Abstract
A series of novel 4-ferrocenylchroman-2-one derivatives were designed and synthesised to discover potent anti-inflammatory agents for treatment of arthritis. All the target compounds had been screened for their anti-inflammatory activity by evaluating the inhibition effect of LPS-induced NO production in RAW 264.7 macrophages. Among them, 4-ferrocenyl-3,4-dihydro-2H-benzo[g]chromen-2-one (3h) was found to be the most potent compound in inhibiting the productions of NO with low toxicity. This compound also exhibited significant inhibition of the productions of IL-6 and TNF-α in RAW 264.7 macrophages. Preliminary mechanism studies indicated that compound 3h could inhibit the activation of LPS-induced NF-κB and MAPKs signalling pathways. The in vivo anti-inflammatory effect of this compound was determined in the rat adjuvant-induced arthritis model.
Introduction
Rheumatoid arthritis (RA), which affects about 1% of the population worldwide, is a chronic systemic inflammatory disease characterised by inflammation and progressive joint destructionCitation1,Citation2. The clinical therapies to date mainly focused on nonsteroidal anti-inflammatory drugs (NSAIDs), disease-modifying antirheumatic drugs (DMARDs), and biological products like TNF-α antibody infliximab, the soluble TNF-α receptor etanercept, and interleukin-1 (IL-1)-receptor antagonist anakinraCitation3,Citation4. The unknown cause and doubtful prognosis make the disease more complicated. Moreover, the current conventional and biological therapies for RA give only partial responsesCitation5. There is still a critical need for discovering novel agents with different mechanisms of action for patients unresponsive to current therapies.
The pathologic lesions of RA are driven, in part, by the production of inflammatory mediators in synoviocytes and macrophages, likely involving the transcription factor nuclear factor κB (NF-κB)Citation6. In a previous paper, we reported a resveratrol-based cinnamic ester hybridbenzo[d] [1,3] dioxol-5-yl (E)-3-(2,4-dimethoxy-6-((E)-4-methoxystyryl) phenyl) acrylate (A, ), this compound exhibited potent inhibitory effect against LPS-stimulated NO production as well as the expressions of iNOS and COX-2 in RAW 264.7 cellsCitation7. Furthermore, this compound could exert the anti-inflammatory activity partly due to its inhibitory effect on the NF-κB signalling pathway. As our continuous work to discover novel anti-inflammatory agentsCitation8, we have made some modifications to this compound. First, given that ferrocene and its derivatives usually are robust, lipophilic, nontoxic, and have good redox propertiesCitation9, the resveratrol fragment of compound A was replaced by ferrocene moiety to afford intermediate B. Then, the acrylate group was cyclised to the corresponding compounds those bearing chromen-2-one rings (3a–3s). We then preliminarily evaluated their anti-inflammatory activities by evaluating their inhibitory effects against LPS-stimulated NO, IL-6, and TNF-α production in RAW 264.7 cells. The results indicated that when the substituted groups were naphthalenyl and benzodioxol, the corresponding compounds (3h–3j) exhibited the most potent anti-inflammatory activities. Among them, compound 3h was selected to carry out further in vitro and in vivo evaluations for exploration of the possible anti-inflammatory mechanisms.
Materials and methods
General
All commercially available reagents and solvents were purchased and used without further purification. Reactions were monitored by analytical thin-layer chromatography (TLC) and visualised under UV light (λ = 254 or 365 nm). Purification by chromatography column was carried on by using silica gel (200–300 mesh). 1H and 13C NMR (nuclear magnetic resonance) spectra were recorded using an Agilent 600 MHz spectrometer (Agilent Technologies, Palo Alto, CA). The ESI-MS spectra were recorded on a Mariner System 5304 Mass spectrometer. Melting points were determined on a XT4MP apparatus (Taike Corp., Beijing, China), and are uncorrected.
Chemistry
Synthesis of compound (2)
A mixture of ferrocenecarboxaldehyde 1 (2.14 g, 10 mmol) and malonic acid (1.22 g, 12 mmol) in pyridine (30 mL) was stirred at room temperature for 30 min, then 0.2 mL of piperidine were added dropwise, followed by stirring at 95 °C for 4 hCitation10. The reaction was monitored by TLC. After the reaction was completed, the mixture was poured into dilute acid and extracted with ethyl acetate. The organic phase was collected, dried, and evaporated under reduced pressure to afford the crude product. After purifying by column chromatography on silica gel (ethyl acetate/petroleum ether = 1:3), compound 2 was obtained as yellow solid. Yield: 79%. 1H NMR (600 MHz, CDCl3): δ 7.72–7.69 (d, J = 18.0 Hz, 1H), 6.05–6.03 (d, J = 12.0 Hz, 1H), 4.52 (s, 2H), 4.45 (s, 2H), and 4.17 (s, 5H).
