Abstract
A series of benzenesulfonamides incorporating selenazoles with diverse substitution patterns were investigated as inhibitors of six bacterial carbonic anhydrases (CAs, EC 4.2.1.1) from bacterial pathogens, such as Helicobacter pylori (hpCAα was the investigated enzyme), Vibrio cholerae (all the three CAs from this pathogen were considered, VchCAα, VchCAβ and VchCAγ) and Burkholderia pseudomallei (with its two CAs, BpsCAβ and BpsCAγ). All these sulfonamides were effective CA inhibitors, with potencies in the low micromolar or submicromolar range, making them attractive as lead compounds for designing antibacterials with a novel mechanism of action, which could counteract the extensive resistance problem observed with many clinically used antibiotics.
1. Introduction
Carbonic anhydrases (CAs, EC 4.2.1.1) are a superfamily of ubiquitous metalloenzymes which catalyze the simple but physiologically crucial interconversion of carbon dioxide and water, with the formation of bicarbonate and protons: CO2 + H2O ⇌ HCO3− + H+Citation1–11. The hydration/dehydration of CO2 is a key physiological reaction for the life cycle of most organisms, including bacteria, since it is connected with numerous metabolic pathways, such as the biosynthetic processes requiring CO2 or HCO3− (photosynthesis and carboxylation reactions) and biochemical pathways including pH homeostasis, secretion of electrolytes, transport of CO2 and bicarbonate, etc.Citation12,Citation13. shows the transport of carbon dioxide and bicarbonate assisted by bacterial CAs denoting their pivotal role in the bacterial metabolism. In fact, bacteria encode such enzymes belonging to three different genetic families, the α-, β- and γ-CAsCitation11–13.
Figure 1 Proposed role of CAs in Gram-negative bacteria. The α-CAs convert the diffused CO2 inside the periplasmic space into bicarbonate, whereas the cytosolic β-and γ-CAs are responsible for the supplementation of CO2 and bicarbonate for the cellular metabolism. Furthermore, all these enzymes play an important role in the cellular pH homeostasisCitation1.
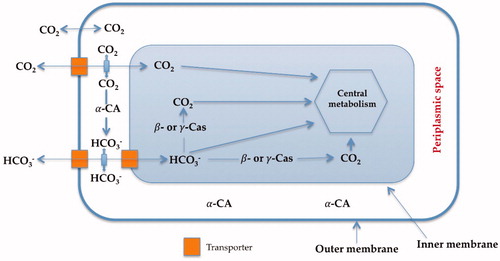
Inhibition of one or more of the CAs present in bacteria was shown to interfere with the growth and/or pathogenicity of many such organismsCitation1–5,Citation13. The reason for that is explained as follows: these enzymes participate in tightly controlled metabolic processes, or in the pH homeostasis, which are highly relevant in all organisms, including bacteriaCitation1–13. It has been demonstrated that the two CAs (α and β) encoded by the genome of the pathogen Helicobacter pylori, a Gram-negative bacterium colonizing the human stomach, are essential for the acid acclimatization of the pathogen within the human stomach and thus, for the bacterial survival in the hostCitation14. On the other hand, Vibrio cholerae (a Gram-negative bacterium provoking cholera) uses its CAs (α, β and γ) for producing sodium bicarbonate, which induces cholera toxin expressionCitation15, and for colonizing the hostCitation16. Once more, the causative agent of brucellosis, Brucella suis, a non-motile Gram-negative coccobacillus, and the Mycobacterium tuberculosis, an obligate pathogenic bacterium responsible for tuberculosis, were needed of functional CAs for growingCitation17–19. Indeed, a large number of interesting studies were dedicated in the last decade for finding effective in vitro CA inhibitors (CAIs) targeting these pathogenic enzymesCitation8–14,Citation17–19, and possibly translating such results to in vivo and eventually clinical activityCitation1. However, CAIs are not yet seriously considered as potential anti-infectives to date, mainly due to the fact that no relevant drug discovery program has been yet started, although these compounds may show indeed a great promise for fighting drug resistant microbes, such as bacteria, fungi and protozoaCitation20.
2. Materials and methods
2.1. Chemistry
Selenazoles 1, 4–5 and 8–11 were reported earlier by our group and were used as followsCitation21.
