Abstract
In connection with our research program on the development of novel anticancer candidates, herein we report the design and synthesis of novel series of 1-(2-methyl-6-arylpyridin-3-yl)-3-phenylureas 5a–l. The target pyridins were evaluated for their in vitro anticancer activity against two cancer cell lines: non-small cell lung cancer A549 cell line and colon cancer HCT-116 cell line. Compound 5l emerged as the most active congener towards both A549 and HCT-116 cell lines with IC50 values equal to 3.22 ± 0.2 and 2.71 ± 0.16 µM, respectively, which are comparable to those of Doxorubicin; 2.93 ± 0.28 and 3.10 ± 0.22, respectively. Furthermore, compound 5l stood out as the most potent pyridine derivative (mean % GI = 40), at US-NCI Developmental Therapeutic Program anticancer assay, with broad-spectrum antitumor activity against the most tested cancer cell lines from all subpanels. Compound 5l was able to provoke apoptosis in HCT-116 cells as evidenced by the decreased expression of the anti-apoptotic Bcl-2 protein, and the enhanced expression of the pro-apoptotic proteins levels; Bax, cytochrome C, p53, caspase-3 and caspase-9. Moreover, 5l disrupted the HCT-116 cell cycle via alteration of the Sub-G1 phase and arresting the G2-M stage. Also, 5l showed a significant increase in the percent of annexinV-FITC positive apoptotic cells from 1.99 to 15.76%.
Introduction
Apoptosis, a self-automated cell death, represents the principal pathway in tissue homeostasis and in animal development; in addition, it is the main pathway for the clearance of aged or defective cells in the body. Mainly, two major signaling pathways for apoptotic cell death have been signified. The first one is the extrinsic cytoplasmic pathway that is triggered via pro-apoptotic ligands binding to the cell surface death receptor. Whereas, the second is the intrinsic mitochondrial apoptotic pathway that results from an intracellular cascade of events that are mainly produced by cellular stress, in which mitochondrial permeabilization plays a crucial role. Both extrinsic and intrinsic pathways converge onto the activation of effector caspases, resulting in apoptotic cell death program. During cancer pathogenesis, apoptosis deregulation has been widely recognized as a hallmark of cancer. Accordingly, induction of apoptosis in tumor cells has stood out as a successful tactic for combating different human malignancies, in the current medical eraCitation1–3.
On the other hand, non-fused pyridines have stood out as a promising class of anticancer agents with efficient pro-apoptotic activity. Regorafenib (Stivarga®, ), a pyridine-based biphenyl urea derivative developed by BayerCitation4, inhibits angiogenickinases VEGFR-1/3, FGFR1, PDGFRb, and Tie-2. Regorafenib was approved by FDA, in September 2012, for the treatment of metastatic colorectal cancer (mCRC)Citation5. The anticancer effect of Regorafenib is thought to be mediated by apoptosis induction, in addition to its anti-angiogenic and anti-proliferative effectsCitation6,Citation7. Crizotinib (Xalkori®, ) is an orally active inhibitor of multiple receptor tyrosine kinases, including anaplastic lymphoma kinase (ALK), Hepatocyte Growth Factor Receptor (HGFR, c-Met), and Recepteur d’Origine Nantais (RON)Citation8. Crizotinib was approved for the treatment of adults with previously treated, ALK-positive, advanced non-small cell lung cancer (NSCLC)Citation9. Crizotinib likely exerts its anticancer activity via multiple distinct mechanisms such as apoptosisCitation10.
Figure 1 Structures of certain pyridine-based approved anticancer drugs, and the target pyridines 5a–l.
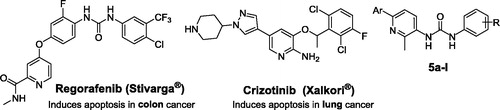
Recently, our research group has explored the anticancer activity for novel series of 1–(2-methyl-6-(4-methoxy/3,4-dimethoxyphenyl)-pyridin-3-yl)-3-phenylureasCitation11. All these derivatives were evaluated for their growth inhibitory activity against the proliferation of breast cancer cell line (MCF-7), where they displayed promising anti-proliferative activity. On the other hand, examination of their potential anti-angiogenic activity towards vascular endothelial growth factor receptor 2 (VEGFR-2) tyrosine kinase unveiled their incompetence to inhibit VEGFR-2 significantlyCitation11.
Based on the aforementioned findings and as a part of our ongoing quest towards developing potent anticancer agentsCitation12–20, herein we report the synthesis and biological evaluation of novel series of 1-(2-methyl-6-arylpyridin-3-yl)-3-phenylureas 5a–l. Ten selected pyridines 5a, 5c–j and 5l were chosen to be in vitro evaluated for their antitumor activity at one dose (concentration 10−5 M) primary anticancer assay towards a panel including 85 cancer lines according to US-NCI protocol. In addition, all pyridines 5a–l were examined for their potential anti-proliferative activity against non-small cell lung cancer A549 cell line and colon cancer HCT-116 cell line. Furthermore, apoptosis induction potential of the target pyridines was examined in HCT-116 cells, in order to acquire more mechanistic insights and to verify and enlighten the antitumor properties of the investigated pyridines.
Materials and methods
Chemistry
Melting points were measured with a Stuart melting point apparatus and were uncorrected. Infrared (IR) Spectra were recorded as KBr disks using Schimadzu FT-IR 8400S spectrophotometer. 1H-NMR and 13C-NMR experiments were carried out using Bruker NMR spectrometer (400/100 MHz). Chemical shifts (δH) are reported relative to TMS as the internal standard. All coupling constant (J) values are given in hertz. Chemical shifts (δC) were reported as follows: s, singlet; d, doublet; m, multiplet. High-resolution mass spectra were recorded using a Bruker MicroTOF spectrometer (Bruker Daltonics, Bremen, Germany). All reagents and solvents were dried and purified by the standard techniques. Compounds 2-methyl-6-arylnicotinohydrazides 2a–cCitation21–23 were previously prepared.
General procedures for preparation of the target pyridines 5a–l
A solution of hydrazides 2a–c (10 mmol) and sodium nitrite (1 g, 14 mmol) in hydrochloric acid was stirred for 1 h in an ice bath, then stirring was continued for an additional 1 h at room temperature. The reaction mixture was poured over crushed ice. The precipitated solid was filtered off and air-dried to yield 2-methyl-6-arylnicotinoyl azides 3a–c, which were used in the next step without further purification. Azides 3a–c were heated in refluxing dry xylene for 1 h, then the appropriate aniline derivative was added to this xylene solution. The reaction mixture was heated under reflux temperature for 4 h. After cooling to room temperature, the formed precipitate was filtered, washed with ether and recrystallized from ethanol to afford the target pyridines 5a–l.