4-Ferrocenyl-7-methylchroman-2-one (3a)
A mixture of 2 (0.26 g, 1.0 mmol) and m-cresol (0.13 g, 1.2 mmol) in TFA (3 mL) was stirred at room temperature for 2 hCitation11. After complete consumption of starting material (TLC monitoring), the reaction mixture was diluted with 20 mL CH2Cl2. The organic layer was washed with water (40 mL) twice, dried over Na2SO4, and concentrated under reduced pressure. The residue was purified via column chromatography on SiO2 to afford 3a. Pale yellow solid, yield: 63%, Mp: 138.5–140.6 °C. 1H NMR (600 MHz, CDCl3): δ 7.02 (d, J = 7.8 Hz, 1H), 6.90 (d, J = 7.2 Hz, 1H), 6.87 (s, 1H), 4.15 (s, 7H), 4.05 (s, 1H), 4.03 (s, 1H), 3.99 (t, J = 6 Hz, 1H), 3.11 (dd, J1 =J2= 5.4 Hz, 1H), 3.01 (dd, J1=J2=6 Hz, 1H), 2.33 (s, 3H). 13CNMR (151 MHz, CDCl3): δ 168.6 (s), 152.3 (s), 150.1 (s), 127.3 (s), 124.2 (s), 123.4 (s), 121.2 (s), 114.0 (s), 89.2 (s), 68.7 (s), 67.9 (s), 65.3 (s), 36.0 (s), 34.7 (s), and 31.2 (s). MS (ESI): 346.1 (C20H18FeO2, [M + H+]).
4-Ferrocenyl-7-ethylchroman-2-one (3b)
A mixture of 2 (0.26 g, 1.0 mmol) and 3-ethylphenol (0.15 g, 1.2 mmol) in TFA (3 mL) was stirred at room temperature for 2 h. After complete consumption of starting material (TLC monitoring), the reaction mixture was diluted with 20 mL CH2Cl2. The organic layer was washed with water (40 mL) twice, dried over Na2SO4, and concentrated under reduced pressure. The residue was purified via column chromatography on SiO2 to afford 3b. Oil, yield: 73%. 1H NMR (600 MHz, CDCl3): δ 7.04 (d, J = 7.8 Hz, 1H), 6.92 (d, J = 7.8 Hz, 1H), 6.90 (s, 1H), 4.16 (s, 7H), 4.05 (d, J = 17.4 Hz, 2H), 4.00 (t, J = 6 Hz, 1H), 3.12 (dd, J1 = 6 Hz, J2 5.4 Hz, 1H), 3.02 (dd, J1 = J2=6 Hz, 1H), 2.63 (q, J = 7.8 Hz, 2H), 1.22 (t, J = 7.2 Hz, 3H). 13CNMR (151 MHz, CDCl3): δ 168.6 (s), 150.9 (s), 145.1 (s), 127.6 (s), 123.9 (s), 116.2 (s), 89.2 (s), 68.7 (s), 68.2 (s), 67.9 (s), 65.3 (s), 36.1 (s), 34.7 (s), 28.3 (s), and 15.3 (s). MS (ESI): 361.2 (C21H20FeO2, [M + H]+).
4-Cyclohexyl-6-pentylchroman-2-one (3c)
A mixture of 2 (0.26 g, 1.0 mmol) and 4-pentylphenol (0.20 g, 1.2 mmol) in TFA (3 mL) was stirred at room temperature for 2 h. After complete consumption of starting material (TLC monitoring), the reaction mixture was diluted with 20 mL CH2Cl2. The organic layer was washed with water (40 mL) twice, dried over Na2SO4, and concentrated under reduced pressure. The residue was purified via column chromatography on SiO2 to afford 3c. Oil, yield: 65%. 1H NMR (600 MHz, CDCl3): δ 7.04 (d, J = 7.8 Hz, 1H), 6.96 (d, J = 8.4 Hz, 1H), 6.75 (s, 1H), 4.22 (s, 7H), 4.14 (s, 2H), 3.97 (s, 1H), 3.09 (d, J = 13.8 Hz, 1H), 3.00 (d, J = 15 Hz, 1H), 2.54 (t, J = 7.2 Hz, 2H), 1.31 (m, 6H), 0.89 (t, J = 7.8 Hz, 3H). 13C NMR (151 MHz, CDCl3): δ 168.6 (s), 153.6 (s), 148.9 (s), 139.0 (s), 135.0 (s), 129.3 (s), 128.2 (s), 116.6 (s), 114.9 (s), 69.3 (s), 68.7 (s), 68.2 (s), 66.1 (s), 36.0 (s), 35.1 (s), 31.3 (s), 22.4 (s), and 13.9 (s). MS (ESI): 402.1 (C24H26FeO2, [M + H]+).
6-(Tert-butyl)-4-cyclohexylchroman-2-one (3d)
A mixture of 2 (0.26 g, 1.0 mmol) and 4-tert-butylphenol (0.18 g, 1.2 mmol) in TFA (3 mL) was stirred at room temperature for 2 h. After complete consumption of starting material (TLC monitoring), the reaction mixture was diluted with 20 mL CH2Cl2. The organic layer was washed with water (40 mL) twice, dried over Na2SO4, and concentrated under reduced pressure. The residue was purified via column chromatography on SiO2 to afford 3d. Pale yellow solid, yield: 81%, Mp: 187.5–188.4 °C. 1H NMR (600 MHz, CDCl3): δ 7.29 (dd, J1=1.8 Hz, J2 =8.4 Hz, 1H), 7.21 (d, J = 1.8 Hz, 1H), 7.00 (d, J = 8.4 Hz, 1H), 4.14 (m, 7H), 4.05 (s, 1H), 4.02 (d, J= 5.4 Hz, 1H), 4.00 (s, 1H), 3.09 (dd, J1 = J2 = 6 Hz, 1H), 3.02 (dd, J1= 5.4 Hz, J2= 4.8 Hz, 1H), and 1.31 (s, 9H). 13C NMR (151 MHz, CDCl3): δ 168.5 (s), 148.8 (s), 147.4 (s), 125.5 (s), 124.9 (s), 116.4 (s), 89.4 (s), 68.7 (s), 68.2 (s), 68.1 (s), 67.8 (s), 67.5 (s), 65. 6 (s), 36.4 (s), 35.4 (s), 34.4 (s), and 31.4 (s). MS (ESI): 388.3 (C23H24FeO2, [M + H]+).