2.2. CA enzyme inhibition assay
An Sx.18Mv-R Applied Photophysics (Oxford, UK) stopped-flow instrument has been used to assay the catalytic activity of various CA isozymes for CO2 hydration reactionCitation22. Phenol red (at a concentration of 0.2 mM) was used as an indicator, working at the absorbance maximum of 557 nm, with 10 mM Hepes (pH 7.5, for α-CAs) or TRIS (pH 8.3, for β- and γ-CAs) as buffers, 0.1 M Na2SO4 (for maintaining constant ionic strength), following the CA-catalyzed CO2 hydration reaction for a period of 10 s at 25 °C. The CO2 concentrations ranged from 1.7 to 17 mM for the determination of the kinetic parameters and inhibition constants. For each inhibitor, at least six traces of the initial 5–10% of the reaction have been used for determining the initial velocity. The uncatalyzed rates were determined in the same manner and subtracted from the total observed rates. Stock solutions of inhibitors (10 mM) were prepared in distilled-deionized water and dilutions up to 1 nM were done thereafter with the assay buffer. Enzyme and inhibitor solutions were pre-incubated together for 15 min (standard assay at room temperature) prior to assay, in order to allow for the formation of the enzyme–inhibitor complex. The inhibition constants were obtained by non-linear least-squares methods, using GraphPad PRISM 3 and the Cheng–Prusoff equation, as reported earlierCitation23. All CAs were recombinant proteins produced as reported earlier by our groupsCitation9–14,Citation24.
3. Results and discussion
3.1. Chemistry
Selenazoles are an important class of heterocycles with significant biological effects and considerable pharmacological relevanceCitation21,Citation25. Moreover, these five-membered selenium heterocycles are easily synthesized from primary selenoamide bearing benzenesulfonamide 1 as starting materials. This compound has been used for the preparation of various 2,5-disubstituted 1,3-selenazoles 4a-f and 5a-c, as reported earlier (Scheme 1)Citation21.
In order to extend our library of selenazole derivatives, we report the synthesis of a variety of double-functionalized and ionic 1,3-selenazoles, as shown in Scheme 2.
Our main interest was to investigate structure–activity relationship related to the inhibition of different CAs classes from three different pathogenic bacteria: Helicobacter pylori (hpCAα), Vibrio cholerae (VchCAα, VchCAβ and VchCAγ) and, Burkholderia pseudomallei (BpsCAβ and BpsCAγ), relevant pathogens in many diseases for which drug-resistant strains were evidencedCitation1,Citation9–11.
3.2. Carbonic anhydrase inhibition
Selenazole scaffolds showed promising results as antibacterial activityCitation26–28. For this reason, we investigated whether such compounds may act as inhibitors of bacterial CAs, thus being possible candidates for anti-infective studies. We tested in vitro compounds 4–11 for their inhibitory activity against six bacterial enzyme: hpCAα, VchCAα, VchCAβ, VhCAγ, BpsCAβ and BpsCAγ, by means of the stopped-flow carbon dioxide hydration assayCitation22. These activities were compared with those of the standard, clinically used CAI acetazolamide (AAZ) ().
Table 1. Inhibition data against bacterial enzyme hpCAα, VchCAα, VchCAβ, VchCAγ, BpsCAβ and BpsCAγ of derivates 4–5, 8–11 and acetazolamide AAZ by a stopped-flow CO2 hydrase assayCitation22.
As seen from data of , all bacterial enzymes investigated here showed inhibition in the low micromolar or submicromolar range, with all selenazoles incorporating benzenesulfonamide mioietes included in the study.
(i) HpCAα was effectively inhibited, with KIs ranging between 0.79 and 2.84 µM, thus leaving little space to structure–activity relationship (SAR) discussion due to this flat profile. However, all these sulfonamides were at least two orders of magnitude less effective as HpCAα inhibitors compared to acetazolamide, which is a very potent inhibitor of this enzyme.
(ii) VchCAα was inhibited in the submicromolar range by selenazoles 4–11, with KIs ranging between 0.21 and 0.94 µM, again without any relevant SAR, since the activity was very similar for the entire series of derivatives. However, the β- and γ-class enzymes from the same pathogen had a weaker sensitivity to this class of CAIs, since the KIs ranged between 2.61–9.12 µM for VchCAβ, and 3.64–9.29 µM for VchCAγ (). Again, AAZ was a better inhibitor for all these CAs compared to the studied derivatives.
