Abstract
Fourteen polyamine analogues, asymmetric or symmetric substituted spermine (1–9) or methoctramine (10–14) analogues, were evaluated as potential inhibitors or substrates of two enzymes of the polyamine catabolic pathway, spermine oxidase (SMOX) and acetylpolyamine oxidase (PAOX). Compound 2 turned out to be the best substrate for PAOX, having the highest affinity and catalytic efficiency with respect to its physiological substrates. Methoctramine (10), a well-known muscarinic M2 receptor antagonist, emerged as the most potent competitive PAOX inhibitor known so far (Ki = 10 nM), endowed with very good selectivity compared with SMOX (Ki=1.2 μM vs SMOX). The efficacy of methoctramine in inhibiting PAOX activity was confirmed in the HT22 cell line. Methoctramine is a very promising tool in the design of drugs targeting the polyamine catabolism pathway, both to understand the physio-pathological role of PAOX vs SMOX and for pharmacological applications, being the polyamine pathway involved in various pathologies.
Introduction
Polyamine (PA) metabolism plays an important role in cellular homeostasis, and its dysregulation may contribute to the development of several pathological states, including cancerCitation1,Citation2. Elevated concentrations of polyamines have been associated with various types of tumoursCitation3; an increase in PA catabolism reaction products, which are reactive oxygen species and aldehydes (such as acrolein), has been implicated in causing cytotoxicity and in the pathogenesis of various diseases, such as liver and neurological disorders, and strokeCitation4–11. For these reasons, the PA metabolic pathway has been considered as a rational target for therapeutic approachesCitation1,Citation12–18. To develop anti-cancer drugs, several PA analogues were designed to ‘hit’ some of the many players of this complex pathway.
To reduce the naturally occurring PA availability in cancer cells, some of the developed analogues targeting the biosynthetic pathway are, for instance, difluoromethyl-ornithine, which inhibits ornithine decarboxylase (ODC, the rate-limiting enzyme in the synthetic pathway)Citation1,Citation3,Citation18, methylglyoxal(bis)guanylhydrazone, which targets S-adenosylmethionine decarboxylaseCitation1,Citation3,Citation18 and the spermine (SPM) derivative named AMXT1501, which blocks the activity of polyamine transporters, avoiding the uptake of exogenous PACitation18. In the last years, the PA catabolic pathway is also emerging as a promising target for chemopreventionCitation1,Citation18–20. In addition to spermidine/spermine N1-acetyltransferase (SSAT), two other key enzymes of this pathway are acetylpolyamine oxidase (PAOX) and spermine oxidase (SMOX), which are H2O2 producers. PAOX and SMOX, FAD-containing amine oxidases, have been biochemically characterised in a wide variety of organisms, including vertebrates, invertebrates and yeastCitation8,Citation21–23. PAOX is a constitutively expressed enzyme and its preferred substrates are N1-acetylspermine (N1AcSPM) and N1-acetylspermidineCitation24, whereas SMOX is an inducible enzyme specific for SPMCitation8 and is highly expressed in some tissues, such as brain and skeletal muscleCitation25–27. Recently, in addition to the cytoplasmic and nuclear SMOX isoforms, SMOX activity was detected also in mitochondriaCitation28. SMOX expression is increased in some type of cancers, it is induced by some bacterial infections and inflammatory cytokines, and by some PA analogues, such as N1,N11-bis(ethyl)norspermine (BenSPM)Citation1,Citation8,Citation20,Citation29–31. In each case, the induction of SMOX is linked to an increase of H2O2 production and DNA damage, which correlates with the risk of developing dysplasia/cancerCitation18,Citation20. In addition, epigenetic factors have been recently involved in the link between increased SMOX expression and carcinogenesisCitation32.
On this basis, the availability of selective SMOX vs PAOX inhibitors would be of great value to develop chemopreventive agents. Furthermore, the selective inhibition of SMOX and PAOX, could allow for better understanding of the involvement of PA catabolism in modulating tumourigenesis and the mechanism through which some PA analogues exert their anticancer activity.
Unfortunately, most of the mammalian PAOX and SMOX inhibitors currently available, suffer from poor selectivity, and overcoming this problem still remains an important goal for the development of novel pharmacological tools. SMOX and PAOX inhibitors are generally PA analoguesCitation33, such as the irreversible and “well-known” MDL 72527 (N1,N4-bis(2,3-butadienyl)-1,4-butanediamine)Citation34 (Ki=20 and 63 μM, for purified murine PAOX and SMOX respectively)Citation33 and other butadienyl-spermidine derivatives with improved potency with respect to MDL 72527Citation35. Interestingly, some guanidine-containing derivatives have also shown promising inhibitory activity towards PAOX and SMOX. They include N-prenylagmatine (G3)Citation33, which has emerged as a good and selective competitive murine PAOX inhibitor (Ki=0.8 μM for PAOX and Ki=46 μM for SMOX), and iminoctadine (also known as guazatine), as a good but non-selective murine SMOX and PAOX inhibitor (Ki ≈ 0.5 μM). Recently, the antiseptic agent chlorhexidine has also been reported to act as a competitive inhibitor of murine PAOX and SMOXCitation36.
Three-dimensional crystal structures of enzymes may be of great support in designing and developing more selective SMOX or PAOX inhibitors. Unfortunately, in the case of mammalian enzymes, the crystal structure of murine PAOXCitation37 has been solved at present, while only molecular models are available for mammalian SMOXCitation38,Citation39. Previous site-directed mutation studies have indicated that the aminoacids Leu195 and Ala197 in the active site of PAOX (Glu216 and Ser218 in SMOX) may be important in determining the different substrate specificity between PAOX and SMOX. The SMOX E216L/S218A mutant, in which Glu216 and Ser218 are mutated into PAOX orthologous residues, can oxidise N1AcSPM in addition to SPMCitation40. However, this experimental result is not easy to explain on the basis of PAOX three-dimensional structuresCitation37, which were obtained in complex with N1AcSPM at pH 5.5, to maintain the oxidised state of FAD, and at pH 8 to obtain the reduced state of FAD, after its reaction with the substrate.
The murine PAOX crystal structureCitation37 revealed the very flexible nature of the protein and an L-shaped active site channel with a hydrophobic cavity in the interface between the domains adjacent to the family-invariant and catalytically relevant Lys315Citation38,Citation41,Citation42. The PAOX residue Asn313 (Thr in SMOX) plays an important role in the interaction with the acetyl group of N1AcSPM through hydrogen bond formation. His64 also appears to be important in the interaction with the N5 (deprotonated form) of N1Ac SPM; Asp211 interacts with both the side-chain of His64 and the backbone of Gly65, maintaining the overall structure of the enzyme. PAOX has an active site ideal for binding PAs, and the hydrophobic portion of the active site, which hosts the N1-acetyl group of the substrate, should be able to accommodate large groups allowing van der Waals interactions with the surrounding residues.