7-(Tert-butyl)-4-cyclohexylchroman-2-one (3e)
A mixture of 2 (0.26 g, 1.0 mmol) and 3-tert-butylphenol (0.18 g, 1.2 mmol) in TFA (3 mL) was stirred at room temperature for 2 h. After complete consumption of starting material (TLC monitoring), the reaction mixture was diluted with 20 mL CH2Cl2. The organic layer was washed with water (40 mL) twice, dried over Na2SO4, and concentrated under reduced pressure. The residue was purified via column chromatography on SiO2 to afford 3e. Pale yellow solid, yield: 77%, Mp: 191.2–192.6 °C. 1H NMR (600 MHz, CDCl3): δ 7.10 (s, 1H), 7.07 (s, 2H), 4.16 (s, 7H), 4.09 (s, 1H), 4.04 (s, 1H), 4.00 (t, J = 6 Hz, 1H), 3.14 (dd, J1 = J2 = 6 Hz, 1H), 3.03 (dd, J1=J2=6.6 Hz, 1H), and 1.29 (s, 9H). 13C NMR (151 MHz, CDCl3): δ 168.4 (s), 147.2 (s), 145.4 (s), 144.2 (s), 124.2 (s), 118.9 (s), 106.9 (s), 101.6 (s), 99.0 (s), 89.0 (s), 68.7 (s), 67.9 (s), 65.2 (s), 35.8 (s), and 34.9 (s). MS (ESI): 388.3 (C23H24FeO2, [M + H]+).
4-Ferrocenyl-6-(2,4,4-trimethylpentan-2-yl)chroman-2-one (3f)
A mixture of 2 (0.26 g, 1.0 mmol) and 4-tert-octylphenol (0.25 g, 1.2 mmol) in TFA (3 mL) was stirred at room temperature for 2 h. After complete consumption of starting material (TLC monitoring), the reaction mixture was diluted with 20 mL CH2Cl2. The organic layer was washed with water (40 mL) twice, dried over Na2SO4, and concentrated under reduced pressure. The residue was purified via column chromatography on SiO2 to afford 3f. Oil, yield: 74%. 1H NMR (600 MHz, CDCl3): δ 7.26 (s, 1H), 7.16 (s, 1H), 6.97 (d, J = 8.4 Hz, 1H), 4.13 (m, 7H), 4.02 (m, 3H), 3.09 (dd, J1 = 6 Hz, J2 = 5.4 Hz, 1H), 3.03 (dd, J1 = 4.2 Hz, J2=4.8 Hz, 1H), 1.71 (s, 2H), 1.34 (s, 6H), and 0.72 (s, 9H). 13C NMR (151 MHz, CDCl3): δ 168.6 (s), 148.6 (s), 146.4 (s), 126.1 (s), 125.6 (s), 125.1 (s), 116.1 (s), 89.3 (s), 68.7 (s), 67.7 (s), 65.4 (s), 56.8 (s), 38.3 (s), 36.1 (s), 35.3 (s), 32.3 (s), 31.7 (s), and 31.5 (s). MS (ESI): 444.4 (C27H32FeO2, [M + H]+).
6-Cyclohexyl-4-ferrocenylchroman-2-one (3g)
A mixture of 2 (0.26 g, 1.0 mmol) and 4-cyclohexylphenol (0.21 g, 1.2 mmol) in TFA (3 mL) was stirred at room temperature for 2 h. After complete consumption of starting material (TLC monitoring), the reaction mixture was diluted with 20 mL CH2Cl2. The organic layer was washed with water (40 mL) twice, dried over Na2SO4, and concentrated under reduced pressure. The residue was purified via column chromatography on SiO2 to afford 3g. Pale yellow solid, yield: 65%, Mp: 146.0–147.0 °C. 1H NMR (600 MHz, CDCl3): δ 7.09 (d, J = 8.4 Hz, 1H), 7.00 (s, 1H), 6.98 (d, J = 7.8 Hz, 1H), 4.17 (s, 7H), 4.08 (d, J = 14.4 Hz, 2H), 3.98 (t, J = 6 Hz, 1H), 3.09 (dd, J1 = 5.4 Hz, J2 = 4.8 Hz, 1H), 3.00 (dd, J1 = 5.4 Hz, J2 = 4.8 Hz, 1H), 2.46 (m, 1H), 1.84 (m, 4H), 1.37 (m, 4H), 1.26 (m, 2H). 13C NMR (151 MHz, CDCl3): δ 168.5 (s), 149.1 (s), 144.3 (s), 126.3 (s), 125.9 (s), 116.7 (s), 69.0 (s), 68.4 (s), 68.0 (s), 67.9 (s), 65.8 (s), 43.9 (s), 36.3 (s), 35.3 (s), 34.6 (s), 26.7 (s), and 26.0 (s). MS (ESI): 414.3 (C25H26FeO2, [M + H]+).
