08 ± 0.51 and 7.57 ± 0.66 µM, respectively, displayed excellent activity in comparison to doxorubicin. Also, compounds 8b and 13b (IC50 = 11.84 ± 1.34 and 11.80 ± 1.16 µM, respectively) exhibited good anti-proliferative activity. Moreover, compounds 6, 8a, 8c, and 15b had moderate activity with IC50 values ranging from 25.07 ± 2.08 to 29.80 ± 2.21 µM.
Concerning activity against MCF-7 cells, compounds 15a and 15b were the most active members that displayed potent anti-proliferative activity with IC50 values of 10.95 ± 0.96 and 10.62 ± 1.35 µM, respectively, in comparison to the standard drug doxorubicin (IC50 = 3.18 ± 0.32 µM). Moreover, compounds 8a, 11a, and 15c were moderately active against MCF-7 cells with IC50 values: 14.30 ± 1.18, 13.86 ± 1.19 and 16.32 ± 1.48 µM, respectively. On the other hand, anti-proliferative activity evaluation in Caco-2 cells showed that compound 8a possessed the best growth inhibitory activity (IC50 = 8.53 ± 0.72), with twofold decreased activity than doxorubicin (IC50 = 4.10 ± 1.37 µM). In addition, compound 11a showed good anti-proliferative activity against Caco-2 cancer cell line (IC50 = 10.12 ± 0.90). Whilst, both compounds 8d and 11d displayed moderate activity against Caco-2 cancer cell line with IC50 values: 16.02 ± 1.32 and 16.06 ± 1.28, respectively. These results suggest that diverse functionalities could be incorporated into the coumarin sulfonamide scaffold to obtain promising anti-proliferative activity against different types of cancer cells.
In vitro cytotoxicity towards human normal WI-38 cells
The cell growth inhibitory activity of the most potent compounds 13a and 15a was examined towards non-tumorigenic human normal lung fibroblast cell line (WI-38) to investigate the potential safety of the newly synthesized coumarin-6-sulfonamides towards the normal cells. The results were expressed as IC50 values, and selectivity index was calculated (). The tested coumarin-6-sulfonamides (13a and 15a) showed non-significant cytotoxic action with IC50 values of 73.20 ± 3.47 and 55.92 ± 0.39 µM, respectively, with good selectivity index range 21 and 11, thereby providing a high safety profile as anticancer agents.
Table 2. In vitro cytotoxic activity against normal WI-38 cells, and selectivity index of the most active coumarins.
Apoptosis induction in HepG2 cells
Apoptosis induction in cancer cells represents one of the most successful strategies for the development of cancer therapyCitation54–56. As displayed above, coumarins 13a and 15a emerged as the most active ones towards HepG2 liver cancer cells. Accordingly, we investigated the ability of compounds 13a and 15a to provoke apoptosis in HepG2 cells to define the principle mechanism for their anti-proliferative activity.
Effects on mitochondrial apoptosis pathway proteins Bcl-2 and Bax
The Bcl-2 proteins family is mainly responsible for synchronizing the mitochondrial apoptotic pathway, and classified into two major classes: anti-apoptotic proteins such as Bcl-2 protein and the counteracting pro-apoptotic proteins including Bax protein. In this study, we examined the impact of coumarins 13a and 15a on the level of the anti-apoptotic Bcl2 and the level of the pro-apoptotic Bax (). As shown in , compound 13a induced the protein expression of Bax with 16.5 folds of the control while 14.3 folds were recorded for compound 15a. On the other hand, treatment of HepG2 cells with compounds 13a and 15a significantly reduced the expression levels of the anti-apoptotic protein Bcl-2 by 21.4 and 38.6%, respectively, compared to the control.
Table 3. Effect of compounds 13a and 15a on the active caspase-3 level, and the expression levels of Bcl-2 and Bax in HepG2 cancer cells treated with each compound at its IC50 concentration.