In this context, structure-activity relationship studies with novel PA analogues may reveal information about the key features of molecules which give rise to selectivity between PAOX and SMOX
In this study, SPM (1–9) and methoctramine (10–14) analoguesCitation43,Citation44, differing in the polyamine backbone and in the aromatic rings on the terminal nitrogen atoms, were used as probes to search for the optimal molecular features of PA analogues responsible for selective interactions with PAOX or SMOX. By means of a kinetic approach, the compounds were screened first as potential substrates and those inactive as substrates were tested as potential inhibitors. Methoctramine emerged as a potent and very selective PAOX inhibitor. Kinetic results were integrated with docking simulations and the effectiveness of the most potent PAOX inhibitor was validated on a cellular model.
Materials and methods
Materials and instrumentations
All chemicals were of analytical-grade and purchased from Fluka-Aldrich S.r.l. (Milan, Italy) with the exception of Amplex Red reagent (10-acetyl-3,7-dihydroxyphenoxazine), purchased from Molecular Probes/Invitrogen (Invitrogen s.r.l, San Giuliano Milanese, Italy). Horseradish peroxidase, polyclonal anti-PAOX antibodies produced in rabbit and secondary antibodies (HrP-labelled anti-rabbit IgG) were purchased from Sigma-Aldrich S.r.l. (Italy).
The protein concentrations of the samples were determined according to the method of BradfordCitation45, with bovine serum albumin as standard protein. Stock solutions of the assayed compounds were prepared in water, with the exception of compound 6, which was dissolved in dimethyl sulphoxide, and compounds 8 and 9, dissolved in chloroform/methanol (1/1 v/v). A Cary-Eclipse fluorimeter (Varian Inc., Palo Alto, CA, USA) and a Cary 50 Scan UV-visible spectrophotometer (Varian Inc.) were used for fluorescence and spectrophotometric measurements, respectively.
PA analogues were synthesised as previously reported (compounds 1, 8 and 9 in Bonaiuto et al.Citation46; compounds 2–7 in Bonaiuto et al.,Citation47; methoctramine derivatives 10–12 in Melchiorre et al.,Citation48, and Minarini et al.,Citation49; compound 13 in Tumiatti et al.,Citation50; compound 14 in Melchiorre et al.,Citation51.
Expression and purification of PAOX and SMOX proteins in E. coli cells
The recombinant PAOX and SMOX proteins were expressed in E. coli BL21 DE3 cells and purified according to Bianchi et al.Citation33 and Cervelli et al.Citation25, respectively. Purified recombinant proteins were analysed by SDS/PAGE electrophoresis to assess the grade of purity. Protein concentration was measured by the 460-nm molar extinction coefficient (ε460=9000 M−1 cm−1) which accounts for FAD absorption.
Amine oxidase activity assay
Amine oxidase activity was determined by measuring the H2O2 generation rate with a peroxidase-coupled continuous assay. Amplex Red reagent was used as fluorogenic substrate for horseradish peroxidaseCitation52. All experiments were carried out in Hepes 50 mM, at pH 7.5 and 37 °C. Phosphate buffer was not used, in order to avoid the possible formation of phosphate-SPM derivative complexesCitation53. Assays were carried out in a final volume of 800 μl, in the presence of Amplex Red (100 μM) and horseradish peroxidase type II (5 U/ml). The assay solutions containing SMOX or PAOX were pre-incubated for 2 min (in the presence or absence of the various compounds); the substrate was then added and the reaction was run continuously for 3 min. Spermine and N1AcSPM were used as substrates for SMOX and PAOX, respectively. Enzyme assay concentrations were 0.5 μg/ml and 0.3 μg/ml for PAOX and SMOX respectively, unless otherwise specified.
Initial velocities were determined by measuring the increase in fluorescence intensity (λexc=563 nm and λem=586 nm) over time; H2O2 generation rate was calculated from the change in fluorescence intensity, by means of calibration curves obtained by serial dilution of a stock solution of H2O2. For each measurement, the corresponding blank, measured in the absence of the substrate, was subtracted. No significant interference of the various compounds was observed on fluorescence intensity.
Docking simulations
Docking simulations were performed with the recently solved crystal structure of PAOX (PDB ID: 5LFOCitation37). The three-dimensional structure of small molecule ligands was built with Schrödinger Maestro 10.1 (Schrödinger, LLC, New York, NY, 2015). As literature data indicate that PAs bind within the PAOX active site in tri-protonated formCitation42, the atomic charges of the ligands in this form were calculated with the AtomicChargeCalculator web serverCitation54. Simulations were carried out with AutoDock VinaCitation55. In all simulations, to carry out “blind” predictions of small-molecule binding sites, the search space (docking grid) included the whole PAOX structure. The grid spacing was set to 1 Å per grid unit, and the exhaustiveness parameter was increased from the default value of 8–24, as suggested by Autodock Vina developers for grid sizes larger than 27,000 ÅCitation3 (see http://vina.scripps.edu/tutorial.html).
Simulations were carried out by allowing the flexibility of the residues to build the walls of the PAOX active sites, i.e. His61, Trp62, His64, Glu184, Cys186, Val187, Ser188, Phe201, Tyr204, Asn313, Lys315, Ile358, Phe375, Tyr430, Tyr472, Ser473 and Thr474. The rotatable bonds of the ligands were kept flexible in all simulations.
Cell cultures and PAOX activity in HT22 cells
HT22 cells (mouse hippocampal neuronal cell line) were cultured in Dulbecco’s modified Eagle medium, DMEM high glucose supplemented with 10% foetal bovine serum, 4 mM L-glutamine, 100 U/ml penicillin and 100 μg/ml streptomycin (all from Gibco, Thermo Fischer Scientific) and maintained in a 5% CO2 (v/v) humidified atmosphere at 37 °C. Cells were grown until they reached 70% confluence for a maximum of 25–30 passages.
After trypsinisation, HT22 cell pellets were washed twice with cold PBS, centrifuged (300 x g for 5 min), resuspended at a density of about 6 million cells/ml, and incubated in the presence of 2′7′-dichlorodihydrofluorescein diacetate (H2DCF-DA, Invitrogen) 20 μM, at 37 °C for 20 min in the dark. The cells were then pelleted, washed in PBS, resuspended in PBS containing 5 mM glucose (at about 0.5 million cells/ml) and then incubated in the presence of substrate and/or methoctramine (10). All incubations were performed in the dark. Cells in the absence of substrate and compounds were run in parallel and were taken as control samples of the basal H2O2 production and DCF fluorescence increments. After 2 min of pre-incubation at 37 °C with methoctramine (10), N1AcSPM 2 μM was added as substrate. DCF fluorescence was monitored at various times for about 30 min (excitation at 488 nm; emission spectra in the range 500–650 nm, peak at about 530 nm). The increase of fluorescence of H2DCF of each sample was normalised to the protein concentration of the samples, which was determined at the end of each experiment after cell lysis. PAOX activity was calculated as the difference between the specific activity of samples in the presence of substrate and the corresponding control sample (absence of substrate). N1-Acetylspermine concentration was varied from 1 to 150 μM and, in whole cells, maximum PAOX activity was found at about 5–10 μM; tyramine 1 mM was also used to evaluate the MAO activity in this cellular system. At the end of the experiment, a 200-μM pulse of H2O2 was added, to confirm that H2DCF was not saturated.