4-Ferrocenyl-3,4-dihydro-2H-benzo[g]chromen-2-one (3h)
A mixture of 2 (0.26 g, 1.0 mmol) and 2-naphthol (0.17 g, 1.2 mmol) in TFA (3 mL) was stirred at room temperature for 2 h. After complete consumption of starting material (TLC monitoring), the reaction mixture was diluted with 20 mL CH2Cl2. The organic layer was washed with water (40 mL) twice, dried over Na2SO4, and concentrated under reduced pressure. The residue was purified via column chromatography on SiO2 to afford 3h. Yellow needle-like solid, yield: 70%, Mp: 159.0–161.0 °C. 1H NMR (600 MHz, CDCl3): δ 8.09 (d, J = 8.4 Hz, 1H), 7.86 (d, J = 7.8 Hz, 1H), 7.78 (d, J = 8.4 Hz, 1H), 7.62 (t, J = 8.4 Hz, 1H), 7.48 (t, J = 7.8 Hz, 1H), 7.27 (d, J = 8.4 Hz, 1H), 4.67 (d, J = 6.6 Hz, 1H), 4.14 (s, 7H), 4.04 (s, 1H), 3.41 (d, J = 16.2 Hz, 1H), and 3.08 (dd, J1 = J2 = 6.6 Hz, 1H). 13C NMR (151 MHz, CDCl3): δ 168.5 (s), 148.5 (s), 131.1 (s), 130.7 (s), 129.3 (s), 128.8 (s), 127.0 (s), 125.0 (s), 123.3 (s), 119.7 (s), 117.7 (s), 90.0 (s), 68.9 (s), 67.8 (s), 66.9 (s), 35.3 (s), and 30.9 (s). MS (ESI): 383.1 (C23H18FeO2, [M + H]+).
4-Ferrocenyl-3,4-dihydro-2H-benzo[h]chromen-2-one (3i)
A mixture of 2 (0.26 g, 1.0 mmol) and 1-naphthol (0.17 g, 1.2 mmol) in TFA (3 mL) was stirred at room temperature for 2 h. After complete consumption of starting material (TLC monitoring), the reaction mixture was diluted with 20 mL CH2Cl2. The organic layer was washed with water (40 mL) twice, dried over Na2SO4, and concentrated under reduced pressure. The residue was purified via column chromatography on SiO2 to afford 3i. Yellow needle-like solid, yield: 68%, Mp: 162.0–164.0 °C. 1H NMR (600 MHz, CDCl3): δ 8.27 (d, J = 8.4 Hz, 1H), 7.82 (d, J = 7.8 Hz, 1H), 7.60 (d, J = 7.8 Hz, 1H), 7.54 (m, 2H), 7.29 (d, J = 8.4 Hz, 1H), 4.16 (m, 7H), 4.08 (m, 2H), 3.23 (dd, J1 = J2 = 6 Hz, 1H), and 3.17 (dd, J1= 4.8 Hz, J2 = 5.4 Hz, 1H). 13C NMR (151 MHz, CDCl3): δ 170.8 (s), 136.2 (s), 130.2 (s), 129.3 (s), 127.6 (s), 126.9 (s), 126.6 (s), 126.3 (s), 123.9 (s), 123.5 (s), 92.0 (s), 71.4 (s), 71.0 (s), 70.5 (s), 68.2 (s), 38.9 (s), and 38.1 (s). MS (ESI): 383.1 (C23H18FeO2, [M + H]+).
8-Ferrocenyl-7,8-dihydro-6H-[1,3]dioxolo[4,5-g]chromen-6-one (3j)
A mixture of 2 (0.26 g, 1.0 mmol) and 1,3-benzodioxol-5-ol (0.17 g, 1.2 mmol) in TFA (3 mL) was stirred at room temperature for 2 h. After complete consumption of starting material (TLC monitoring), the reaction mixture was diluted with 20 mL CH2Cl2. The organic layer was washed with water (40 mL) twice, dried over Na2SO4, and concentrated under reduced pressure. The residue was purified via column chromatography on SiO2 to afford 3j. Yellow needle-like solid, yield: 81%, Mp: 162.7–168.1 °C. 1H NMR (600 MHz, CDCl3): δ 6.58 (s, 1H), 6.55 (s, 1H), 5.94 (s, 1H), 5.92 (s, 1H), 4.16 (s, 7H), 4.07 (s, 1H), 4.02 (s, 1H), 3.91 (t, J = 6 Hz, 1H), 3.11 (dd, J1= 5.4 Hz, J2 = 6 Hz, 1H), and 2.99 (dd, J1 = 6.6 Hz, J2 = 6 Hz, 1H). 13C NMR (151 MHz, CDCl3): δ 168.6 (s), 150.8 (s), 138.7 (s), 127.5 (s), 125.1 (s), 124.2 (s), 123.4 (s), 117.3 (s), 89.2 (s), 66.7 (s), 68.2 (s), 67.8 (s), 65.4 (s), 36.2 (s), 34.7 (s), and 21.0 (s). MS (ESI):376.2 (C20H16FeO4, [M + Na]+).
