Abstract
Some new 3H-quinazolin-4-one derivatives were synthesised and screened for anticancer, antiphospholipases, antiproteases, and antimetabolic syndrome activities. Compound 15d was more potent in reducing the cell viabilities of HT-29 and SW620 cells lines to 38%, 36.7%, compared to 5-FU which demonstrated cell viabilities of 65.9 and 42.7% respectively. The IC50 values of 15d were ∼20 µg/ml. Assessment of apoptotic activity revealed that 15d decreased the cell viability by down regulating Bcl2 and BclxL. Moreover, compounds, 8j, 8d/15a/15e, 5b, and 8f displayed lowered IC50 values than oleanolic acid against proinflammatory isoforms of hGV, hG-X, NmPLA2, and AmPLA2. In addition, 8d, 8h, 8j, 15a, 15b, 15e, and 15f showed better anti-α-amylase than quercetin, whereas 8g, 8h, and 8i showed higher anti-α-glucosidase activity than allopurinol. Thus, these compounds can be considered as potential antidiabetic agents. Finally, none of the compounds showed higher antiproteases or xanthine oxidase activities than the used reference drugs.
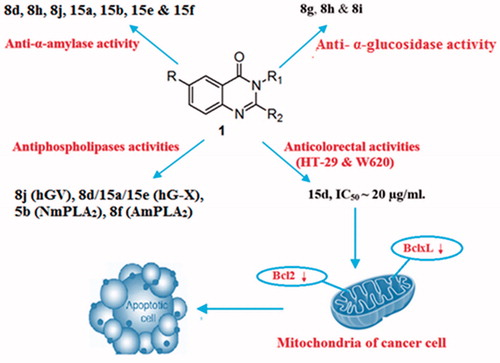
Introduction
Colorectal cancer (CRC) is the third-most common cancer and the fourth-most common cause of cancer-related death worldwideCitation1. One of the key features of cancer is deregulation of apoptosis of the altered cellsCitation2. Apoptosis is mediated by a complex network of BCL-2 family of proteins, comprising two types of proteins, namely anti-apoptotic proteins, which include Bcl2, BclxL, and Mcl-1, and pro-apoptotic proteins, which include Bax and BakCitation3. Hence, inhibiting the anti-apoptotic BCL-2 proteins can be established as a mechanism of anticancer defence for the treatment of CRCCitation4.
Several in vitro and in vivo clinical studies have suggested a positive correlation between the activity of certain proteases, such as trypsinCitation5, cathepsin BCitation6, collagenasesCitation7, thrombinCitation8, and neutrophil elastaseCitation9 and colorectal carcinogenesis, invasion, proliferation, and metastasis.
Furthermore, three phospholipases, namely (hsPLA2-IIA), group V, and group XCitation10 are found to paly indirect pro-tumorigenic roles by promoting chronic inflammatory bowel diseases (IBD) that are common risk factors associated with CRCCitation11.
In addition, recent studies have linked the incidence of CRC to certain metabolic syndrome components, especially elevated plasma glucose (type 2 diabetes mellitus)Citation12. Several enzymes are reported to be involved in glucose homeostasis, among them α-amylase, α-glucosidaseCitation13, and xanthine oxidaseCitation14. Hence, targeting these enzymes has high potential in preventing CRC.
The 3H-quinazolin-4-one scaffold is known to be an important building block of various bioactive natural productsCitation15. In addition to this, many marketed drugs or experimental drug candidates incorporate this pharmacophore as part of the overall topography of the moleculesCitation16 such as ispinesib, nolatrexed, and raltitrexed (the anticancer agents); afloqualone with sedative and muscle relaxant effects, balaglitazone with antidiabetic activities, proquazone (as non-steroidal anti-inflammatory agent), and elinogrel (as reversible P2Y12 receptor antagonist) which is used for the treatment of acute coronary syndrome. Moreover, several new methodologies have been developed for the synthesis of 4-quinazolinone derivatives either using one pot multicomponent approachesCitation17 or via multistep routes for investigating their pharmacological potential as dual EGFR/HER2 inhibitorsCitation18, phosphoinositide 3-kinase inhibitorsCitation19, and as antihypertensiveCitation20, antioxidantCitation21, anticonvulsantCitation22, and anti-inflammatoryCitation23 agents.
Based on these observations, and in continuation to our ongoing research programme aims to identify new bioactive moleculesCitation24,Citation25 herein, we report the synthesis, characterisation, and cytotoxicity screening (including evaluation of apoptotic activity), for a new series of 3H-quinazolin-4-one derivatives against HT-29 and SW620 cell lines derived from CRC. Furthermore, the inhibitory potentials of the synthesised compounds against certain phospholipases and proteases implicated in cancer and inflammatory disorders, in addition to α-amylase, α-glucosidase, and xanthine oxidase, managing metabolic syndrome are investigated with the aim of eradicating the risk factors for CRC or combatting the disease.
Materials and methods
Chemistry
The reactions were monitored and the purity of the final products was checked using thin layer chromatography (TLC) on pre-coated silica gel sheets (60 F254, Merck, Kenilworth, NJ). The developed plates were examined by exposure to ultraviolet light using VL-6.LC (254, 365 nm, 50/60 Hz). All the melting points were determined in open capillary tubes with a Gallenkamp melting point apparatus (°C). The infra-red (IR) spectra were recorded on a Perkin Elmer Fourier transform infra-red (FTIR) spectrophotometer (Spectrum BX 1000) in wave numbers (cm−1) with potassium bromide (KBr) discs. Nuclear magnetic resonance (NMR) spectra were recorded on a Bruker NMR spectrometer: 1-Ascend 850 MHz for 1H and 213 MHz for 13C, or on 2-Avance 600 MHz for 1H and 150 MHz for 13C (Nuclear Magnetic Resonance Center, KAU, Jeddah, KSA), or on a Bruker Avance 500 spectrometer operating at 500 MHz for 1H and 125 MHz for 13C at 25 °C (Research Unit, College of Pharmacy, Prince Sattam Bin Abdulaziz University, AlKharj, KSA), or on an Eclipse 300 FT NMR spectrometer operating at 300 MHz spectrometer for 1H and at 75 MHz for 13C at 25 °C (KSU, Riyadh, KSA). The chemical shifts were expressed in (ppm) using tetramethylsilane as the internal standard; coupling constants (J) were expressed in Hz. Deuterochloroform (CDCl3) was used as solvent. The splitting patterns (multiplicities) in 1HNMR were designated as: singlet (s), broad singlet (br. s), doublet (d), double of doublet (dd), triplet (t), and multiplet (m). Mass spectra were recorded on Shimadzu Qp-2010 Plus mass spectrometer in ionisation mode: EI (Micro Analytical Center, Cairo University, Egypt). Elemental analyses were obtained from the Microanalytical Data Unit at Cairo University, Giza, Egypt.
Compounds 3a,bCitation26, 3cCitation27, 3dCitation28, 6aCitation29, 6bCitation30, 13a, 13b, and 15aCitation31 were reported previously.
General procedure for the preparation of compounds 5a–cCitation32,Citation33
A mixture of the appropriate 2-methyl-benzo[d][1,3]oxazin-4-one derivative 3a-c (0.003 mol) and 3,4,5-trimethoxyaniline 4 (0.003 mol, 0.55 g) was fused in oil bath at 200 °C for 2 h. After cooling down the temperature to 100 °C, 37% HCl (Conc) (3 ml) was added till all the material was dissolved completely. The reaction mixture was cooled down to room temperature and was made alkaline with diluted NaOH solution (25%). The crude precipitated solid in each case, was filtered, washed with water, dried, and recrystallised from ethanol to yield compounds 5a–c.
2 -Methyl-3-(3,4,5-trimethoxy-phenyl)-3H-quinazolin-4-one (5a)
Black powder, yield (53%), mp 88–90 °C; νmax (KBr)/cm−1 3080 (CH-Ar), 2935 & 2837 (CH-aliphatic), 2368, 1682 (C = O), 1600 & 1503 (C = C), 1465, 1422, 1376, 1321, 1233, 1124, 1002, 936, 835, 771, 700, 653, 524; δH (850 MHz; CDCl3) 8.26 (1H, d, J= 7.6, CH5-quinazolin-4-one), 7.77–7.75 (1H, m, CH6-quinazolin-4-one), 7.66 (1H, dd, J= 3.4, 8.5, CH8-quinazolin-4-one), 7.47–7.45 (1H, m, CH7-quinazolin-4-one), 6.47 (2H, s, H2' and H6'-trimethoxyphenyl), 3.90 (3H, s, OCH3), 3.84 (6H, s, 2 × OCH3), 2.32 (3H, s, CH3); δC (213 MHz; CDCl3) 162.36 (C = O), 154.41 (C = N), 154.22, 147.41, 138.46, 134.70, 133.29, 127.04, 126.79, 126.73, 120.69, 105.19 (6 × CH-Ar & 6 × Cq-Ar), 60.96 (OCH3), 56.27 (2 × OCH3), 24.04 (CH3); MS (EI) m/z (%) [M+ + 2] 328.10 (2.50), [M+ + 1] 327.10 (16.69), [M+] 326.10 (77.52), 311.10 (46.20), 297.10 (3.85), 279.05 (2.13), 265.05 (1.90), 183.05 (4.87), 168.10 (7.32), 147.65 (8.78), 144.10 (14.54), 143.10 (100.00), 134.10 (4.69), 116.10 (6.24), 102.05 (4.96), 77.00 (6.38), 57.05 (3.38). Anal. calcd. for C18H18N2O4 (326.13): C, 66.25; H, 5.56; N, 8.58. Found: C, 66.23; H, 5.46; N, 8.66.
6 -Fluoro-2-methyl-3–(3,4,5-trimethoxy-phenyl)-3H-quinazolin-4-one (5b)
Black crystals, yield (55%), mp 193–195 °C; νmax (KBr)/cm−1 3079 (CH-Ar), 2931 & 2835 (CH-aliphatic), 2375, 2278, 1673 (C = O), 1601 & 1484 (C = C), 1425, 1374, 1339, 1313, 1235, 1183, 1125 (C–F), 1065, 1008, 966, 878, 843, 778, 752, 723, 674, 626, 558, 526, 458; δH (500 MHz; CDCl3) 7.84–7.82 (1H, m, CH5-quinazolin-4-one), 7.67–7.65 (1H, m, CH7-quinazolin-4-one), 7.49–7.45 (1H, m, CH8-quinazolin-4-one), 6.52 (2H, s, H2' and H6'-trimethoxyphenyl), 3.91 (3H, s, OCH3), 3.86 (6H s, 2 × OCH3), 2.32 (3H, s, CH3); δC (125 MHz; CDCl3) 161.62 (C = O), 159.65 (Cq-F), 154.20 (C = N), 153.65, 144.06, 138.48, 133.03, 129.23, 123.18, 122.99, 121.87, 105.12 (5 × CH-Ar & 6 × Cq-Ar), 60.89 (OCH3), 56.24 (2 × OCH3), 23.87 (CH3); MS (EI) m/z (%) [M+ + 1] 345.10 (21.69), [M+] 344.10 (100) 330.10 (11.87), 329.10 (57.89), 313.10 (3.99), 297.05 (2.71), 161.05 (86.15), 162.05 (11.84), 134.10 (7.80), 107.05 (4.64), 94.05 (7.76), 77.00 (1.91), 57.00 (3.40). Anal. calcd. for C18H17FN2O4 (344.12): C, 62.79; H, 4.98; N, 8.14. Found: C, 62.68; H, 4.63; N, 8.02.
