Abstract
New anticancer agents are highly needed to overcome cancer cell resistance. A novel series of pyrimidine pyrazoline-anthracene derivatives (PPADs) (4a-t) were designed and synthesised. The anti-liver cancer activity of all compounds was screened in vitro against two hepatocellular carcinoma (HCC) cell lines (HepG2 and Huh-7) as well as normal fibroblast cells by resazurin assay. The designed compounds 4a-t showed a broad-spectrum anticancer activity against the two cell lines and their activity was more prominent on cancer compared to normal cells. Compound 4e showed high potency against HepG2 and Huh-7 cell lines ((IC50=5.34 and 6.13 μg/mL, respectively) comparable to that of doxorubicin (DOX) activities. A structure activity relationship (SAR) has been investigated and compounds 4e, 4i, 4m, and 4q were the most promising anticancer agents against tested cell lines. These compounds induced apoptosis in HepG2 and Huh-7 cells through significant activation of caspase 3/7 at all tested concentrations. In conclusion, 4e could be a potent anticancer drug.
Graphical Abstract
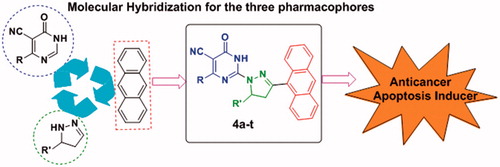
Introduction
Cancer (called tumour) is the result of uncontrolled growth and spread of abnormal cellsCitation1. Cancer is considered the leading cause of death worldwide based on the world health organisation (WHO) statistics. The most common types of cancer are lung, stomach, liver, colorectal, and female breast cancers. Hepatocellular carcinoma (HCC), one of the most common cancer types, is a primary malignancy of the liver and occurs predominantly in patients with underlying chronic liver disease. The mortality rate of HCC has significantly increased worldwide. In 2018, the American Cancer Society estimated that new liver cancer patients in USA to be over 40,000 casesCitation2. One of the most common risk factors for developing HCC is the chronic viral infection with hepatitis C virus (HCV) or hepatitis B virus (HBV) or bothCitation3. There are many therapeutic strategies for targeting HCC. This includes chemotherapy, radiotherapy, and immunotherapy. These types of treatment are often accompanied by high systemic toxicity and sometimes drug resistance. Therefore, new therapeutic agents are highly needed to overcome this tumour drug resistanceCitation4.
Pyrimidine ring is one of the mostly used heterocyclic scaffolds in medicinal chemistry. Pyrimidine derivatives have been well recognised for their therapeutic applications i.e. antiviralCitation5,Citation6, antibacterialCitation7,Citation8, antifungalCitation9, anti-inflammatoryCitation10, COX-2 inhibitorsCitation11, antioxidantCitation12, antithyroidCitation13, anticonvulsantCitation14, and anti-diabeticCitation15. Pyrimidine ring is also an integral part of DNA nucleic acid composition. Various drugs containing pyrimidine nucleus are being used as potent anticancer agents through different mechanisms of action i.e. 5-Fluorouracil® (5-FU) I as thymidylate synthase inhibitorCitation16, Merbarone® II as DNA topoisomerase II (topoII) catalytic inhibitorCitation17, Ceritinib (LDK378) III as anaplastic lymphoma kinase (ALK) inhibitorCitation18, Dasatinib IV as multi-targeted of Bcr-Abl and Src family kinases inhibitor apoptosis inducerCitation19, Imatinib V as a receptor tyrosine kinase (TKI) inhibitorCitation20, Ibrutinib (IBR) VI as Bruton’s tyrosine kinase (BTK) inhibitorCitation21, Ruxolitinib (INC424) VII as Janus kinase (JAK) inhibitorCitation22 and Nilotinib VIII as tyrosine kinase inhibitor and apoptosis inducerCitation23 ().
Pyrazoline ring is another widely used heterocyclic nucleus in medicinal chemistry because of its wide spectrum of pharmacological activities i.e. anti-inflamatoryCitation24, antibacterialCitation25, and antioxidantCitation26 activities. Many pyrazoline derivatives was found to have anticancer activity against various human cancer types with different mode of action i.e. Pyrazoloacridine (PZA) (IX)Citation27, Axitinib (AG013736) (X)Citation28, Pazopanib (GW786034) (XI)Citation29 and 3–(5′-Hydroxymethyl-2′-furyl)-1-benzyl indazole (YC-1) (XII)Citation30. New pyrazoline derivatives XIII and XIV were reported as potential anticancer agents in hepatic HepG2 cancer cell line and induced HepG2 cells apoptosis Citation31,Citation32 ().
Another organic moiety of high interest as anticancer pharmacophore is the anthracene nucleus. Several anthracene derivatives showed significant anticancer activity against a wide range of human tumour cell linesCitation33–35 i.e. the well-known Doxorubicin (DOX) XV, is a Topoisomerase II inhibitorCitation36 and anthracene-9-ylmethylene-[2-methoxyethoxymethylsulfanyl]-5-pyridin-3-yl- [1, 2, 4] triazole-4-amine (HL-37) XVICitation37 ().
The medicinal chemistry importance of the three pharmacophores, i.e. pyrimidine, pyrazoline and anthracene, encouraged us to design novel series of compounds hybridised with the three moieties. The hybridisation of these pharmacophores in a single molecule () is expected to result in a hybrid molecule that possesses anticancer activity. In this study, we report the design, synthesis and anticancer activity of a novel hybrids of pyrimidine derivatives (4a-t) bearing pyrazoline and anthracene pharmacophores against both human liver cancer cell lines (HepG2 and Huh-7) and normal fibroblast cells by resazurin assay. In addition, apoptosis and effects on caspase-3 and -7 activation against human liver cancer cell lines HepG2 and Huh-7 were also performed for the most active derivatives. Structure and activity relationship (SAR) and possible mechanisms of action of these compounds were also investigated.
Materials and methods
Instruments
All melting points were determined with Electro-thermal IA 9100 apparatus (Shimadzu, Tokyo, Japan) and were uncorrected. FT-IR spectra were recorded as potassium bromide pellets on a Perkin-Elmer 1650 spectrophotometer (PerkinElmer, Waltham, MA), Faculty of Science, Cairo University, Cairo, Egypt. 1HNMR and 13C-NMR spectra were recorded in DMSO-d6 on a Varian Mercury (300 MHz) spectrometer (Varian, Oxford, UK) and chemical shifts were given as ppm from TMS as internal reference (Faculty of Science, Cairo University, Cairo, Egypt). Mass spectra were recorded on 70 eV EI Ms-QP 1000 EX (Shimadzu, Tokyo, Japan), Faculty of Science, Cairo University, Cairo, Egypt. Microanalyses were performed using Vario, Elementar apparatus (Shimadzu, Tokyo, Japan), Organic Microanalysis Unit, Faculty of Science, Cairo University, Cairo, Egypt and the results were within the accepted range (0.40) of the calculated values. Column chromatography was performed on (Merck & Co., Kenilworth, NJ) silica gel 60 (particle size 0.06–0.20 mm).
Chemistry
6-Aryl-4-oxo-2-thioxo-1,2,3,4-tetrahydropyrimidine-5-carbonitriles (1a-e)
The titled compounds 1a-e was synthesised according to the reported methodsCitation38.
6-Aryl-2-hydrazinyl-4-oxo-1,4-dihydropyrimidine-5-carbonitriles (2a-e)
The titled compounds 2aCitation39, 2bCitation40,Citation41, 2cCitation42, 2dCitation43, and 2e were synthesised according to the reported methods.
A mixture of 1a-d (0.005 mol) and hydrazine hydrate (0.005 mol, 99%) in 30 mL ethanol was refluxed for 30 h, then cooled and poured on ice/water. The produced precipitate was filtered off, dried and crystallised from ethanol to give compounds 2a-e.