After the trypsinisation procedure, HT22 cell lysate was obtained by adding the pelleted cells of 20 mM Hepes (pH 7.5), 1 mM EDTA, and protease inhibitor cocktails (4 million cells/0.1 ml). The lysate was immediately used for amine oxidase activity assays or frozen in liquid nitrogen until use. The amine oxidase activity of lysates was ascertained with the standard Amplex Red horseradish peroxidase assay method reported above, in the presence of various substrates to test the different amine oxidase activity.
Western blot analysis
SDS-polyacrylamide gel electrophoresis was performed according to the standard Laemmli methodCitation56, with a 10% acrylamide separating gel and a 5% acrylamide spacer gel in Tris-HCl buffer. Samples (HT22 lysates) were solubilised in buffer containing 8 M urea, 2% SDS and 5% β-mercaptethanol in 62.5 mM Tris-HCl, pH 6.8, boiled at T = 100 °C for about 1 min; 20% glycerol containing bromophenol blue for SDS-PAGE was then added. After electrophoresis, gels were stained for protein determination with Coomassie Brilliant Blue R-250 or for Western blotting. Western blotting was performed overnight at T = 25 °C, on nitrocellulose membranes (at 10 V and 25 mA), with a transfer buffer consisting of 20% methanol, 0.1% SDS in 50 mM Tris and 384 mM Glycine. Non-specific binding sites on membranes were blocked by incubation for 2 h in 3% of bovine serum albumin in TBS (50 mM Tris, 150 mM NaCl, pH = 7.5), prior to incubation with primary antibodies (anti-PAOX 1/400) overnight at 4 °C, washed in TBS with 0.1% Tween 20 for 4–5 h, and then incubated with secondary HRP-conjugated antibodies diluted in blocking solution. Immunoreactive species were detected by chemiluminescence reaction with luminol.
Kinetic and statistical analysis
Steady-state kinetic parameters (Vmax and Km) were calculated by fitting the Michaelis-Menten equation to the experimental data (initial rate of reactions vs substrate concentrations) by non-linear regression analysis, with Sigma Plot software, version 9.0 (Jandel Scientific, San Rafael, CA, USA) and the value of the kinetic parameter obtained from the best fit and its SEM are reported.
The mode of inhibition was determined by global fit analysis (GraphPad 5.0 software) of the initial rate of reaction vs substrate concentration curves, in the presence and absence of inhibitor, to fit equations for competitive, mixed, non-competitive and uncompetitive inhibition models. The fit giving the highest r2 value was selected to calculate the inhibition constant value (KI).
Unless stated otherwise, the correlation coefficient for linear regression was 0.98 or greater.
For calculating the IC50 value of compound 10 in the cellular system, curve fitting was performed by non-linear regression analysis using the standard “dose-response” curve equation and the Sigma Plot software.
To compare the kinetic parameters of sample with inhibitor with control sample, the Student's t-test was applied and p < .05 was defined as statistically significant.
All experiments were repeated independently at least three times, and the experimental data were expressed as the mean ± standard deviation (SD).
Results
Selection of polyamine analogues
The aim of this investigation was to discover novel PA derivatives able to discriminate between PAOX and SMOX active sites. Two series of PAs were therefore selected (). The first series included SPM analogues unsymmetrically substituted on one of the terminal amino groups with different aromatic rings, such as benzene (compound 1), naphthalene (compound 2), pyridine (compound 3) or thiophene (compound 4). In addition, to evaluate the role of secondary amino groups of SPM on SMOX/PAOX selectivity, PAs 5–8 were included in this study. In particular, the inner nitrogen atoms of compound 4 were replaced with one or two methylene groups (compounds 5 and 6, respectively) or with oxygen atoms (compound 7). Compound 8 (Bza-DIADO) is an analogue of compound 1 in which the inner nitrogen atoms were replaced with methylene groups. Compounds 6 and 8 are of particular interest, since they are derivatives of 1,12-diaminododecane (DIADO), a known PAOX inhibitorCitation33. The second group of compounds includes symmetrically substituted PAs such as N1,N12-dibenzyldodecanediamine (9), tetramine methoctramine (10) and its derivatives 11–14. Methoctramine (10) is a tetramine bearing a 2-methoxybenzyl moiety on the terminal nitrogen atoms; compounds 11–14 are characterised by inner polymethylene chains of different lengths and features. In particular, compounds 10–14 could give information on the role played by: (i) different lengths of the methylene chain separating the inner nitrogen atoms (10–12); (ii) the reduction of the inner flexibility of the molecule, as in compound 13, which bears a more rigid dipiperidine moiety; (iii) the inner secondary amine functions, by replacing them with amide groups (compound 14, also known as caproctamine).
Table 1. Chemical structure of polyamine derivatives.
Polyamine analogues as potential substrates of SMOX and PAOX
All the compounds listed in were first assayed as potential substrates; only some of the unsymmetric, monosubstituted SPM analogues behaved as substrates of SMOX and/or PAOX (see ). Compound 1 has already been reported to be a substrate of SMOX and PAOX (1 is named BnSPM in Häkkinen et al.Citation57).
Table 2. Kinetic parameters of compounds 1–8 acting as substrates of murine SMOX and PAOX.
shows that the analogues acting as substrates showed higher affinity (lower Km values) and specificity for PAOX than for SMOX, as Km values were in the nanomolar-submicromolar range for PAOX and micromolar range for SMOX. Compounds 2 and 4 were the best substrates, with Km of 25 and 44 nM for PAOX, and 15 and 10 μM for SMOX, respectively. In particular, the thiophene derivative of SPM (compound 4) emerged as the best substrate for SMOX (Km=10 μM with respect to Km SPM=490 μM and catalytic efficiency (Vmax/Km) 19 times that for SPM). For PAOX, the best substrate was compound 2, bearing the more hydrophobic and hindering naphthalene ring (Km=25 nM with respect to KmN1AcSPM=382 nM and catalytic efficiency 12 times that of N1AcSPM). It is worth noting that, among the SPM derivatives (1–4), the presence of a less hydrophobic pyridine (3) ring reduced the affinity for both enzymes.
These results clearly indicated that the presence of an aromatic ring at one of the terminal amino functions of the SPM chain maintains – and in some cases increases – the substrate profile towards SMOX and PAOX. Conversely, substitution of the two inner nitrogen atoms of the SPM chain with methylene groups (compounds 5, 6 and 8) or oxygen atoms (compound 7), greatly reduces the capability of the enzymes to catalyse the oxidative deamination of these compounds. The poor activity of PAOX for triamine 5 and the lack of activity of SMOX and PAOX towards compounds 6 and 8 support and confirm the crucial role of the inner secondary amine groups of an SPM derivative as a substrate, since their substitution may transform a substrate into an inhibitor.