Effects on the levels of active caspase-3 (key executor of apoptosis)
As a key executioner protease, caspase-3 is activated by upstream initiator caspases as caspase-9. Herein, treatment of HepG2 cells with coumarins 13a and 15a resulted in a significant elevation in the level of active caspase-3 by about 6.6 and 5.7 folds, respectively, compared to control ().
Cell-cycle analysis
The impact of compound 13a on cell cycle progression was examined in HepG2 cancer cell line after 24 h of treatment (). This impact was illustrated by DNA flow cytometric analysis where HepG2 cells were treated with 13a at concentration equals to its IC50. showed that exposure of HepG2 cells to compound 13a induced a significant increase in the percentage of cells at Pre-G1 by 6.4-folds, with concurrent significant arrest in the G2-M phase by 5.4-folds compared to control. Alteration of the Pre-G1 phase and arrest of G2-M phase were significant remarks for compound 13a to induce apoptosis in HepG2 cells.
Annexin V-FITC apoptosis assay
The apoptotic effect of coumarin 13a was further assured by Annexin V-FITC/PI (AV/PI) dual staining assay to investigate the occurrence of phosphatidylserine externalization (). Flow cytometric analysis revealed that HepG2 cells treated with 13a showed a significant increase in the percent of annexin V-FITC positive apoptotic cells (UR + LR) from 1.75% to 13.76% which comprises about 7.9 folds compared to control.
Figure 3. Effect of compound 13a on the percentage of annexin V-FITC-positive staining in HepG2 cells. The experiments were done in triplicates. The four quadrants identified as: LL, viable; LR, early apoptotic; UR, late apoptotic; UL, necrotic. *Significantly different from control at p < .05.
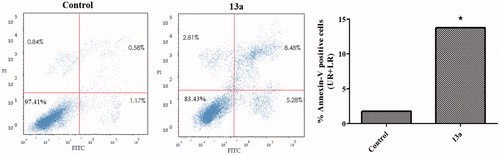
2D QSAR study
Development of QSAR models
With the aim to assess the structural basis for the anti-proliferative activity of the newly prepared coumarin sulfonamides (4a, b, 6, 8a–d, 9, 11a–d, 13a, b and 15a–c), 2D-QSAR analysis was carried out. This analysis was run by means of the DS 4.0 software (Discovery Studio 4.0, Accelrys, Co. Ltd)Citation57. A set of the newly synthesized compounds (4a, b, 6, 8a–d, 9, 11a–d, 13a, b and 15a–c) was used as training set with their measured pIC50 against HepG2 and Caco-2 for QSAR modeling, compounds 6, 8d, 15a and 15c were chosen as statistical outliers while building model for HepG2 whereas, compounds 6, 9, and 13a were chosen for the Caco-2 model.
“Calculate Molecular Properties” module was used for calculating the molecular properties. Genetic function approximation (GFA) protocol was applied in order to choose the best descriptors that characterize the activity. Multiple linear regression (MLR) protocol was then employed to search for optimal QSAR models with the best statistical validation measures and capable of correlating bioactivity variation across the used training set collection. QSAR model was validated employing leave one-out cross-validation by setting the folds to a number much larger than the number of samples, r2 (squared correlation coefficient value) and r2 prediction (predictive squared correlation coefficient value), residuals between the predicted and experimental activity of the test set and training set ().
QSAR study results
EquationEquation (1)(1)
(1) Represents the best performing QSAR model for the activity against HepG2;
(1)
(1)
Table 4. Experimental activities of the synthesized derivatives against the predicted activity according to EquationEquations (1)(1)
(1) and Equation(2)
(2)
(2) .
EquationEquation (2)(2)
(2) Represents the best performing QSAR model for the activity against Caco-2;
(2)
(2)
According to the former equations these QSAR models were represented graphically by scattering plots of the experimental versus the predicted bioactivity values –log IC50 for the training set compounds as shown in and .
Figure 4. Predicted versus experimental pIC50 of the tested compounds against HepG2 according to EquationEquation (1)(1)
(1) (r2 = 0.940).