6,8-Dimethyl-4-ferrocenylchroman-2-one (3k)
A mixture of 2 (0.26 g, 1.0 mmol) and 2,4-dimethylphenol (0.15 g, 1.2 mmol) in TFA (3 mL) was stirred at room temperature for 2 h. After complete consumption of starting material (TLC monitoring), the reaction mixture was diluted with 20 mL CH2Cl2. The organic layer was washed with water (40 mL) twice, dried over Na2SO4, and concentrated under reduced pressure. The residue was purified via column chromatography on SiO2 to afford 3k. Yellow needle-like solid, Yield: 83%, Mp: 155.0–157.0 °C. 1H NMR (600 MHz, CDCl3): δ 6.91 (s, 1H), 6.76 (s, 1H), 4.15 (s, 7H), 4.08 (s, 1H), 4.05 (s, 1H), 3.96 (t, J = 6 Hz, 1H), 3.10 (dd, J1 = 6 Hz, J2 = 5.4 Hz, 1H), 3.02 (dd, J1 = J2 = 6 Hz, 1H), 2.27 (s, 3H), and 2.26 (s, 3H). 13C NMR (151 MHz, CDCl3): δ 168.7 (s), 147.0 (s), 133.3 (s), 130.5 (s), 125.9 (s), 125.6 (s), 89.2 (s), 68.7 (s), 68.1 (s), 67.9 (s), 65.4 (s), 36.0 (s), 35.2 (s), 20.7 (s), and 15.6 (s). MS (ESI): 399.0 (C21H20FeO2, [M + K]+).
7,8-Dimethyl-4-ferrocenylchroman-2-one (3l)
A mixture of 2 (0.26 g, 1.0 mmol) and 2,3-dimethylphenol (0.15 g, 1.2 mmol) in TFA (3 mL) was stirred at room temperature for 2 h. After complete consumption of starting material (TLC monitoring), the reaction mixture was diluted with 20 mL CH2Cl2. The organic layer was washed with water (40 mL) twice, dried over Na2SO4, and concentrated under reduced pressure. The residue was purified via column chromatography on SiO2 to afford 3l. Yellow solid, yield: 73%, Mp: 151.0–153.0 °C. 1H NMR (600 MHz, CDCl3): δ 6.89 (d, J = 7.2 Hz, 1H), 6.85 (d, J = 7.2 Hz, 1H), 4.20 (m, 7H), 4.11 (s, 2H), 3.97 (t, J = 6 Hz, 1H), 3.08 (dd, J1 = 4.8 Hz, J2 = 4.2 Hz, 1H), 3.00 (dd, J1 = J2 = 4.2 Hz, 1H), 2.27 (s, 3H), and 2.22 (s, 3H). 13C NMR (151 MHz, CDCl3): δ 168.9 (s), 153.6 (s), 148.9 (s), 137.3 (s), 125.9 (s), 124.7 (s), 124.4 (s), 123.8 (s), 122.2 (s), 112.5 (s), 89.6 (s), 69.0 (s), 68.4 (s), 65.7 (s), 35.9 (s), 35.1 (s), 19.8 (s), and 11.7 (s). MS (ESI): 362.3 (C21H20FeO2, [M + H]+).
6,7-Dimethyl-4-ferrocenylchroman-2-one (3m)
A mixture of 2 (0.26 g, 1.0 mmol) and 3,4-dimethylphenol (0.15 g, 1.2 mmol) in TFA (3 mL) was stirred at room temperature for 2 h. After complete consumption of starting material (TLC monitoring), the reaction mixture was diluted with 20 mL CH2Cl2. The organic layer was washed with water (40 mL) twice, dried over Na2SO4, and concentrated under reduced pressure. The residue was purified via column chromatography on SiO2 to afford 3m. Yellow needle solid, yield: 76%, Mp: 157.0–159.0 °C. 1H NMR (600 MHz, CDCl3): δ 6.88 (s, 1H), 6.83 (s, 1H), 4.15 (s, 7H), 4.07 (s, 1H), 4.03 (s, 1H), 3.96 (t, J = 6 Hz, 1H), 3.10 (dd, J1 = J2 = 6 Hz, 1H), 3.01 (dd, J1 = J2 = 6 Hz, 1H), 2.22 (s, 3H), and 2.20 (s, 3H). 13C NMR (151 MHz, CDCl3): δ 168.7 (s), 148.8 (s), 137.0 (s), 132.5 (s), 128.6 (s), 128.5 (s), 123.3 (s), 117.7 (s), 89.4 (s), 68.7 (s), 65.3 (s), 36.2 (s), 34.7 (s), 19.5 (s), and 19.1 (s). MS (ESI): 383.1 (C21H20FeO2, [M + Na]+).
5,7-Dimethyl-4-ferrocenylchroman-2-one (3n)
A mixture of 2 (0.26 g, 1.0 mmol) and 3,5-dimethylphenol (0.15 g, 1.2 mmol) in TFA (3 mL) was stirred at room temperature for 2 h. After complete consumption of starting material (TLC monitoring), the reaction mixture was diluted with 20 mL CH2Cl2. The organic layer was washed with water (40 mL) twice, dried over Na2SO4, and concentrated under reduced pressure. The residue was purified via column chromatography on SiO2 to afford 3n. Yellow needle solid, yield: 66%, Mp: 116.6–120.0 °C. 1H NMR (600 MHz, CDCl3): δ 6.78 (s, 1H), 6.73 (s, 1H), 4.15 (s, 5H), 4.11 (d, J = 7.8 Hz, 2H), 4.08 (s, 1H), 4.04 (d, J = 6.6 Hz 1H), 4.00 (s, 1H), 3.32 (d, J = 16.2 Hz, 1H), 2.94 (dd, J1 = J2 = 6.6 Hz, 1H), 2.38 (s, 3H), 2.28 (s, 3H). 13C NMR (151 MHz, CDCl3): δ 169.1 (s), 151.0 (s), 138.1 (s), 135.5 (s), 127.3 (s), 122.1 (s), 115.5 (s), 90.4 (s), 68.9 (s), 67.9 (s), 67.4 (s), 65.8 (s), 36.1 (s), 31.4 (s), 20.9 (s), and 18.9 (s). MS (ESI): 383.1 (C21H20FeO2, [M + Na]+).