Screening and selection of potential inhibitors
Compounds which did not behave like SMOX or PAOX substrates, such as 6 and 8, were tested as potential inhibitors together with compounds 9–14; chlorhexidine and DIADO were used as reference competitive inhibitorsCitation33,Citation36. For the preliminary screening, the effects of a fixed concentration of the compounds (50 μM) on the kinetic parameters were evaluated. lists Km values and residual Vmax relative to the control sample. These data clearly show that all the compounds act mainly by decreasing the affinity of the substrate for both enzymes, with a low or negligible effect on Vmax (i.e. the catalytic constant). The more effective compounds were methoctramine and its derivatives (compounds 10–12), in particular towards PAOX: the affinity for the substrate decreased more than ten times in the case of SMOX and much more in the case of PAOX (not determinable at this inhibitor concentration). Compound 13, bearing a rigid dipiperidine moiety, and compound 14, containing the inner amide functions, were very effective in decreasing the affinity of substrate for PAOX (Km increase of more than two orders of magnitude), but not for SMOX. The unsymmetrically substituted SPM analogues appeared in general less effective than methoctramine derivatives, in particular towards SMOX. Compounds 6, 8 and 9, representing derivatives of DIADO (PAOX competitive inhibitor), reduced the PAOX affinity for the substrate by about one order of magnitude, being more effective as inhibitors than their precursor DIADO. On this basis, compounds 10–13, the most potent in decreasing the affinity of the substrate for SMOX and PAOX, were selected for kinetic characterisation.
Table 3. Screening of PA analogues as potential inhibitors of SMOX and PAOX.
Kinetic characterisation of the most effective inhibitors of SMOX and PAOX activity
To investigate the type of inhibition of the selected compounds, the kinetic parameters (Vmax, Km and Vmax/Km) of SMOX and PAOX were determined in the presence of various concentrations of these PA analogues, with SPM and N1AcSPM as substrates, respectively. All the tested compounds were found to act as competitive inhibitors, as clearly shown in . The Lineweaver-Burk plots (double reciprocal plots) of the initial rate of reaction in the presence of various concentrations of methoctramine derivatives (10–13) did in fact show that only the Km values (intercept on the x-axis represents −1/Km) change in the presence of the compounds. lists the inhibition constant (Ki) and selectivity (KiSMOX/KiPAOX) values. These results clearly show that methoctramine and its derivatives (10–13) mainly target PAOX, and, in particular, methoctramine (10), with a Ki of 10 nM for PAOX vs 1200 nM for SMOX (selectivity 1:120), resulted the most potent and selective inhibitor. The decrease in the length of the inner polymethylene chain, as in 11 and 12, led to a reduced inhibitory potency and selectivity (KiSMOX: KiPAOX 9:1 for 12). A sterically constrained dipiperidine chain, as in 13 with respect to 10, also strongly affected inhibitory potency and specificity (Ki=10 nM of 10 vs Ki=440 nM of 13 for PAOX). In addition, all these methoctramine derivatives were found to be more potent PAOX inhibitors than the reference inhibitors (chlorhexidine and DIADO). In the case of SMOX, compounds 10–13 showed increased potency with respect to DIADO and similar potency with respect to chlorhexidine.
Figure 1. Compounds 10, 11 and 13 act as competitive inhibitors of murine PAOX and SMOX. Double reciprocal plots of PAOX activity in the presence of various concentration of compound 10 (A), 11 (B) and 13 (C); Double reciprocal plots of SMOX activity in the presence of various concentration of compound 10 (D), compound 11 (E) and 13 (F). Continuous lines are the results of linear regression analysis of experimental data (r > 0.98). The Ki values for the competitive mode of inhibition were calculated by global fit analysis (GraphPad 5.0 software) and are reported in .
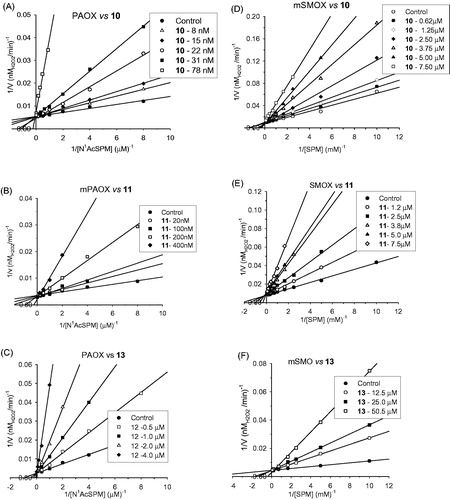
Table 4. Inhibition constant values of the most potent methoctramine derivatives and comparison with the reference inhibitors, chlorhexidine and DIADO.
Docking simulations of selected compounds on PAOX crystal structure
The structural bases of the binding of selected substrates and inhibitors to PAOX and SMOX were analysed by docking simulations via AutoDock Vina (see Materials and Methods for details). shows the PAOX complexes obtained by docking simulations with substrates 2 and 4. In both cases, the compounds bind to the PAOX active site with a conformation very similar to that observed in the PAOX crystal structure for substrate N1AcSPM, the terminal primary amino group of the compound being located near Phe201 and the hydrophobic moiety on the other end of the molecule contacting Phe375. Both compounds are characterised by the presence of secondary amino groups and this is a prerequisite for a PA to act as a substrate of animal polyamine oxidases, which, in fact, oxidise the carbon atom in eso with respect to a secondary amino group. Thus, the binding mode observed and the vicinity of the carbon atom in eso of the compounds to the redox-active N5 atom of FAD (as observed for the experimental structure of the PAOX- N1AcSPM complex), explain fairly well their ability to act as substrates.
Figure 2. Best docking poses obtained for the substrates 2 (A) and 4 (B) compared with the experimentally determined structure of the PAOX-N1AcSPM complex (C).
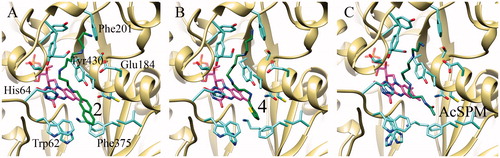
Inhibitors 10–13 are all predicted to bind in the PAOX active site, matching the competitive nature of their inhibition. Analysis of the docking complexes () shows that all the inhibitors mainly bind through hydrophobic interactions with Trp62 and Phe375. However, in the case of 10, a hydrogen bond was observed between one of the inhibitor’s amino groups and Tyr430. In addition, the latter inhibitor adopts a “folded” conformation within the PAOX active site, probably due to its length, as the 2-methoxybenzyl rings stack on to each other on Trp62. This intramolecular interaction and the hydrogen bond formed with Tyr430 may lie at the root of the high affinity for PAOX measured for this inhibitor.
Figure 3. Best docking poses obtained for the inhibitors structurally related to methoctramine (10–13) in complex with PAOX. Compound 10 in panel A, 11 in B, 12 in C and 13 in D. Hydrogen bond between Tyr430 and the inhibitor 10 is highlighted in green and indicated by a black arrow.