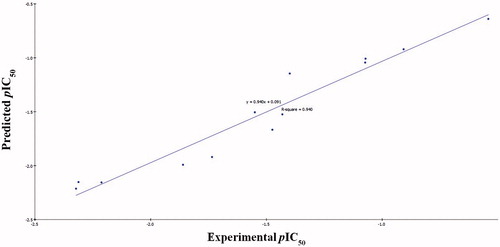
Figure 5. Predicted versus experimental pIC50 of the tested compounds against Caco-2 according to EquationEquation (2)(2)
(2) (r2 = 0.896).
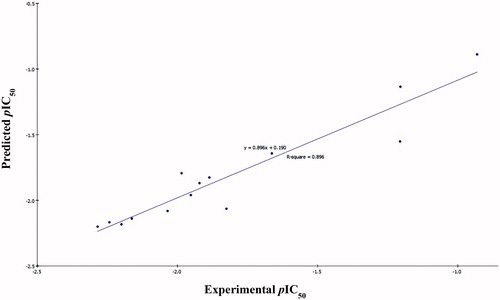
The two MLR models exhibited good correlation coefficient r2 of 0.940 and 0.896, r2 (adj) = 0.910 and 0.865, respectively, r2 (pred) = 0.878 and 0.789, Least-Squared error = 0.0168 and 0.0173 respectively, where r2 (adj) is r2 adjusted for the number of terms in the model; r2 (pred) is the prediction r2, equivalent to q2 from a leave-1-out cross-validationCitation58.
EquationEquations (1) and (2)(2)
(2) for HepG2 and Caco-2 suggested that the anti-proliferative activity of the prepared compounds was positively affected by Dipole, which is a 3D electronic descriptors describing the strength and orientation behavior of a molecule in an electrostatic field. Both the magnitude and the components (X, Y, Z) of the dipole moment are calculated (Debyes). It is estimated by utilizing partial atomic charges and atomic coordinates. Partial atomic charges are computed using Gasteiger if not present. Dipole properties have been correlated to long range ligand-receptor recognition and subsequent binding. The anti-proliferative activity towards HepG2 was also affected by the high and negative value of Shadow_YZfrac while for Caco-2 affected by Shadow_Ylength. Shadow indices are a set of geometric descriptors that characterize the shape of the molecules. They are calculated by projecting the model surface on three mutually perpendicular planes: xy, yz, and xz. These descriptors depend not only on conformation, but also on the orientation of the model. In order to calculate them, the models are first rotated to align the principal moments of inertia with the x-, y-, and z-axes. Shadow_YZ is area of the molecular shadow in the yz plane while Shadow_Ylength is indicator for length of molecule in the y dimensionCitation59.
EquationEquation (1)(1)
(1) also showed that the anti-proliferative activity was also affected by jurs descriptors. Jurs descriptors are a group of geometric descriptors that combine both shape and electronic information which may characterize the moleculesCitation60. In particular, Jurs_PNSA_3, which contributes inversely to the activity, represents the atomic charge weighted negative surface area and calculated by Sum of the product of solvent-accessible surface area × partial charge for all negatively charged atoms. This indicates increasing this value in a molecule could increase reuptake inhibition activity because it is negatively correlated with the activity. EquationEquations (1)
(1)
(1) and Equation(2)
(2)
(2) are also affected by topological descriptors which are a special class of descriptors that do not rely on a three-dimensional model. All calculations are derived from the two-dimensional topology of the molecule. As CHI_3_C which is connectivity indices while IAC_Mean indicate Graph-Theoretical InfoContent descriptors.