4-[4-(Dimethylamino)phenyl]-2-hydrazino-6-oxo-1,6-dihydropyrimidine-5-carbonitrile (2e)
Yellow solid, yield 60%, m.p. 214–216 °C. IR (KBr) v max (cm−1): 3366, 3295 (NH, NH2), 3069 (CH-Ar), 2959 (CH-sp3), 2209 (CN), 1689 (C=O).1H NMR (300 MHz, DMSO-d6) δ: 2.5 (s, 6H, CH3), 5.2 (s, 2H, NH2, D2O exchangeable), 7.38–7.57 (m, 4H, Ar-H), 10, 11 (s, 2H, 2NH, D2O exchangeable). 13C NMR (300 MHz, DMSO-d6): δ 43.6 (N-CH3), 90.4 (C-5 pyrimidine), 114.1–157.9 (aromatic Cs), 115.3 (CN), 159.5 (C-2 pyrimidine), 161.9(C=O), 175.7(C-6 pyrimidine). MS(EI): m/z: 270[M+] (12.3%),77 (100%). Anal. Calcd for C13H14N6O (270.29): C, 57.77; H, 5.22; N, 31.09; Found: C, 57.78; H, 5.32; N, 31.10.
(2E)-1–(9-anthryl)-3- substituted prop-2-en-1-ones (3a-d)
Compounds 3a, b, and cCitation44 were synthesised according to the literature procedure.
General procedure for the preparation of compounds (3a-d)
A mixture of 9-acetylanthracene (0.01 mol) and the appropriate aldehyde (0.01 mol) in 50 mL 10% ethanolic KOH solution was stirred at room temperature for 24 h. The solution was cooled, poured on ice/water acidified with dil. HCl. The produced precipitate was filtered off, dried and crystallised from ethanol to give compounds 3a-d.
(2E)-1–(9-anthryl)-3-(1H-indol-3-yl)prop-2-en-1-one (3d)
Brown solid, yield 85%, m.p. 170–172 °C. IR (KBr) v max (cm−1): 3177 (CH-Ar), 1665 (C=O), 1604 (C=C).1H NMR (300 MHz, DMSO-d6) δ: 6.6–8.7(m, 14H, Ar-H), 7.3 (d, 1H, J = 6.9 Hz,CH=CH), 7.5 (d, 1H, J = 19.2 Hz, CH=CH) 11.2 (s, 1H, NH, D2O exchangeable). 13C NMR (300 MHz, DMSO-d6) δ: 112.7–144.1 (aromatic Cs), 131.26, 140.8 (CH=CH), 189.7 (C=O). MS (EI): m/z: 347 [M+] (20%), 206 (100%). Anal. Calcd for C25H17NO (347.408): C, 86.43; H, 4.93; N, 4.03; O, 4.61; Found: C, 86.52; H, 5.03; N, 4.12; O, 4.70.
General procedure for the preparation of compounds (4a-t)
A mixture of compound 2a-e (4 mmol), the appropriate propenone 3a-d (4 mmol) and potassium hydroxide (0.2 g, 5 mmol) in absolute ethanol (30 mL) was refluxed for 72 h. The reaction mixture was poured on water, neutralised with 2 N hydrochloric acid and the residue was filtered off. The crude product obtained was crystallised from ethanol.
2-[3-Anthracene-9-yl-5–(4-fluoro-phenyl)-4,5-dihydro-pyrazol-1-yl]-6-oxo-4-phenyl-1,6-dihydro-pyrimidine-5-carbonitrile (4a)
Yellow solid, yield 51%, m.p. 150–151 °C. IR (KBr) v max (cm−1): 3077 (CH-Ar), 2828 (CH-sp3), 2228 (CN), 1685 (C=O), 1603 (C=N). 1HNMR (300 MHz, DMSO-d6) δ: 2.5–3.38 (dd, 2H, C4-Hpyrazoline), 4.50 (dd, 1H, J = 7.5 Hz, J = 13.8 Hz, C5-Hpyrazoline), 7–8.8 (m, 18H, Ar-H), 10.2 (s, 1H, NH, D2O exchangeable). 13C NMR (300 MHz, DMSO-d6): δ 41.7 (C-4 pyrazoline), 42.0 (C-5 pyrazoline), 91.7 (C-5 pyrimidine), 111.6–147.3 (aromatic Cs), 117.8 (CN), 152.4 (C-2 pyrazoline), 163.0 (C-2 pyrimidine), 168.1 (C=O), 175.7 (C, C-6 pyrimidine). MS (EI): m/z: 535 [M+] (8%), 178 (100). Anal. Calcd for C34H22FN5O (535.569): C, 76.25; H, 4.14; N, 13.08; Found: C, 76.29; H, 4.18; N, 13.17.
2-[3-Anthracene-9-yl-5–(4-dimethylamino-phenyl)-4,5-dihydro-pyrazol-1-yl]-6-oxo-4-phenyl-1,6-dihydro-pyrimidine-5-carbonitrile (4b)
Yellowish brown crystals, yield 58%, m.p. 120–122 °C. IR (KBr) v max (cm−1): 3062 (CH-Ar), 2920 (CH-sp3), 2223(CN), 1681 (C=O), 1620 (C=N). 1H NMR (300 MHz, DMSO-d6) δ: 2.3, 2.4 (s, 6H, N-CH3), 2.5–3.5 (dd, 2H, C4-H pyrazoline), 5.2 (dd, 1H, J = 6.6 Hz, J = 17.8 Hz, C5-H pyrazoline), 7.1–8.9 (m, 18H, Ar-H), 10.1(s, 1H, NH, D2O exchangeable). 13 C NMR (300 MHz, DMSO-d6): δ 41.0 (C-4 pyrazoline), 42.1 (C-5 pyrazoline), 43.0 (N-CH3), 91.2 (C-5 pyrimidine), 110.0–140.0 (aromatic Cs), 117.0 (CN), 152.0 (C-2 pyrazoline), 163.0 (C-2 pyrimidine), 168.0 (C=O), 175.0 (C, C-6 pyrimidine). MS (EI): m/z: 560 [M+] (4%), 439 (100%). Anal. Calcd for C36H28 N6O (560.647): C, 77.12; H, 5.03; N, 14.99; Found: C, 77.18; H, 5.14; N, 15.02.
2-[3-Anthracene-9-yl-5–(3,4,5-trimethoxy-phenyl)-4,5-dihydro-pyrazol-1-yl]-6-oxo-4-phenyl-1,6-dihydro-pyrimidine-5-carbonitrile (4c)
Brown crystals, yield 60%, m.p. 110–112 °C. IR (KBr) v max (cm−1): 3060 (CH-Ar), 2922, 2850 (CH-sp3), 2222 (CN),1680 (C=O), 1618 (C=N). 1H NMR (300 MHz, DMSO-d6) δ: 2.6–3.2 (dd, 2H, C4-H pyrazoline), 5.4 (dd, 1H, J = 6.4 Hz, J = 17.5 Hz, C5-H pyrazoline), 3.3–3.5 (s, 9H, OCH3), 6.9–8.5 (m, 16H, Ar-H), 10.2 (s, 1H, NH, D2O exchangeable). 13C NMR (300 MHz, DMSO-d6): δ 41.2 (C-4 pyrazoline), 42.0 (C-5 pyrazoline), 56.2, 56.4 (OCH3),92.0 (C-5 pyrimidine), 111.0–142.0 (aromatic Cs), 117.0 (CN), 152.2 (C-2 pyrazoline), 163.0 (C-2 pyrimidine), 168.0 (C=O), 176.0 (C, C-6 pyrimidine). MS (EI): m/z: 607 [M+] (6%), 437 (100%). Anal. Calcd for C37H29N5O4 (607.657): C, 73.13; H, 4.81; N, 11.53; Found: C, 73.18; H, 4.90; N, 11.59.
2-[3-Anthracene-9-yl-5-(1H-indol-3-yl)-4,5-dihydro-pyrazol-1-yl]-6-oxo-4-phenyl-1,6-dihydro-pyrimidine-5-carbonitrile(4d)
Yellow crystals, yield 59%, m.p. 135–137 °C. IR(KBr) v max (cm−1): 3062 (CH-Ar), 2852 (CH-sp3), 2220 (CN), 1682 (C=O), 1608 (C=N). 1HNMR (300 MHz, DMSO-d6) δ: 2.51–3.42 (dd, 2H, C4-H pyrazoline), 4.51 (dd, 1H, J = 3.3 Hz, J = 14.7 Hz, C5-H pyrazoline), 7.4–8.40 (m, 19 H, Ar-H), 10.2, 11.8 (s, 2H, NH, D2O exchangeable). 13C NMR (300 MHz, DMSO-d6): δ 41.5 (C-4 pyrazoline), 42.2 (C-5 pyrazoline), 91.2 (C-5 pyrimidine), 112.0–145.0 (aromatic Cs), 117 (CN), 152.0 (C-2 pyrazoline), 162.0 (C-2 pyrimidine), 168.0 (C=O), 170.0 (C, C-6 pyrimidine). MS (EI): m/z: 556 [M+] (3%), 419 (100%). Anal. Calcd for C36H24N6O (556.61536): C, 77.68; H, 4.35; N, 15.10; Found: C, 77.71; H, 4.41; N, 15.22.