5,7,8-Trimethyl-4-ferrocenylchroman-2-one (3o)
A mixture of 2 (0.26 g, 1.0 mmol) and 2,3,5-dimethylphenol (0.16 g, 1.2 mmol) in TFA (3 mL) was stirred at room temperature for 2 h. After complete consumption of starting material (TLC monitoring), the reaction mixture was diluted with 20 mL CH2Cl2. The organic layer was washed with water (40 mL) twice, dried over Na2SO4, and concentrated under reduced pressure. The residue was purified via column chromatography on SiO2 to afford 3o. Yellow needle-like solid, Yield: 77%, Mp: 188.6–191.6 °C. 1H NMR (600 MHz, CDCl3): δ 6.79 (s, 1H), 4.19 (m, 7H), 4.10 (s, 3H), 3.29 (d, J = 7.2 Hz, 1H), 2.90 (dd, J1 = J2 =6 Hz, 1H), 2.38 (s, 3H), 2.19 (s, 3H), 2.12 (s, 3H). 13C NMR (151 MHz, CDCl3): δ 169.4 (s), 153.4 (s), 149.0 (s), 137.9 (s), 136.7 (s), 131.8 (s), 127.5 (s), 123.1 (s), 119.1 (s), 113.3 (s), 69.1 (s), 68.0 (s), 66.1 (s), 35.8 (s), 20.8 (s), 18.7 (s), and 11.5 (s). MS (ESI): 374.3 (C22H22FeO2, [M + H]+).
6-Isopropyl-7-methyl-4-ferrocenylchroman-2-one (3p)
A mixture of 2 (0.26 g, 1.0 mmol) and 3-methyl-4-propan-2-ylphenol (0.18 g, 1.2 mmol) in TFA (3 mL) was stirred at room temperature for 2 h. After complete consumption of starting material (TLC monitoring), the reaction mixture was diluted with 20 mL CH2Cl2. The organic layer was washed with water (40 mL) twice, dried over Na2SO4, and concentrated under reduced pressure. The residue was purified via column chromatography on SiO2 to afford 3p. Oil, yield: 83%. 1H NMR (600 MHz, CDCl3): δ 7.01 (s, 1H), 6.82 (s, 1H), 4.24 (m, 7H), 4.17 (s, 1H), 4.14 (s, 1H), 3.95 (s, 1H), 3.09 (m, 1H), 3.04 (d, J = 15 Hz, 1H), 2.96 (d, J = 15 Hz, 1H), 2.31 (s, 3H), and 1.20 (d, J = 6.6 Hz, 6H). 13C NMR (151 MHz, CDCl3): δ 168.5 (s), 148.4 (s), 142.9 (s), 135.6 (s), 124.2 (s), 123.3 (s), 118.0 (s), 107.2 (s), 69.5 (s), 36.5 (s), 35.1 (s), 28.7 (s), 23.3 (s), 23.2 (s), and 18.9 (s). MS (ESI):411.3 (C23H24FeO2, [M + Na]+).
8-(Tert-butyl)-6-methyl-4-ferrocenylchroman-2-one (3q)
A mixture of 2 (0.26 g, 1.0 mmol) and 2-tert-butyl-4-methylphenol (0.20 g, 1.2 mmol) in TFA (3 mL) was stirred at room temperature for 2 h. After complete consumption of starting material (TLC monitoring), the reaction mixture was diluted with 20 mL CH2Cl2. The organic layer was washed with water (40 mL) twice, dried over Na2SO4, and concentrated under reduced pressure. The residue was purified via column chromatography on SiO2 to afford 3q. Oil, yield: 86%. 1H NMR (600 MHz, CDCl3): δ 7.07 (s, 1H), 6.79 (s, 1H), 4.18 (s, 7H), 4.09 (d, J = 11.4 Hz, 2H), 3.97 (t, J = 6 Hz, 1H), 3.11 (dd, J1 = J2 =5.4 Hz, 1H), 3.00 (dd, J1 = J2 =6 Hz, 1H), 2.28 (s, 3H), and 1.42 (s, 9H). 13C NMR (151 MHz, CDCl3): δ 168.7 (s), 151.9 (s), 147.4 (s), 137.6 (s), 133.1 (s), 129.3 (s), 116.3 (s), 68.8 (s), 68.3 (s), 68.1 (s), 67.8 (s), 65.6 (s), 35.8 (s), 29.5 (s), and 21.1 (s). MS (ESI): 402.3 (C24H26FeO2, [M + H]+).