QSAR validation
The established QSAR models (1 and 2) were verified by applying; Leave-one-out (LOO) internal validation (r2 = 0.940 and 0.896, respectively). Cross-validation was also employed where q2, which is equivalent to r2 (pred), was 0.878 and 0.789, respectively. In addition, validation was employed by measuring the residuals between the experimental and the predicted activities of the training set listed in . Interestingly, the predicted activities of the QSAR models were found very close to those experimentally observed, Furthermore, to evaluate the predictive ability of the developed models HepG2, compounds 6, 8d, 15a, and 15c were applied as test compounds, where they were not included in model generation, the same was also applied for the Caco-2 model using compounds 6, 9, and 13a ().
Table 5. External validation for the established QSAR models.
Conclusion
In our endeavor to develop potent anti-cancer agents, different sets of coumarin-6-sulfonamide derivatives (4a, b, 8a–d, 11a–d, 13a, b, and 15a–c) were synthesized. Anti-proliferative activities of the newly synthesized coumarins was invistigated in three human tumor cancer cell lines, namely, HepG2 hepatocellular carcinoma, MCF-7 breast cancer and Caco-2 colon cancer using sulforhodamine B (SRB) colorimetric assay. Compounds 13a and 15a emerged as the most active derivatives towards HepG2 cells (IC50 = 3.48 ± 0.28 and 5.03 ± 0.39 µM, respectively), with 1.5- and 1.1-fold increased activity than the reference drug, doxorubicin (IC50 = 6.9 ± 2.05 µM), respectively. Besides, compounds 15a and 15b were the most active members against MCF-7 cells with IC50 values of 10.95 ± 0.96 and 10.62 ± 1.35 µM, respectively. Also, compound 8a possessed the best growth inhibitory activity against Caco-2 cells (IC50 = 8.53 ± 0.72), with 2-fold decreased activity than doxorubicin (IC50 = 4.10 ± 1.37 µM). Compounds 13a and 15a were able to induce apoptosis in HepG2 cells, as assured by the up-regulation of the Bax and down-regulation of the Bcl-2, besides boosting caspase-3 levels. Besides, compound 13a induced a significant increase in the percentage of cells at Pre-G1 by 6.4-folds, with concurrent significant arrest in the G2-M phase by 5.4-folds compared to control. Also, 13a displayed significant increase in the percentage of annexin V-FITC positive apoptotic cells from 1.75% to 13.76%. Moreover, QSAR models were established to explore the structural requirements controlling the anti-proliferative activities.
Supplemental Material
Download PDF (1.6 MB)Disclosure statement
No potential conflict of interest was reported by the authors.
References
- Miller KD, Siegel RL, Lin CC, et al. Cancer treatment and survivorship statistics. CA Cancer J Clin 2016;66:271–89.
- Grover J, Jachak SM. Coumarins as privileged scaffold for anti-inflammatory drug development. RSC Adv 2015;5:38892–905.
- Al-Majedy Y, Kadhum AA, Ibraheem H, et al. A systematic review on pharmacological activities of 4-methylumbelliferon. Sys Rev Pharm 2018;8:24–30.
- Hassan MZ, Osman H, Ali MA, Ahsan MJ. Therapeutic potential of coumarins as antiviral agents. Eur J Med Chem 2016;123:236–55.
- Pinto DC, Silva AM. Anticancer natural coumarins as lead compounds for the discovery of new drugs. Curr Top Med Chem 2017;17:3190–98.
- Thakur A, Singla R, Jaitak V. Coumarins as anticancer agents: a review on synthetic strategies, mechanism of action and SAR studies. Eur J Med Chem 2015;101:476–95.
- Kaur M, Kohli S, Sandhu S, et al. Coumarin: a promising scaffold for anticancer agents. Anticancer Agents Med Chem 2015;15:1032–48.
- Dandriyal J, Singla R, Kumar M, Jaitak V. Recent developments of C-4 substituted coumarin derivatives as anticancer agents. Eur J Med Chem 2016;119:141–68.
- Degorce SL, Bailey A, Callis R, et al. Investigation of (E)-3-[4-(2-Oxo-3-aryl-chromen-4-yl) oxyphenyl] acrylic acids as oral selective estrogen receptor down-regulators. J Med Chem. 2015:58:3522–33.