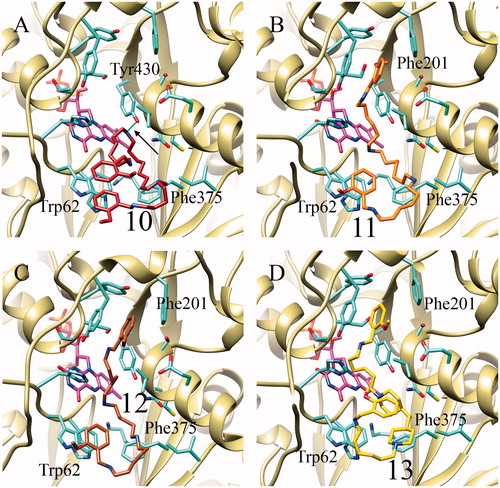
For completeness, docking simulations of substrates and inhibitors were also carried out on a recently published SMOX molecular model based on the PAOX crystal structureCitation39. shows the SMOX complexes obtained by docking simulations with substrates 2, 4 and SPM. Both the synthetic substrates and the natural substrate are predicted to bind through hydrophobic interactions with Trp225. Additional electrostatic interactions with His82 and Glu205 are predicted to stabilise compound 2. Similarly, both 4 and SPM are predicted to interact with Glu208. It is worth noting that, in SMOX, the primary amino group points towards the FAD cofactor, unlike PAOX. Also in the case of SMOX, inhibitors 10–13 are all predicted to bind in the active site, once again matching the competitive nature of their inhibition. Analysis of the docking complexes () indicates that all the inhibitors mainly act through hydrophobic interaction with Trp225. An additional hydrophobic interaction with Trp80 was observed in the case of the compound 10 complex, the most potent according to the kinetic results. Lastly, in the case of compounds 10–12-SMOX complexes, electrostatic interactions were also observed between the inhibitors’ secondary amine groups and Glu208.
Figure 4. Representative docking poses obtained for the substrates 2 (A), 4 (B) and SPM (C) onto the SMOX structural model. In each case the representative pose shown in the figure is one of the nine top-scoring poses according to Autodock Vina scoring function.
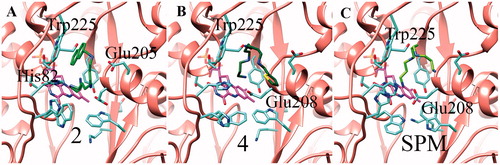
Figure 5. Representative docking poses obtained for the inhibitors 10-13 in complex with the SMOX structural model. Compound 10 in panel A, 11 in B, 12 in C and 13 in D. In each case the representative pose shown in the figure is one of the nine top-scoring poses according to Autodock Vina scoring function.
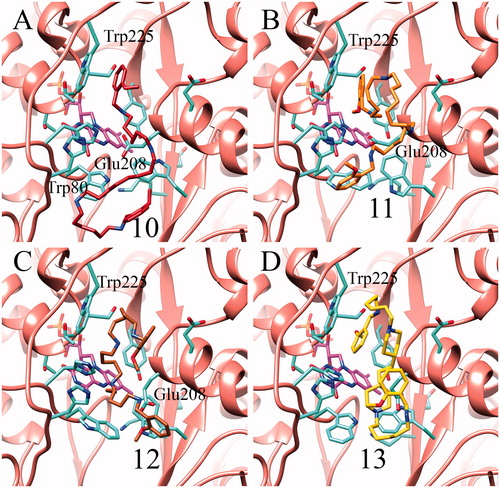
Biological activity of methoctramine as inhibitor: selectivity towards other AOs and in cell activity
In order to evaluate the potential effects of the PA analogues on a cellular system and then in a more complex biological system, it is important to collect information on their selectivity also on other types of amine oxidases. In , the behaviour and the kinetic parameters of compounds 2, 4 (the best SMOX and PAOX substrates), 10 and 13 (the best inhibitors) were compared with those previously obtained with human semicarbazide-sensitive amine oxidase/vascular adhesion protein-1 (SSAO/VAP-1)Citation46 and MAOs (human MAO A and MAO B)Citation47. It clearly appears that compound 10 emerged as the best and most selective PAOX inhibitor. The less potent and selective compound 13 (KiSMOX/KiPAOX =17/1) was previously found to be a very poor, almost unaffected inhibitor of MAOs and SSAO/VAP-1 (Ki>100 μM) in comparison with PAOX and SMOX. It is to emphasise that the selectivity profile of methoctramine versus the other amine oxidases is much better in comparison with that of DIADO, which is a selective murine PAOX inhibitor, (Ki=8 μM for PAOX vs Ki=1 mM for SMOX)Citation33, but also an excellent substrate of the human SSAO/VAP-1 (Km=13 μM) and of MAO ACitation58, differently from methoctramine.
Table 5. Inhibition constant (Ki) or Km values of the most effective inhibitors (10 and 13) or substrates (2 and 4) of SMOX and PAOX in comparison with the Ki or Km values for human MAO A, MAO B and SSAO/VAP-1.
In addition, shows that compound 2, the best PAOX substrate, is not active on MAOs and SSAO/VAP-1, whereas compound 4, the best SMOX substrate, was found to act as a competitive inhibitor of MAO B.
On these bases, we focussed attention on the most selective compound 10 and evaluated its inhibitory activity in the mouse hippocampal HT22 cell line. This cell line was chosen because, with N1AcSPM as substrate, significant PAOX activity has been found in its cell lysate. In addition, methoctramine (10) has already been evaluated on HT22 cells (at micromolar concentrations) as an M2/M4 muscarinic receptor antagonistCitation59, proving that this cell line, widely used to evaluate neuroprotective agentsCitation60–62, possesses the property of correcting cholinergic neuron hypofunctionality by increasing the release of acetylcholine.
The presence of other amine oxidases in HT22 cell lysates was evaluated with specific substrates: tyramine for MAO, SPM for SMOX, and putrescine for diamine oxidase. The results () confirmed the known presence of MAOs (as previously reportedCitation61) and the absence of SMOX and diamine oxidase activity. In addition, activity on N1AcSPM was found to be inhibited by pre-incubation with the irreversible inhibitor MDL 72527, supporting the presence of PAOX. The expression of PAOX was also demonstrated by immunodetection of the protein in HT22 lysates (). On this basis, the PAOX activity was evaluated on whole HT22 cells, with N1AcSPM as substrate, in both the presence and absence of 10. The results () confirm the effectiveness of 10 in inhibiting PAOX activity in this cellular model, with an IC50 = 160 ± 10 nM, about 16 times higher than the Ki value found with the recombinant enzyme in vitro.
Figure 6. Amine oxidase activity and immunodetection of PAOX protein in HT22 cell lysate. (A) Amine oxidase activity was carried out in Hepes buffer, using the Amplex Red-horseradish peroxidase assay method, at 37 °C and specific substrates for the different type of amine oxidases: tyramine (1mM) for MAOs; N1AcSPM (0.25 mM) for APAOX; SPM (10mM) for SMOX and Putrescine (10 mM) for diamine oxidases. Activity with tyramine was decreased to 2% with respect to control sample, after pre-incubation of sample with pargyline (specific MAO inhibitor); activity with N1AcSPM was no detectable after pre-incubation of sample with MDL 72572. The data were presented as the means ± SD. Statistical significance: p-values <.05 when control (no inhibitor) compared with sample pre-incubated with inhibitor (paired Student’s t-test). (B) Immunodetection of PAOX protein in HT22 cells lysate. (a):SDS-PAGE 10%; (b) nitrocellulose membranes immunostained with anti-PAOX. Lane 1: lysate from HT22 cells (20 µg); Lane 2: purified mouse PAOX (1 µg); in Lane 3: purified mouse SMOX (1 µg).