(iii) Some of the selenazole sulfonamides investigated here were, on one hand, more effective BpsCAβ inhibitors compared to the standard drug AAZ, as they showed submicromolar inhibitory power. Among them were 10a (KI of 90 nM), 9b (KI of 320 nM), 5b and 5c (KIs of 0.33–0.36 µM), compared to the KI of 0.74 µM of acetazolamide. These data show that small structural differences lead to drastic effects on the CA inhibition. For example, 10b, possessing an extra methyl group compared to 10a, was 52 times a weaker BpsCAβ inhibitor compared to 10a. BpsCAγ was, on the other hand, less sensitive to these inhibitors compared to the β-class enzyme from the same pathogen, although some compounds (4a–4e, 10c) were submicromolar inhibitors, with KIs ranging between 0.70 and 0.91 µM.
4. Conclusions
A series of benzenesulfonamides incorporating selenazoles with diverse substitution patterns were investigated as inhibitors of six bacterial CAs from pathogens such as Helicobacter pylori (tested enzyme was hpCAα), Vibrio cholerae (all the three CAs from this pathogen were considered, VchCAα, VchCAβ and VchCAγ) and Burkholderia pseudomallei (with its two CAs, BpsCAβ and BpsCAγ). All these sulfonamides were effective inhibitors, with potencies in the low micromolar or submicromolar range, making this class of CA inhibitors attractive as lead compounds for designing antibacterials with a novel mechanism of action, which could counteract the extensive antibiotic resistance problem encountered with most clinically used such drugs.
Disclosure statement
No potential conflict of interest was reported by the authors.
Additional information
Funding
References
- (a) Supuran CT. Carbonic anhydrase inhibitors and their potential in a range of therapeutic areas. Expert Opin Ther Pat 2018;28:709–12. (b) Supuran CT. Applications of carbonic anhydrases inhibitors in renal and central nervous system diseases. Expert Opin Ther Pat 2018;28:713–21. (c) Nocentini A, Supuran CT. Carbonic anhydrase inhibitors as antitumor/antimetastatic agents: a patent review (2008–2018). Expert Opin Ther Pat 2018;28:729–40. (d) Supuran CT, Capasso C. Biomedical applications of prokaryotic carbonic anhydrases. Expert Opin Ther Pat 2018;28:745–54.
- (a) Supuran CT. Carbonic anhydrases: from biomedical applications of the inhibitors and activators to biotechnological use for CO2 capture. J Enzyme Inhib Med Chem 2013;28:229–30. (b) Supuran CT. How many carbonic anhydrase inhibition mechanisms exist? J Enzyme Inhib Med Chem 2016; 31:345–60. (c) Alterio V, Di Fiore A, D’Ambrosio K, et al. Multiple binding modes of inhibitors to carbonic anhydrases: how to design specific drugs targeting 15 different isoforms? Chem Rev 2012;112:4421–68. (d) Capasso C, Supuran CT. An overview of the alpha-, beta-and gamma-carbonic anhydrases from Bacteria: can bacterial carbonic anhydrases shed new light on evolution of bacteria? J Enzyme Inhib Med Chem 2015;30:325–32.
- (a) Supuran CT. Advances in structure-based drug discovery of carbonic anhydrase inhibitors. Expert Opin Drug Discov 2017;12:61–88. (b) Supuran CT. Structure and function of carbonic anhydrases. Biochem J 2016;473:2023–32. (c) Supuran CT. Carbonic anhydrases: novel therapeutic applications for inhibitors and activators. Nat Rev Drug Discov 2008;7:168–81. (d) Neri D, Supuran CT. Interfering with pH regulation in tumours as a therapeutic strategy. Nat Rev Drug Discov 2011;10:767–77. (e) Supuran CT, Vullo D, Manole G, et al. Designing of novel carbonic anhydrase inhibitors and activators. Curr Med Chem Cardiovasc Hematol Agents 2004;2:49–68.
- (a) Carta F, Supuran CT. Diuretics with carbonic anhydrase inhibitory action: a patent and literature review (2005–2013). Expert Opin Ther Pat 2013;23:681–91. (b) Temperini C, Cecchi A, Scozzafava A, Supuran CT. Carbonic anhydrase inhibitors. Sulfonamide diuretics revisited—old leads for new applications? Org Biomol Chem 2008;6:2499–506.
- (a) Masini E, Carta F, Scozzafava A, Supuran CT. Antiglaucoma carbonic anhydrase inhibitors: a patent review. Expert Opin Ther Pat 2013;23:705–16. (b) Borras J, Scozzafava A, Menabuoni L, et al. Carbonic anhydrase inhibitors: synthesis of water-soluble, topically effective intraocular pressure lowering aromatic/heterocyclic sulfonamides containing 8-quinoline-sulfonyl moieties: is the tail more important than the ring? Bioorg Med Chem 1999;7:2397–406.