- Civelli M, Preti AP, Cenacchi V, et al. Single and multiple ascending dose studies of a novel tissue‐selective oestrogen receptor modulator, CHF 4227, in healthy postmenopausal women. Br J Clin Pharmacol. 2007:64:304–16.
- Suzuki N, Liu X, Laxmi YR, et al. Anti‐breast cancer potential of SS5020, a novel benzopyran antiestrogen. Int J Cancer. 2011:128:974–82.
- Liu MM, Chen XY, Huang YQ, et al. Hybrids of phenylsulfonylfuroxan and coumarin as potent antitumor agents. J Med Chem. 2014:57:9343–56.
- Wang C, Xu F, Niu Y, et al. Synthesis and biological evaluations of 3-benzothiazol-2-yl coumarin derivatives as MEK1 inhibitors. Lett Drug Des Discov. 2013:10:727–32.
- Han S, Zhou V, Pan S, et al. Identification of coumarin derivatives as a novel class of allosteric MEK1 inhibitors. Bioorg Med Chem Lett. 2005:15:5467–73.
- Palmieri C, Januszewski A, Stanway S, Coombes RC. Irosustat: a first-generation steroid sulfatase inhibitor in breast cancer. Expert Rev. Anticancer Ther. 2011:11:179–83.
- Daśko M, Przybyłowska M, Rachon J, et al. Synthesis and biological evaluation of fluorinated N-benzoyl and N-phenylacetoyl derivatives of 3-(4-aminophenyl)-coumarin-7-O-sulfamate as steroid sulfatase inhibitors. Eur J Med Chem. 2017:128:79–87.
- Demkowicz S, Daśko M, Kozak W, et al. Synthesis and biological evaluation of fluorinated 3‐phenylcoumarin‐7‐O‐sulfamate derivatives as steroid sulfatase inhibitors. Chem Biol Drug Des. 2016:87:233–8.
- Bana E, Sibille E, Valente S, et al. A novel coumarin‐quinone derivative SV37 inhibits CDC25 phosphatases, impairs proliferation, and induces cell death. Mol Carcinog. 2015:54:229–41.
- Zwergel C, Czepukojc B, Evain-Bana E, et al. Novel coumarin-and quinolinone-based polycycles as cell division cycle 25-A and-C phosphatases inhibitors induce proliferation arrest and apoptosis in cancer cells. Eur J Med Chem. 2017:134:316–33.
- Valente S, Bana E, Viry E, et al. Synthesis and biological evaluation of novel coumarin-based inhibitors of Cdc25 phosphatases. Bioorg Med Chem Lett. 2010:20:5827–30.
- Cao D, Liu Y, Yan W, et al. Design, synthesis, and evaluation of in vitro and in vivo anticancer activity of 4-substituted coumarins: a novel class of potent tubulin polymerization inhibitors. J Med Chem. 2016:59:5721–39.
- Singh H, Kumar M, Nepali K, et al. Triazole tethered C5-curcuminoid-coumarin based molecular hybrids as novel antitubulin agents: Design, synthesis, biological investigation and docking studies. Eur J Med Chem. 2016:116:102–15.
- Samundeeswari S, Kulkarni MV, Joshi SD, et al. Synthesis and human anticancer cell line studies on coumarin‐β‐carboline hybrids as possible antimitotic agents. ChemistrySelect. 2016:1:5019–24.
- Ghorab MM, Alsaid MS, Al-Ansary GH, et al. Analogue based drug design, synthesis, molecular docking and anticancer evaluation of novel chromene sulfonamide hybrids as aromatase inhibitors and apoptosis enhancers. Eur J Med Chem. 2016:124:946–58.
- Chen S, Cho M, Karlsberg K, et al. Biochemical and biological characterization of a novel anti-aromatase coumarin derivative. J Biol Chem. 2004:279:48071–8.