1-(6-(4-Fluorophenyl)-2-methylpyridin-3-yl)-3-(3-(trifluoromethyl)phenyl)urea (5a)
White crystals (yield 70%), m.p. 223–225 °C; IR (KBr, ν cm−1) 3393 (NH), 1731 (C=O); 1H NMR (CDCl3-d) δ ppm: 2.64 (s, 3H, CH3), 6.30 (s, 1H, NH, D2O exchangeable), 6.61 (s, 1H, NH, D2O exchangeable), 7.15 (t, 2H, J = 8.8 Hz, Ar-H), 7.38 (d, 1H, J = 8.4 Hz, Ar-H), 7.46 (t, 1H, J = 8.0 Hz, Ar-H), 7.60–7.65 (m, 2H, Ar-H), 7.71 (s, 1H, Ar-H), 7.98 (dd, 2H, J = 8.8 Hz, J = 5.6 Hz, Ar-H), 8.06 (d, 1H, J = 8.4 Hz, Ar-H);13C NMR (DMSO-d6) δ ppm: 21.34 (CH3), 115.36, 115.53, 117.69, 121.75, 128.04, 128.67, 130.06, 132.47, 135.00, 140.42, 148.02, 148.45, 152.62 (CO), 161.43, 163.38 (=C-F); HRMS (ESI) m/z calcd for [M + H]+ (C20H16N3OF4): 390.12240, found: 390.12286.
1-(3,5-Bis(trifluoromethyl)phenyl)-3-(6-(4-fluorophenyl)-2-methylpyridin-3-yl)urea (5b)
White crystals (yield 65%), m.p. 235–237 °C; IR (KBr, ν cm−1) 3390 (NH), 1733 (C=O); 1H NMR (CDCl3-d) δ ppm: 2.58 (s, 3H, CH3), 6.31 (s, 1H, NH, D2O exchangeable), 6.59 (s, 1H, NH, D2O exchangeable), 7.17 (t, 2H, J = 8.8 Hz, Ar-H), 7.59 (s, 1H, Ar-H), 7.63 (d, 1H, J = 8.4 Hz, Ar-H), 7.89 (s, 2H, Ar-H), 8.02–8.10 (m, 3H, Ar-H); 13 C NMR (DMSO-d6) δ ppm: 21.58 (CH3), 115.44, 115.61, 117.75, 128.09, 128.15, 128.66, 132.72, 135.10, 147.79, 148.34, 152.94 (C=O), 161.49, 163.44 (=C–F).
Ethyl 4-(3-(6-(4-fluorophenyl)-2-methylpyridin-3-yl)ureido)benzoate (5c)
White crystals (yield 73%), m.p. 209–211 °C; IR (KBr, ν cm−1) 3389 (NH), 1733 (C=O); 1H NMR (CDCl3-d) δ ppm: 1.39 (t, 3H, J = 7.2 Hz, –OCH2CH3), 2.62 (s, 3H, –CH3), 4.37 (q, 2H, J = 7.2 Hz, –OCH2CH3), 6.36 (s, 1H, NH, D2O exchangeable), 6.72 (s, 1H, NH, D2O exchangeable), 7.14 (t, 2H, J = 8.8 Hz, Ar-H), 7.49 (d, 2H, J = 8.4 Hz, Ar-H), 7.60 (d, 1H, J = 8.4 Hz, Ar-H), 7.98–8.10 (m, 5H, Ar-H); 13 C NMR (DMSO-d6) δ ppm: 14.30 (CH3), 21.37 (CH3), 60.39 (CH2), 115.41, 115.58, 117.34, 117.75, 122.98, 128.08, 128.50, 130.51, 132.50, 135.04, 144.16, 147.93, 148.47, 152.35 (C=O), 161.48, 163.43 (=C–F), 165.48 (–COO–) HRMS (ESI) m/z calcd for [M + H]+ (C22H21N3O3F): 394.15615, found: 394.15628.
1-(Benzo[d][1, 3]dioxol-5-yl)-3-(6-(4-fluorophenyl)-2-methylpyridin-3-yl)urea (5d)
White crystals (yield 62%), m.p. 254–256 °C; IR (KBr, ν cm−1) 3394 (NH), 1733 (C=O); 1H NMR (CDCl3-d) δ ppm: 2.48 (s, 3H, CH3), 6.04 (s, 2H, CH2), 6.23 (s, 1H, NH, D2O exchangeable), 6.34 (s, 1H, NH, D2O exchangeable), 6.84 (d, 1H, J = 8.0 Hz, Ar-H), 6.97–7.02 (m, 2H, Ar-H), 7.12 (t, 2H, J = 8.4 Hz, Ar-H), 7.54–7.57 (m, 1H, Ar-H), 7.94–7.98 (m, 2H, Ar-H), 8.19 (d, 1H, J = 8.0 Hz, Ar-H); 13C NMR (DMSO-d6) δ ppm: 21.36 (CH3), 100.82 (O–CH2–O), 108.20, 110.93, 115.33, 115.50, 117.65, 127.99, 132.94, 133.89, 135.08, 142.16, 147.27, 147.83, 152.66 (C=O), 161.35, 163.30 (=C–F); HRMS (ESI) m/z calcd for [M + H]+ (C20H17N3O3F): 366.12485, found: 366.12405.
1-(6-(4-Chlorophenyl)-2-methylpyridin-3-yl)-3-(3-(trifluoromethyl)phenyl)urea (5e)
White crystals (yield 68%), m.p. 241-242 °C; IR (KBr, ν cm−1) 3378 (NH), 1733 (C=O); 1H NMR (CDCl3-d) δ ppm: 2.48 (s, 3H, CH3), 6.25 (s, 1H, NH, D2O exchangeable), 6.36 (s, 1H, NH, D2O exchangeable), 7.38 (d, 1H, J = 8.4 Hz, Ar-H), 7.41 (d, 2H, J = 8.8 Hz, Ar-H), 7.52-7.58 (m, 3H, Ar-H), 7.78 (s, 1H, Ar-H), 7.91 (d, 2H, J = 8.4 Hz, Ar-H), 8.24 (d, 1H, J = 8.4 Hz, Ar-H); 13 C NMR (DMSO-d6) δ ppm: 21.57 (CH3), 1117.91, 127.69, 128.41, 128.68, 133.05, 137.32, 147.75, 147.84, 152.82 (C=O); HRMS (ESI) m/z calcd for [M-H]+ (C20H14N3OClF3): 404.07830, found: 404.07779.