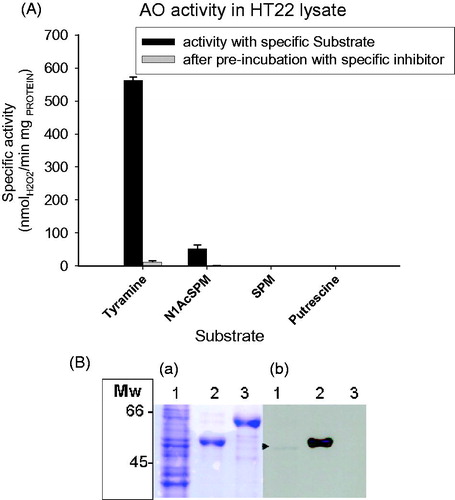
Figure 7. Effect of compound 10 on PAOX activity in HT22 cells. PAOX activity in HT22 cells was determined by using H2DCF-DA as fluorescence probe for the detection of the hydrogen peroxide generation. Cells loaded with H2DCF-DA were pre-incubated with 10 before the addition of substrate (N1AcSPM), as describe in the “Experimental procedure” Polyamine oxidase activity was calculated as the difference between the change in fluorescence of samples in the presence of substrate and the corresponding control sample (absence of substrate). Results were normalised to protein content of cells. Continuous line is the result of the best fitting of the “dose-response” curve to experimental data using the SigmaPlot 9.0 software (IC50= 160 ± 10 nM; r > 0.98; p<.05).
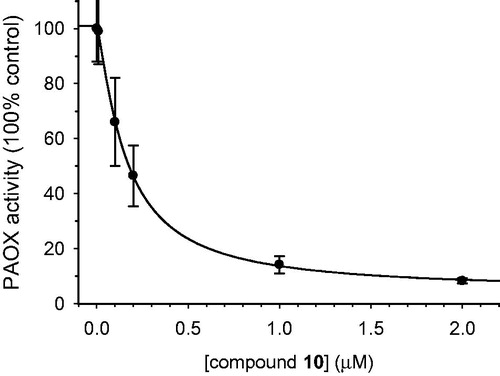
Discussion
With the aim to develop novel anticancer agents and therapeutic approaches, many researches have been focussed on the design of PA analogues to target the catabolic PA pathwayCitation1,Citation14,Citation16,Citation18. In particular, the discovery of PA analogues acting as substrates or inhibitors of PAOX and SMOX deserves great interest in the search of anticancer and chemopreventive agents.
According to the literature, a novel anticancer pharmacological approach proposes the use of PA analogues as substrates of polyamino oxidases, to induce cell death. It has been demonstrated that, after bovine serum amino oxidase delivering into B16 melanoma cells, a slow release of endogenous SPM metabolites (H2O2 and acrolein) was induced, leading to apoptosis and inhibition of tumour growthCitation12,Citation13,Citation63,Citation64. In previous studies, among the various types of PA analogues investigated to discover selective SMOX vs PAOX substratesCitation24,Citation57,Citation65–67, the pentamine N1-(3-aminopropyl)-N4-(3-((3-aminopropyl)amino)propyl)butane-1,4-diamine pentahydrochloride (3343 in Takao et al.Citation67) emerged as a very good substrate of SMOX (Vmax/Km about 2 order of magnitude higher than that for SPM and no data on PAOX available).
On this basis, in the present study, unsymmetrically and symmetrically substituted polyamines structurally related to SPM (compounds 1–9) and methoctramine (compounds 10–14) were evaluated by a kinetic approach as potential substrates or inhibitors of SMOX and PAOX enzymes. Among the unsymmetrical SPM derivatives, we found that the presence of the naphthalene and thiophene ring at one terminal end (compounds 2 and 4, respectively) confers a strong affinity and very high catalytic efficiency for PAOX and SMOX, respectively, much higher than those of their physiological substrates, N1AcSPM and SPM.
According to the new pharmacological approach described above, exploiting the amino oxidase activity on PA substrates, compound 2, which joins both high catalytic efficiency and selectivity in comparison with other amine oxidases (), could be considered a valuable starting point for developing new anticancer agents. The novel substrates (PA analogues, free or after conjugation with nanoparticles) could be delivered into cancer cells highly expressing SMOX and/or PAOX to generate in situ cytotoxic products. In addition, the low Km and high Vmax/Km values of substrates, such as compounds 2 and 4, could allow their use in clinical application at low concentration, reducing drastically side effectsCitation12,Citation13.
Interestingly, several studies have highlighted the relevant role of PAOX and SMOX in carcinogenesis, and the level of these enzymes has been or highCitation29,Citation68,Citation69 in some types of cancers. The aberrant activation of PA-driven oxidative stress due to high level of SMOX and leading to tumourigenesis, supports a different and emerging pharmacological approach concerning the development of PA analogues capable to selectively inhibit SMOX or PAOX. These inhibitors could be valuable probes to investigate the carcinogenesis pathway and could have potential as chemopreventive agentsCitation18,Citation20.
In this study, we found that unlike unsymmetrical SPM analogues, the symmetrically substituted methoctramine analogues behaved mainly as PAOX inhibitors.
The main finding emerging from our analyses is that compound 10 (methoctramine), already known as a selective M2/M4 muscarinic receptor antagonistCitation70, acts also as a potent reversible murine PAOX inhibitor (Ki=10 nM on the recombinant enzyme), with excellent selectivity with respect to SMOX and other amine oxidases (MAOs and SSAO/VAP-1). To our knowledge, this is the most potent competitive inhibitor of PAOX found so far. Preliminary biological data support its effectiveness at submicromolar concentration in inhibiting PAOX activity in HT22 cells.
Taking into account the low cytotoxicity of compound 10 (methoctramine)Citation71,Citation72 and that, at a concentration of 10 μM, it did not significantly affect other targets of PA metabolism (such as ODC and SSAT)Citation71, methoctramine emerged as a very promising tool in the design of potential drugs targeting the PA catabolism pathway.
The availability of selective inhibitors of PAOX could enable to discriminate between the role played by SMOX compared to PAOX in the inflammation-induced carcinogenesis and in other pathologies, in view of new therapeutic applications.
Further studies are necessary and are in progress to evaluate the novel SMOX and PAOX substrates (compounds 2 and 4) in cancer cells and to deeply investigate the inhibitory behaviour of methoctramine in different cellular systems and in systems expressing both SMOX and PAOX.
Acknowledgements
The authors thank the “International Polyamine Foundation - ONLUS” for the availability to look up in the Polyamine documentation. Dr. Andrea Pasquadibisceglie is gratefully acknowledged for his contribution to docking studies.
Disclosure statement
No potential conflict of interest was reported by the authors.