- Lu XY, Wang ZC, Ren SZ, et al. Coumarin sulfonamides derivatives as potent and selective COX-2 inhibitors with efficacy in suppressing cancer proliferation and metastasis. Bioorg Med Chem Lett. 2016:26:3491–8.
- (a) Avin BV, Thirusangu P, Ranganatha VL, et al. Synthesis and tumor inhibitory activity of novel coumarin analogs targeting angiogenesis and apoptosis. Eur J Med Chem. 2014:75:211–21. (b) Lin MH, Cheng CH, Chen KC, et al. Induction of ROS-independent JNK-activation-mediated apoptosis by a novel coumarin-derivative, DMAC, in human colon cancer cells. Chem-Biol Interact. 2014:218:42–9.
- (a) Han HW, Zheng CS, Chu SJ, et al. The evaluation of potent antitumor activities of shikonin coumarin-carboxylic acid, PMMB232 through HIF–1α–mediated apoptosis. Biomed Pharmacother. 2018:97:656–66. (b) Lopez-Gonzalez JS, Prado-Garcia H, Aguilar-Cazares D, et al. Apoptosis and cell cycle disturbances induced by coumarin and 7-hydroxycoumarin on human lung carcinoma cell lines. Lung cancer. 2004:43:275–83.
- (a) Wang Q, Guo Y, Jiang S, et al. A hybrid of coumarin and phenylsulfonylfuroxan induces caspase-dependent apoptosis and cytoprotective autophagy in lung adenocarcinoma cells. Phytomedicine. 2017:160–67. (b) Perumalsamy H, Sankarapandian K, Veerapan K, et al. In silico and In vitro analysis of coumarin derivative induced anticancer effects by undergoing intrinsic pathway mediated apoptosis in human stomach cancer. Phytomedicine. 2018.
- (a) Mirunalini S, Deepalakshmi K, Manimozhi J. Antiproliferative effect of coumarin by modulating oxidant/antioxidant status and inducing apoptosis in Hep2 cells. Biomed Aging Path. 2014:4:131–5. (b) Domracheva I, Kanepe-Lapsa I, Jackevica L, et al. Selenopheno quinolinones and coumarins promote cancer cell apoptosis by ROS depletion and caspase-7 activation. Life Sci. 2017:186:92–101.
- (a) Ma YM, Zhou YB, Xie CM, et al. Novel microtubule-targeted agent 6-chloro-4-(methoxyphenyl) coumarin induces G 2-M arrest and apoptosis in HeLa cells. Acta Pharmacol Sin. 2012:33:407–17. (b) Álvarez‐Delgado C, Reyes‐Chilpa R, Estrada‐Muñiz E, et al. Coumarin A/AA induces apoptosis‐like cell death in HeLa cells mediated by the release of apoptosis‐inducing factor. J Biochem Mol Toxicol. 2009:23:263–72.
- (a) Scozzafava A, Mastrolorenzo A, Supuran CT. Sulfonamides and sulfonylated derivatives as anticancer agents. Curr Cancer Drug Targets 2002;2:55–75. (b) Uehara T, Minoshima Y, Sagane K, et al. Selective degradation of splicing factor CAPERα by anticancer sulfonamides. Nat Chem Biol 2017; 13:675.