1-(6-(4-Chlorophenyl)-2-methylpyridin-3-yl)-3-(4-methoxyphenyl) urea (5f)
White crystals (yield 55%), m.p. 264-265 °C; IR (KBr, ν cm−1) 3392 (NH), 1733 (C=O); 1H NMR (CDCl3-d) δ ppm: 2.41 (s, 3H, CH3), 3.86 (s, 3H, –OCH3), 6.27 (s, 1H, NH, D2O exchangeable), 6.33 (s, 1H, NH, D2O exchangeable), 6.97 (d, 2H, J = 8.4 Hz, Ar-H), 7.31 (d, 2H, J = 8.8 Hz, Ar-H), 7.41 (d, 2H, J = 8.8 Hz, Ar-H), 7.57 (d, 1H, J = 8.0 Hz, Ar-H), 7.91 (d, 2H, J = 8.4 Hz, Ar-H), 8.26 (d, 1H, J = 8.4 Hz, Ar-H); 13 C NMR (DMSO-d6) δ ppm: 21.37 (CH3), 55.18 (OCH3), 114.08, 117.88, 119.92, 127.45, 127.57, 127.66, 128.61, 132.49, 132.87, 133.47, 137.30, 137.39, 147.13, 147.23, 147.69, 147.79, 152.67 (C=O), 154.58 (=C–OCH3); HRMS (ESI) m/z calcd for [M − H]+ (C20H17N3O2Cl): 366.10148, found: 366.10152.
1-(Benzo[d][1,3]dioxol-5-yl)-3-(6-(4-chlorophenyl)-2-methylpyridin-3-yl)urea (5g)
White crystals (yield 63%), m.p. 271–273 °C; IR (KBr, ν cm−1) 3388 (NH), 1733 (C=O); 1H NMR (CDCl3-d) δ ppm: 2.47 (s, 3H, CH3), 6.04 (s, 2H, –OCH2O–), 6.28 (s, 1H, NH, D2O exchangeable), 6.38 (s, 1H, NH, D2O exchangeable), 6.79–6.87 (m, 2H, Ar-H), 6.96 (d, 1H, J = 2.1 Hz, Ar-H), 7.42 (d, 2H, J = 8.4 Hz, Ar-H), 7.57 (d, 1H, J = 8.4 Hz, Ar-H), 7.91 (d, 2H, J = 8.8 Hz, Ar-H), 8.22 (d, 1H, J = 8.4 Hz, Ar-H); 13 C NMR (DMSO-d6) δ ppm: 21.36 (CH3), 100.84 (O–CH2–O), 108.21, 110.96, 117.88, 127.59, 128.62, 132.90, 133.32, 133.83, 137.36, 142.20, 147.28, 152.60 (C=O); HRMS (ESI) m/z calcd for [M-H]+ (C20H15N3O3Cl): 380.08074, found: 380.08115.
1-(4-Fluorophenyl)-3-(2-methyl-6-(thiophen-2-yl)pyridin-3-yl)urea (5h)
White crystals (yield 60%), m.p. 217–219 °C; IR (KBr, ν cm−1) 3393 (NH), 1733 (C=O); 1H NMR (CDCl3-d) δ ppm: 2.50 (s, 3H, CH3), 6.20 (s, 1H, NH, D2O exchangeable), 6.33 (s, 1H, NH, D2O exchangeable), 7.07–7.13 (m, 3H, Ar-H), 7.35–7.39 (m, 3H, Ar-H), 7.54–7.56 (m, 2H, Ar-H), 8.05 (d, 1H, J = 8.4 Hz, Ar-H); 13 C NMR (DMSO-d6) δ ppm: 21.09 (CH3), 115.30, 115.47, 116.47, 119.85, 119.91, 123.81, 127.07, 127.11, 128.16, 128.21, 132.55, 135.86, 144.65, 145.36, 147.51, 152.64 (C=O); HRMS (ESI) m/z calcd for [M-H]+ (C17H13N3OFS): 326.07688, found: 326.07718.
1-(4-Chlorophenyl)-3-(2-methyl-6-(thiophen-2-yl) pyridin-3-yl)urea (5i)
White crystals (yield 71%), m.p. 234–236 °C; IR (KBr, ν cm−1) 3398 (NH), 1733 (C=O); 1H NMR (CDCl3-d) δ ppm: 2.53 (s, 3H, CH3), 6.18 (s, 1H, NH, D2O exchangeable), 6.36 (s, 1H, NH, D2O exchangeable), 7.06–7.14 (m, 1H, Ar-H), 7.35-7.40 (m, 5H, Ar-H), 7.52-7.54 (m, 2H, J = 6.5 Hz, Ar-H), 7.99 (d, 1H, J = 8.4 Hz, Ar-H); 13 C NMR (DMSO-d6) δ ppm: 21.08 (CH3), 116.48, 119.67, 123.88, 125.51, 127.13, 128.30, 128.72, 132.38, 138.53, 144.61, 145.51, 147.66, 152.46 (C=O); HRMS (ESI) m/z calcd for [M-H]+ (C17H13N3OClS): 342.04733, found: 342.04752.
Ethyl 4-(3-(2-methyl-6-(thiophen-2-yl) pyridin-3-yl)ureido)benzoate (5j)
White crystals (yield 69%), m.p. 203–204 °C; IR (KBr, ν cm−1) 3393 (NH), 1733 (C=O); 1H NMR (CDCl3-d) δ ppm: 1.39 (t, 3H, J = 7.2 Hz, –OCH2CH3), 2.55 (s, 3H, –CH3), 4.35 (q, 2H, J = 7.2 Hz, –OCH2CH3), 6.53 (s, 1H, NH, D2O exchangeable), 6.98 (s, 1H, NH, D2O exchangeable), 7.10 (t, 1H, J = 4.4 Hz, Ar-H), 7.38 (d, 1H, J = 5.2 Hz, Ar-H), 7.46 (d, 2H, J = 8.4 Hz, Ar-H), 7.52–7.55 (m, 2H, Ar-H), 7.99–8.02 (m, 3H, Ar-H); 13 C NMR (DMSO-d6) δ ppm: 14.26 (CH3), 21.08 (CH3), 60.32 (O–CH2), 116.49, 117.28, 122.91, 123.97, 127.21, 128.25, 128.50, 130.45, 132.17, 144.12, 144.57, 145.73, 147.86, 152.26 (C=O), 165.40 (–COO–); HRMS (ESI) m/z calcd for [M − H]+ (C20H18N3O3S): 380.10744, found: 380.10764.