2-[3-Anthracene-9-yl-5–(4-fluoro-phenyl)-4,5-dihydro-pyrazol-1-yl]-4–(4-fluoro-phenyl)-6-oxo-1,6-dihydro-pyrimidine-5-carbonitrile (4e)
Yellowish brown crystals, yield 50%, m.p. 138–140 °C. IR (KBr) v max (cm−1): 3058 (CH-Ar), 2920, 2848 (CH-sp3), 2222(CN), 1672 (C=O), 1621 (C = N). 1H NMR (300 MHz, DMSO-d6) δ: 2.50–3.38 (dd, 2H, C4-H pyrazoline), 5.5 (dd, 1H, J = 3.6 Hz, J = 12.6 Hz, C5-H pyrazoline), 7.30–8.25 (m, 17H, Ar-H), 10.1 (s, 1H, NH, D2O exchangeable). 13C NMR (300 MHz, DMSO-d6): δ 40.2(C-4 pyrazoline), 42.2 (C-5 pyrazoline), 93.0 (C-5 pyrimidine), 103.0–150.0 (aromatic Cs), 115.0 (CN), 158.2 (C-2 pyrazoline), 164.1 (C-2 pyrimidine), 86.2(C=O), 172.1 (C, C-6 pyrimidine). MS (EI): m/z: 553 [M+] (8%), 95 (100%). Anal. Calcd for C34H21F2N5 O (553.56): C, 73.77; H, 3.82; N, 12.65; Found: C, 73.80; H, 3.90; N, 12.70.
2-[3-Anthracene-9-yl-5–(4-dimethylamino-phenyl)-4,5-dihydro-pyrazol-1-yl]-4–(4-fluoro-phenyl)-6-oxo-1,6-dihydro-pyrimidine-5-carbonitrile (4f)
Brown crystals, yield 54%, m.p. 130–132 °C. IR (KBr) v max (cm−1): 3059 (CH-Ar), 2922, 2872 (CH-sp3), 2220 (CN), 1680 (C=O), 1614 (C=N). 1H NMR (300 MHz, DMSO-d6) δ: 2.3, 2.5 (s, 6H, N-CH3), 2.65–3.1 (dd, 2H, C4-H pyrazoline), 5.6 (dd, 1H, J = 11.8 Hz, J = 17.8 Hz, C5-H pyrazoline), 7–8.5 (m, 17 H, Ar-H), 10.0 (s, 1H, NH, D2O exchangeable). 13C NMR (300 MHz, DMSO-d6): δ 40.4 (C-4 pyrazoline), 43.0 (N-CH3), 42.4 (C-5 pyrazoline), 93.3 (C-5 pyrimidine), 104.0–147.0 (aromatic Cs), 115.0 (CN), 158.0 (C-2 pyrazoline), 164.0 (C-2 pyrimidine), 168.2 (C=O), 172.0 (C, C-6 pyrimidine). MS (EI): m/z: 578 [M+] (12%), 482 (100%). Anal. Calcd for C36H27FN6O (578.637): C, 74.72; H, 4.70; N, 14.52; Found: C, 74.83; H, 4.80; N, 14.60
2-[3-Anthracene-9-yl-5–(3,4,5-trimethoxy-phenyl)-4,5-dihydro-pyrazol-1-yl]-4–(4-fluoro-phenyl)-6-oxo-1,6-dihydro-pyrimidine-5-carbonitrile (4g)
Brown crystals, yield 58%, m.p. 154–156 °C. IR (KBr) v max (cm−1): 3062 (CH-Ar), 2930, 2850 (CH-sp3), 2223 (CN), 1681 (C=O), 1620 (C=N). 1HNMR (300 MHz, DMSO-d6) δ: 2.62–3.2 (dd, 2H, C4-H pyrazoline), 5.7 (dd, 1H, J = 6.4 Hz, J = 11.8 Hz, C5-H pyrazoline), 3.4–3.6 (s, 9H, OCH3), 7.1–8.6 (m, 15H, Ar-H), 10.1 (s, 1H, NH, D2O exchangeable). 13C NMR (300 MHz, DMSO-d6): δ 40.2 (C-4 pyrazoline), 42.3 (C-5 pyrazoline), 56.2, 56.4 (OCH3), 93.2 (C-5 pyrimidine), 105.0–145.0 (aromatic Cs), 115.0 (CN), 158.4 (C-2 pyrazoline), 164.2 (C-2 pyrimidine), 168.1 (C=O), 172.4 (C, C-6 pyrimidine). MS (EI): m/z: 625 [M+] (12%), 108 (100%). Anal. Calcd for C37H28FN5O4 (625.647): C, 71.03; H, 4.51; N, 11.19; Found: C, 71.13; H, 4.60; N, 11.22.
2-[3-Anthracene-9-yl-5-(1H-indol-3-yl)-4,5-dihydro-pyrazol-1-yl]-4–(4-fluoro-phenyl)-6-oxo-1,6-dihydro-pyrimidine-5-carbonitrile (4h)
Brown crystals, yield 59%, m.p. 143–145 °C. IR (KBr) v max (cm−1): 3177 (CH-Ar), 2893 (CH-sp3), 2226 (CN), 1680 (C=O), 1604 (C=N). 1H NMR (300 MHz, DMSO-d6) δ: 2.51–3.38 (dd, 2H, C4-H pyrazoline), 4.51 (dd, 1H, J = 7.5 Hz, J = 10.8 Hz, C5-H pyrazoline), 7.0–8.38 (m, 18H, Ar-H), 9.58, 10.20 (s, 2H, NH, D2O exchangeable). 13C NMR (300 MHz, DMSO-d6): δ 40.2 (C-4 pyrazoline), 42 0.0 (C-5 pyrazoline), 93.7 (C-5 pyrimidine), 103.6–152.0 (aromatic Cs), 115.2 (CN), 158.0 (C-2 pyrazoline), 164.0 (C-2 pyrimidine), 168.0 (C=O), 172.0 (C, C-6 pyrimidine). MS (EI): m/z: 574 [M+] (10%), 178 (100%). Anal. Calcd for C36H23FN6 O (574.605): C, 75.25; H, 4.03; N, 14.63; Found: C, 75.31; H, 4.15; N, 14.71.
2-[3-Anthracene-9-yl-5–(4-fluoro-phenyl)-4,5-dihydro-pyrazol-1-yl]-4-(1H-indol-3-yl)-6-oxo-1,6-dihydro-pyrimidine-5-carbonitrile (4i)
A brown solid, yield 55%, m.p. 184–185 °C. IR (KBr) v max (cm−1): 3062 (CH-Ar), 2940, 2850 (CH-sp3), 2219 (CN), 1682 (C=O), 1601 (C=N). 1HNMR (300 MHz, DMSO-d6) δ: 2.95–4.1 (dd, 2H, C4-H pyrazoline), 4.9 (dd, 1H, J = 6.5 Hz, J = 17.7 Hz, C5-H pyrazoline), 7–8.5 (m, 18H, Ar-H), 10.1, 10.3 (s, 2H, NH, D2O exchangeable). 13C NMR (300 MHz, DMSO-d6): δ 41.4 (C-4 pyrazoline), 43.0 (C-5 pyrazoline), 93.1 (C-5 pyrimidine), 102.1–148.1 (aromatic Cs), 114.2 (CN), 152.4 (C-2 pyrazoline), 162.0 (C-2 pyrimidine), 165.0 (C=O), 178.0 (C, C-6 pyrimidine). MS (EI): m/z: 574 [M+] (3%), 117 (100%). Anal. Calcd for C36H23FN6O (574.605): C, 75.25; H, 4.03; N, 14.63; Found: C, 75.29; H, 4.15; N, 14.68.