Additional information
Funding
References
- Nowotarski SL, Woster PM, Casero RA. Jr., Polyamines and cancer: implications for chemotherapy and chemoprevention. Expert Rev Mol Med 2013;15:e3.
- Cervelli M, Angelucci E, Germani F, et al. Inflammation, carcinogenesis and neurodegeneration studies in transgenic animal models for polyamine research. Amino Acids 2014;46:521–30.
- Gerner EW, Meyskens FL. Jr., Polyamines and cancer: old molecules, new understanding. Nat Rev Cancer 2004;4:781–92.
- Seiler N. Oxidation of polyamines and brain injury. Neurochem Res 2000; 25:471–90.
- Tomitori H, Usui T, Saeki N, et al. Polyamine oxidase and acrolein as novel biochemical markers for diagnosis of cerebral stroke. Stroke 2005;36:2609–13.
- Park MH, Igarashi K. Polyamines and their metabolites as diagnostic markers of human diseases. Biomol Ther (Seoul) 2013;21:1–9.
- Pegg AE. Toxicity of polyamines and their metabolic products. Chem Res Toxicol 2013;26:1782–800.
- Cervelli M, Amendola R, Polticelli F, Mariottini P. Spermine oxidase: ten years after. Amino Acids 2012;42:441–50.
- Cervelli M, Bellavia G, D'Amelio M. A new transgenic mouse model for studying the neurotoxicity of spermine oxidase dosage in the response to excitotoxic injury. PLoS One 2013;8:e64810.
- Cervetto C, Vergani L, Passalacqua M, et al. Astrocyte-dependent vulnerability to excitotoxicity in spermine oxidase-overexpressing mouse. Neuromolecular Med 2016;18:50–68.
- Sharmin S, Sakata K, Kashiwagi K, et al. Polyamine cytotoxicity in the presence of bovine serum amine oxidase. Biochem Biophys Res Commun 2001;282:228–35.
- Agostinelli E, Belli F, Molinari A, et al. Toxicity of enzymatic oxidation products of spermine to human melanoma cells (M14): sensitization by heat and MDL 72527. Biochim Biophis Acta 2006;1763:1040–50.
- Agostinelli E, Condello M, Tempera G, et al. The combined treatment with chloroquine and the enzymatic oxidation products of spermine overcomes multidrug resistance of melanoma M14 ADR2 cells: a new therapeutic approach. Int J Oncol 2014;45:1109–22.
- Wallace HM, Fraser AV. Inhibitors of polyamine metabolism: review article. Amino Acids 2004;26:353–65.
- Amendola R, Cervelli M, Tempera G, et al. Spermine metabolism and radiation-derived reactive oxygen species for future therapeutic implications in cancer: an additive or adaptive response. Amino Acids 2014;46:487–98.
- Casero RA, Jr, Marton LJ. Targeting polyamine metabolism and function in cancer and other hyperproliferative diseases. Nat Rev Drug Discov 2007;6:373–90.
- Calcabrini A, Arancia G, Marra M, et al. Enzymatic oxidation products of spermine induce greater cytotoxic effects on human multidrug-resistant colon carcinoma cells (LoVo) than on their wild-type counterparts. Int J Cancer 2002;99:43–52.
- Murray-Stewart TR, Woster PM, Casero RA. Jr., Targeting polyamine metabolism for cancer therapy and prevention. Biochem J 2016;473:2937–53.
- Casero RA, Jr, Pegg AE. Polyamine catabolism and disease. Biochem J 2009;421:323–38.
- Murray Stewart T, Dunston TT, Woster PM, Casero RA. Jr., Polyamine catabolism and oxidative damage. J Biol Chem 2018;293:18736–45.
- Pozzi MH, Gawandi V, Fitzpatrick PF. Mechanistic studies of para-substituted N,N'-dibenzyl-1,4-diaminobutanes as substrates for a mammalian polyamine oxidase. Biochemistry 2009;48:12305–13.
- Cervelli M, Salvi D, Polticelli F, et al. Structure-function relationships in the evolutionary framework of spermine oxidase. J Mol Evol 2013;76:365–70.
- Polticelli F, Salvi D, Mariottini P, et al. Molecular evolution of the polyamine oxidase gene family in Metazoa. BMC Evol Biol 2012;12:90.
- Wang Y, Hacker A, Murray-Stewart T, et al. Properties of recombinant human N1-acetylpolyamine oxidase (hPAO): potential role in determining drug sensitivity. Cancer Chemother Pharmacol 2005;56:83–90.
- Cervelli M, Bellini A, Bianchi M, et al. Mouse spermine oxidase gene splice variants. Nuclear subcellular localization of a novel active isoform. Eur J Biochem 2004;271:760–70.
- Cervelli M, Fratini E, Amendola R, et al. Increased spermine oxidase (SMO) activity as a novel differentiation marker of myogenic C2C12 cells. Int J Biochem Cell Biol 2009;41:934–44.
- Bongers KS, Fox DK, Kunkel SD, et al. Spermine oxidase maintains basal skeletal muscle gene expression and fiber size and is strongly repressed by conditions that cause skeletal muscle atrophy. Am J Physiol Endocrinol Metab 2015;308:E144–58.
- Bonaiuto E, Grancara S, Martinis P, et al. A novel enzyme with spermine oxidase properties in bovine liver mitochondria: identification and kinetic characterization. Free Radic Biol Med 2015;82:88–99.
- Goodwin AC, Jadallah S, Toubaji A, et al. Increased spermine oxidase expression in human prostate cancer and prostatic intraepithelial neoplasia tissues. Prostate 2008;68:766–72.
- Chaturvedi R, Asim M, Romero-Gallo J, et al. Spermine oxidase mediates the gastric cancer risk associated with Helicobacter pylori CagA. Gastroenterology 2011;141:1696–708.
- Hu T, Sun D, Zhang J, et al. Spermine oxidase is upregulated and promotes tumor growth in hepatocellular carcinoma. Hepatol Res 2018;48:967–77.
- Murray-Stewart T, Sierra JC, Piazuelo MB, et al. Epigenetic silencing of miR-124 prevents spermine oxidase regulation: implications for Helicobacter pylori-induced gastric cancer. Oncogene 2016;35:5480–8.
- Bianchi M, Polticelli F, Ascenzi P, et al. Inhibition of polyamine and spermine oxidases by polyamine analogues. FEBS J 2006;273:1115–23.
- Bey P, Bolkenius FN, Seiler N, Casara PN. 2,3-Butadienyl-1,4-butanediamine derivatives: potent irreversible inactivators of mammalian polyamine oxidase. J Med Chem 1985;28:1–2.
- Moriya SS, Miura T, Takao K, et al. Development of irreversible inactivators of spermine oxidase and N1-acetylpolyamine oxidase. Biol Pharm Bull 2014;37:475–80.
- Cervelli M, Polticelli F, Fiorucci L, et al. Inhibition of acetylpolyamine and spermine oxidases by the polyamine analogue chlorhexidine. J Enzyme Inhib Med Chem 2013;28:463–7.