6 -Chloro-2-methyl-3–(3,4,5-trimethoxy-phenyl)-3H-quinazolin-4-one (5c)
Black powder, yield (60%), mp 188–190 °C; νmax (KBr)/cm−1 3075 (CH-Ar), 2934 & 2839 (CH-aliphatic), 2369, 1680 (C = O), 1598 & 1504 (C = C), 1467, 1423, 1375, 1312, 1236, 1126, 998, 948, 892, 834 (C–Cl), 771, 738, 692, 613, 524, 452; δH (500 MHz; CDCl3) 8.18 (1H, d, J= 2.4, CH5-quinazolin-4-one), 7.69 (1H, dd, J= 8.7, 2.4, CH7-quinazolin-4-one), 7.60 (1H, d, J= 8.7, CH8-quinazolin-4-one), 6.51 (2H, s, H2' and H6'-trimethoxyphenyl), 3.92 (3H, s, OCH3), 3.88 (6H, s, 2 × OCH3), 2.32 (3H, s, CH3); δC (125 MHz; CDCl3) 161.28 (C = O), 154.75 (C = N), 154.25, 145.91, 138.56, 135.02, 132.95, 132.36, 128.52, 126.23, 121.73, 105.09 (5 × CH-Ar & 7 × Cq-Ar), 60.93 (OCH3), 56.28 (2 × OCH3), 24.02 (CH3); MS (EI) m/z (%) [M+, Cl37] 362.10 (34.88), [M+, Cl35] 360.10 (100.00), 347.05 (22.20), 345.10 (60.80), 331.05 (4.80), 329.05 (4.53), 315.05 (1.90), 313.05 (3.09), 301.05 (1.04), 299.10 (1.56), 179.00 (27.46), 177.00 (84.58), 152.05 (3.81), 150.05 (6.54), 136.05 (5.31), 123.05 (3.29), 110.00 (6.17), 75.00 (6.71), 57.10 (3.05). Anal. calcd. for C18H17ClN2O4 (360.09): C, 59.92; H, 4.75; N, 7.76. Found: C, 59.88; H, 4.62; N, 7.59.
Synthesis of Schiff’s base derivatives (8a–j)Citation34
A mixture of 3-amino-2-methyl-3H-quinazolin-4-one derivatives 6a,b (0.002 mol) and the appropriate aromatic aldehyde 7a–e, namely, 2-naphthaldehyde, 4-(pyridin-2-yl) benzaldehyde, 3-methoxy-2-nitrobenzaldehyde, 4-formylbenzonitrile, and 1,1′-biphenyl]-4-carbaldehyde (0.002 mol) in absolute ethanol (20 ml) containing (3 ml) glacial acetic acid was heated under reflux for 10 h. The excess solvent was removed under reduced pressure. The obtained solid in each case was washed with water, air dried, and recrystallised from the appropriate solvent to obtain the corresponding Schiff's base.
(E)-2-Methyl-3-[(naphthalen-2-ylmethylene)-amino]-3H-quinazolin-4-one (8a)
White powder (ethanol), yield (74%), mp 163–165 °C; νmax (KBr)/cm−1; 3062 (CH-Ar), 2929 (CH-alipahtic), 2371, 2340, 1667 (C = O), 1596 & 1468 (C = C), 1426, 1377, 1346, 1320, 1268, 1227, 1156, 1121, 1031, 934, 898, 866, 831, 778, 755, 693, 660, 621, 477; δH (600 MHz; CDCl3) 9.18 (1H, s, CH = N), 8.30 (1H, d, J= 8.4, CH-Ar), 8.16–8.14 (2H, m, 2 × CH-Ar), 7.93–7.92 (2H, m, 2 × CH-Ar), 7.89 (1H, d, J= 7.8, CH-Ar), 7.75 (1H, d, J= 7.2, CH-Ar), 7.67 (1H, d, J= 8.4, CH-Ar), 7.59 (1H, t, J= 7.2, CH-Ar), 7.55 (1H, t, J= 7.8, CH-Ar), 7.47 (1H, t, J= 7.8, CH-Ar), 2.70 (3H, s, CH3); δC (150 MHz; CDCl3) 166.32 (C = O), 158.84 (HC = N), 154.21 (Cq=N), 146.49, 135.41, 134.37, 132.93, 132.42, 130.46, 128.97, 128.95, 128.20, 128.02, 127.30, 126.95, 126.94, 126.46, 122.88, 121.58 (11 × CH-Ar & 5 × Cq-Ar), 23.00 (CH3); MS (EI) m/z (%) [M+ + 1] 314.10 (0.44), [M+] 313.10 (1.41), 161.05 (10.24), 160.10 (100.00), 153.10 (14.99), 139.10 (4.10), 127.10 (8.79), 118.10 (11.47), 90.05 (11.47), 76.00 (13.65), 63.00 (2.80), 50.00 (3.12). Anal. calcd. for C20H15N3O (313.12): C, 76.66; H, 4.82; N, 13.41. Found: C, 76.62; H, 4.77; N, 13.29.
(E)-6-Bromo-2-methyl-3-[(naphthalen-2-ylmethylene)-amino]-3H-quinazolin-4-one (8b)
White powder (ethanol), yield (65%), mp 200 °C; νmax (KBr)/cm−1 3056 (CH-Ar), 2926 (CH-aliphatic), 1932, 1675 (C = O), 1602 & 1465 (C = C), 1371, 1347, 1313, 1272, 1235, 1208, 1180, 1153, 1122, 1072, 1037, 954, 890, 860, 831, 812, 777, 740, 700, 672 (C-Br), 629, 569, 539, 514, 473; δH (500 MHz; CDCl3) 9.15 (1H, s, CH = N), 8.39 (1H, s, CH-Ar), 8.15 (1H, s, CH-Ar), 8.12 (1H, d, J= 8.5, CH-Ar), 7.92 (2H, d, J= 8.2, 2 × CH-Ar), 7.88 (1H, d, J= 7.9, CH-Ar), 7.79 (1H, dd, J= 8.5, 1.8, CH-Ar), 7.61–7.53 (3H, m, 3 × CH-Ar), 2.68 (3H, s, CH3); δC (125 MHz; CDCl3) 166.60 (C = O), 157.65 (HC = N), 154.66 (Cq=N), 145.31, 137.46, 135.46, 132.90, 132.56, 130.26, 129.69, 128.98, 128.80, 128.28, 128.01, 126.98, 122.98, 122.81, 119.86 (10 × CH-Ar & 6 × Cq-Ar), 22.98 (CH3); MS (EI) m/z (%) [M+, 81Br] 393.05 (2.94), [M+, 79Br] 391.00 (2.85), 239.95 (100.00), 237.95 (98.69), 197.90 (6.54), 195.95 (4.69), 155.95 (5.37), 153.05 (22.90), 127.10 (21.27), 100.05 (3.19), 75.00 (18.97), 63.00 (3.47), 57.05 (1.26). Anal. calcd. for C20H14BrN3O (391.03): C, 61.24; H, 3.60; N, 10.71. Found: C, 61.08; H, 3.39; N, 10.65.
(E)-2-Methyl-3-[(4-pyridin-2-yl-benzylidene)-amino]-3H-quinazolin-4-one (8c)
Beige powder (benzene), yield (85%), mp 188–190 °C; νmax (KBr)/cm−1 3042 (CH-Ar), 2940 (CH-aliphatic), 1675 (C = O), 1600 & 1468 (C = C), 1436, 1378, 1327, 1230, 1181, 1154, 1108, 987, 939, 875, 847, 772, 718, 688, 663, 626, 589, 479; δH (500 MHz; CDCl3) 9.12 (1H, s, CH = N), 8.74 (1H, d, J= 4.7, CH-Ar), 8.30 (1H, d, J= 7.9, CH-Ar), 8.15 (2H, d, J= 8.2, 2 × CH-Ar), 8.00 (2H, d, J= 8.2, 2 × CH-Ar), 7.80–7.73 (3H, m, 3 × CH-Ar), 7.68 (1H, d, J= 8.1, CH-Ar), 7.48 (1H, t, J= 7.5, CH-Ar), 7.36–7.28 (1H, m, CH-Ar), 2.69 (3H, s, CH3); δC (125 MHz; CDCl3) 165.64 (C = O), 158.80 (HC = N), 156.12 (HC = N), 154.18 (Cq=N), 149.93, 146.46, 143.05, 136.92, 134.34, 133.19, 129.24, 127.35, 127.27, 126.93 126.43, 122.87, 121.56, 120.88 (11 × CH-Ar & 5 × Cq-Ar), 22.94 (CH3); MS (EI) m/z (%) [M+ + 1] 341.10 (0.69), [M+] 340.10 (1.39), 181.05 (4.88), 161.05 (10.51), 160.10 (100.00), 145.10 (2.83), 132.10 (3.91), 118.10 (12.08), 90.00 (5.53), 76.00 (16.01), 50.00 (3.71). Anal. calcd. for C21H16N4O (340.13): C, 74.10; H, 4.74; N, 16.46. Found: C, 73.92; H, 4.63; N, 16.28.
(E)-6-Bromo-2-methyl-3-[(4-pyridin-2-yl-benzylidene)-amino]-3H-quinazolin-4-one (8d)
Beige powder (benzene), yield (77%), mp 209–210 °C; νmax (KBr)/cm−1 3149 & 3060 (CH-Ar), 2952 (CH-aliphatic), 1670 (C = O), 1604 & 1465 (C = C), 1375, 1319, 1278, 1240, 1208, 1158, 1124, 1961, 1035, 987, 876, 833, 775, 727, 674 (C-Br), 645, 564; δH (850 MHz; CDCl3) 9.10 (1H, s, CH = N), 8.77 (1H, d, J= 4.3, CH-Ar), 8.41 (1H, d, J= 2.6, CH-Ar), 8.17 (2H, d, J= 7.7, 2 × CH-Ar), 8.01 (2H, d, J= 7.7, 2 × CH-Ar), 7.85–7.79 (3H, m, 3 × CH-Ar), 7.55 (1H, d, J= 8.5, CH-Ar), 7.36–7.32 (1H, m, CH-Ar), 2.68 (3H, s, CH3); δC (213 MHz; CDCl3) 165.98 (C = O), 157.67 (HC = N), 155.79 (HC = N), 154.69 (Cq=N), 149.51, 145.26, 137.56, 133.23, 129.74, 129.40, 128.79, 127.54, 123.08, 122.95, 121.23, 119.94 (10 × CH-Ar & 6 × Cq-Ar), 22.95 (CH3); MS (EI) m/z (%) [M+ + 1] 419.05 (1.37), [M+] 418.00 (2.03), 240.00 (11.07), 239.95 (100.00%), 237.95 (98.10), 199.90 (2.69), 197.90 (6.83), 181.05 (6.55), 179.05 (4.60), 154.05 (13.02), 152.05 (1.88), 139.10 (3.04), 127.10 (6.15), 116.10 (3.42), 90.05 (4.15), 78.05 (3.66), 75.00 (17.16). Anal. calcd. for C21H1579BrN4O (418.04): C, 60.16; H, 3.61; N, 13.36. Found: C, 60.03; H, 3.43; N, 13.11.