2-[3-Anthracene-9-yl-5–(4-dimethylamino-phenyl)-4,5-dihydro-pyrazol-1-yl]-4-(1H-indol-3-yl)-6-oxo-1,6-dihydro-pyrimidine-5-carbonitrile (4j)
A dark brown solid, yield 59%, m.p. 240–242 °C. IR (KBr) v max (cm−1): 3064 (CH-Ar), 2920, 2850 (CH-sp3), 2218 (CN), 1686 (C=O), 1598 (C=N). 1HNMR (300 MHz, DMSO-d6) δ: 2.3, 2.5 (s, 6H, N-CH3), 2.95–4.2 (dd, 2H, C4-H pyrazoline), 4.80 (dd, 1H, J = 6.3 Hz, J = 17.8 Hz, C5-H pyrazoline), 6.8–8.6 (m, 18H, Ar-H), 10, 10.2 (s, 2H, NH, D2O exchangeable). 13CNMR (300 MHz, DMSO-d6): δ 41.6 (C-4 pyrazoline), 43.0 (N-CH3), 43.2 (C-5 pyrazoline), 93.0 (C-5 pyrimidine), 105.0–145.0(aromatic Cs), 114.0 (CN), 152.4 (C-2 pyrazoline), 162.0 (C-2 pyrimidine), 164.5 (C=O), 177.0 (C, C-6 pyrimidine). MS (EI): m/z: 599 [M+] (6%), 478 (100%). Anal. Calcd for C38H29N7O (599.683): C, 76.11; H, 4.87; N, 16.35; Found: C, 76.18; H, 4.91; N, 16.40.
2-[3-Anthracene-9-yl-5–(3,4,5-trimethoxy-phenyl)-4,5-dihydro-pyrazol-1-yl]-4-(1H-indol-3-yl)-6-oxo-1,6-dihydro-pyrimidine-5-carbonitrile (4k)
A brown solid, yield 61%, m.p. 228–230 °C. IR (KBr) v max (cm−1): 3344 (CH-Ar), 2959 (CH-sp3), 2213 (CN), 1686 (C=O), 1606 (C=N). 1H NMR (300 MHz, DMSO-d6) δ: 2.5–3.03 (dd, 2H, C4-H pyrazoline), 5.5 (dd, 1H, J = 6 Hz, J = 15.6 Hz, C5-H pyrazoline), 3.3 (s, 9H, OCH3), 6.76–8.12 (m, 16H, Ar-H), 9.6, 10.6 (s, 2H, NH, D2O exchangeable). 13C NMR (300 MHz, DMSO-d6): δ 41.6 (C-4 pyrazoline), 43.7 (C-5 pyrazoline), 55.5, 55.7 (OCH3), 93.0 (C-5 pyrimidine), 102.2–146.7 (aromatic Cs), 114.0 (CN), 152.0 (C-2 pyrazoline), 162.9 (C-2 pyrimidine), 164.7 (C = O), 178.0 (C, C-6 pyrimidine). MS (EI): m/z: 646 [M+] (9%), 77 (100%). Anal. Calcd for C39H30N6O4 (646.6933): C, 72.43; H, 4.68; N, 13.00; Found: C, 72.48; H, 4.70; N, 13.04.
2-[3-Anthracene-9-yl-5-(1H-indol-3-yl)-4,5-dihydro-pyrazol-1-yl]-4-(1H-indol-3-yl)-6-oxo–1,6-dihydro-pyrimidine-5-carbonitrile (4l)
Reddish brown crystals, yield 62%, m.p. 220–222 °C. IR (KBr) v max (cm−1): 3070 (CH-Ar), 2920, 2850 (CH-sp3), 2222 (CN), 1686 (C=O), 1608 (C=N). 1H NMR (300 MHz, DMSO-d6) δ: 2.62–3.03 (dd, 2H, C4-H pyrazoline), 5.6 (dd, 1H, J = 3.6 Hz, J = 13.2 Hz, C5-H pyrazoline), 6.7–7.9 (m, 19H, Ar-H), 10, 11.6, 11.7 (s, 3H, NH, D2O exchangeable). 13CNMR (300 MHz, DMSO-d6): δ 41.4 (C-4 pyrazoline), 43.0 (C-5 pyrazoline), 93.0 (C-5 pyrimidine), 104.0 –144.2 (aromatic Cs), 114.0 (CN), 152.2 (C-2 pyrazoline), 162.4 (C-2 pyrimidine), 164.0 (C=O), 178.0 (C, C-6 pyrimidine). MS (EI): m/z: 595 [M+] (12%), 178 (100%). Anal. Calcd for C38H25N7O (595.6514): C, 76.62; H, 4.23; N, 16.46; Found: C, 76.68; H, 4.33; N, 16.51.
2-[3-Anthracene-9-yl-5–(4-fluoro-phenyl)-4,5-dihydro-pyrazol-1-yl]-6-oxo-4–(3,4,5-trimethoxy-phenyl)-1,6-dihydro-pyrimidine-5-carbonitrile (4m)
Dark brown crystals, yield 58%, m.p. 148–150 °C. IR (KBr) v max (cm−1): 3348 (CH-Ar), 2953, 2850 (CH-sp3), 2212 (CN), 1684 (C=O), 1601 (C=N). 1H NMR (300 MHz, DMSO-d6) δ: 2.9–3.1 (dd, 2H, C4-H pyrazoline), 4.9 (dd, 1H, J = 6.6 Hz, J = 16.5 Hz, C5-H pyrazoline), 3.45 (9H, s, OCH3), 6.8–8.7 (m, 15H, Ar-H), 11.4 (s, 1H, NH, D2O exchangeable). 13C NMR (300 MHz, DMSO-d6): δ 41.5 (C-4 pyrazoline), 43.7 (C-5 pyrazoline), 56.2, 56.7 (OCH3), 95.7 (C-5 pyrimidine), 102.0–146.4 (aromatic Cs), 115.5 (CN), 152.4 (C-2 pyrazoline), 159.6 (C-2 pyrimidine), 168.5(C=O), 175.7 (C, C-6 pyrimidine). MS (EI): m/z: 625 [M+] (12%), 77 (100%). Anal. Calcd for C37H28FN5O4 (625.647): C, 71.03; H, 4.51; N, 11.19; Found: C, 71.14; H, 4.60; N, 11.20.
2-[3-Anthracene-9-yl-5–(4-dimethylamino-phenyl)-4,5-dihydro-pyrazol-1-yl]-6-oxo-4–(3,4,5-trimethoxy-phenyl)-1,6-dihydro-pyrimidine-5-carbonitrile (4n)
Dark brown crystals, yield 60%, m.p. 170–172 °C. IR (KBr) v max (cm−1): 3061 (CH-Ar), 2922, 2850 (CH-sp3), 2222 (CN), 1686 (C=O), 1620(C=N). 1H NMR (300 MHz, DMSO-d6) δ: 2.5, 2.8 (s, 6H, N-CH3), 2.95–3.4 (dd, 2H, C4-H pyrazoline), 3.5 (s, 9H, OCH3), 5.3 (dd, 1H, J = 6.5 Hz, J = 17.9 Hz, C5-H pyrazoline), 6.7–8.8 (m, 15H, Ar-H), 11.1 (s, 1H, NH, D2O exchangeable). 13C NMR (300 MHz, DMSO-d6): δ 41.2 (C-4pyrazoline), 42.2 (C-5 pyrazoline), 43.0 (N-CH3), 56.3, 56.4 (OCH3), 95.7 (C-5 pyrimidine), 102.0–148.0 (aromatic Cs), 115.0 (CN), 152.2 (C-2 pyrazoline), 160.1 (C-2 pyrimidine), 168.5 (C=O), 175.4 (C, C-6 pyrimidine). MS (EI): m/z: 725 [M+] (4%), 108 (100%). Anal. Calcd for C39H34N6O4 (650.725.06): C, 71.98; H, 5.27; N, 12.91; Found: C, 72.01; H, 5.31; N,13.02.