1-(Benzo[d][1, 3]dioxol-5-yl)-3-(2-methyl-6-(thiophen-2-yl)pyridin-3-yl)urea (5k)
White crystals (yield 58%), m.p. 239–241 °C; IR (KBr, ν cm−1) 3388 (NH), 1733 (C=O); 1H NMR (CDCl3-d) δ ppm: 2.44 (s, 3H, CH3), 6.03 (s, 2H, –OCH2O–), 6.23 (s, 1H, NH, D2O exchangeable), 6.31 (s, 1H, NH, D2O exchangeable), 6.77 (dd, 1H, J = 2.0 Hz, J = 8.0 Hz, Ar-H), 6.83 (d, 1H, J = 8.0 Hz, Ar-H), 6.97 (d, 1H, J = 2.0 Hz, Ar-H), 7.08 (dd, 1H, J = 4.0 Hz, J = 5.2 Hz, Ar-H), 7.35 (d, 1H, J = 5.0 Hz, Ar-H), 7.53-7.54 (m, 2H, Ar-H), 8.12 (d, 1H, J = 8.4 Hz, Ar-H); 13 C NMR (DMSO-d6) δ ppm: 21.08 (CH3), 100.87 (O–CH2–O), 108.20, 110.96, 116.47, 123.74, 127.01, 127.92, 128.21, 132.66, 133.86, 142.18, 144.68, 145.20, 147.27, 152.61 (C=O); HRMS (ESI) m/z calcd for [M − H]+ (C18H14N3O3S): 352.07614, found: 352.07642.
2-(3-(2-Methyl-6-(thiophen-2-yl)pyridin-3-yl)ureido)benzenesulfonamide (5l)
White crystals (yield 60%), m.p. 265–266 °C; IR (KBr, ν cm−1) 3369, 3207 (NH, NH2), 1733 (C=O), 1330, 1157 (SO2); 1H NMR (DMSO-d6, 400 MHz) δ ppm: 2.50 (s, 3H, CH3), 7.11 (t, 1H, H-4 of 2-thienyl, J = 4.0 Hz), 7.18 (t, 1H, H-4 of 2-(H2NO2S)-C6H4, J = 7.6 Hz), 7.52-7.56 (m, 2H, H-5 of 2-(H2NO2S)-C6H4, and H-5 of 2-thienyl), 7.60 (s, 2H, SO2NH2), 7.67 (d, 1H, H-3 of 2-thienyl, J = 4.0 Hz), 7.71 (d, 1H, H-5 pyridine, J = 8.4 Hz), 7.82 (d, 1H, H-6 of 2-(H2NO2S)–C6H4, J = 7.6 Hz), 7.97 (d, 1H, H-3 of 2-(H2NO2S)-C6H4, J = 8.0 Hz), 8.04 (d, 1H, H-4 pyridine, J = 8.4 Hz), 8.73 (s, 1H, 8.21 (s, 1H, NH, D2O exchangeable), 9.15 (s, 1H, NH, D2O exchangeable).
Biological evaluation
In vitro antitumor activity towards 60 cancer cell lines (NCI, USA)
The antitumor assay was performed according to the protocol of the Drug Evaluation Branch, NCI, BethesdaCitation24–26. A 48 h drug exposure protocol was adopted, and sulforhodamine B (SRB) assayCitation27 was utilized to assess the cell growth and viability, as reported earlierCitation17,Citation28.
In vitro anti-Proliferative activity towards A549 and HCT-116 cell lines
A549 (non-small cell lung cancer cell line) and HCT-116 (human colon cancer cell line), were obtained from American Type Culture Collection (Manassas, VA, USA). The cells were maintained in Dulbecco’s Modified Eagle’s Medium (DMEM) (Sigma-Aldrich, St. Louis, MO), and supplemented with 10% heat-inactivated FBS (Hyclone), 10 μg/mL of insulin (Manufacturer, Sigma, St. Louis, MO, USA), and 1% penicillin-streptomycin. MTT assayCitation29 was adopted to assess the in vitro antitumor activity of the newly synthesized pyridines 5a–l according to the reported proceduresCitation30, using Doxorubicin as a standard treatment. Experimental conditions were tested using three replicates (three wells of the 96-well plate per experimental condition) and all experiments were carried out in triplicates. IC50 values were calculated by the use of the equation for Boltzman sigmoidal concentration–response curve using the nonlinear regression fitting models by Graph Pad, Prism version 5 (GraphPad Software Inc., La Jolla, CA).
ELISA immunoassay
The levels of the apoptotic markers Bax, cytochrome C, p53, caspase-3 and caspase-9 as well as the anti-apoptotic protein Bcl-2 were evaluated using ELISA colorimetric kits per the manufacturer’s instructions, as reported earlierCitation31,Citation32.
Cell cycle analysis
HCT-116 cells were treated with pyridine 5l at its IC50 concentration (IC50 = 2.71 μM) for 24 h, then cells were washed with ice-cold phosphate-buffered saline (PBS). The treated cells were collected by centrifugation, fixed in ice-cold 70% (v/v) ethanol, washed with PBS, re-suspended with 100 μg/mL RNase, stained with 40 μg/mL PI, and analyzed by flow cytometry using FACS Calibur (Becton Dickinson, BD, USA). The cell cycle distributions were calculated using CellQuest software 5.1 (Becton Dickinson)Citation33.
Annexin V-FITC apoptosis assay
Phosphatidylserine externalization was assayed using Annexin V-FITC/PI apoptosis detection kit (BD Biosciences, USA) according to the manufacturer’s instructions, as reported earlierCitation33,Citation34.
Results and discussion
Chemistry
The method adopted for preparation of the target pyridines 5a–l is depicted in Scheme 1. Firstly, esters 1a–c were hydrazinolyzed via reaction with hydrazine hydrate in methanol under reflux temperature to furnish 2-methyl-6-arylnicotinohydrazides 2a–c in 75, 71 and 80% yields, respectively. Treatment of hydrazides 2a–c with sodium nitrite in cold hydrochloric acid afforded 2-methyl-6-arylnicotinoyl azides 3a–c, which subsequently subjected to Curtius rearrangement upon heating in xylene to give the corresponding isocyanates derivatives 4a–c. The target hybrids 5a–l was obtained by reaction of isocyanates derivatives 4a–c with the appropriate aniline derivative in xylene with 55–73% yield (Scheme 1).
Scheme 1 Synthesis of target derivatives 5a–l; (i) Ethyl alcohol, NH2NH2·H2O, reflux 3 h.; (ii) NaNO2, HCl, stirring 2 h.; (iii) Xylene, reflux 1 h.; (iv) Xylene, reflux 4 h.