8-(Tert-butyl)-6-methyl-4-ferrocenylchroman-2-one (3r)
A mixture of 2 (0.26 g, 1.0 mmol) and 2-tert-butyl-4-ethylphenol (0.21 g, 1.2 mmol) in TFA (3 mL) was stirred at room temperature for 2 h. After complete consumption of starting material (TLC monitoring), the reaction mixture was diluted with 20 mL CH2Cl2. The organic layer was washed with water (40 mL) twice, dried over Na2SO4, and concentrated under reduced pressure. The residue was purified via column chromatography on SiO2 to afford 3r. Oil, yield: 76%. 1H NMR (600 MHz, CDCl3): δ 7.10 (s, 1H), 6.84 (s, 1H), 4.18 (s, 7H), 4.10 (d, J = 9 Hz, 2H), 3.98 (t, J = 5.4 Hz, 1H), 3.10 (dd, J1 = J2 =5.4 Hz, 1H), 3.00 (dd, J1 = J2 =5.4 Hz, 1H), 2.61 (q, J = 7.8 Hz, 2H), 1.42 (s, 9H), 1.22 (t, J = 9.6 Hz, 3H). 13C NMR (151 MHz, CDCl3): δ 168.7 (s), 152.1 (s), 147.6 (s), 139.5 (s), 137.7 (s), 135.8 (s), 125.8 (s), 116.3 (s), 68.9 (s), 68.3 (s), 67.9 (s), 65.8 (s), 35.9 (s), 34.7 (s), 29.5 (s), 28.5 (s), and 15.9 (s). MS (ESI): 416.1 (C25H28FeO2, [M + H]+).
6,8-Di-tert-butyl-4-ferrocenylchroman-2-one (3s)
A mixture of 2 (0.26 g, 1.0 mmol) and 2,4-di-tert-butylphenol (0.25 g, 1.2 mmol) in TFA (3 mL) was stirred at room temperature for 2 h. After complete consumption of starting material (TLC monitoring), the reaction mixture was diluted with 20 mL CH2Cl2. The organic layer was washed with water (40 mL) twice, dried over Na2SO4, and concentrated under reduced pressure. The residue was purified via column chromatography on SiO2 to afford 3s. Oil, yield: 81%. 1H NMR (600 MHz, CDCl3): δ 7.31 (s, 1H), 7.07 (s, 1H), 4.16 (s, 7H), 4.10 (s, 1H), 4.03 (s, 1H), 3.09 (dd, J1 = J2 =5.4 Hz, 1H), 3.00 (dd, J1 = 4.8 Hz, J2 = 4.2 Hz, 1H), 1.43 (s, 9H), 1.32 (s, 9H). 13C NMR (151 MHz, CDCl3): δ 168.7 (s), 151.8 (s), 146.3 (s), 137.2 (s), 135.1 (s), 126.2 (s), 123.4 (s), 122.9 (s), 115.8 (s), 68.8 (s), 68.2 (s), 67.8 (s), 65.8 (s), 36.2 (s), 35.0 (s), 31.4 (s), and 30.6 (s). MS (ESI): 444.2 (C27H32FeO2, [M + H]+).
In vitro anti-inflammatory activity
Cell culture
RAW 264.7 cells were obtained from the Cell Bank of the Chinese Academy of Sciences (Shanghai, China). The cells were grown in Dulbecco’s modified Eagle’s medium containing 10% (v/v) foetal calf serum (Hyclone, Logan, UT), 100 U/mL penicillin, and 100 mg/mL streptomycin and incubated in a humidified atmosphere of 5% CO2 and 95% air at 37 °C.
Assay for NO production
RAW 264.7 cells were seeded at a density of 7 × 104 cells per well in 24-well plate with 500 μL of culture medium and incubated for 24 h. The cells were then pretreated with compound 3h for 1 h before stimulation with LPS (1 μg/mL) for 24 h. NO synthesis was spectrophotometrically determined by assaying the culture supernatants for nitrite using the Griess reagent (Beyotime Biotechnology, Haimen, China). Absorbance was measured at 540 nm and NO concentration was determined using sodium nitrite as a standard.
Cytokine analysis
RAW 264.7 cells were pretreated with compound 3h at concentration of 2.5, 5, and 10 μM for 1 h, then incubated with LPS (0.5 μg/mL) for 24 h. The levels of TNF-α and IL-6 in serum were analysed using ELISA kits (Beyotime, Haimen, China) according to the manufacturer’s instruction. The absorbance was measured with the microplate reader at 450 nm.
Cell viability assay (MTT)
RAW 264.7 cells were seeded at a density of 1 × 103 cells per well in 96-well plate for 24 h before treatment. Following treatment with LPS and different concentrations of compound 3h, cell viability was determined using the MTT assay. Briefly, 10 μL (5 mg/mL) MTT working solution was added to each well and after incubation at 37 °C for 4 h. Then the MTT solution was removed and 200 μL of dimethyl sulphoxide (DMSO) was added to dissolve the crystals. The absorbance of each well at 570 nm was measured using a microplate reader (MQX200, Bio-Tek, Winooski, VT).
Western blotting
RAW 264.7 cells were cultured for 24 h and then treated with different concentrations of compound 3h for 1 h before exposure to LPS. The cells were lysed in 280 μL RIPA cell lysis buffer (Beyotime, Haimen, China) and incubated on ice for 30 min. For Western blotting, proteins were resolved by SDS-PAGE then transferred to PVDF membrane (GE Healthcare, Amersham, UK). The blotted membrane was incubated with specific primary antibody for overnight at 4 °C and further incubated for 1 h with HRP-conjugated secondary antibody.
In vivo anti-inflammatory activity
Animals
Healthy male Sprague–Dawley (SD) rats weighing 160–180 g were purchased from Experimental Animal Center of Anhui Medical University (Hefei, China). The animals were cultured under standardised conditions with the humidity of 50–55%, temperature of 23 °C ± 2 °C and have free access to clean food and water. All animal protocols were approved by the Ethics Committee in Animal Experimentation at Hefei University of Technology (Hefei, China) following the guidelines for Care and Use of Laboratory Animals.