2-[3-Anthracene-9-yl-5–(3,4,5-trimethoxy-phenyl)-4,5-dihydro-pyrazol-1-yl]-6-oxo-4–(3,4,5-trimethoxy-phenyl)-1,6-dihydro-pyrimidine-5-carbonitrile (4o)
Brown crystals, yield 62%, m.p. 165–166 °C. IR (KBr) v max (cm−1): 3061 (CH-Ar), 2920, 2850 (CH-sp3), 2223(CN), 1680 (C=O), 1618 (C=N). 1HNMR (300 MHz, DMSO-d6) δ: 2.6–3.1 (dd, 2H, C4-H pyrazoline), 4.85(dd, 1H, J = 6.6 Hz, J = 18.2 Hz, C5-H pyrazoline), 3.3, 3.5 (s, 18H, OCH3), 6.5–8.5 (m, 13H, Ar-H), 10.3 (s,1H, NH, D2O exchangeable). 13C NMR (300 MHz, DMSO-d6): δ 41.2 (C-4 pyrazoline), 42.7 (C-5 pyrazoline), 56.2, 56.4, 56.5 (OCH3), 95.2 (C-5 pyrimidine), 102.0–147.0 (aromatic Cs), 115.0 (CN), 152.4 (C-2 pyrazoline), 160.2 (C-2 pyrimidine), 168.2 (C=O), 175.7 (C, C-6 pyrimidine). MS (EI): m/z: 697 [M+] (5%), 457 (100%). Anal. Calcd for C40H35N5O7 (697.7352): C, 68.86; H, 5.06; N, 10.04; Found: C, 68.91; H, 5.17; N, 10.15.
2-[3-Anthracene-9-yl-5-(1H-indol-3-yl)-4,5-dihydro-pyrazol-1-yl]-6-oxo-4–(3,4,5-trimethoxy-phenyl)-1,6-dihydro-pyrimidine-5-carbonitrile (4p)
Brown crystals, yield 63%, m.p. 157–158 °C. IR (KBr) v max (cm−1): 3064 (CH-Ar), 2920, 2850 (CH-sp3), 2220 (CN), 1686 (C=O), 1618 (C=N). 1H NMR (300 MHz, DMSO-d6) δ: 2.51–4.03 (dd, 2H, C4-H pyrazoline), 5.51 (dd, 1H, J = 6.5 Hz, J = 17.2 Hz, C5-H pyrazoline), 3.5 (s, 9H, OCH3), 6.5–8.7 (m, 16H, Ar-H), 11.2, 11.3 (s, 2H, NH, D2O exchangeable). 13CNMR (300 MHz, DMSO-d6): δ 41.3 (C-4 pyrazoline), 42.4 (C-5 pyrazoline), 56.2, 56.7 (OCH3), 95.1 (C-5 pyrimidine), 102.0–146.0 (aromatic Cs), 115.0 (CN), 152.0 (C-2pyrazoline), 160.1 (C-2 pyrimi dine), 168.5 (C=O), 175.4 (C, C-6 pyrimidine). MS (EI): m/z: 644 [M+] (9%), 77 (100%). Anal. Calcd for C40H32N6O3 (644.720): C, 74.52; H, 5.00; N, 13.04; Found: C, 74.61; H, 5.12; N, 13.15.
2-[3-Anthracene-9-yl-5–(4-fluoro-phenyl)-4,5-dihydro-pyrazol-1-yl]-4–(4-dimethylamino-phenyl)-6-oxo-1,6-dihydro-pyrimidine-5-carbonitrile (4q)
Reddish brown solid, yield 57%, m.p. 182–184 °C. IR (KBr) v max (cm−1): 3066 (CH-Ar), 2923 (CH-sp3), 2222 (CN), 1680 (C=O), 1602 (C=N). 1H NMR (300 MHz, DMSO-d6) δ: 2.5 (s, 6H, N-CH3), 2.9–3.36 (dd, 2H, C4-H pyrazoline), 5.50 (dd, 1H, J = 6.4 Hz, J = 18.2 Hz, C5-H pyrazoline), 6.7–8.8 (m, 17H, Ar-H), 10.2 (s, 1H, NH, D2O exchangeable). 13C NMR (300 MHz, DMSO-d6): δ 40.2 (C-4 pyrazoline), 43.2 (N-CH3), 55.2 (C-5 pyrazoline), 93.2 (C-5 pyrimidine), 104.0–142.0 (aromatic Cs), 114.0 (CN), 152.1(C-2 pyrazoline), 164.0 (C-2 pyrimidine), 160.0 (C=O), 170.0 (C, C-6 pyrimidine). MS (EI): m/z: 578 [M+] (12%), 178 (100%). Anal. Calcd for C36H27FN6O (578.637): C, 74.72; H, 4.70; N, 14.52; Found: C, 74.80; H, 4.78; N, 14.60.
2-[3-Anthracene-9-yl-5–(4-dimethylamino-phenyl)-4,5-dihydro-pyrazol-1-yl]-4–(4-dimethylamino-phenyl)-6-oxo-1,6-dihydro-pyrimidine-5-carbonitrile (4r)
Brown crystals, yield 59%, m.p. 174–175 °C. IR (KBr) v max (cm−1): 3061 (CH-Ar), 2920, 2850 (CH-sp3), 2222 (CN), 1682 (C=O), 1602 (C=N). 1H NMR (300 MHz, DMSO-d6) δ: 2.3, 2.5 (s, 12H, N-CH3), 2.95–3.23 (dd, 2H, C4-H pyrazoline), 5.70 (dd, 1H, J = 6.3 Hz, J = 17.6 Hz, C5-H pyrazoline), 6.7–8.5 (m, 17H, Ar-H), 10.1 (s, 1H, NH, D2O exchangeable). 13C NMR (300 MHz, DMSO-d6): δ 40.1 (C-4 pyrazoline), 43.1 (N-CH3), 55.4 (C-5 pyrazoline), 93.2 (C-5 pyrimidine), 104.0–144.0 (aromatic Cs), 114.0 (CN), 152.0 (C-2 pyrazoline), 164.0 (C-2 pyrimidine), 160.0 (C=O), 170.2 (C, C-6 pyrimidine). MS (EI): m/z: 603 [M+] (8%), 77 (100%). Anal. Calcd for C38H33N7O (603.714): C, 75.60; H, 5.51; N, 16.24; Found: C, 75.67; H, 5.61; N, 16.30.
2-[3-Anthracene-9-yl-5–(3,4,5-trimethoxy-phenyl)-4,5-dihydro-pyrazol-1-yl]-4–(4-dimethylamino-phenyl)-6-oxo-1,6-dihydro-pyrimidine-5-carbonitrile (4s)
A brown solid, yield 61%, m.p. 160–162 °C. IR (KBr) v max (cm−1): 3064 (CH-Ar), 2922, 2850 (CH-sp3), 2222 (CN), 1684 (C=O), 1604 (CN). 1H NMR (300 MHz, DMSO-d6) δ: 2.5 (s, 6H, N-CH3), 2.85–3.3 (dd, 2H, C4-H pyrazoline), 5.60 (dd, 1H, J = 6.6 Hz, J = 17.6 Hz, C5-H pyrazoline), 3.5 (s, 9H, OCH3), 6.6–8.4 (m, 15H, Ar-H), 10.3(s, 1H, NH, D2O exchangeable). 13C NMR (300 MHz, DMSO-d6): δ 40.0 (C-4 pyrazoline), 43.3 (N-CH3), 55.2 (C-5 pyrazoline), 56.2, 56.7 (OCH3), 93.2 (C-5 pyrimidine), 104.0–143.0 (aromatic Cs), 114.0 (CN), 152.2 (C-2 pyrazoline), 164.0 (C-2 pyrimidine), 160.0 (C=O), 170.0 (C, C-6 pyrimidine). MS (EI): m/z: 650 [M+] (9%), 480 (100%). Anal. Calcd for C39H34N6O4 (650.725): C, 71.98; H, 5.27; N, 12.91; Found: C, 72.01; H, 5.32; N, 13.00.