- (a) Scozzafava A, Supuran CT, Carta F. Antiobesity carbonic anhydrase inhibitors: a literature and patent review. Expert Opin Ther Pat 2013;23:725–35. (b) Supuran CT. Carbonic anhydrases and metabolism. Metabolites 2018;8:25.
- (a) Monti SM, Supuran CT, De Simone G. Anticancer carbonic anhydrase inhibitors: a patent review (2008–2013). Expert Opin Ther Pat 2013;23:737–49. (b) Supuran CT. Carbonic anhydrase inhibition and the management of hypoxic tumors. Metabolites 2017; 7:E48. (c) Ward C, Langdon SP, Mullen P, Harris AL, Harrison DJ, Supuran CT, Kunkler IH. New strategies for targeting the hypoxic tumour microenvironment in breast cancer. Cancer Treat Rev 2013; 39:171–9. (d) Garaj V, Puccetti L, Fasolis G, et al. Carbonic anhydrase inhibitors: novel sulfonamides incorporating 1,3,5-triazine moieties as inhibitors of the cytosolic and tumour-associated carbonic anhydrase isozymes I, II and IX. Bioorg Med Chem Lett 2005;15:3102–8. (e) Casey JR, Morgan PE, Vullo D, et al. Carbonic anhydrase inhibitors. Design of selective, membrane-impermeant inhibitors targeting the human tumor-associated isozyme IX. J Med Chem 2004;47:2337–47.
- (a) Annunziato G, Angeli A, D'Alba F, et al. Discovery of new potential anti-infective compounds based on carbonic anhydrase inhibitors by rational target-focused repurposing approaches. ChemMedChem 2016;11:1904–14. (b) Ozensoy Guler O, Capasso C, Supuran CT. A magnificent enzyme superfamily: Carbonic anhydrases, their purification and characterization. J Enzyme Inhib Med Chem 2016;31:689–94. (c) Del Prete S, Vullo D, De Luca V, et al. Sulfonamide inhibition studies of the beta-carbonic anhydrase from the pathogenic bacterium Vibrio cholerae. Bioorg Med Chem 2016;24:1115–20.
- (a) Capasso C, Supuran CT. An overview of the carbonic anhydrases from two pathogens of the oral cavity: Streptococcus mutans and Porphyromonas gingivalis. Curr Top Med Chem 2016;16:2359–68. (b) Del Prete S, Vullo D, De Luca V, et al. Anion inhibition profiles of alpha-, beta- and gamma-carbonic anhydrases from the pathogenic bacterium Vibrio cholerae. Bioorg Med Chem 2016;24:3413–7. (c) Del Prete S, Vullo D, De Luca V, et al. Comparison of the sulfonamide inhibition profiles of the alpha-, beta- and gamma-carbonic anhydrases from the pathogenic bacterium Vibrio cholerae. Bioorg Med Chem Lett 2016;26:1941–6.
- (a) Nishimori I, Onishi S, Takeuchi H, Supuran CT. The alpha and beta classes carbonic anhydrases from Helicobacter pylori as novel drug targets. Curr Pharm Des 2008;14:622–30. (b) Capasso C, Supuran CT. Bacterial, fungal and protozoan carbonic anhydrases as drug targets. Expert Opin Ther Targets 2015;19:1689–704. (c) Capasso C, Supuran CT. An overview of the selectivity and efficiency of the bacterial carbonic anhydrase inhibitors. Curr Med Chem 2015;22:2130–9.
- (a) Annunziato G, Giovati L, Angeli A, et al. Discovering a new class of antifungal agents that selectively inhibits microbial carbonic anhydrases. J Enzyme Inhib Med Chem 2018;33:1537–44. (b) Güzel-Akdemir Ö, Angeli A, Demir K, et al. Novel thiazolidinone-containing compounds, without the well-known sulphonamide zinc-binding group acting as human carbonic anhydrase IX inhibitors. J Enzyme Inhib Med Chem 2018;33:1299–308. (c) Murray AB, Aggarwal M, Pinard M, et al. Structural mapping of anion inhibitors to β-carbonic anhydrase psca3 from Pseudomonas aeruginosa. ChemMedChem 2018;13:2024–9. (d) Ferraroni M, Del Prete S, Vullo D, et al. Crystal structure and kinetic studies of a tetrameric type ii beta-carbonic anhydrase from the pathogenic bacterium Vibrio cholerae. Acta Cryst D, Biol Cryst 2015;71:2449–56.