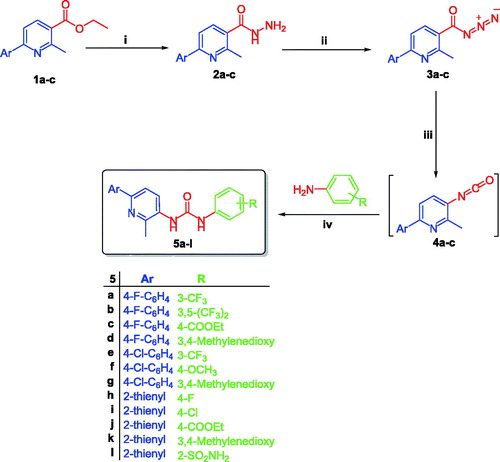
The structures of the newly prepared pyridines 5a–l were confirmed under the basis of spectral and elemental analyses which were in full agreement with the postulated structures (Supplementary Material).
Biological evaluation
In vitro antitumor activity towards 60 cancer cell lines (NCI, USA)
The structures of all the newly synthesized pyridines 5a–l were submitted to the National Cancer Institute (NCI) Developmental Therapeutic Program (www.dtp.nci.nih.gov). Ten pyridines 5a, 5c–j and 5l were chosen to be in vitro evaluated for their antitumor activity. The selected pyridines 5a, 5c–j and 5l were examined at one dose (concentration 10−5 M) primary anticancer assay towards a panel including 85 cancer lines. Nine different types of cancer were tested in this assay: colon, ovarian, prostate, leukemia, melanoma, CNS, renal, breast and lung cancers. A 48 h drug exposure protocol was adopted, and sulforhodamine B (SRB) assayCitation27 was utilized to assess the cell growth and viability. The results were reported as mean-graph of the percentage growth of the treated cells, and displayed as percentage growth inhibition (GI%) caused by the test pyridines ( and ). Investigation of data in and revealed that the examined pyridines exhibited distinctive patterns of sensitivity and selectivity against the different NCI cancer cell panels.
Table 1. Percentage growth inhibition (GI%) of in vitro subpanel tumor cell lines at 10 μM concentration for pyridines 5a and 5c–f.
Table 2. Percentage growth inhibition (GI%) of in vitro subpanel tumor cell lines at 10 μM concentration for pyridines 5g–j and 5l.
Inspecting the GI% values in and , highlighted that compound 5l stood out as the most potent pyridine derivative assayed in this study (mean % GI = 40). Pyridine 5l possessed broad spectrum antitumor activity against all tested cancer cell lines from all subpanels with an exception to non-small cell lung cancer (HOP-92 and NCI-H226), melanoma (MALME-3M), ovarian cancer (OVCAR-5), renal cancer (A498) and breast cancer (HS 578T) cell lines. In particular, 5l showed a potent growth inhibitory activity towards leukemia MOLT-4, non-small cell lung cancer NCI-H460, colon cancer HCT-116 and HCT-15, melanoma LOX IMVI and breast cancer MCF7 cell lines with inhibition % 80, 82, 74, 71, 86 and 75, respectively. In addition, it displayed GI more than 50% over leukemia (CCRF-CEM, K-562, RPMI-8226 and SR), non-small cell lung cancer (A549 and HOP-62), colon cancer (COLO205, HT29, KM12 and SW-620), CNS (SF-295, SF-539 and U251), ovarian (OVCAR-8 and prostate (PC-3) cell lines, .
Figure 2 The most susceptible cancer cell lines towards the impact of target pyridines 5a and 5l according to the GI%.
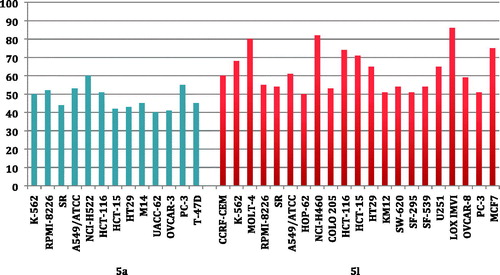
Furthermore, pyridine 5a was found to be the second most active member (mean % GI = 22) with broad spectrum activity against 42 cell lines represent all subpanels. Compound 5a exerted cytotoxic activity with GI more than 40% against leukemia (K-562, RPMI-8226 and SR), non-small cell lung cancer (A549 and NCI-H522), colon cancer (HCT-116, HCT-15 and HT29), melanoma (M14 and UACC-62), ovarian (OVCAR-3), prostate (PC-3) and breast (T-47D) cell lines ().
Further investigation of results in and unveiled that all cell lines of the leukemia subpanel were sensitive to six tested pyridines 5a, 5e, 5f, 5h, 5j and 5l with GI ranging from 10% to 91%. It is noteworthy that only non-small cell lung cancer A549 and NCI-H522 cells were sensitive to all the tested pyridines with GI% range of 10–61% and 24–60%, respectively. Additionally, leukemia SR (except 5i), leukemia HL-60 (except 5d) and colon cancer HT29 (except 5i) cell line were susceptible to nine tested pyridines. The most susceptible cell lines towards the impact of pyridines 5a and 5l are displayed in .
In vitro anti-proliferative activity against A549 and HCT-116 cell lines
All newly synthesized pyridines 5a–l were examined for their anti-proliferative activity towards two cancer cell lines: non-small cell lung cancer A549 cell line and colon cancer HCT-116 cell line. The MTT colorimetric assay was adopted to assess the anti-proliferative activity as described by MosmannCitation29. Doxorubicin was used as a control in this assay. The results were expressed as median growth inhibitory concentration (IC50) values that represent the compound concentration required to produce a 50% inhibition of cell growth after 48 h of incubation ().
Table 3. In vitro anti-proliferative activity of target pyridines 5a–l against A549 and HCT-116 cell lines.
The results of the MTT assay listed in suggested that the examined pyridines 5a–l exhibited excellent to moderate growth inhibitory activity against the tested A549 and HCT-116 cancer cell lines. Also, HCT-116 cells were found to be more sensitive to the impact of the tested compounds than A549 cells, except compound 5j which is more effective towards A549 cells. Interestingly, compound 5l emerged as the most active one towards both A549 and HCT-116 cell lines with IC50 values equal 3.22 ± 0.2 and 2.71 ± 0.16 µM, respectively, which are comparable to those of Doxorubicin: 2.93 ± 0.28and 3.10 ± 0.22, respectively.
Regarding activity against A549 cells, pyridines 5a, 5c, 5f, 5g, 5i and 5j displayed potent antitumor activity with IC50 values in the range of 6.72–9.61 μM, whereas the remaining tested pyridines exhibited moderate potency towards A549 cell line (IC50 range: 10.64–24.05 μM). On the other hand, investigation of the anti-proliferative activity against HCT-116 cell line elucidated that 5a, 5e, 5 h and 5j had potent anti-proliferative activity with IC50 values equal 5.49 ± 0.30, 7.05 ± 0.72, 8.25 ± 0.84 and 9.38 ± 0.67 μM, respectively. Furthermore, pyridines 5b, 5d, 5f and 5k were moderately active towards HCT-116 cells with IC50 values of 16.03 ± 1.52, 10.37 ± 0.84, 12.61 ± 1.08 and 16.43 ± 1.30 μM, respectively.