2-[3-Anthracene-9-yl-5-(1H-indol-3-yl)-4,5-dihydro-pyrazol-1-yl]-4–(4-dimethylamino-phenyl)-6-oxo-1,6-dihydro-pyrimidine-5-carbonitrile (4t)
A brown solid, yield 62%, m.p. 190–191 °C. IR (KBr) v max (cm−1): 3333 (CH-Ar), 2944 (CH-sp3), 2211(CN), 1681 (C=O), 1600 (C=N). 1H NMR (300 MHz, DMSO-d6) δ: 2.49 (s, 6H, N-CH3), 2.56–3.33 (dd, 2H, C4-H pyrazoline), 5.49 (dd, 1H, J = 1.5 Hz, J = 18 Hz, C5-H pyrazoline), 7.4–8.5 (m, 19H, Ar-H), 9.3, 10.09 (s, 2H, NH, D2O exchangeable). 13C NMR (300 MHz, DMSO-d6): δ 40.2 (C-4 pyrazoline), 43.8 (N-CH3), 55.4 (C-5 pyrazoline), 93.9 (C-5 pyrimidine), 104.2–141.0 (aromatic Cs), 114.0 (CN), 152.0 (C-2 pyrazoline), 164.0 (C-2 pyrimidine), 159.7.0 (C=O), 170.0 (C, C-6 pyrimidine). MS (EI): m/z: 599 [M+] (8%), 117 (100%). Anal. Calcd for C38H29N7O (599.68316): C, 76.11; H, 4.87; N, 16.35; Found: C, 76.14; H, 4.94; N,16.50.
In vitro cytotoxicity activity
All compounds 2a-e, 3a-d, and PPAD’s 4a-t were subjected to a screening system for evaluation of their anticancer activity against cell line of human cancer, namely liver (HepG2 and Huh-7) cancer and normal fibroblast cells obtained from the German Cancer Research Center (DKFZ), following the Resazurin Cell Growth Inhibition AssayCitation45 in comparison to the standard treatment using DOX (see Supplementary).
Caspase-Glo 3/7 assay
The influence of our test samples on caspase 3/7 activity in liver cancer cells (HepG2 cells) was detected using Caspase-Glo 3/7 Assay kit (Promega, Madison, WI) as previously describedCitation45 (see Supplementary).
Data analysis
Results are represented as M ± SEM of at least three independent experiments. Data analysis has been done using graph pad prism and Sigma Plot 11 software.
Molecular modelling procedure
The modelling experiment described in his study was performed by using the Discovery Studio version 4.5 (Accelrys Inc., San Diego, CA) software as previously describedCitation13. The required pdb coordinates were downloaded from the Brookhaven website (www.rcsb.org). The hydrogen atoms were then added to both the small molecule and the DNA structure. The atom and bond types as well as the protonation state for the small molecule and the binding site were checked and corrected when needed. Water molecules were deleted. This was followed by minimising the complex with the Discovery Studio (DS) force field by using the default parameters. The binding mode of the designed compound and that of the DOX in bound to part of the DNA structure will be discussed later.
Results and discussion
Chemistry
The synthetic pathways adopted for the synthesis of the desired new compounds are illustrated in Scheme 1A–C. The starting 6-aryl-4-oxo-2-thioxo-1,2,3,4-tetrahydro pyrimidine-5-carbonitriles (1a-e) were synthesised from thiourea, ethyl cyanoacetate and the corresponding aldehydes in sodium ethoxideCitation38 in a one pot reaction. The corresponding 2-hydrazino-6-oxo-4-phenyl/(4-substitutedphenyl)-1,6-dihydropyrimi-dine-5-carbonitriles 2a-e were obtained through hydrazinolysis of the precursor thio derivativesCitation40 (Scheme 1A). The (2E)-1–(9-anthryl)-3- substituted prop-2-en-1-ones derivatives 3a-d were synthesised via a base-catalysed Claisen Schmidt condensation of the appropriate aldehyde and 9-acetylanthracene in 10% ethanolic KOH (Scheme 1B). Cyclocondensation reaction of the corresponding 2-hydrazinopyrimdine derivatives 2a-e and the appropriate propenones 3a-d in absolute ethanol in the presence of potassium hydroxide afforded the target compounds 4a-t (Scheme 1C). The structures of the newly synthesised compounds were in good agreement with their IR, MS, 1H-NMR, 13C-NMR and elemental analyses data. The 1H NMR spectra of compounds 4a-t showed the protons at C-4 and C-5 of the pyrazoline ring. Proton at C-4 appeared as doublet of doublet at δ 2.50–4.3 ppm and proton at C-5 appeared as doublet of doublet at δ 4.5–5.70 ppm. Other protons (N-CH3, OCH3, and aromatic Hs) were shown in their usual range. 13C-NMR showed the characteristic C-4 and C-5 carbon signals of the pyrazoline ring at δ 40– 41.7 and 42– 55.4 ppm, respectively, in addition to the other signals for the carbons of the target compounds (c.f. Experimental section).
Anticancer evaluation
This study presents the synthesis and antiproliferative activity of compounds having pyrimidine, pyrazoline, and anthracene pharmacophores. All compounds 2a-e, 3a-d, and PPAD’s 4a-t were investigated for their in vitro anti-HCC activity against HepG2, Huh-7 cell lines, and normal fibroblast cells and the anticancer drug DOX was used as standard treatment. The cytotoxic activities of tested compounds were measured as IC50 (in μg/mL) value (the dose that reduces cell growth to 50%) (). The results showed that the tested compounds exhibited good to moderate anti-proliferative activities against the tested cell lines compared to normal cells. Compounds 2a-e, 3a-d were found to show moderate activity against the two cell lines. Regarding the activity of PPAD’s 4a-t against HepG2 cell line, the results showed that compound 4f (IC50=4.2 μg/mL which is equivalent to 7.2 μM) possessed the highest degree of cytotoxicity, 4e (IC50=5.34 μg/mL which is equivalent to 9.6 μM) was almost equipotent to DOX (IC50=5.43 μg/mL which is equivalent to 10.1 μM) and 4q (IC50=6.85 μg/mL which is equivalent to 11.8 μM) was quite less potent than DOX (). The anticancer activity of the tested PPAD’s against HepG2 cell line had the following descending order: (4f > 4e > 4q > 4g > 4h > 4m > 4r > 4s> 4t > 4i > 4n > 4o > 4p >4a> 4j > 4k > 4l > 4b > 4c >4d).
Figure 5. The most potent pyrimidine derivatives against HepG2 cell line and Huh-7 cell line, respectively.
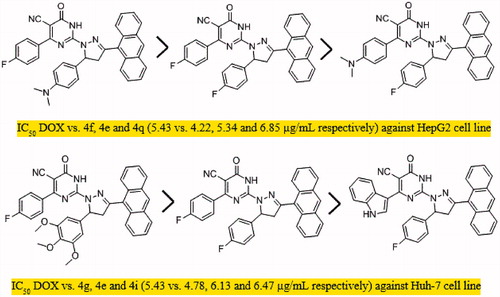
Table 1. IC50 values in μg/mL for cytotoxic activity of the compounds against hepatocellular carcinoma cell lines and fibroblast cells.
To shed the light on the SAR of these series of compound. The data suggest that the promising compounds emerging were those substituted with fluorine atom (-F) in both R and R’ such as 4e or -F and N, N(CH3)2 such as 4f and 4q. Compounds substituted with both (R) and (R’) were more effective than those having only R’ substituent as in 4b-d against HepG2 cell line. On the other hand, testing the compounds against Huh-7 cell line, all the tested PPAD’s 4a-s showed anticancer activities with IC50 values ranging from 4.78 to 16.75 μg/mL. Interestingly, compounds 4e and 4g (IC50=6.13 which is equivalent to 11.1 μM and 4.78 μg/mL which is equivalent to 7.6 μM, respectively) showed activity against Huh-7 cell line higher than DOX. Compound 4i (IC50=6.47 μg/mL which is equivalent to 11.25 μM) showed activity comparable to DOX (). However, compounds 4m, 4r, 4t, 4f, 4p, and 4q had significant activity (IC50=7.50, 7.61, 7.42, 8.45, 8.14, and 8.66 μg/mL, respectively). The activity of the tested compounds against Huh-7cell line had the following descending order: (4g > 4e >4i > 4t > 4m > 4r > 4p> 4d >4f > 4q > 4n> 4h >4b > 4s> 4c > 4l > 4j > 4k > 4o> 4a).