- (a) Berrino E, Bozdag M, Del Prete S, et al. Inhibition of α-, β-, γ-, and δ-carbonic anhydrases from bacteria and diatoms with N'-aryl-N-hydroxy-ureas. J Enzyme Inhib Med Chem 2018; 33:1194–8. (b) Bua S, Berrino E, Del Prete S, et al. Synthesis of novel benzenesulfamide derivatives with inhibitory activity against human cytosolic carbonic anhydrase I and II and Vibrio cholerae α- and β-class enzymes. J Enzyme Inhib Med Chem 2018;33:1125–36. (c) Angeli A, Abbas G, Del Prete S, et al. Selenides bearing benzenesulfonamide show potent inhibition activity against carbonic anhydrases from pathogenic bacteria Vibrio cholerae and Burkholderia pseudomallei. Bioorg Chem 2018;79:319–22. (d) Del Prete S, Isik S, Vullo D, et al. DNA cloning, characterization, and inhibition studies of an alpha-carbonic anhydrase from the pathogenic bacterium Vibrio cholerae. J Med Chem 2012;55:10742–8.
- (a) Wani TV, Bua S, Khude PS, et al. Evaluation of sulphonamide derivatives acting as inhibitors of human carbonic anhydrase isoforms I, II and Mycobacterium tuberculosis β-class enzyme Rv3273. J Enzyme Inhib Med Chem 2018;33:962–71. (b) Stefanucci A, Angeli A, Dimmito MP, et al. Activation of β- and γ-carbonic anhydrases from pathogenic bacteria with tripeptides. J Enzyme Inhib Med Chem 2018;33:945–50. (c) Supuran CT, Capasso C. An overview of the bacterial carbonic anhydrases. Metabolites 2017;7:E56.
- (a) Modak JK, Liu YC, Machuca MA, et al. Structural basis for the inhibition of Helicobacter pylori α-carbonic anhydrase by sulfonamides. PloS One 2015;10:e0127149 (b) Buzás GM, Supuran CT. The history and rationale of using carbonic anhydrase inhibitors in the treatment of peptic ulcers. In memoriam Ioan Puşcaş (1932–2015). J Enzyme Inhib Med Chem 2016;31:527–33.
- Cobaxin M, Martinez H, Ayala G, et al. Cholera toxin expression by El Tor Vibrio cholerae in shallow culture growth conditions. Microb Pathog 2014;66:5–13.
- Abuaita BH, Withey JH. Bicarbonate induces Vibrio cholerae virulence gene expression by enhancing toxt activity. Infect Immun 2009;77:4111–20.
- (a) Nishimori I, Vullo D, Minakuchi T, et al. Carbonic anhydrase inhibitors: cloning and sulfonamide inhibition studies of a carboxyterminal truncated alpha-carbonic anhydrase from Helicobacter pylori. Bioorg Med Chem Lett 2006;16:2182–8. (b) Kohler S, Ouahrani-Bettache S, Winum JY. Brucella suis carbonic anhydrases and their inhibitors: towards alternative antibiotics? J Enzyme Inhib Med Chem 2017;32:683–7.
- Nishimori I, Minakuchi T, Maresca A, et al. The β-carbonic anhydrases from Mycobacterium tuberculosis as drug targets. Curr Pharm Des 2010;16:3300–9.
- Gu¨zel O¨z, Maresca A, Scozzafava A, et al. Discovery of low nanomolar and subnanomolar inhibitors of the mycobacterial beta-carbonic anhydrases Rv1284 and Rv3273. J Med Chem 2009; 52:4063–7 (b) Minakuchi T, Nishimori I, Vullo D, et al. Molecular cloning, characterization, and inhibition studies of the Rv1284 beta-carbonic anhydrase from Mycobacterium tuberculosis with sulfonamides and a sulfamate. J Med Chem 2009;52:2226–32. (c) Nishimori I, Minakuchi T, Vullo D, et al. Carbonic anhydrase inhibitors. Cloning, characterization, and inhibition studies of a new beta-carbonic anhydrase from Mycobacterium tuberculosis. J Med Chem 2009;52:3116–20.