- (a) Eldehna WM, Abo-Ashour MF, Nocentini A, et al. Novel 4/3-((4-oxo-5-(2-oxoindolin-3-ylidene) thiazolidin-2-ylidene) amino) benzenesulfonamides: Synthesis, carbonic anhydrase inhibitory activity, anticancer activity and molecular modelling studies. Eur J Med Chem 2017;139:250–62. (b) Fares M, Eladwy RA, Nocentini A, et al. Synthesis of bulky-tailed sulfonamides incorporating pyrido [2, 3-d][1, 2, 4] triazolo [4, 3-a] pyrimidin-1 (5H)-yl) moieties and evaluation of their carbonic anhydrases I, II, IV and IX inhibitory effects. Bioorg Med Chem. 2017;25:2210–7. (c) Alafeefy AM, Ahmad R, Abdulla M, et al. Development of certain new 2-substituted-quinazolin-4-yl-aminobenzenesulfonamide as potential antitumor agents. Eur J Med Chem 2016;109:247–53. (d) Eldehna WM, Fares M, Ceruso M, et al. Amido/ureidosubstituted benzenesulfonamides-isatin conjugates as low nanomolar/subnanomolar inhibitors of the tumor-associated carbonic anhydrase isoform XII. Eur J Med Chem 2016;110:259–66. (e) Eldehna WM, Al-Ansary GH, Bua S, et al. Novel indolin-2-one-based sulfonamides as carbonic anhydrase inhibitors: Synthesis, in vitro biological evaluation against carbonic anhydrases isoforms I, II, IV and VII and molecular docking studies. Eur J Med Chem 2017;127:521–30.
- Reddy NS, Mallireddigari MR, Cosenza S, et al. Synthesis of new coumarin 3-(N-aryl) sulfonamides and their anticancer activity. Bioorg Med Chem Lett 2004;14:4093–7.
- Musa MA, Cooperwood JS, Khan MO. A review of coumarin derivatives in pharmacotherapy of breast cancer. Curr Med Chem 2008;15:2664–79.
- Bonardi A, Falsini M, Catarzi D, et al. Structural investigations on coumarins leading to chromeno [4, 3-c] pyrazol-4-ones and pyrano [4, 3-c] pyrazol-4-ones: New scaffolds for the design of the tumor-associated carbonic anhydrase isoforms IX and XII. Eur J Med Chem 2018.
- Chandak N, Ceruso M, Supuran CT, Sharma PK. Novel sulfonamide bearing coumarin scaffolds as selective inhibitors of tumor associated carbonic anhydrase isoforms IX and XII. Bioorg Med Chem 2016;24:2882–6.
- Pacchiano F, Carta F, McDonald PC, et al. Ureido-substituted benzenesulfonamides potently inhibit carbonic anhydrase IX and show antimetastatic activity in a model of breast cancer metastasis. J Med Chem 2011:54:1896–902.
- Safety Study of SLC-0111 in Subjects with Advanced Solid Tumours. See at: https://clinicaltrials.gov/ct2/show/NCT02215850.
- Lou Y, McDonald PC, Oloumi A, et al. Targeting tumor hypoxia: suppression of breast tumor growth and metastasis by novel carbonic anhydrase IX inhibitors. Cancer Res 2011:71:3364–76.
- Wang W, Ao L, Rayburn ER, et al. KCN1, a novel synthetic sulfonamide anticancer agent: in vitro and in vivo anti-pancreatic cancer activities and preclinical pharmacology. PloS One 2012:7:e44883.
- Yin S, Kaluz S, Devi NS, et al. Arylsulfonamide KCN1 inhibits in vivo glioma growth and interferes with HIF signaling by disrupting HIF-1α interaction with cofactors p300/CBP. Clin Cancer Res 2012.
- Fukuoka K, Usuda J, Iwamoto Y, et al. Mechanisms of action of the novel sulfonamide anticancer agent E7070 on cell cycle progression in human non-small cell lung cancer cells. Invest New Drugs 2001:19:219–27.
- Ozawa Y, Sugi NH, Nagasu T, et al. E7070, a novel sulphonamide agent with potent antitumour activity in vitro and in vivo. Eur J Cancer 2001:37:2275–82.
- Ozawa Y, Kusano K, Owa T, et al. Therapeutic potential and molecular mechanism of a novel sulfonamide anticancer drug, indisulam (E7070) in combination with CPT-11 for cancer treatment. Cancer Chemother Pharmacol. 2012:69:1353–62.
- Manasa KL. E7010: investigational anticancer agents targeting the microtubules. Int J Pharm Sci Res. 2015:6:3713.