The data presented in this study revealed that all the tested PPAD’s 4a-t had broad spectrum anticancer activity against all screened HCC cell lines compared to normal cells. Some of our PPAD’s exhibited potent antiproliferative activity against HepG2 and Huh-7 cell lines. The cytotoxicity of all compounds was more prominent on cancer cells compared to normal ones (). These results suggest that pyrimidine pyrazoline-anthracene backbone is an interesting anticancer pharmacophore. Moreover, some PPAD’s were even more potent than the standard drug Dox. The most potent compound in this study was 4e. This could be explained by the electronegative effect of the fluorine atom on the pyrimidine and pyrazoline -anthracene backbone. PPAD’s 4e, 4i, 4m, and 4q were the most promising anticancer agents against all the tested cell lines (). Interestingly, PPAD’s 4e showed high potency against HepG2 and Huh-7 cell lines. Regarding the structure SAR of compounds (), there was a consistent relation between the lipophilicity and/or electronic property of the substituent groups R and R’ groups and the antiproliferative activity. Concerning the nature of substituent groups R and R’, the order of activity of tested compounds against tested cell lines was the following: (F > N(CH3)2 >3,4,5-(OCH3)3 > 3-Indolyl > H). The introduction of a more electronegative and a lipophilic substituent to phenyl rings of the pyrimidine and pyrazoline enhance potency against HepG2 and Huh-7 cancer cell lines as shown in the fluorine substituted PPAD’s 4e. However, a drop in potency against the same cell lines was observed with unsubstituted phenyl ring or 3-indolyl substituted PPAD’s as in 4a-d. The sensitivity of the tested cell lines to PPAD’s 4a-t was in the following descending order: (HepG2 > Huh-7).
Caspase 3/7 assay
The caspases are a family of endoproteases that provide critical links in cell regulatory networks through controlling inflammation and cell death (apoptosis). Caspase-3 and caspase-7 cleave proteins involved in programmed cell death eventsCitation46. It is well established that the induction of the apoptotic cascade is one of the main mechanisms of most of the currently available anticancer agentsCitation45,Citation47,Citation48. To determine the mechanism involved in the antitumor activity of our PPADs demonstrated above whether is a result of apoptosis or not, Caspase-Glo 3/7 assay was performedCitation47. HepG-2 () and Huh-7 cell lines () were treated with the target PPAD’s samples or DMSO (solvent control). and show that PPADs 4e, 4i, 4m, and 4q stimulated caspase activity in both cell lines at all tested concentrations and caused significant increase in activation of caspase 3/7 in a dose-dependent manner. These results suggest that our compounds induced apoptosis is, in part, due to activation of caspses3/7 and apoptosis is may be the main mechanism of action. Moreover, the apoptosis induced by the tested target compounds on Huh-7 cell line was greater than its effect on HepG2 cell line.
Molecular modelling
One possible reason for the potent anticancer activity of the designed compounds is the presence of the planar aromatic tricyclic (Anthracene) ring. The anthracene containing compounds, such as DOX or the designed compounds 4a-t, are hypothesised to function primarily at the DNA level by blocking the replication and transcription processes. The binding to DNA structure is generally hypothesised to be essential for the cytotoxic activity of these compounds. To predict and understand the possible binding mode and the respective interactions of the designed compounds with the cell DNA structure, the co-crystal structure of DOX-DNA sequence d(CGATCG) complex (PDB: 1D12) was usedCitation49. As shown in , the anthracene planar chromophore of compound 4e is intercalated with the DNA helix. While the rest of the structure is directed towards the minor groove of DNA forming additional van der Waals interactions.
Conclusions
A novel series of pyrimidine pyrazoline-anthracene hybrids 4a-t were designed and synthesised via a cyclocondensation reaction of 2-hydrazinopyrimdine derivatives 2a-e and the appropriate chalcones 3a-d and their spectral and elemental analyses proved chemical structures. The antiproliferative activity of all synthesised compounds was screened against both HCC cell lines (HepG2 and Huh-7) and normal fibroblast cells, using DOX as reference. PPAD’s 4a-t had broad spectrum anticancer activity against two HCC cell lines compared to normal cells. Compound 4f possessed the most potent cytotoxicity against HepG2 cell line. In addition, PPAD’s 4e and 4g showed decent activity against Huh-7 cell line higher than DOX. Compound 4e showed high potency against HepG2 and Huh-7 cell lines. PPAD’s 4e, 4i, 4m, and 4q were the most promising anticancer agents against all the tested cell lines. Further studies on the mechanism of action demonstrated that these compounds induce apoptosis in HepG2 and Huh-7 cell lines through significant activation of caspase 3/7 at all tested concentrations. The molecular modelling study performed suggested another possible mechanism of action for these compounds. Similar to the DOX, the presence of the tricyclic planar anthracene chromophore could intercalate with the DNA helix of the cancer cell. While the rest of the structure will be directed towards the minor groove of DNA forming additional van der Waals interactions. In conclusion, the designed pyrimidine pyrazoline-anthracene scaffold is an interesting anticancer pharmacophore and considered as novel lead scaffold for any future optimisation.
Supplemental Material
Download PDF (8.5 KB)Acknowledgements
We like to convey our grateful thanks for Mossad Sayed Mohamed (Professor of Pharmaceutical Organic Chemistry) for his inspiration and support. The authors are also thankful to Dr. Joerg Hoheisel, head of functional genome analysis department, German Cancer Research Center, Germany for his generous help and support throughout the whole work.
Disclosure statement
No conflict of interest was reported by the authors.
References
- Siegel R, DeSantis C, Virgo K, et al. Cancer treatment and survivorship statistics, 2012. CA Cancer J Clin 2012;62:220–41.
- Siegel RL, Miller KD, Jemal A. Cancer statistics, 2018. CA Cancer J Clin 2018;68:7–30.
- Tannus RK, Almeida-Carvalho SR, Loureiro-Matos CA, et al. Evaluation of survival of patients with hepatocellular carcinoma: a comparative analysis of prognostic systems. PLoS One 2018;13:e0194922.
- Zhuang H, Jiang W, Cheng W, et al. Down-regulation of HSP27 sensitizes TRAIL-resistant tumor cell to TRAIL-induced apoptosis. Lung Cancer 2010;68:27–38.
- Jin K, Yin H, De Clercq E, et al. Discovery of biphenyl-substituted diarylpyrimidines as non-nucleoside reverse transcriptase inhibitors with high potency against wild-type and mutant HIV-1. Eur J Med Chem 2018;145:726–34.
- Bai S, Liu S, Zhu Y, Wu Q. Asymmetric synthesis and antiviral activity of novel chiral amino-pyrimidine derivatives. Tetrahedron Lett 2018;59:3179–83.
- Madhu Sekhar M, Nagarjuna U, Padmavathi V, et al. Synthesis and antimicrobial activity of pyrimidinyl 1,3,4-oxadiazoles, 1,3,4-thiadiazoles and 1,2,4-triazoles. Eur J Med Chem 2018;145:1–10.
- Rizk SA, El-Naggar AM, El-Badawy AA. Synthesis, spectroscopic characterization and computational chemical study of 5-cyano-2-thiouracil derivatives as potential antimicrobial agents. J Mol Struct 2018;1155:720–33.
- Saleeb M, Sundin C, Aglar O, et al. Structure-activity relationships for inhibitors of Pseudomonas aeruginosa exoenzyme S ADP-ribosyltransferase activity. Eur J Med Chem 2018;143:568–76.
- Borik RM, Fawzy NM, Abu-Bakr SM, Aly MS. Design, synthesis, anticancer evaluation and docking studies of novel heterocyclic derivatives obtained via reactions Involving curcumin. Molecules 2018;23:1398.
- Abdelgawad MA, Bakr RB, Azouz AA. Novel pyrimidine-pyridine hybrids: synthesis, cyclooxygenase inhibition, anti-inflammatory activity and ulcerogenic liability. Bioorg Chem 2018;77:339–48.
- Mohamed MS, Youns MM, Ahmed NM. Synthesis, antimicrobial, antioxidant activities of novel 6-aryl-5-cyano thiouracil derivatives. Eur J Med Chem 2013;69:591–600.