In vitro cytotoxicity towards non-tumorigenic human WI-38 cells
The cytotoxic activity of all synthesized pyridines 5a–l were assessed against non-tumorigenic human lung fibroblast WI-38 cell line to investigate their safety, using the MTT colorimetric assayCitation29. The results were expressed as IC50 values and the calculated selectivity index are presented in .
Table 4. Cytotoxicity of pyridines 5a–l towards non-tumorigenic human lung fibroblast WI-38 cell line and their selectivity index (S. I.) towards lung A549 cancer cells.
The examined pyridines 5a–l displayed non-significant cytotoxic impact towards human lung fibroblast WI-38 cell line with IC50 values spanning from 63.48 to 151.08 µM, thereby providing a good safety profile as anticancer agents with selectivity index range (6.3–17.6).
Induction of apoptosis in colorectal cancer HCT-116 cells
To investigate the mechanism of antitumor activity of the target pyridines and in continuation of our efforts to develop potent pro-apoptotic anticancer agentsCitation35–39, the ability of sulfonamide 5l to provoke apoptosis in HCT-116 cells was evaluated.
Effects on mitochondrial apoptosis pathway proteins Bcl-2 and Bax
Bcl-2 and Bax are two discrete members of a gene family involved in the regulation of cellular apoptosis known as BcL-2 family, which finely tune the apoptotic switch on/off mechanism and considered as an important gatekeeper to the apoptotic response. While Bcl-2 protein is functionally characterized as an apoptosis-suppressing factor, the Bax protein is more functionally characterized as an apoptosis-promoting factor. So, the intracellular Bax/Bcl-2 ratio can profoundly influence the ability of a cell to respond to an apoptotic signalCitation40,Citation41.
In this study, treatment of HCT-116 cells with the IC50 of pyridine 5l (IC50 = 3.22 ± 0.25 µM) resulted in a significant up-regulation of the expression level of the pro-apoptotic Bax protein by 6-fold compared to untreated control, with a concomitant significant decrease in the expression level of the anti-apoptotic Bcl-2 protein by approximately 75% compared to control (). These results revealed that pyridine 5l significantly boosted the Bax/Bcl-2 ratio 25-fold in compared to control.
Table 5. Impact of pyridine 5l on the expression levels of Bax and Bcl-2 in HCT-116 cancer cells treated with the compound at its IC50 concentration.
Effect on the level of cytochrome C
The interplay between the pro-apoptotic Bax and anti-apoptotic Bcl-2 proteins triggers the activated Bax to bind to the mitochondrial outer membrane which induces the opening of the mitochondrial voltage-dependent anion channel (VDAC), resulting in the release of cytochrome C from mitochondria into cytosol where it activates the caspase-dependent signaling and subsequent apoptosis. Involvement of cytochrome C release from mitochondria is an indicator of activation of the intrinsic apoptotic pathwayCitation42.
Herein, we assessed the expression level of cytochrome C to assure the adoption of the intrinsic pathway. As shown in , the level of cytochrome C was induced significantly higher (12-folds) in HCT-116 cells treated with pyridine 5l, compared to untreated control ().
Table 6. Impact of pyridine 5l on the expression levels of cytochrome C, p53, active caspases-3 and -9, in HCT-116 cancer cells treated with the compound at its IC50 concentration.
Effect on the level of p53
One of the major apoptosis signaling pathways involves the p53 tumor suppressor. The ability of p53 to control apoptosis in response to abnormal proliferative signals and stress is crucial for its tumor suppression role. p53 tumor suppressor protein is a nuclear transcription factor that regulates the expression of a wide variety of genes involved in apoptosis. p53 is able to induce Bax oligomerization and cytochrome c release from mitochondriaCitation43.
The effect of pyridine 5l on p53 expression in HCT-116 cells was evaluated in this study. Results in highlighted that treatment of HCT-116 cells with pyridine 5l led to 21-fold enhanced expression levels of p53, compared to control ().
Effects on the levels of active caspase-3 and caspase-9
Caspases, cysteine-dependent aspartate-directed proteases, are key factors in apoptotic cell death that have been shown to play an important role in cleavage of vital structural and regulatory proteins important for cells survival, so activation of caspases is a hallmark for apoptosis inductionCitation44. The leading upstream caspases are caspase-9 in the intrinsic pathway and caspase-8 in the extrinsic pathway, where both converge to caspase-3 which is the key executioner of apoptosisCitation45.
In comparison with the untreated control, the expression levels of active caspase-3 and caspases-9 in HCT-116 cells were 5.1- and 2.5-fold increased, respectively, in response to pyridine 5l treatment with its IC50 concentration ().
Cell cycle analysis
Targeting the cell cycle of cancer cells has emerged as a promising approach for cancer therapyCitation46. In the current study, pyridine 5l was examined for its effect on the cell cycle distribution in HCT-116 cells (). The results of the DNA flow cytometric assay showed that treatment of HCT-116 cells with pyridine 5l at its IC50 concentration for 24 h resulted in a significant 7.3-fold increased percentage of HCT-116 cells at Sub-G1, with concurrent significant reduction in the G2-M phase by approximately 2.2-fold. Both arrest of G2-M phase and alteration of the Sub-G1 phase are considered significant remarks for pyridine 5l to induce apoptosis in HCT-116 cells.
AnnexinV-FITC/propidium iodide analysis of apoptosis
Translocation of phosphatidylserine (PS) from the inner to the outer membrane leaflet of the cell is an early apoptotic event, which could be detected by fluorescein-labeled annexinV (annexinV-FITC), a Ca2+-dependent phospholipid-binding protein with high affinity for PS. Combined with propidium iodide PI (an indicator of cell integrity), a measure of percentage cell population in early apoptosis can be achieved. Cells displaying increased annexinV-FITC fluorescence without a concurrent increase in PI fluorescence are considered to be in early apoptosis, whereas an increase is seen in both fluorescence channels, signifies a late apoptosisCitation47.
In this study, AnnexinV-FITC/PI dual staining assay was performed to evaluate the effect of compound 5l on both early and late apoptosis percentages in HCT-116 cells (, ). As presented in , the assay outcomes clearly indicate that the treatment of HCT-116 cells with 5l resulted in a significant increase in the percentage of annexinV-FITC-positive apoptotic cells, including both the early and late apoptotic phases (LR; from 1.18% to 6.79%, and UR; from 0.81% to 8.97%), that represents about eightfold total increase in comparison with control ().