- Assi R, Kantarjian HM, Kadia TM, et al. Final results of a phase 2, open‐label study of indisulam, idarubicin, and cytarabine in patients with relapsed or refractory acute myeloid leukemia and high‐risk myelodysplastic syndrome. Cancer. 2018. (Accepted manuscript, DOI: 10.1002/cncr.31398)
- Miller JN. Coumarin-6-sulphonyl chloride: a novel label in fluorimetry and phosphorimetry Part 1. Synthesis and Luminescence Properties. Anal Chim Acta 1989;227:145–53.
- Bary HM, Aleem AH, Ismail II. Reactions with coumarin IV. Afinidad 1995;52:344–6.
- (a) Skehan P, Storeng R, Scudiero D, et al. New colorimetric cytotoxicity assay for anticancer-drug screening. JNCI: J Natl Cancer Inst 1990;82:1107–12. (b) Eldehna WM, Fares M, Ibrahim HS, et al. Synthesis and cytotoxic activity of biphenylurea derivatives containing indolin-2-one moieties. Molecules. 2016;21(6):762.
- Eldehna WM, Almahli H, Al-Ansary GH, et al. Synthesis and in vitro anti-proliferative activity of some novel isatins conjugated with quinazoline/phthalazine hydrazines against triple-negative breast cancer MDA-MB-231 cells as apoptosis-inducing agents. J Enz Inhib Med Chem 2017;32:600–13.
- Almahli H, Hadchity E, Jaballah MY, et al. Development of novel synthesized phthalazinone-based PARP-1 inhibitors with apoptosis inducing mechanism in lung cancer. Bioorg Chem 2018;77:443–56.
- (a) Eldehna WM, EL-Naggar DH, Hamed AR, et al. One-pot three-component synthesis of novel spirooxindoles with potential cytotoxic activity against triple-negative breast cancer MDA-MB-231 cells. J Enz Inhib Med Chem 2018;33:309–18. (b) Abdel-Aziz HA, Ghabbour HA, Eldehna WM, et al. 2-((Benzimidazol-2-yl) thio)-1-arylethan-1-ones: Synthesis, crystal study and cancer stem cells CD133 targeting potential. Eur J Med Chem 2015;104:1–10.
- Hu W, Kavanagh JJ. Anticancer therapy targeting the apoptotic pathway. Lancet Oncol 2003;4:721–9.
- Attia MI, Eldehna WM, Afifi SA, et al. New hydrazonoindolin- 2-ones: synthesis, exploration of the possible anti-proliferative mechanism of action and encapsulation into PLGA microspheres. PLoS One 2017;12:e0181241.
- (a) Eldehna WM, Ibrahim HS, Abdel-Aziz HA, et al. Design, synthesis and in vitro antitumor activity of novel N-substituted- 4-phenyl/benzylphthalazin-1-ones. Eur J Med Chem 2015;89:549–60. (b) Abdel-Aziz HA, Eldehna WM, Ghabbour H, et al. Synthesis, crystal study, and anti-proliferative activity of some 2-benzimidazolylthioacetophenones towards triple-negative breast cancer MDA-MB-468 cells as apoptosis-inducing agents. Int J Mol Sci. 2016;17:1221.
- Discovery Studio 4.0 (Accelrys, Co. Ltd). 2017. http://www.accelrys.com/ [last accessed 24 July 2017].
- Mitra I, Saha A, Roy K. Chemometric QSAR modeling and in silico design of antioxidant NO donor phenols. Sci Pharma 2010;79:31–58.
- Behmaram B, Foroughifar N, Foroughifar N, Hallajian S. Synthesis of some derivatives of 4-phenyl-1, 3-dihydro-2H-imidazole-2-thion using ionic liquid as catalyst and evaluation of their antimicrobial activity. Int J Chem 2017;9:45.
- Ghose AK, Crippen GM. Atomic physicochemical parameters for three-dimensional structure-directed quantitative structure-activity relationships I. Partition coefficients as a measure of hydrophobicity. J Comput Chem 1986;7:565–77.