- Awad SM, Zohny YM, Ali SA, et al. Design, synthesis, molecular modeling, and biological evaluation of novel thiouracil derivatives as potential antithyroid agents. Molecules 2018;23:2913.
- Sahu M, Siddiqui N, Sharma V, Wakode S. 5,6-Dihydropyrimidine-1(2H)-carbothioamides: synthesis, in vitro GABA-AT screening, anticonvulsant activity and molecular modelling study. Bioorg Chem 2018;77:56–67.
- Jha V, Bhadoriya KS. Synthesis, pharmacological evaluation and molecular docking studies of pyrimidinedione based DPP-4 inhibitors as antidiabetic agents. J Mol Struct 2018;1158:96–105.
- Santi DV, McHenry CS, Sommer H. Mechanism of interaction of thymidylate synthetase with 5-fluorodeoxyuridylate. Biochemistry 1974;13:471–81.
- Pastor N, Dominguez I, Orta ML, et al. The DNA topoisomerase II catalytic inhibitor merbarone is genotoxic and induces endoreduplication. Mutat Res 2012;738–739:45–51.
- Cooper MR, Chim H, Chan H, Durand C. Ceritinib: a new tyrosine kinase inhibitor for non-small-cell lung cancer. Ann Pharmacother 2015;49:107–12.
- Chan WY, Lau PM, Yeung KW, Kong SK. The second-generation tyrosine kinase inhibitor dasatinib induced eryptosis in human erythrocytes-An in vitro study. Toxicol Lett 2018;295:10–21.
- El-Mezayen NS, El-Hadidy WF, El-Refaie WM, et al. Hepatic stellate cell-targeted imatinib nanomedicine versus conventional imatinib: a novel strategy with potent efficacy in experimental liver fibrosis. J Control Release 2017;266:226–37.
- Tang B, Tang P, He J, et al. Characterization of the binding of a novel antitumor drug ibrutinib with human serum albumin: insights from spectroscopic, calorimetric and docking studies. J Photochem Photobiol B Biol 2018;184:18–26.
- Wilson GS, Tian A, Hebbard L, et al. Tumoricidal effects of the JAK inhibitor Ruxolitinib (INC424) on hepatocellular carcinoma in vitro. Cancer Lett 2013;341:224–30.
- Shaker ME, Ghani A, Shiha GE, et al. Nilotinib induces apoptosis and autophagic cell death of activated hepatic stellate cells via inhibition of histone deacetylases. Biochim Biophys Acta 2013;1833:1992–2003.
- He J, Ma L, Wei Z, et al. Synthesis and biological evaluation of novel pyrazoline derivatives as potent anti-inflammatory agents. Bioorg Med Chem Lett 2015;25:2429–33.
- Shubhalaxmi Pathak L, Ananda K, Bhat KS. Synthesis of focused library of novel aryloxyacids and pyrazoline derivatives: molecular docking studies and antimicrobial investigation. Cogent Chem 2016;2:1141388.
- P. James J, Ishwar Bhat K, More UA, Joshi SD. Design, synthesis, molecular modeling, and ADMET studies of some pyrazoline derivatives as shikimate kinase inhibitors. Med Chem Res 2018;27:546–59.
- Ramaswamy B, Mrozek E, Kuebler JP, et al. Phase II trial of pyrazoloacridine (NSC#366140) in patients with metastatic breast cancer. Invest New Drugs 2011;29:347–51.
- Ranieri G, Mammi M, Donato Di Paola E, et al. Pazopanib a tyrosine kinase inhibitor with strong anti-angiogenetic activity: a new treatment for metastatic soft tissue sarcoma. Crit Rev Oncol Hematol 2014;89:322–9.
- Na JI, Na JY, Choi WY, et al. The HIF-1 inhibitor YC-1 decreases reactive astrocyte formation in a rodent ischemia model. Am J Transl Res 2015;7:751–60.
- George DJ. Phase 2 studies of sunitinib and AG013736 in patients with cytokine-refractory renal cell carcinoma. Clin Cancer Res 2007;13:753s–7s.
- Abd-Rabou AA, Abdel-Wahab BF, Bekheit MS. Synthesis, molecular docking, and evaluation of novel bivalent pyrazolinyl-1,2,3-triazoles as potential VEGFR TK inhibitors and anti-cancer agents. Chem Pap 2018;72:2225–37.
- Xu W, Pan Y, Wang H, et al. Synthesis and evaluation of new pyrazoline derivatives as potential anticancer agents in hepG-2 cell line. Molecules 2017;22:pii: E467.
- Sangthong S, Ha H, Teerawattananon T, et al. Overcoming doxorubicin-resistance in the NCI/ADR-RES model cancer cell line by novel anthracene-9,10-dione derivatives. Bioorg Med Chem Lett 2013;23:6156–60.
- Kraicheva I, Vodenicharova E, Shenkov S, et al. Synthesis, characterization, antitumor activity and safety testing of novel polyphosphoesters bearing anthracene-derived aminophosphonate units. Bioorg Med Chem 2014;22:874–82.
- Pavitha P, Prashanth J, Ramu G, et al. Synthesis, structural, spectroscopic, anti-cancer and molecular docking studies on novel 2-[(Anthracene-9-ylmethylene) amino]-2-methylpropane-1,3-diol using XRD, FTIR, NMR, UV–Vis spectra and DFT. J Mol Struct 2017;1147:406–26.
- Cutts SM, Nudelman A, Rephaeli A, Phillips DR. The power and potential of doxorubicin-DNA adducts. IUBMB Life 2005;57:73–81.
- Xie SQ, Zhang ZQ, Hu GQ, et al. HL-37, a novel anthracene derivative, induces Ca(2+)-mediated apoptosis in human breast cancer cells. Toxicology 2008;254:68–74.
- Mohamed MS, Awad SM, Ahmed NM. Synthesis and antimicrobial evaluation of some 6-aryl-5-cyano-2-thiouracil derivatives. Acta Pharm 2011;61:171–85.
- Marella A, Akhter M, Shaquiquzzaman M, et al. Synthesis, 3D-QSAR and docking studies of pyrimidine nitrile-pyrazoline: a novel class of hybrid antimalarial agents. Med Chem Res 2015;24:1018–37.
- Taher AT, Helwa AA. Novel pyrimidinone derivatives: synthesis, antitumor and antimicrobial evaluation. Chem Pharm Bull (Tokyo) 2012;60:521–30.
- Aqlan FMS, Makki MST, Abdel-Rahman RM. Synthesis, spectroscopic studies of fluorinated pyrimido-1,2,4-triazines: protective effect against some plant pathogenic fungi. J Heterocycl Chem 2016;53:1310–7.
- Mohamed MS, Awad SM, Ahmed NM. Synthesis and antimicrobial activities of new indolyl-pyrimidine derivatives. J Appl Pharm Sci 2011;1:76–80.
- Mohamed MS, Ahmed NM. Synthesis, characterization and study of antimicrobial activity of some novel 5-cyano-2-thiouracil derivatives. Int J Pharm Sci 2014;4:591–600.
- Prabhakar M, Merry T, Tsurho S, et al. Synthesis, characterization and biological evaluation of 9-anthracenyl chalcones as anti-cancer agents. J Chem Pharm Res 2017;9:185–92.
- Nasr T, Bondock S, Youns M. Anticancer activity of new coumarin substituted hydrazide-hydrazone derivatives. Eur J Med Chem 2014;76:539–48.
- Riedl SJ, Shi Y. Molecular mechanisms of caspase regulation during apoptosis. Nat Rev Mol Cell Biol 2004;5:897–907.
- Abdallah MA, Gomha SM, Morad MA, Elaasser MM. Synthesis of pyridotriazolopyrimidines as antitumor agents. J Heterocycl Chem 2017;54:1242–51.
- Abbas I, Gomha S, El-Neairy M, et al. Synthesis and biological evaluation of novel fused triazolo[4,3-a] pyrimidinones. Turk J Chem 2015;39:510–31.
- Frederick CA, Williams LD, Ughetto G, et al. Structural comparison of anticancer drug-DNA complexes: adriamycin and daunomycin. Biochemistry 1990;29:2538–49.