Figure 4 Effect of sulfonamide 5l on the percentage of annexin V-FITC-positive staining in HCT-116 cells. The experiments were done in triplicates. The four quadrants identified as: LL: viable; LR: early apoptotic; UR: late apoptotic; UL: necrotic.
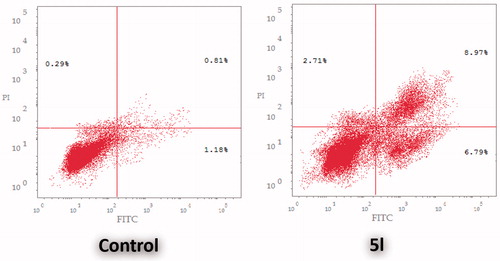
Table 7. Distribution of apoptotic cells in the annexin V-FITC experiment.
Conclusion
In summary, herein we report the synthesis of novel series of 1-(2-methyl-6-arylpyridin-3-yl)-3-phenylureas 5a–l. All the prepared pyridins were evaluated for their in vitro anticancer activity against two cancer cell lines: non-small cell lung cancer A549 cell line and colon cancer HCT-116 cell line. Compound 5l was found to be the most active congener towards both A549 and HCT-116 cell lines with IC50 values equal to 3.22 ± 0.2 and 2.71 ± 0.16 µM, respectively, which are comparable with those of Doxorubicin: 2.93 ± 0.28 and 3.10 ± 0.22, respectively. Furthermore, compound 5l stood out as the most potent pyridine derivative (mean % GI = 40), at US-NCI Developmental Therapeutic Program anticancer assay, with broad-spectrum antitumor activity against the most tested cancer cell lines from all subpanels. The ability of sulfonamide 5l to provoke apoptosis in HCT-116 cells was evaluated. Results revealed that pyridine 5l significantly boosted the Bax/Bcl-2 ratio 25-fold compared to control. Also, the expression levels of cytochrome C, p53, active caspase-3 and caspases-9 in HCT-116 cells were 12-, 21-, 5.1- and 2.5-fold increased, respectively, in response to pyridine 5l treatment. Furthermore, treatment of HCT-116 cells with pyridine 5l at its IC50 concentration resulted in a significant 7.3-fold increased percentage of HCT-116 cells at Sub-G1, with concurrent significant reduction in the G2-M phase by approximately 2.2-fold, in addition to a significant increase in the percentage of annexinV-FITC-positive apoptotic cells, including both the early and late apoptotic phases (LR; from 1.18% to 6.79%, and UR; from 0.81% to 8.97%) that represent about eightfold total increase in comparison with control.
Supplemental Material
Download PDF (434 KB)Supplemental Material
Download PDF (827.1 KB)Disclosure statement
No potential conflict of interest was reported by the authors.
Additional information
Funding
References
- Delbridge AR, Grabow S, Strasser A, Vaux DL. Thirty years of BCL-2: translating cell death discoveries into novel cancer therapies. Nat Rev Cancer 2016;16:99
- Hu W, Kavanagh JJ. Anticancer therapy targeting the apoptotic pathway. Lancet Oncol 2003;4:721–9.
- Lopez J, Tait SWG. Mitochondrial apoptosis: killing cancer using the enemy within. Br J Cancer 2015;112:957
- Wilhelm S, Dumas J, Ladouceur G, Lynch M, Scott W. Diaryl ureas with kinase inhibiting activity. 2007; U.S. Pat 20070020704.
- Skårderud MR, Polk A, Vistisen KK, et al. Efficacy and safety of regorafenib in the treatment of metastatic colorectal cancer: a systematic review. Cancer Treat Rev 2017;62:61–73.
- Zhang L, Yu J. Role of apoptosis in colon cancer biology, therapy, and prevention. Curr Colorectal Cancer Rep 2013;9:331–40.
- Chen D, Wei L, Yu J, Zhang L. Regorafenib inhibits colorectal tumor growth through PUMA-mediated apoptosis. Clin Cancer Res. 2014;20:3472–84.
- Sahu A, Prabhash K, Noronha V, et al. Crizotinib: a comprehensive review. South Asian J Cancer 2013;2:91.
- Frampton JE. Crizotinib: a review of its use in the treatment of anaplastic lymphoma kinase-positive, advanced non-small cell lung cancer. Drugs 2013;73:2031–51.
- Dai X, Guo G, Zou P, et al. (S)-crizotinib induces apoptosis in human non-small cell lung cancer cells by activating ROS independent of MTH1. J Exp Clin Cancer Res 2017;36:120.
- El-Naggar M, Almahli H, Ibrahim HS, et al. Pyridine-ureas as potential anticancer agents: synthesis and in vitro biological evaluation. Molecules 2018;23:1459.
- Eldehna WM, Fares M, Ibrahim HS, et al. Synthesis and biological evaluation of certain hydrazonoindolin-2-one derivatives as new potent antiproliferative agents. J Enz Inhib Med Chem 2018;33:867–78.
- Sabt A, Abdelhafez OM, El-Haggar RS, et al. Novel coumarin-6-sulfonamides as apoptotic anti-proliferative agents: synthesis, in vitro biological evaluation, and QSAR studies. J Enz Inhib Med Chem 2018;33:1095–107.
- Al-Ansary GH, Eldehna WM, Ghabbour HA, et al. Cancer stem cells CD133 inhibition and cytotoxicity of certain 3-phenylthiazolo [3, 2-a] benzimidazoles: design, direct synthesis, crystal study and in vitro biological evaluation. J Enzym Inhib Med Chem 2017;32:986–91.
- Attia MI, Eldehna WM, Afifi SA, et al. New hydrazonoindolin-2-ones: synthesis, exploration of the possible antiproliferative mechanism of action and encapsulation into PLGA microspheres. PLoS One 2017;12:e0181241.
- Abdel-Aziz HA, Eldehna WM, Keeton AB, et al. Isatin-benzoazine molecular hybrids as potential antiproliferative agents: synthesis and in vitro pharmacological profiling. Drug Des Dev Ther 2017;11:2333.
- Eldehna WM, Abou-Seri SM, El Kerdawy AM, et al. Increasing the binding affinity of VEGFR-2 inhibitors by extending their hydrophobic interaction with the active site: design, synthesis and biological evaluation of 1-substituted-4-(4-methoxybenzyl)phthalazine derivatives. Eur J Med Chem 2016;113:50–62.
- Abdel-Aziz HA, Ghabbour HA, Eldehna WM, et al. 2-((Benzimidazol-2-yl)thio)-1-arylethan-1-ones: synthesis, crystal study and cancer stem cells CD133 targeting potential. Eur J Med Chem 2015;104:1–10.