- Sjögren T, Wassvik CM, Snijder A, et al. The structure of murine N1 acetylspermine oxidase reveals molecular details of vertebrate polyamine catabolism. Biochemistry 2017;56:458–67.
- Tavladoraki P, Cervelli M, Antonangeli F, et al. Probing mammalian spermine oxidase enzyme-substrate complex through molecular modeling, site-directed mutagenesis and biochemical characterization. Amino Acids 2011;40:1115–26.
- Leonetti A, Cervoni L, Polticelli F, et al. Spectroscopic and calorimetric characterization of spermine oxidase and its association forms. Biochem J 2017;474:4253–68.
- Cervelli M, Angelucci E, Stano P, et al. The Glu216/Ser218 pocket is a major determinant of spermine oxidase substrate specificity. Biochem J 2014; 461:453–9.
- Polticelli F, Basran J, Faso C, et al. Lys300 plays a major role in the catalytic mechanism of maize polyamine oxidase. Biochemistry 2005;44:16108–20.
- Henderson Pozzi M, Gawandi V, Fitzpatrick PF. pH dependence of a mammalian polyamine oxidase: insights into substrate specificity and the role of lysine 315. Biochemistry 2009;48:1508–16.
- Minarini A, Milelli A, Tumiatti V, et al. Synthetic polyamines: an overview of their multiple biological activities. Amino Acids 2010;38:383–92.
- Melchiorre C, Bolognesi ML, Minarini A, et al. Polyamines in drugs discovery: from the universal template approach to the multitarget–directed ligand design strategy. J Med Chem 2010;53:5906–14.
- Bradford MM. A rapid and sensitive method for the quantitation of microgram quantities of protein utilizing the principle of protein-dye binding. Anal Biochem 1976;72:248–54.
- Bonaiuto E, Minarini A, Tumiatti V, et al. Synthetic polyamines as potential amine oxidase inhibitors: a preliminary study. Amino Acids 2012;42:913–28.
- Bonaiuto E, Milelli A, Cozza G, et al. Novel polyamine analogues: from substrates towards potential inhibitors of monoamine oxidases. Eur J Med Chem 2013;70:88–101.
- Melchiorre C, Minarini A, Quaglia W, Tumiatti V. Methoctramine. Drugs Future 1989;14:628–31.
- Minarini A, Budriesi R, Chiarini A, et al. Further investigation on methoctramine-related tetraamines: effects of terminal N-substitution and of chain length separating the four nitrogens on M2 muscarinic receptor blocking activity. Farmaco 1991;46:1167–78.
- Tumiatti V, Minarini A, Milelli A, et al. Structure-activity relationships of methoctramine-related polyamines as muscarinic antagonist: effect of replacing the inner polymethylene chain with cyclic moieties. Bioorg Med Chem 2007;15:2312–21.
- Melchiorre C, Andrisano M, Bolognesi ML, et al. Acetylcholinesterase noncovalent inhibitors based on a polyamine backbone for potential use against Alzheimer’s disease. J Med Chem 1998;41:4186–9.
- Zhou N, Panchuk-Voloshina N. A one-step fluorometric method for the continuous measurement of monoamine oxidase activity. Anal. Biochem 1997;253:169–74.
- Corazza A, Stevanato R, Di Paolo ML, et al. Effect of phosphate ion on the activity of bovine plasma amine oxidase. Biochem Biophys Res Commun 1992;189:722–7.
- Ionescu CM, Sehnal D, Falginella FL, et al. AtomicChargeCalculator: interactive web-based calculation of atomic charges in large biomolecular complexes and drug like molecules. J Cheminform 2015;7:50.
- Trott O, Olson AJ. AutoDock Vina: improving the speed and accuracy of docking with a new scoring function, efficient optimization, and multithreading. J Comp Chem 2010;31:455–61.
- Laemmli UK. Cleavage of structural proteins during the assembly of the head of bacteriophage T4. Nature 1970;227:680–5.
- Häkkinen MR, Hyvönen M, Auriola S, et al. Metabolism of N-alkylated spermine analogues by polyamine and spermine oxidases. Amino Acids 2010;38:369–81.
- Bonaiuto E, Lunelli M, Scarpa M, et al. A structure-activity study to identify novel and efficient substrates of the human semicarbazide-sensitive amine oxidase/VAP-1 enzyme. Biochimie 2010;92:858–68.
- Liu J, Li L, Suo WZ. HT22 hippocampal neuronal cell line possesses functional cholinergic properties. Life Sci 2009;84:267–71.
- Lee DS, Ko W, Kim DC, et al. Cudarflavone B provides neuroprotection against glutamate-induced mouse hippocampal HT22cell damage through the Nrf2 and PI3K/Akt signaling pathways. Molecules 2014;19:10818–31.
- Cao X, Wei Z, Gabriel GG, et al. Calcium-sensitive regulation of monoamine oxidase-A contributes to the production of peroxyradicals in hippocampal cultures: implications for Alzheimer disease-related pathology. BMC Neurosci 2007;8:73.
- Sawa K, Uematsu T, Korenaga Y, et al. Krebs cycle intermediates protective against oxidative stress by modulating the level of reactive oxygen species in neuronal HT22. Cells 2017; 6:E21.
- Averill-Bates DA, Cherif A, Agostinelli E, et al. Anti-tumoral effect of native and immobilized bovine serum amine oxidase in a mouse melanoma model. Biochem Pharmacol 2005;69:1693–704.
- Averill-Bates DA, Ke Q, Tanel A, et al. Mechanism of cell death induced by spermine and amine oxidase in mouse melanoma cells. Int J Oncol 2008;32:79–88.
- Weisell J, Hyvo¨nen MT, Ha¨kkinen MR, et al. Synthesis and biological characterization of novel charge-deficient spermine analogues. J Med Chem 2010;53:5738–48.
- Ucal S, Häkkinen MR, Alanne AL, et al. Controlling of N-alkylpolyamine analogue metabolism by selective deuteration. Biochem J 2018;475:663–76.
- Takao K, Shirahata A, Samejima K, et al. Pentamines as substrate for human spermine oxidase. Biol Pharm Bull 2013;36:407–11.
- Goodwin AC, Shields CED, Wu S, et al. Polyamine catabolism contributes to enterotoxigenic Bacteroides fragilis-induced colon tumorigenesis. Proc Natl Acad Sci USA 2011;108:15354–9.
- Chaturvedi R, de Sablet T, Asim M, et al. Increased Helicobacter pylori-associated gastric cancer risk in the Andean region of Colombia is mediated by spermine oxidase. Oncogene 2015;34:3429–40.
- Melchiorre C, Minarini A, Angeli P, et al. Polymethylene tetraamines as muscarinic receptor probes. Trends Pharmacol Sci 1989;Suppl:55–9.
- Zini M, Passariello CL, Gottardi D, et al. Cytotoxicity of methoctramine and methoctramine-related polyamines. Chem Biol Interact 2009;181:409–16.
- Minarini A, Zini M, Milelli A, et al. Synthetic polyamines activating autophagy: effects on cancer cell death. Eur J Med Chem 2013;67:359–66.