(E)-3-[(3-Methoxy-2-nitro-benzylidene)-amino]-2-methyl-3H-quinazolin-4-one (8e)
Beige powder (ethanol), yield (55%), mp 178–180 °C; νmax (KBr)/cm−1 3017 (CH-Ar), 2975 & 2935 (CH-aliphatic), 1680 (C = O), 1615 & 1571 (C = C), 1537, 1464, 1428, 1376, 1283, 1227, 1156, 1107, 1055, 1029, 964, 933, 875, 850, 772, 743, 691, 651, 589, 471, 419; δH (850 MHz; CDCl3) 9.41 (1H, s, CH = N), 8.26 (1H, d, J= 7.7, CH-Ar), 7.75 (1H, t, J= 7.7, CH-Ar), 7.67 (1H, d, J= 7.7, CH-Ar), 7.56 (1H, t, J= 7.7, CH-Ar), 7.49–7.46 (2H, m, 2 × CH-Ar), 7.23 (1H, d, J= 8.5, CH-Ar), 3.96 (3H, s, OCH3), 2.6 (3H, s, CH3); δC (213 MHz; CDCl3) 159.22 (C = O), 158.52 (HC = N), 154.87 (Cq=N), 151.44, 139.74, 134.76, 131.30, 127.39, 126.77, 126.14, 122.03, 121.38, 115.92 (7 × CH-Ar & 5 × Cq-Ar), 56.78 (OCH3), 22.65 (CH3); MS (EI) m/z (%) [M+] 338.10 (4.91), 321.10 (8.62), 290.10 (8.60), 261.10 (2.25), 186.05 (2.04), 160.10 (100.00), 143.10 (3.47), 132.10 (4.81), 117.10 (18.04), 104.05 (3.96), 76.00 (26.95), 63.00 (4.85), 50.00 (6.06). Anal. calcd. for C17H14N4O4 (338.10): C, 60.35; H, 4.17; N, 16.56. Found: C, 60.03; H, 3.94; N, 16.37.
(E)-6-Bromo-3-[(3-methoxy-2-nitro-benzylidene)-amino]-2-methyl-3H-quinazolin-4-one (8f)
Beige powder (ethanol), yield (66%), mp 234–235 °C; νmax (KBr)/cm−1 3070 & 3012 (CH-Ar), 2977 & 2926 (CH-aliphatic), 1677 (C = O), 1601 & 1533 (C = C), 1464, 1436, 1373, 1303, 1207, 1153, 1104, 1063, 973, 937, 897, 836, 779, 739, 670 (C-Br), 639, 589; δH (850 MHz; CDCl3) 9.35 (1H, s, CH = N), 8.38 (1H, d, J= 2.6, CH-Ar), 7.82 (1H, dd, J= 8.5, 2.6, CH-Ar), 7.58–7.56 (2H, m, 2 × CH-Ar), 7.48 (1H, d, J= 8.5, CH-Ar), 7.25 (1H, d, J= 8.5, CH-Ar), 3.97 (3H, s, OCH3), 2.63 (3H, s, CH3); δC (213 MHz; CDCl3) 159.28 (C = O), 157.93 (HC = N), 155.38 (Cq=N), 151.47, 144.56, 139.68, 137.94, 131.36, 129.85, 128.57, 125.86, 122.76, 122.14, 120.31, 116.16 (6 × CH-Ar & 6 × Cq-Ar), 56.80 (OCH3), 22.63 (CH3); MS (EI) m/z (%) [M+, 81Br] 418.00 (4.65), [M+, 79Br] 416.00 (4.58), 401.00 (6.90), 399.00 (6.63), 370.00 (5.89), 368.00 (5.13), 341.00 (1.54), 334.00 (1.46), 265.95 (2.41), 263.95 (2.42), 239.95 (97.78), 237.95 (100.00), 197.90 (8.61), 195.95 (6.33), 169.95 (3.24), 167.95 (2.54), 155.95 (7.13), 153.95 (7.22), 133.10 (1.53), 131.10 (3.71), 117.10 (7.38), 116.10 (5.63), 101.10 (4.34), 89.00 (4.73), 76.00 (9.56), 59.05 (13.18), 57.05 (4.20). Anal. calcd. for C17H1379BrN4O4 (416.01): C, 48.94; H, 3.14; N, 19.15. Found: C, 48.83; H, 2.97; N, 18.93.
(E)-4-[(2-Methyl-4-oxo-4H-quinazolin-3-ylimino)-methyl]-benzonitrile (8g)
White powder (acetone), yield (55%), mp 222–224 °C; νmax (KBr)/cm−1 3043 (CH-Ar), 2960 (CH-aliphatic), 2226 (CN), 1674 (C = O), 1599 & 1467 (C = C), 1406, 1376, 1320, 1235, 1145, 1111, 1034, 944, 868, 836, 766, 687, 660, 555; δH (500 MHz; CDCl3) 9.37 (1H, s, CH = N), 8.28 (1H, d, J= 8.0, CH5-quinazolin-4-one), 8.00 (2H, d, J= 8.2, 2 × CH-benzonitrile ring), 7.80 (2H, d, J= 8.2, 2 × CH-benzonitrile ring), 7.77 (1H, app. t, J= 7.1, CH7-quinazolin-4-one), 7.68 (1H, d, J= 8.0, CH8-quinazolin-4-one), 7.49 (1H, app. t, J= 7.6, CH6-quinazolin-4-one), 2.73 (3H, s, CH3); δC (125 MHz; CDCl3) 162.10 (C = O), 159.10 (HC = N), 154.14 (Cq=N), 146.23, 137.25, 134.71, 132.69, 128.98, 127.34, 127.07, 126.75, 121.48, 118.16, 115.36 (8 × CH-Ar, 4 × Cq-Ar & C≡N), 23.02 (CH3); (EI) m/z (%) [M+ + 2] 290.10 (3.21), [M+ + 1] 289.10 (1.58), [M+] 288.10 (5.65), 273.10 (3.00), 272.10 (6.19), 260.10 (1.60), 186.05 (3.69), 161.10 (10.65), 160.10 (100.00), 145.10 (4.68), 143.10 (3.86), 131.10 (14.47), 130.10 (17.06), 119.10 (11.52), 117.10 (19.54), 102.10 (18.65), 101.10 (5.04), 92.05 (5.14), 90.05 (11.61), 77.00 (20.32), 76.00 (37.53), 60.00 (4.25), 59.05 (19.07), 51.00 (6.85), 50.00 (14.71). Anal. calcd. for C17H12N4O (288.10): C, 70.82; H, 4.20; N, 19.43. Found: C, 70.69; H, 4.13; N, 19.27.
(E)-4-[(6-Bromo-2-methyl-4-oxo-4H-quinazolin-3-ylimino)-methyl]-benzonitrile (8h)
White powder (acetone), yield (75%), mp 228–230 °C; νmax (KBr)/cm−1 3059 (CH-Ar), 2927 (CH-aliphatic), 2230 (CN), 1680 (C = O), 1602 & 1467 (C = C), 1420, 1376, 1328, 1277, 1242, 1211, 1156, 1123, 1071, 981, 899, 876, 831, 778, 723, 675 (C-Br), 644, 555, 433; δH (500 MHz; CDCl3) 9.32 (1H, s, CH = N), 8.40 (1H, s, CH5-quinazolin-4-one ring), 8.00 (2H, d, J= 7.7, 2 × CH-benzonitrile ring), 7.84 (1H, d, J= 8.5, CH7-quinazolin-4-one ring), 7.81 (2H, d, J= 7.7, 2 × CH-benzonitrile ring), 7.55 (1H, d, J= 8.5, CH8-quinazolin-4-one ring), 2.64 (3H, s, CH3); δC (125 MHz; CDCl3) 162.65 (C = O), 157.83 (HC = N), 154.53 (Cq=N), 145.027, 137.87, 136.93, 132.67, 129.78, 129.01, 128.9, 122.81, 120.14, 118.04, 115.49 (7 × CH-Ar, 5 × Cq-Ar & C≡N), 22.98 (CH3); MS (EI) m/z (%) [M+, 81Br] 368.01 (2.60), [M+, 79Br] 366.01 (2.56), 354.30 (3.01), 313.30 (12.92), 297.30 (5.50), 239.20 (12.54), 227.10 (2.29), 213.15 (2.33), 185.10 (2.94), 171.10 (2.88), 143.15 (3.48), 129.10 (12.26), 111.15 (14.46), 97.15 (21.74), 83.05 (26.49), 71.05 (43.57), 69.05 (42.50), 59.05 (56.18), 57.05 (100.00). Anal. calcd. for C17H1179BrN4O (366.01): C55.61; H, 3.02; N, 15.26. Found: C, 55.49; H, 2.88; N, 15.19.
(E)-3-[(Biphenyl-4-ylmethylene)-amino]-6-bromo-2-methyl-3H-quinazolin-4-one (8j)
White powder (ethanol), yield (97%), mp 208–210 °C; νmax (KBr)/cm−1 3058 (CH-Ar), 2920 (CH-aliphatic), 1676 (C = O), 1604 & 1467 (C = C), 1373, 1319, 1276, 1236, 1206, 1180, 1154, 1074, 1007, 907, 875, 831, 757, 722, 689 (C-Br), 644, 555, 467; δH (500 MHz; CDCl3) 9.03 (1H, s, CH = N), 8.41 (1H, d, J= 2.3, CH-Ar), 7.96 (2H, d, J= 8.3, 2 × CH-Ar), 7.82 (1H, dd, J= 8.7, 2.3, CH-Ar), 7.73 (2H, d, J= 8.3, 2 × CH-Ar), 7.66 (2H, d, J= 7.3, 2 × CH-Ar), 7.54 (1H, d, J= 8.7, CH-Ar), 7.49 (2H, t, J= 7.3, 2 × CH-Ar), 7.41 (1H, t, J= 7.3 CH-Ar), 2.63 (3H, s, CH3); δC (125 MHz; CDCl3) 166.41 (C = O), 157.64 (HC = N), 154.61 (Cq=N), 145.45, 145.34, 139.92, 137.49, 131.44, 129.71, 129.43, 129.02, 128.81, 128.25, 127.66, 127.23, 122.97, 119.87 (12 × CH-Ar & 6 × Cq-Ar), 22.94 (CH3); MS (EI) m/z (%) [M+, 81Br] 419.05 (1.70), [M+, 79Br] 417.05 (1.67), 239.95 (100.00), 237.95 (98.77), 197.90 (6.94), 195.95 (4.46), 179.05 (24.10), 165.05 (5.04), 152.10 (13.04), 131.10 (2.31), 116.10 (3.58), 116.10 (3.58), 90.05 (3.62), 75.00 (15.91), 63.00 (3.20), 57.05 (2.43). Anal. calcd. for C22H1679BrN3O (417.05): C, 63.17; H, 3.86; N, 10.05. Found: C, 62.97; H, 3.63; N, 9.89.