- Abdel-Aziz HA, Ghabbour HA, Eldehna WM, et al. Synthesis, crystal structure and biological activity of cis/trans amide rotomers of (Z)-N′-(2-oxoindolin-3-ylidene)formohydrazide. J Chem 2014;2014:1. doi:10.1155/2014/760434.
- Eldehna WM, Fares M, Ibrahim HS, et al. Synthesis and cytotoxic activity of biphenylurea derivatives containing indolin-2-one moieties. Molecules 2016;21:762.
- Soliman DH, Eldehna WM, Ghabbour HA, et al. Novel 6-phenylnicotinohydrazide derivatives: design, synthesis and biological evaluation as a novel class of antitubercular and antimicrobial agents. Biol Pharm Bull 2017;40:1883–93.
- Eldehna WM, Altoukhy A, Mahrous H, Abdel-Aziz HA. Design, synthesis and QSAR study of certain isatin-pyridine hybrids as potential antiproliferative agents. Eur J Med Chem 2015;90:684–94.
- Eldehna WM, Fares M, Abdel-Aziz MM, Abdel-Aziz HA. Design, synthesis and antitubercular activity of certain nicotinic acid hydrazides. Molecules 2015;20:8800–15.
- Boyd MR, Paull KD. Some practical considerations and applications of the National Cancer Institute in vitro anticancer drug discovery screen. Drug Dev Res 1995;34:91–109.
- Boyd MR. The NCI human tumor cell line (60-Cell) screen. In: Teicher BA, ed. Cancer drug discovery and development: anticancer drug development guide: preclinical screening, clinical trials and approval. Totowa, NJ: Humana Press; 2014:Chapter 1, 41–62.
- Monks A, Scudiero D, Skehan P, et al. Feasibility of a high-flux anticancer drug screen using a diverse panel of cultured human tumor cell lines. J Natl Cancer Inst 1991;83:757–66.
- Skehan P, Storeng R, Scudiero D, et al. New colorimetric cytotoxicity assay for anticancer-drug screening. J. Natl Cancer Inst 1990;82:1107–12.
- Abou-Seri SM, Eldehna WM, Ali MM, El Ella DAA. 1-Piperazinylphthalazines as potential VEGFR-2 inhibitors and anticancer agents: synthesis and in vitro biological evaluation. Eur J Med Chem 2016;107:165–79.
- Mosmann T. Rapid colorimetric assay for cellular growth and survival: application to proliferation and cytotoxicity assays. J Immunol Methods 1983;65:55–63.
- Ismail RSM, Abou-Seri SM, Eldehna WM, et al. Novel series of 6-(2-substitutedacetamido)-4-anilinoquinazolines as EGFR-ERK signal transduction inhibitors in MCF-7 breast cancer cells. Eur J Med Chem 2018;155:782–96.
- Eldehna WM, Abo-Ashour MF, Nocentini A, et al. Novel 4/3-((4-oxo-5-(2-oxoindolin-3-ylidene) thiazolidin-2-ylidene) amino) benzenesulfonamides: synthesis, carbonic anhydrase inhibitory activity, anticancer activity and molecular modelling studies. Eur J Med Chem 2017;139:250–62.
- Eldehna WM, Ibrahim HS, Abdel-Aziz HA, et al. Design, synthesis and in vitro antitumor activity of novel N-substituted-4-phenyl/benzylphthalazin-1-ones. Eur J Med Chem 2015;89:549–60.
- Eldehna WM, Nocentini A, Al-Rashood ST, et al. Tumor-associated carbonic anhydrase isoform IX and XII inhibitory properties of certain isatin-bearing sulfonamides endowed with in vitro antitumor activity towards colon cancer. Bioorg Chem 2018;81:425–32.
- Ghabbour HA, Qabeel MM, Eldehna WM, et al. Design, synthesis, and molecular docking of 1-(1-(4-chlorophenyl)-2-(phenylsulfonyl)ethylidene)-2-phenylhydrazine as potent nonazole anticandidal agent. J Chem 2014;2014:1.
- Eldehna WM, EL-Naggar DH, Hamed AR, et al. One-pot three-component synthesis of novel spirooxindoles with potential cytotoxic activity against triple-negative breast cancer MDA-MB-231 cells. J Enzym Inhib Med Chem 2018;33:309–18.
- Almahli H, Hadchity E, Jaballah MY, et al. Development of novel synthesized phthalazinone-based PARP-1 inhibitors with apoptosis inducing mechanism in lung cancer. Bioorg Chem 2018;77:443–56.
- Eldehna WM, Abo-Ashour MF, Ibrahim HS, et al. [(3-indolylmethylene)hydrazono]indolin-2-ones as apoptotic anti-proliferativeagents: design, synthesis and in vitro biological evaluation. J Enzym Inhib Med Chem 2018;33:686–700.
- El-Naggar M, Eldehna WM, Almahli H, et al. Novel thiazolidinone/thiazolo [3, 2-a] benzimidazolone-isatin conjugates as apoptotic anti-proliferative agents towards breast cancer: one-pot synthesis and in vitro biological evaluation. Molecules 2018;23:1420.
- Abdel-Aziz HA, Eldehna WM, Ghabbour H, et al. Synthesis, crystal study, and anti-proliferative activity of some 2-benzimidazolylthioacetophenones towards triple-negative breast cancer MDA-MB-468 cells as apoptosis-inducing agents. Int J Mol Sci 2016;17:1221.
- Youle RJ, Strasser A. The BCL-2 protein family: opposing activities that mediate cell death. Nat Rev Mol Cell Biol 2008;9:47–59.
- Jiang H, Zhao PJ, Su D, et al. Paris saponin I induces apoptosis via increasing the Bax/Bcl-2 ratio and caspase-3 expression in gefitinib-resistant non-small cell lung cancer in vitro and in vivo. Mol Med Rep 2014;9:2265–72.
- Shimizu S, Narita M, Tsujimoto Y. Bcl-2 family proteins regulate the release of apoptogenic cytochrome c by the mitochondrial channel VDAC. Nature 1999;399:483–7.
- Fridman JS, Lowe SW. Control of apoptosis by p53. Oncogene 2003;22:9030–40.
- McIlwain DR, Berger T, Mak TW. Caspase functions in cell death and disease. Cold Spring Harb Perspect Biol 2013;5:a008656.
- Cohen GM. Caspases: the executioners of apoptosis. Biochem J 1997;326:1–16.
- Evan GI, Vousden KH. Proliferation, cell cycle and apoptosis in cancer. Nature 2001;411:342–8.
- Vermes I, Haanen C, Steffens-Nakken H, Reutellingsperger C. A novel assay for apoptosis flow cytometric detection of phosphatidylserine expression on early apoptotic cells using fluorescein labelled annexin V. J Immunol Methods 1995;184:39–51.