- (a) Capasso C, Supuran CT. Bacterial carbonic anhydrases. In: Supuran CT, Capasso C, eds. Zinc enzyme inhibitors – volume 1: enzymes from microorganisms. Topics in Medicinal Chemistry. Vol 22. Cham: Springer; 2017:135–52. (b) Supuran CT, Capasso C. Protozoan carbonic anhydrases. In: Supuran CT, Capasso C, eds. Zinc enzyme inhibitors – volume 1: enzymes from microorganisms. Topics in Medicinal Chemistry. Vol 22. Cham: Springer; 2017:114–34. (c) Lehneck R, Poggeler S. Fungal carbonic anhydrases and their inhibition. In: Supuran CT, Capasso C, eds. Zinc enzyme inhibitors – volume 1: enzymes from microorganisms. Topics in Medicinal Chemistry. Vol 22. Cham: Springer; 2017:95–110.
- Angeli A, Trallori E, Ferraroni M, et al. Discovery of new 2, 5-disubstituted 1,3-selenazoles as selective human carbonic anhydrase IX inhibitors with potent anti-tumor activity. Eur J Med Chem 2018; 157:1214–22.
- Khalifah RG. The carbon dioxide hydration activity of carbonic anhydrase. I. Stop-flow kinetic studies on the native human isoenzymes B and C. J Biol Chem 1971; 246:2561–73.
- (a) Menchise V, De Simone G, Alterio V, et al. Carbonic anhydrase inhibitors: stacking with Phe131 determines active site binding region of inhibitors as exemplified by the X-ray crystal structure of a membrane-impermeant antitumor sulfonamide complexed with isozyme II. J Med Chem 2005;48:5721–7. (b) Supuran CT, Mincione F, Scozzafava A, et al. Carbonic anhydrase inhibitors—part 52. Metal complexes of heterocyclic sulfonamides: a new class of strong topical intraocular pressure-lowering agents in rabbits. Eur J Med Chem 1998;33:247–54. (c) Şentürk M, Gülçin İ, Beydemir Ş, et al. In vitro inhibition of human carbonic anhydrase I and II isozymes with natural phenolic compounds. Chem Biol Drug Des 2011;77:494–9.
- (a) Krall N, Pretto F, Decurtins W, et al. A small-molecule drug conjugate for the treatment of carbonic anhydrase IX expressing tumors. Angew Chem Int Ed Engl 2014;53:4231–5. (b) Rehman SU, Chohan ZH, Gulnaz F, Supuran CT. In-vitro antibacterial, antifungal and cytotoxic activities of some coumarins and their metal complexes. J Enzyme Inhib Med Chem 2005;20:333–40. (c) Clare BW, Supuran CT. Carbonic anhydrase activators. 3: structure‐activity correlations for a series of isozyme II activators. J Pharm Sci 1994;83:768–73. (d) Dubois L, Peeters S, Lieuwes NG, et al. Specific inhibition of carbonic anhydrase IX activity enhances the in vivo therapeutic effect of tumor irradiation. Radiother Oncol 2011;99:424–31. (e) Chohan ZH, Munawar A, Supuran CT. Transition metal ion complexes of Schiff-bases. Synthesis, characterization and antibacterial properties. Met Based Drugs 2001;8:137–43. (f) Zimmerman SA, Ferry JG, Supuran CT. Inhibition of the archaeal β-class (Cab) and γ-class (Cam) carbonic anhydrases. Curr Top Med Chem 2007;7:901–8.
- Zhao HC, Shi YP, Liu YM, et al. Synthesis and antitumor-evaluation of 1,3-selenazole-containing 1,3,4-thiadiazole derivatives. Bioorg Med Chem Lett 2013;23:6577–9.
- Łączkowski KZ, Biernasiuk A, Baranowska-Łączkowska A, et al. Synthesis, antimicrobial and anticonvulsant screening of small library of tetrahydro-2H-thiopyran-4-yl based thiazoles and selenazoles. J Enzyme Inhib Med Chem 2016;31:24–39.
- Mbaveng AT, Ignat AG, Ngameni B, et al. In vitro antibacterial activities of p-toluenesulfonyl-hydrazinothiazoles and hydrazinoselenazoles against multi-drug resistant Gram-negative phenotypes. BMC Pharmacol Toxicol 2016;17:e3.
- Koketsu M, Ishihara H, Hatsu M. Novel compounds, 1,3-selenazine derivatives, as antibacterial agents against Escherichia coli and Staphylococcus aureus. Res Commun Mol Pathol Pharmacol 1998;101:179–86.