Synthesis of (2-methyl-4-oxo-4H-quinazolin-3-yl)-carbamic acid isobutyl ester (10)
A mixture of 3-amino-2-methyl-3H-quinazolin-4-one 6a (0.0028 mol, 0.5 g) and isobutyl chloroformate 9 (13.6 equiv., 0.038 mol, 5.2 g, 4.94 ml) was refluxed for 10 hCitation35. The excess reagent was evaporated under reduced pressure and the remaining residue was treated with diethyl ether. The precipitated solid was filtered, washed with water, and purified by recrystallisation from benzene to obtain the title compound as a beige powder with 50% yield, mp 168–170 °C; vmax (KBr)/cm−1 3223 (NH), 3080 (CH-Ar), 2963 & 2875 (CH-aliphatic), 1755 (C = O), 1694 (C = O), 1651 (C = N), 1606 & 1508 (C = C), 1468, 1380, 1232, 1108, 1046, 988, 942, 872, 772, 719, 695, 633, 520, 462; δH (850 MHz; CDCl3) 8.19 (1H, d, J= 7.7, CH-Ar), 7.75 (1H, app. t, J= 7.7, CH-Ar), 7.64 (1H, d, J= 8.5, CH-Ar), 7.62 (1H, br. s, NH), 7.44 (1H, t, J= 7.7, CH-Ar), 4.00 (2H, apparent s, O– CH2– CH–(CH3)2), 2.60 (3H, s, CH3), 1.96–1.93 (1H, m, O-CH2-CH-(CH3)2), 0.94 (6H, d, J= 6.0, O– CH2–CH–(CH3)2); δC (125 MHz; CDCl3) 160.78 (C = O), 156.28 (C = O), 156.15 (C = N), 146.83, 134.89, 126.94, 126.85, 126.58, 120.60 (4 × CH-Ar, 2 × Cq-Ar), 72.71 (OCH2), 27.78 (CH), 21.52 (CH3), 18.63 (2 × CH3); MS (EI) m/z (%) [M+ + 1] 276.10 (21.00), [M+] 275.10 (100.00), 260.10 (1.46), 220.05 (4.41), 202.00 (11.98), 175.05 (95.02), 160.05 (9.43), 146.10 (85.56), 131.10 (4.53), 131.10 (4.53), 117.10 (27.22), 90.00 (23.15), 76.00 (30.46), 63.00 (8.24), 57.05 (86.01). Anal. calcd. for C14H17N3O3 (275.13): C, 61.08; H, 6.22; N, 15.26. Found: C, 61.00; H, 6.01; N, 15.17.
Synthesis of (E)-N,N-dimethyl-N′-(2-methyl-4-oxo-4H-quinazolin-3-yl)-formamidine (12)
A mixture of 3-amino-2-methyl-3H-quinazolin-4-one 6a (0.0028 mol, 0.5 g) and N,N-dimethyl formamide dimethyl acetal 11 (5.39 equiv. 0.0151 mol, 1.79 g, 2 ml) in ethanol (15 ml) was refluxed for 2 hCitation36. The excess solvent was removed under reduced pressure. The remaining solid was washed with n-hexane and recrystallised from ethanol to obtain the title compound as a yellow powder with 50% yield, mp 100–102 °C; νmax (KBr)/cm−1 3010 (CH-Ar), 2924 & 2807 (CH-aliphatic), 2145, 1660 (C = O), 1621 (C = N), 1609 & 1589 (C = C), 1475, 1418, 1366, 1323, 1237, 1174, 1120, 1069, 944, 874, 783, 701, 660, 626, 551, 509; δH (500 MHz; CDCl3) 8.23 (1H, dd, J= 7.7, 1.7, CH-Ar), 7.74 (1H, s, HC = N), 7.69–7.67 (1H, m, CH-Ar), 7.62 (1H, dd, J= 8.5, 2.6, CH-Ar), 7.41–7.39 (1H, m, CH-Ar), 3.08 (3H, s, N-CH3), 3.04 (3H, s, N-CH3), 2.52 (3H, s, CH3); δC (125 MHz; CDCl3) 162.22 (C = O), 160.08 (HC = N), 155.08 (Cq=N), 146.39, 133.39, 126.41, 125.61, 121.04 (4 × CH-Ar & 2 × Cq-Ar), 40.88, 34.53 (N(CH3)2), 22.56 (CH3); MS (EI) m/z (%) [M+ + 1] 231.10 (5.52), [M+] 230.10 (34.52), 215.05 (1.48), 186.00 (10.30), 161.10 (11.53), 160.10 (100.00), 145.10 (5.33), 132.10 (7.42), 118.10 (21.02), 104.10 (1.60), 90.00 (9.13), 76.00 (16.94), 63.00 (5.01), 50.00 (6.27). Anal. calcd. for C12H14N4O (230.12): C, 62.59; H, 6.13; N, 24.33. Found: C, 62.47; H, 5.98.; N, 24.18.
Synthesis of 2-(4-chloro-benzylsulfanyl)-6-methyl-3-phenyl-3H-quinazolin-4-one (15a31, 15b) and 2-(4-oxo-3-phenyl-3,4-dihydro-quinazolin-2-ylsulfanyl)-acetamide derivatives (15c–f)
A mixture of the appropriate derivative of 2-mercapto-3-phenyl-3H-quinazolin-4-one (13a',b') (0.0018 mol) and 4-chlorobenzyl chloride 14a or chloroacetamide derivatives 14b,cCitation37,Citation38 (1 equiv., 0.0018 mol) and potassium carbonate (2.5 equiv., 0.0045 mol, 0.62 g) in dry acetone (20 ml) was refluxed for 6 hCitation39. The hot reaction mixture was filtered and the filtrate was concentrated under reduced pressure. The resulting solid in each case was washed with water, air dried, and recrystallised from ethanol to obtain compounds 15a–f.
2 -(4-Chloro-benzylsulfanyl)-6-methyl-3-phenyl-3H-quinazolin-4-one (15b)
White powder, yield (96%), mp 164–165 °C; νmax (KBr)/cm−1 3058 (CH-Ar), 2915 (CH-aliphatic), 1689 (C = O), 1617 & 1575 (C = C), 1548, 1487, 1401, 1399, 1335, 1299, 1259, 1204, 1128, 1089, 1014, 963, 824 (C–Cl), 742, 692, 641, 539, 506; δH (500 MHz; CDCl3) 8.01 (1H, s, CH5-quinazolin-4-one), 7.54–7.48 (5H, m, 5 × CH-Ar), 7.29–7.18 (6H, m, 6 × CH-Ar), 4.32 (2H, s, CH2), 2.44 (3H, s, CH3); δC (125 MHz; CDCl3) 161.83 (C = O), 155.60 (C = N), 145.82, 136.17, 136.12, 135.90, 135.38, 133.28, 130.77, 130.00, 129.72, 129.26, 128.68, 126.76, 126.05, 119.72 (12 × CH-Ar & 6 × Cq-Ar), 36.22 (CH2), 21.33 (CH3); MS (EI) m/z (%) [M+, 37Cl] 394.05 (27.71), [M+ + 1, 35Cl] 393.10 (19.45), [M+, 35Cl] 392.10 (69.22), 375.05 (1.96), 361.10 (35.50), 359.10 (100.00), 324.10 (11.09), 301.00 (12.62), 283.05 (37.48), 267.05 (81.50), 249.10 (51.70), 235.05 (33.05), 201.00 (89.80), 193.05 (3.31), 180.05 (4.87), 166.05 (14.31), 150.05 (8.84), 133.10 (77.88), 104.10 (28.46), 89.00 (25.12), 77.00 (63.94), 63.00 (10.37), 51.00 (11.84). Anal. calcd. for C22H1735ClN2OS (392.08): C, 67.25; H, 4.36; N, 7.13. Found: C, 67.20; H, 4.32; N, 7.06.
N-(2,6-Dimethyl-phenyl)-2-(4-oxo-3-phenyl-3,4-dihydro-quinazolin-2-ylsulfanyl)-acetamide (15c)
White crystals, yield (55%), mp 218–220 °C; νmax (KBr)/cm−1 3331 (NH), 3065 (CH-Ar), 2971 & 2917 (CH-aliphatic), 2366, 1664 (C = O), 1601 (C = O), 1552 & 1493 (C = C), 1467, 1362, 1335, 1299, 1264, 1205, 1112, 1030, 962, 881, 768, 695, 641, 595, 568, 523, 468; δH (850 MHz; CDCl3) 8.66 (1H, br. s, NH), 8.27 (1H, d, J= 7.7, CH-Ar), 7.76 (1H, t, J= 7.7, CH-Ar), 7.62 (1H, d, J= 7.7, CH-Ar), 7.59–7.58 (2H, m, 2 × CH-Ar), 7.47 (1H, t, J= 7.7, CH-Ar), 7.33–7.32 (2H, m, 2 × CH-Ar), 7.11–7.07 (2H, m, 2 × CH-Ar), 7.04 (2H, d, J= 6.8, 2 × CH-Ar), 3.99 (2H, s, CH2), 2.18 (6H, s, 2 × CH3); δC (312 MHz; CDCl3) 166.94 (C = O), 161.33 (C = O), 157.78 (C = N), 146.99, 135.26, 135.23, 135.19, 133.55, 130.55, 130.05, 129.02, 128.27, 127.66, 127.38, 126.75, 125.73, 119.91 (12 × CH-Ar, 6 × Cq-Ar), 35.49 (CH2), 18.33 (2 × CH3); MS (EI) m/z (%) [M+ + 1] 416.15 (1.05), [M+] 415.15 (2.86), 400.10 (9.45), 296.05 (20.13), 295.05 (100.00), 268.05 (28.58), 253.05 (10.77), 235.10 (30.01), 221.05 (5.97), 177.05 (7.25), 162.00 (2.47), 148.05 (3.93), 132.10 (20.59), 119.05 (11.21), 105.10 (2.87), 91.05 (12.43). Anal. calcd. for C24H21N3O2S (415.14): C, 69.37; H, 5.09; N, 10.11. Found: C, 69.18; H, 4.92; N, 10.00.
N-(2,6-Dimethyl-phenyl)-2-(6-methyl-4-oxo-3-phenyl-3,4-dihydro-quinazolin-2-ylsulfanyl) -acetamide (15d)
White powder, yield (57%), mp 229–230 °C; νmax (KBr)/cm−1 3269 (NH), 3024 (CH-Ar), 2961 & 2919 (CH-aliphatic), 2372, 2341, 1688 (C = O), 1651 (C = O), 1574 & 1547 (C = C), 1486, 1408, 1336, 1296, 1260, 1199, 1103, 1032, 963, 912, 836, 774, 748, 692, 648, 604, 543, 504; δH (500 MHz; CDCl3) 8.70 (1H, br. s, NH), 8.05 (1H, s, CH-Ar), 7.57–7.49 (5H, m, 5 × CH-Ar), 7.32–7.30 (2H, m, 2 × CH-Ar), 7.07–7.02 (3H, m, 3 × CH-Ar), 3.96 (2H, s, CH2), 2.46 (3H, s, CH3), 2.16 (6H, s, 2 × CH3); δC (125 MHz; CDCl3) 166.99 (C = O), 161.39 (C = O), 156.66 (C = N), 145.07, 136.98, 136.57, 135.42, 135.18, 133.64, 130.46, 130.01, 129.08, 128.25, 127.32, 127.05, 125.59, 119.64 (11 × CH-Ar, 7 × Cq-Ar), 35.49 (CH2), 21.30 (CH3), 18.35 (2 × CH3); MS (EI) m/z (%) [M+ + 1] 430.10 (1.24), [M+] 429.15 (3.40), 414.10 (9.75), 309.05 (100.00), 282.05 (35.11), 267.05 (10.35), 249.10 (38.79), 235.05 (5.16), 205.05 (1.86), 177.05 (5.60), 160.05 (2.87), 146.10 (16.74), 133.10 (10.36), 121.10 (4.97), 104.05 (10.15), 91.05 (11.20), 77.00 (21.32), 59.00 (3.71). Anal. calcd. for C25H23N3O2S (429.15): C, 69.91; H, 5.40; N, 9.78. Found: C, 69.76; H, 5.32; N, 9.64.
N-(2,3-Dimethyl-phenyl)-2-(6-methyl-4-oxo-3-phenyl-3,4-dihydro-quinazolin-2-ylsulfanyl) -acetamide (15f)
White powder, yield (76%), mp 218–220 °C; νmax (KBr/cm−1 3259 (NH), 3000 (CH-Ar), 2925 (CH-aliphatic), 2371, 2341, 1692 (C = O), 1648 (C = O), 1574 & 1545 (C = C), 1487, 1300, 1263, 1204, 1147, 963, 834, 787, 750, 694, 651; δH (500 MHz; CDCl3) 8.90 (1H, s, NH), 8.06 (1H, s, CH-Ar), 7.57–7.53 (5H, m, 5 × CH-Ar), 7.49 (1H, d, J= 7.4, CH-Ar), 7.32–7.25 (2H, m, 2 × CH-Ar), 7.08 (1H, apparent t, J= 7.3, CH-Ar), 6.97 (1H, d, J= 6.9, CH-Ar), 3.97 (2H, s, CH2), 2.48 (3H, s, CH3), 2.23 (3H, s, CH3), 2.01 (3H, s, CH3); δC (125 MHz; CDCl3) 167.11 (C = O), 161.38 (C = O), 156.74 (C = N), 145.11, 137.41, 136.94, 136.50, 135.39, 135.31, 130.46, 129.96, 129.10, 128.95, 127.31, 127.05, 125.93, 125.67, 121.93, 119.66 (11 × CH-Ar & 7 × Cq-Ar), 36.14 (CH2), 21.30 (CH3), 20.55 (CH3), 13.82 (CH3); MS (EI) m/z (%) [M+] 429.15 (0.15), 414.10 (0.65), 309.05 (100.00), 282.05 (5.52), 269.10 (6.32), 249.10 (13.83), 235.05 (3.23), 189.05 (1.52), 161.10 (1.76), 146.10 (10.05), 133.10 (6.23), 113.10 (7.77), 101.10 (4.81), 77.00 (13.43), 59.00 (21.59). Anal. calcd. for C25H23N3O2S (429.15): C, 69.91; H, 5.40; N, 9.78. Found: C, 69.85; H, 5.35; N, 9.75.
Biological evaluation
Cell culture
Human CRC cell lines, HT-29 and SW620 cells were grown in RPMI (Invitrogen) containing 10% heat-inactivated foetal bovine serum, 100 µg/ml streptomycin, 100 units/ml penicillin and 2 mmol/l L-glutamine.
Cell viability assay
Cell viability was determined using the 3-(4, 5-dimethylthiazolyl-2)–2, 5-diphenyltetrazolium bromide) MTT assay according to MosmannCitation40. 5-Fluorouracil (5-FU) was used as positive control. Briefly, after culturing cells in 96 well plates for 24 h, they were treated with different compounds (30 μg/ml) for 24 h. Freshly prepared MTT (5 mM) solutions were added to the cells and were further incubated for 2 h at 37 °C in 5% CO2. One hundred microliters of dimethyl sulphoxide (DMSO) was added in each well to dissolve the formazan crystal, formed in the reaction during incubation by careful pipetting. The absorbance of the product was measured at 540 nm using a microplate reader. The experiments were performed in triplicate for each condition. The graph illustrates the mean and standard deviation (SD) values of three independent experiments.
Cytotoxicity assay using the xCELLigence system
The optimal seeding concentration for proliferation of HT-29 was determined. HT-29 cells (5000 cell in 150 µl medium/well) and SW620 cells (12,000 cell in 150 µl medium/well) were seeded in 16 well plates (E-plate 16-ACEA Biosciences Inc., San Diego, CA) as described in the xCELLigence Real Time Cell Analyzer (RTCA) DP instrument manual provided by the manufacturer. After 24 h, 20 and 40 µg/ml of compound 15d were added and the experiments were allowed to run for another 3 d. Baseline cell indexes were calculated for at least two measurements from three replicate experiments.
Western blotting
Whole cell lysates were prepared using radioimmunoprecipitation assay (RIPA) lysis buffer as described by Al-Khayal and co-workersCitation41. Total protein concentration was determined using Bradford protein reagent (Bio-Rad, Hercules, CA). Soluble proteins were loaded on precast TGX gels and were analysed using immunoblotting with anti-Bcl2, anti-BclxL (dilution 1:1000; Santa Cruz Biotechnology, Dallas, TX), and anti-β-actin (1:10,000 Sigma, St. Louis, MO). Reactivity was detected using horseradish peroxidase-conjugated secondary antibodies and chemiluminescence by Clarity Western ECL substrate (Bio-Rad). Membrane was developed using C-Digit Blot Scanner (LI-COR, Hamburg, Germany).
In vitro evaluation of antiphospholipases activities
The inhibitory activities of the synthesised 3H-quinazolin-4-one derivatives against a group of phospholipases were investigated according to the method of De Aranjo and RadvanyCitation42 using five sPLA2s: human group IIA (hG- IIA), human group V (hG-V), human group X (hG-X), Apis mellifera bee venom (AmPLA2) and Naja mossambica mossambica sPLA2 (NmPLA2). Ten microliters of each sPLA2 solution (20 μg/ml) was mixed with 10 μl of each compound at different concentrations (0–50 μg/ml) and the mixture was incubated for 20 min at room temperature. Then, 1 ml of the PLA2 substrate (3.5 mM lecithin suspended in 100 mM NaCl, 10 mM CaCl2, 3 mM NaTDC, and 0.055 mM red phenol, pH 7.6) was added. The hydrolysis kinetics was followed spectrophotometrically for 5 min at 558 nm. The results were reported as the inhibition percentage that was calculated by comparison with a control experiment (absence of compound) and the IC50 values were determined from the curve. Oleanolic acid (Sigma) was used as the positive control.
In vitro evaluation of antiproteases activities
The inhibitory effects of 21 3H-quinazolin-4-one derivatives on several available therapeutically important proteases such as cathepsin-B, collagenase, elastase, thrombin, and trypsin were investigated. The inhibition of the enzymes in the presence of test compounds was evaluated by adding different concentrations of each compound (0–75 μg/ml) to the respective reaction mixture, preincubating for 15 min and then measuring the remaining enzyme activity as previously describedCitation43. The protease inhibitory activity was expressed as inhibition percentage, which was determined by comparison with a control experiment. The results were expressed as IC50 values that were determined from the standard curve. A protease inhibitor cocktail (Sigma) was used as the positive control.
In vitro inhibition of α-amylase activity
The α-amylase inhibitory activity of the tested compounds was investigated according to the reported method by SubranianCitation44. Briefly, 10 µL of α-amylase (3,3 U) (Sigma) was mixed with 10 µl of different concentrations (20–200 µg/ml) of each compound, the appropriate solvent, or quercetin (positive control), and incubated at 37 °C for 5 min. After adding 180 µl of Labtest (amylase substrate), the samples were incubated for 8 min and the absorbance of first reaction was measured at 620 nm. Then, 100 µl of the reaction mixture was incubated for more five additional minutes at 37 °C and the absorbance of the second reaction was measured. Labtest was diluted in distilled water (1:1) before being added to the microplate. The concentration of quercetin used was same as those of the test compounds. The α-amylase inhibition percentage was calculated as follows: % inhibition = 100 _ (X2 sample _ X1 sample/X2 control _ X1 control) × 100 where X1 is the absorbance of the initial reading and X2 is the absorbance of the final reading. The results were expressed as IC50 determined from the curve.
In vitro inhibition of α-glucosidase activity
The α-glucosidase inhibitory activity of the tested compounds was evaluated according to the method described by Andrade-Cetto and collaboratorsCitation45 based on the release of 4-nitrophenol α-D-glucopyranoside (4 NPGP). Briefly, 20 µl of different concentrations (ranging from 0 to 50 µg/ml) of each compound, the appropriate solvent, or quercetin (positive control) was mixed with 180 µl of the α-glucosidase enzyme from Saccharomyces cerevisiae (Sigma) and the obtained mixture was incubated at 37 °C for 2 min, followed by additional incubation for more than 15 min at 37 °C after the addition of 150 µl of the colour reagent NPGP. The colorimetric assay included 2 U of α-glucosidase, 5 mM of 4-NPGP, and 10 mM potassium phosphate buffer, pH 6.9. The concentration of quercetin used was identical to that of the tested compounds. The reading assay was performed using a microplate reader at 405 nm. The α-glucosidase inhibition percentage was calculated as follows: % inhibition = 100 _ (X2 sample _ X1 sample/X2 control _ X1 control) × 100 where X1 is the absorbance of the initial reading and X2 is the absorbance of the final reading, control is the absorbance of the assay with the appropriate solvent. The results were expressed as IC50 determined from the curve.
In vitro inhibition of xanthine oxidase
The xanthine oxidase inhibitory activities of the examined chemical compounds were determined by following the formation of uric acid from xanthine according to the reported method by Bondet and co-workersCitation46. Forty microliters xanthine oxidase (667 mM) and 15 µl of each compound (0–150 µg/ml), allopurinol (positive control), or appropriate solvent (negative control) were added to each microplate well and preincubated for 5 min at 37 °C. Then, 95 µl of reagent 1 (mixture of hydroxylamine (0.2 mM), EDTA (0.1 mM), and xanthine oxidase (667 mM) in 50 mM phosphate buffer solution (pH 7.5) were added to the reaction mixture and incubated at the same temperature for 30 min. Subsequently, the absorbance was measured at 295 nm using a microplate reader. Finally, after addition of 150 µl of uric acid reagent, the absorbance was measured again. The concentration of allopurinol used was same as that of the tested compounds. Xanthine oxidase inhibition was calculated as follows: % inhibition = 100 _ (X2 sample _ X1 sample/X2 control _ X1 control) × 100 where X1 is the absorbance of the initial reading and X2 is the absorbance of the final reading. The results were expressed as IC50 determined from the curve.
Results and discussion
Chemistry
The main targets of this investigation were 3H-quinazolin-4-one derivatives possessing general structure 1.
Two synthetic approaches were used for the synthesis of these scaffolds.
The first synthetic approach featured the preparation and chemical transformations of the key intermediates benzo[d][1,3]oxazin-4-one derivatives 3a–d to various 3H-quinazolin-4-one derivatives as depicted in Scheme 1.
Scheme 1. (i) Heating in domestic microwave at 540 watt for 8 min, cooling, and washing with petroleum ether; (ii) Fusion in oil bath at 200 °C, for 2 h, cooling to 100 °C, treatment with 37% HCl (Conc) till dissolution of the reaction mixture; cooling to room temperature and alkalisation with dilute NaOH (25%); (iii) ethanol, reflux for 8 h; (iv) ethanol and acetic acid catalytic, reflux for 10 h; (v) reflux for 10 h; (vi) ethanol, reflux for 2 h.
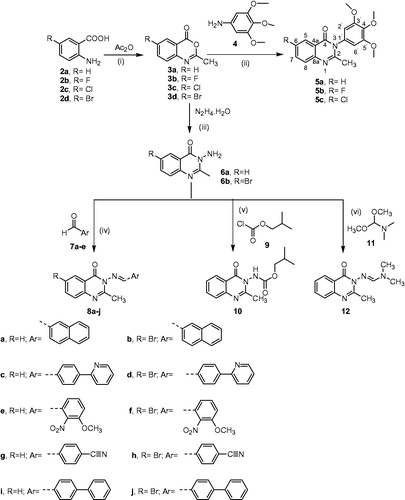
The conventional methods available for the synthesis of compounds 3a–d via cyclocondensation of anthranilic acid derivatives 2a–d with acetic anhydrideCitation26–28 were time consuming, therefore, these compounds were synthesised using the more efficient microwave-assisted method. Fusion of oxazinones 3a–c with 3,4,5-trimetrhoxyanline 4Citation32,Citation33 yielded 2-methyl-3–(3,4,5-trimethoxy-phenyl)-3H-quinazolin-4-one derivatives 5a–c. The assigned molecular structures of these new quinazolin-4-one derivatives were in full agreement with their spectroscopic and elemental analyses. Thus the IR spectrum (KBr) of compound 5c, for example, displayed absorption bands at vmax 3075, 2934; 2839, 1680, 1598; 1504 and 834 cm−1 for aromatic-CH, aliphatic-CH, the carbonyl group, C = C and C–Cl, respectively. The 1H NMR spectrum of compound 5c revealed the presence of five aromatic protons, which were displayed as; a one proton doublet signal at δ 8.18 ppm with coupling constant J of 2.4 Hz corresponding to CH5-quinazolin-4-one, a one-proton double-doublet signal at δ 7.69 ppm with coupling constant J of 8.7, 2.4 Hz due to CH7-quinazolin-4-one, a one-proton doublet signal at δ 7.60 ppm with coupling constant J of 8.7 Hz corresponding to CH8-quinazolin-4-one, and a two-protons singlet peak at δ 6.51 ppm, which was attributed to the two methine protons of 3,4,5-trimethoxyphenyl moiety (H2' and H6'). The nine protons of the three methoxy groups were displayed as a three-protons singlet at δ 3.92, and a six-protons singlet at δ 3.88 ppm. Finally, the three protons of the methyl group were characterised by a three-protons singlet signal at δ 2.32 ppm. Moreover, the 13C NMR spectrum also confirmed the assigned structure based on the emergence of fifteen spectral lines at δC 161.28 (CO), 154.75 (C = N), 154.25, 145.91, 138.56, 135.02, 132.95, 132.36, 128.52, 126.23, 121.73, 105.09 (5 × CH-Ar & 7 × Cq-Ar), 60.93 (OCH3), 56.28 (2 × OCH3) and 24.02 (CH3-quinazolin-4-one). The mass spectrum (EI) of compound 5c indicated the presence of the expected molecular ion peak [M+] at m/z 360.10 (100%) for C18H1735ClN2O4.
Refluxing of oxazinones 3a,d with excess hydrazine hydrate in ethanol yielded 3-aminoquinazolinons 6a,bCitation29,Citation30, which were converted to certain novel Schiff bases 8a–j via condensation with a series of aryl aldehydes 7a–e in absolute ethanol containing catalytic amount of glacial acetic acidCitation34. The structures of the new Schiff’s bases were verified by their IR, 1H NMR, 13C NMR, mass spectroscopic (MS) data, and CHN analyses. The IR spectra of compounds 8a–j indicated disappearance of the stretching bands of the amino groups in 3-aminoquinazolin-4-one derivatives 6a,b. The 1H NMR spectra were characterised by the disappearance of the two-protons singlet signals corresponding to the amino protons of starting materials, which resonated at δ 5.79 and 4.89 ppm, accompanied by emergence of new one-proton singlet signals attributed to azomethine protons (CH = N) at δ 9.41_8.70 ppm range. In addition, the 13C NMR spectra displayed the expected spectral lines for each compound with the signals corresponding to (CH = N) groups resonating at δC 159.10–156.46 ppm.
Furthermore, the amino group of 3-amino-2-methyl-3H-quinazolin-4-one 6a was transformed to the corresponding carbamate group via direct nucleophilic substitution reaction for isobutyl chloroformate 935 to yield the new carbamate derivative 10. The structure of the latter product was established on the basis of its IR, 1H NMR, 13C NMR, MS and HCN elemental analyses. The IR spectrum displayed the characteristic stretching absorption bands at vmax 3223, 1755, and 1694 cm−1 indicating the presence of NH and two C = O groups. The 1H NMR spectrum of compound 10 was characterised by the presence of a one-proton broad singlet peak at δ 7.62 ppm due to NH group, in addition to the presence of a two-protons apparent singlet signal, a one-proton multiplet, and a six-protons doublet signal with coupling constant value J of 6.0 Hz at δ 4.00, 1.96–1.93 and 0.94 ppm, respectively corresponding to (O-CH2-CH-(CH3)2) isobutoxy group. Moreover, the 13C NMR spectrum of carbamate 10 revealed the emergence of a new spectral line at δC 156.28 ppm attributable to the C = O of the carbamate functional group. The methylene, methine and two methyl groups of isobutoxy moiety demonstrated three spectral lines at δC 72.71, 27.78, and 18.72 ppm, respectively. Finally, the mass spectrum (EI) of compound 10 showed the anticipated molecular ion peaks [M+ + 1] at m/z 276.10 (21.00%) for C14H17N3O3 and [M+] 275.10 (100.00%).
Next, 3-amino-2-methyl-3H-quinazolin-4-one 6a was transformed to the corresponding N,N-dimethylformamidine derivative 12 by refluxing with excess N,N-dimethyl formamide dimethyl acetal (DMF-DMA) 11 in absolute ethanolCitation36. The structure of this new compound was deduced from its IR, 1HNMR, 13CNMR, MS data, and elemental analyses. The IR spectrum revealed the presence of absorption bands at vmax 3010, 2924; 2807, 1660, 1621, 1589; 1475 cm−1 which were attributed to CH-aromatic, CH-aliphatic, C = O, C = N, and C = C, respectively. In addition, the 1H NMR spectrum of formamidine 12 was characterised by the presence of a new one-proton singlet signal at δ 7.74 ppm attributed to azomethine proton (HC = N) and two singlet signals, each integrating to three protons resonating at δ 3.08 and 3.04 ppm, corresponding to the two methyl groups in dimethylamino N(CH3)2 moiety. Furthermore, the 13C NMR spectrum of compound 12 demonstrated three new peaks at δC 160.08, 40.88, and 34.53 ppm which were attributed to HC=N-N(CH3)2 and, two carbons of the methyl groups in N(CH3)2 moiety, respectively. The mass spectrum (EI) of compound 12 showed the anticipated molecular ion peak [M+] at m/z 230.00 (34.52%) for C12H14N4O.
The second synthetic approach for preparation of 3H-quinazolin-4-one derivatives involved the synthesis of compounds 15a–f via nucleophilic attack by 2-mercapto-3-phenyl-3H-quinazolin-4-one derivatives 13a',b' on 4-chlorobenzyl chloride 14a or chloroacetamide derivatives 14b,cCitation37,Citation38 to yield compounds 15a-f by adapting modified procedure to what was reported by Salman et al.Citation39 as illustrated in Scheme 2.
Structures of the new quinazolinone derivatives 15b and 15d,f were verified based on their respective IR, 1H NMR, 13C NMR, MS data, and elemental analyses. The IR spectra (KBr) of these products were characterised by the disappearance of the absorption bands at vmax 3245 and, 3169 cm−1 for the NH group (NH, SH exchangeable) and at vmax 1195 cm−1 for the thiocarbonyl group (C = S) of starting substrates 13a,b. Furthermore, their 1H NMR spectra indicated the disappearance of the signals at δ 13.05 and 12.99 ppm due to the thiol protons of the starting materials. In addition, the spectrum of compound 15b showed characteristic two-protons singlet signal at δ 4.32 corresponding to the benzylic methylene group. Analogously, the spectra of compounds 15d,f were characterised by the emergence of new one-proton broad singlet signals at δ 8.70 and 8.90 ppm corresponding to NH groups, and two-protons singlet signals at δ 3.96 and, 3.97 belong corresponding to methylene groups. In addition, the two methyl groups of the acetamide moieties were represented by a six-protons singlet signal at δ 2.16 ppm for compound 15d, and two singlet signals each integrating to three-protons at δ 2.23 and, 2.01 ppm for compound 15f.
Furthermore, the 13C NMR spectra of compounds 15b,d,f revealed the absence of spectral lines at δC 176.05 and 176.00 ppm corresponding to the thiocarbonyl group in the starting materials 13a,b and the presence of new peaks at δC 36.22, 35.49, and 36.14 ppm attributed to the new methylene groups. The carbonyl and methyl groups of the acetamide moieties in compounds 15d,f were observed at δC 166.99, 167.11, 18.35, 20.55, and 13.82, respectively. In addition, the mass spectra (EI) of compounds 15b,d,f displayed the anticipated molecular ion peaks.
Biological evaluation
Effects of the synthesised 3H-quinazolin-4-one derivatives on cell viability of human colorectal cancer cell lines HT-29 and SW620
As CRC starts with initial benign stages advanced to late stage leading to metastasis. Therefore, two human CRC cell lines which belong to two different stages namely, HT-29 which is derived from adenocarcinoma CRC and SW620 which is derived from metastatic site were selected for in vitro evaluation of the cytotoxicity (30 μg/ml) of twenty-one compounds incorporating the 3H-quinazolin-4-one scaffold.
Cell viability was monitored using the MTT assay and the results are presented in Supplementary Figure 1. Compared to the negative control, and the positive control (5-FU), the synthesised 3H-quinazolin-4-one derivatives displayed varied degrees of cytotoxicity against the examined colorectal cell lines. Eight out of all the examined compounds showed significant decrease in cell viability. In one hand, compound 15c inhibited only HT-29 cell line (47.5% viability) whereas compound 5b inhibited only SW620 cell line (46.6% viability). On the other hand, six compounds namely, 8a, 8f, 8j, 15a, 15b, and 15d, were potently capable of inhibiting cell viability of both human CRC cell lines. Compound 15d was the most active in this set reducing the cell viabilities of HT-29 and SW620 cells lines to 38% and, 36.7%, compared to 5-FU which demonstrated cell viabilities of 65.9 and 42.7%, respectively. Hence, compound 15d was selected for further mechanistic studies. The results indicated that compound 15d inhibited cell viability in a dose-dependent manner. The IC50 for 15d was ∼20 µg/ml for HT-29 and SW620 cells (Supplementary Figures 2 and 3). These results were again confirmed using the xCELLigence RTCA system, which measures the cytotoxic effect of inhibitors in real time at different time points and 15d was found to inhibit the cell proliferation in human CRC HT-29 and SW620 cell lines in dose- and time-dependent manner (Supplementary Figures 4 and 5).
Evaluation of anti-apoptotic protein expression by compound 15d
SW620 cells were treated with various concentrations of 15d for 24 h. Subsequently, cell lysates were immunoblotted with the indicated antibodies in order to investigate the mechanism via which this compound inhibited cell viability of human CRC cells. Results shown in Supplementary Figure 6 indicated that 15d inhibited the anti-apoptotic proteins, Bcl2 and BclxL. These findings demonstrate that compound 15d inhibited the cell viability of human CRC cells by down regulating the expression of Bcl2 and BclxL and triggering the apoptotic pathway.
In vitro evaluation of antiphospholipase A2 (PLA2) and antiproteases activities of the synthesised 3H-quinazolin-4-one derivatives
As inflammation is associated with several types of cancers via influencing growth, apoptosis, and proliferation of cancer and stromal cells, the synthesised 3H-quinazolin-4-one derivatives were further screened to evaluate their antiphospholipases activities against proinflammatory enzymes implicated in cancer namely, hGIIA, hGV, and X10. In addition, the inhibitory activity of these compounds towards the allergen and inflammatory PLA2 from honey bee venom (Apis mellifera, AmPLA2)Citation47 and proinflammatory, heamolytic, myotoxic and neurotoxic Cobra (Naja mosambique mosambique) venom PLA2 (NmPLA2)Citation48 were evaluated by determining their IC50 values. Results (Supplementary Table 1) revealed that six compounds, namely, 5a, 8c, 8d, 8j, 15a, and 15d displayed the most promising potency in inhibiting the catalytic activity of the studied hG-IIA, with IC50 values ranging from 5.75 ± 1.06 to 8.60 ± 0.56 µg/ml compared to that recorded with oleanolic acid which was used as a standard drug (5.25 ± 0.35 µg/ml). Among these compounds, 8d exhibited the lowest IC50 value (5.75 ± 1.06 µg/ml).
The anti-PLA2 hGV data showed that three compounds; 5a, 8d, and 8j inhibited the enzyme with IC50 values ranging from 6.25 ± 0.78 to 9.60 ± 0.85 µg/ml compared to the value recorded with the standard drug (oleanolic acid, IC50; 7.50 ± 0.71 µg/ml). Amongst them, compound 8j (IC50; 6.25 ± 0.78 µg/ml), was being more potent than the reference drug.
The analysis of the inhibitory potency of the examined compounds against PLA2 hG-X showed that compounds; 5a, 5 b, 5c, 8d, 8j, 10, 15a, and 15e inhibited the enzymatic activity with IC50 values ranging from 6.60 ± 0.85 to 9.50 ± 0.71 µg/ml. It worth to note that compounds 8d, 15a, and 15e exhibited lowered IC50 values than the reference drug (IC50; 7.55 ± 0.64 µg/ml).
Amongst the tested 3H-quinazolin-4-one derivatives which exhibited inhibitory activity against NmPLA2 were compounds; 5a, 5b, 5c, 8c, 8d, 8e, 8f, 15a, and 15d with IC50 values ranging from 4.15 ± 0.50 to 8.50 ± 2.12 µg/ml compared to oleanolic acid (IC50; 4.90 ± 0.42 µg/ml). Interestingly, compound 5b demonstrated the lowest IC50 which is also lower than that of the reference drug fusspot.
Finally, 13 compounds namely, 5a, 5b, 5c, 8b, 8c, 8d, 8e, 8f, 8i, 10, 15a, 15d, and 15e effectively suppressed AmPLA2 with IC50 values ranging from 4.20 ± 0.57 to 9.40 ± 0.85 µg/ml compared to the IC50 4.20 ± 0.42 µg/ml value of oleanolic acid. Based on these results, AmPLA2 appears to be the maximally affected phospholipase by the synthesised compounds with compound 8f (IC50; 4.20 ± 0.57 µg/ml) demonstrating inhibitory activity equivalent to that of the reference drug.
Similarly, the inhibitory potency of the selected 3H-quinazolin-4-one derivatives against five proteases namely, cathepsin-B, collagenase, thrombin, elastase, and trypsin was quantified by measuring and comparing the IC50 values produced by these compounds relative to the value produced by the protease inhibitor cocktail. The results of anti-proteases shown in Supplementary Table 2 indicated that compounds 8j and 15e were being the most active inhibitors against protease cathepsin-B with IC50 values of 1.40 ± 0.06 and 1.60 ± 0.07 µg/ml respectively compared to cocktail (IC50; 0.175 ± 0.04 µg/ml).
Furthermore, compounds 8b, 8d, and 15e were found to be the most active in suppressing the collagenase activity with IC50 values ranging from 3.00 ± 0.01 to 4.50 ± 0.71 µg/ml compared to cocktail (IC50; 0.15 ± 0.07 µg/ml) with compound 8d exhibiting the lowest IC50 value.
The highest anti-thrombin activity was demonstrated by compounds 5b, 5c, 8e, 10, and 15b, with IC50 values ranging from 0.90 ± 0.14 to 2.50±0.71 µg/ml, with 5b demonstrating the lowest IC50 compared to cocktail (IC50; 0.25 ± 0.07 µg/ml).
The highest anti-elastase activity was demonstrated by compounds 5b, 5c, 8e, 10, and 15b, with IC50 values ranging from 0.65 ± 0.02 µg/ml (compound 15b) to 1.75 ± 0.05 µg/ml (compound 5c) compared to that of cocktail (IC50; 0.125 ± 0.04 µg/ml).
Finally, the lowest IC50 values ranging from 0.50 ± 0.00 to 2.25 ± 0.04 µg/ml against trypsin were demonstrated by compounds 5b, 5c, 8e, 10, and 15 b, with compound 15b displaying the lowest IC50 compared to that of the reference drug (IC50; 0.215 ± 0.04 µg/ml).
In vitro evaluation of inhibitory activities of 3H-quinazolin-4-one derivatives against enzymes implicated in metabolic syndrome
Considering an interactive relationship between diabetes and cancers, the in vitro inhibitory potential of the prepared 3H-quinazolin-4-one derivative against α-amylase, α-glucosidase, and xanthine oxidase (enzymes related to metabolic syndrome) was investigated.
The IC50 values exhibited by the chemical compounds against α-amylase activity are shown in Supplementary Table 3, which indicated that compounds 8d, 8h, 8j, 15a, 15b, 15e, and 15f, were more potent in suppressing the enzyme with IC50 values ranging from 94.00 ± 2.83 µg/ml (compound 15b) to 121.00 ± 9.90 µg/ml (compound 15e) than quercetin the reference drug (IC50 123.0 ± 2.83 µg/ml).
In addition, compounds 8g (IC50 3.25 ± 0.35 µg/ml), 8h (IC50 3.05 ± 0.21 µg/ml), and 8i (IC50 3.45 ± 0.64 µg/ml) were more potent in inhibiting α-glucosidase activity than quercetin (IC50 3.80 ± 0.28 µg/ml) (Supplementary Table 4).
Although none of the tested compounds was more effective than allopurinol (reference drug) in inhibiting xanthine oxidase (XO) activity (Supplementary Table 5) (IC50 0.65 ± 0.07 µg/ml), compound 8f (IC50 3.00 ± 1.41 µg/ml) was the most active among the tested compounds.
Conclusions
A series of 25 compounds incorporating the 3H-quinazolin-4-one scaffold were synthesised , 20 of which were new and fully characterised. Twenty-one compounds were screened for their cytotoxicity on human CRC cell lines HT-29 and SW620 using the MTT assay. Six compounds namely, 8a, 8f, 8j, 15a, 15b, and 15d potently reduced viability of both cell lines, with 15d the most active in this set displaying residual viability 38%, 36.7% against HT-29 and SW620 cells lines, respectively. Further mechanistic studies using 15d revealed that this compound inhibited the cell proliferation of HT-29 and SW620 cell lines in a dose- and time-dependent manner with IC50 values ∼20 µg/ml. Evaluation of anti-apoptotic protein expression by compound 15d revealed that it reduced the viability of the used human CRC cells by down regulating the expression of Bcl2 and BclxL.
Based on these results, 15d could be considered as an attractive candidate for further investigations as a potential anticancer agent. Furthermore, the prepared compounds were screened for their inhibitory activities against certain phospholipases including, hG-IIA, hG-V, hG-X, NmPLA2, and AmPLA2, and five proteases namely, cathepsin-B, collagenase, thrombin, elastase, and trypsin, in addition to three enzymes involved in metabolic syndrome namely, α-amylase, α-glucosidase, and xanthine oxidase. Although none of the tested compounds showed good antiprotease activity, six compounds 8j, 8d/15a/15e, 5b, and 8f demonstrated higher antiphospholipases potential against proinflammatory isoforms hGV, hG-X, NmPLA2 and AmPLA2 than oleanolic acid, which was used as reference drug.
Therefore, these compounds would be of interest as preclinical candidates in trials for anticancer and anti-inflammatory drugs.
All the tested compounds were inactive against xanthine oxidase, whereas compounds 8d, 8h, 8j, 15a, 15b, 15e, and 15f elicited higher α-amylase inhibition than the reference drug quercetin. Furthermore, compounds 8g, 8h, and 8i were more potent in inhibiting α-glucosidase activity than the reference drug allopurinol. Consequently, these compounds can be considered as potential targets that can be further investigated for developing novel anti-diabetes mellitus (DM) drugs.
Supplemental Material
Download MS Word (411.1 KB)Acknowledgements
The authors thank the RSSU at King Saud University for their technical support.
Disclosure statement
No potential conflict of interest was reported by the authors.
Additional information
Funding
References
- Ferlay J, Soerjomataram I, Dikshit R, et al. Cancer incidence and mortality worldwide: sources, methods and major patterns in GLOBOCAN 2012. Int J Cancer 2015;136:E359–86.
- Hanahan D, Weinberg RA. The hallmarks of cancer. Cell 2000;100:57–70.
- Adams JM, Cory S. The Bcl-2 apoptotic switch in cancer development and therapy. Oncogene 2007;26:1324–37.
- Vogler M, Dinsdale D, Dyer MJS, Cohen GM. Bcl-2 inhibitors: small molecules with a big impact on cancer therapy. Cell Death Differ 2009;16:360–7.
- Soreide K, Janssen EA, Korner H, Baak JPA. Trypsin in colorectal cancer: molecular biological mechanisms of proliferation, invasion and metastasis. J Pathol 2006;209:145–56.
- Rakashanda S, Rana F, Rafiq S, et al. Role of proteases in cancer: a review. Biotechnol Mol Biol Rev 2012;7:90–101.
- Zucker S, Vacirca J. Role of matrix metalloproteinases (MMPs) in colorectal cancer. Cancer Metastasis Rev 2004;23:101–17.
- Nierodzik ML, Karpatkin S. Thrombin induces tumor growth, metastasis, and angiogenesis: evidence for a thrombin regulated dormant tumor phenotype. Cancer Cell 2006;10:355–62.
- Ho AS, Chen CH, Cheng CC, et al. Neutrophil elastase as a diagnostic marker and therapeutic target in colorectal cancers. Oncotarget 2014;5:473–80.
- Dennis EA, Cao J, Hsu YH, et al. Phospholipase A2 enzymes: physical structure, biological function, disease implication, chemical inhibition, and therapeutic intervention. Chem Rev 2011;111:6130–85.
- Luo C, Zhang H. The role of proinflammatory pathways in the pathogenesis of colitis-associated colorectal cancer. Mediators Inflamm 2017;2017:1.
- Vulcan A, Manjer J, Ohlsson B. High blood glucose levels are associated with higher risk of colon cancer in men: a cohort study. BMC Cancer 2017;17:842–9.
- Ojo OA, Afon AA, Ojo AB, et al. Inhibitory effects of solvent-partitioned fractions of two nigerian herbs (spondias mombin linn. and mangifera indica L.) on α-amylase and α-glucosidase. Antioxidants 2018;7:73.
- Li X, Meng X, Gao X, et al. Elevated serum xanthine oxidase activity is associated with the development of type 2 diabetes: a prospective cohort study. Diabetes Care 2018;41:88–890.
- Kshirsagar UA. Recent developments in the chemistry of quinazolinone alkaloids. Org Biomol Chem 2015;13:9336–52.
- Tiwary BK, Pradhan K, Nanda AK, Chakraborty R. Implication of quinazoline-4 (3H)-ones in medicinal chemistry: a brief review. J Chem Biol Ther 2015;1:1000104.
- Vavsari VF, Ziarani GM. Synthesis of 4-quinazolinones by transition metal-catalyzed processes (microreview). Chem Heterocycl Comp 2018;54:317–9.
- Ghorab MM, Alsaid MS, Soliman AM, Al-Mishari AA. Benzo[g]quinazolin-based scaffold derivatives as dual EGFR/HER2 inhibitors. J Enzyme Inhib Med Chem 2018;33:67–73.
- Mekala N, Buddepu SR, Dehury SK, et al. A novel strategy for the manufacture of idelalisib: controlling the formation of an enantiomer. RSC Adv 2018;8:15863–9.
- Seema R, Pathak Malhotra V, Nath R, et al. Synthesis and antihypertensive activity of novel quinazolin-4(3H)-one derivatives. Cent Nerv Syst Agents Med Chem 2014;14:34–8.
- Al-Omar MA, El-Azab AS, El-Obeid HA, Abdel Hamide SG. Synthesis of some new 4-(3H)-quinazoline analogs as potential antioxidant agents. J Saudi Chem Soc 2006;10:113–30.
- Jain N, Jaiswal J, Pathak A, Singour PK. Synthesis, molecular docking and evaluation of 3-{4-[2-amino-4-(substitutedphenyl)-2H-[1, 3] oxazin/thiazin-6-yl} 2-phenyl-3H-quinazolin-4-one derivatives for their anticonvulsant activity. Cent Nerv Syst Agents Med Chem 2018;18:63–73.
- Osarumwense PO, Edema MO, Usifoh O. Synthesis and anti-inflammatory activity of 4(3H)-quinazolinone and its 2-methyl and 2-phenyl-4 (3H)- quinazolinone derivatives. IOSR J Appl Chem 2018;11:12–5.
- El-Sayed NN, Alafeefy AM, Bakht MA, et al. Synthesis, antiphospholipase A2, antiprotease, antibacterial evaluation and molecular docking analysis of certain novel hydrazones. Molecules 2016;21:1664–80.
- El-Sayed NN, Al-Balawi NA, Alafeefy AM, et al. Synthesis, characterization and antimicrobial evaluation of some thiazole-derived carbamates, semicarbazones, amides and carboxamide. J Chem Soc Pak 2016;38:358–68.
- Nagase T, Mizutani T, Ishikawa S, et al. Synthesis, structure-activity relationships, and biological profiles of a quinazolinone class of histamine H3 receptor inverse agonists. J Med Chem 2008;51:4780–9.
- Nagase T, Mizutani T, Sekino E, et al. structurally constrained quinazolinone derivatives as potent and selective histamine H3 receptor inverse agonists. J Med Chem 2008;51:6889–901.
- Kumar S, Kaur H, Singh I, et al. Synthesis, characterization and biological activity of various substituted quinazolinone derivatives containing dopamine moiety. World J Chem 2009;4:195–200.
- Kumar P, Shrivastava B, Stables JP, Pandeya SN. Design, synthesis and potential 6 Hz psychomotor seizure test activity of some novel 2-(substituted)-3-{[substituted]amino}quinazolin-4(3H)-one. Eur J Med Chem 2011;46:1006–18.
- Kumar A, Singh S, Saxena AK, Shanker K. Synthesis of quinazolinyl pyrimidinediones and their anti-inflammatory activity. Indian J Chem 1988;27B:443–7.
- Kim H, Lee HJ, Kim DP. Integrated one-flow synthesis of heterocyclic thioquinazolinones through serial microreactions with two organolithium intermediates. Angew Chem Int Ed Engl 2015;54:1877–80.
- van Zyl EF. A survey of reported synthesis of methaqualone and some positional and structural isomers. Forensic Sci Int 2001;122:142–9.
- Soliman FSG, Shafik RM, Elnenaey EA. Synthesis of methaqualone and its diphasic titration in pure and tablet forms. J Pharm Sci 1978;67:411–3.
- Rajasekaran S, GopalKrishna R, Sanjay PPN. Gurpreet SS. Synthesis, antibacterial and in vitro antioxidant activity of 2,3-substituted quinazolin-4(3H)-ones. J Chem Pharm Res 2010;2:482–8.
- El-Baih FEM, Al-Blowy HAS, Al-Hazimi HM. Synthesis of some thienopyrimidine derivatives. Molecules 2006;11:498–513.
- Dineen TA, Zajac MA, Myers AG. Efficient transamidation of primary carboxamides by in situ activation with N,N-dialkylformamide dimethyl acetals. J Am Chem Soc 2006;128:16406–9.
- Reilly TJ. The preparation of lidocaine. J Chem Educ 1999; 76:1557.
- Lofgren NM, Lundquist BJ. Alkyl glycinanilides, Patent 2441498A, USA; 1948.
- Salman AS, Abdel-Aziem A, Alkubbat MJ. Design, synthesis of some new thio-substituted imidazole and their biological activity. Am J Org Chem 2015;5:57–72.
- Mosmann T. Rapid colorimetric assay for cellular growth and survival: application to proliferation and cytotoxicity assay. J Immunol Methods 1983;65:55–63.
- Al-Khayal K, Alafeefy A, Vaali-Mohammed MA, et al. Novel derivative of aminobenzenesulfonamide (3c) induces apoptosis in colorectal cancer cells through ROS generation and inhibits cell migration. BMC Cancer 2017;17:4–15.
- Lôbo de Araújo A, Radvanyi F. Determination of phospholipase A2 activity by a colorimetric assay using a pH indicator. Toxicon 1987;25:1181–8.
- Kunitz M. Crystalline soyabean trypsin inhibitor: II. General properties. J Gen Physiol 1947;30:291–310.
- Subramanian R, Asmawi MZ, Sadikun A. In vitro alpha-glucosidase and alpha-amylase enzyme inhibitory effects of Andrographis paniculata extract and andrographolide. Acta Biochim Pol 2008;55:391–8.
- Andrade-Cetto A, Becerra-Jiménez J, Cardenas VR. Alfa-glucosidase-inhibiting activity of some Mexican plants used in the treatment of type 2 diabetes. J Ethnopharmacol 2008;116:27–32.
- Bondet V, Brand-Williams W, Berset C. Kinetics and mechanisms of antioxidant activity using the DPPH• free radical method. LWT Food Sci Technol 1997;30:609–15.
- Zolfagharian H, Mohajeri M, Babaie M. Honey bee venom (Apis mellifera) contains anticoagulation factors and increases the blood-clotting time. J Pharmacopuncture 2015;18:7–11.
- Wacklin H. Interfacial mechanism of phospholipase A2: pH-dependent inhibition and ME-B-cyclodextrin activation. Biochemistry 2009;48:5874–81.