Establishment of AIA rat
The anti-inflammatory effect of compound 3h was studied on the complete Freund’s adjuvant (CFA)-induced arthritis (AIA) rat modelCitation12. Rat AIA was induced by a single intradermal injection of 0.1 mL CFA (10 mg/mL, Sigma, St. Louis, MO) into the right hind paw. Paw swelling and body weight were measured every three days after primary immunisation with CFA to evaluate arthritis.
Treatment regimen
Twenty five rats were randomly divided into five groups (five rats per group): (i) the rats received single intradermal injection of 0.1 mL saline (normal), (ii) the AIA rats without treatment (AIA), (iii) the AIA rats received intragastrical administration of aspirin at the dose of 40 mg/kg (aspirin, 40 mg/kg), (iv) the AIA rats received intragastrical administration of compound 3h at the dose of 40 mg/kg (compound 3h, 40 mg/kg), and (v) the AIA rats received intragastrical administration of compound 3h at the dose of 80 mg/kg (compound 3h, 80 mg/kg).
Histopathological examination of joints
The rats were sacrificed by CO2 asphyxiation at the end of the experiment. The knee joints were immediately removed, trimmed, fixed in 4% neutral buffered paraformaldehyde solution and then decalcified in 4% HNO3 for two days. After decalcification, the tissues were dehydrated in a gradient ethanol series, embedded in paraffin and sectioned at 4 μM thickness. The tissue sections were stained with haematoxylin and eosin (HE) for histopathological examination. The histological images were taken using an Olympus BX51 microscope system (Olympus Corporation, Tokyo, Japan).
Statistical analysis
Statistical analysis was performed using a one-way ANOVA where p value less than .05 was considered statistically significant. Data were expressed as mean ± SEM and analysed using GraphPad Prism version 5.0 (GraphPad Software, Inc., La Jolla, CA). One-way ANOVA with Tukey’s multiple comparison test was used to compare the means of all experimental groups.
Results and discussion
Chemistry
The synthetic route of 4-ferrocenyl-3,4-dihydrocoumatin derivatives (3a–3s) is shown in Scheme 1. The target compounds were prepared in two steps. First, the commercially available ferrocenecarboxaldehyde (1) was reacted with malonic acid in the Knoevenagel reaction to obtain the intermediate (2). Then, the intermediate 2 was reacted with substituted aromatic phenolic compounds including phenols and naphthols to afford the target compounds (3a–3s) in good yields. The structures of compounds 3a–3s are shown in . All compounds were purified by recrystallisation or column chromatography and characterised by 1H NMR, 13C NMR, and MS.
Table 1. Chemical structures of compounds 3a–3s.
Inhibitory activity against LPS-induced NO release
Nitric oxide (NO) is produced by mammalian cells through metabolism of l-arginine and plays an important role in regulating inflammation and immune responsesCitation13. NO is a key pro-inflammatory mediator and excessive production of NO was proved to be associated with many inflammatory diseasesCitation12,Citation14. Thus, it is an established view that compounds inhibiting the production of NO may offer potential opportunity to treat inflammatory diseases. To evaluate the anti-inflammatory activity of these compounds, RAW 264.7 cell was used as a model cell. RAW 264.7 cells were pre-incubated with all target compounds (20 µM) for 1 h and treated with LPS (1.0 µg/mL) for 24 h. The cell conditioned medium was collected and the NO content in the media was detected by the Griess reagent assay.
The screening results indicated that most of the target compounds could significantly reduce the LPS-induced NO secretion at a dosage of 20 µM (). The inhibitory rates (IRs) of all the target compounds were higher than 85%. In addition, the IR of compounds 3b, 3d, 3h, 3i, 3j, 3l, 3o, 3q, 3r, and 3s were higher than 90%. Among them, compounds 3h, 3i, and 3j showed stronger inhibition of NO compared to the positive compound Celecoxib. It is obvious that compound 3h exhibited the most potent inhibitory activity against NO production (IR > 95%) compared with Celecoxib. According to the results, the structure–activity relationship (SAR) can be easily summarised that introduction of coplanar groups like naphthalenyl (3h and 3i) and benzodioxol (3j) bridging to the dihydrocoumarin ring is beneficial to the NO inhibitory activity.
Toxicity assessment
Preliminary screening results showed that most of the target compounds had good anti-inflammatory activity. To confirm the possibility for further research, the cytotoxicity of compound 3h was evaluated by the MTT assay in RAW264.7 cells. As shown in , compound 3h demonstrated low toxicity even at a high concentration of 40 µM, indicating that compound 3h is worthy of further evaluation.
Inhibition of the cytokines production by compound 3h
The pro-inflammatory cytokines including IL-6 and TNF-α are involved in the pathogenesis of RA. It has been reported that TNF-α and IL-6 participate in synovial proliferation and induced cartilage destructionCitation15. TNF-α was particularly proven to be a key pathogenic inflammatory cytokine of RACitation16. The autocrine production of TNF-α by RA bone erosion could promote the expression of other inflammatory mediators and induce immune cell infiltration to aggravate RA diseaseCitation17. In this study, RAW 264.7 cells were pretreated with compound 3h at a series of concentrations (2.5, 5, and 10 μM) for 1 h and then with LPS (1 µg/mL) for 24 h. The levels of TNF-α and IL-6 in cell culture were evaluated by ELISA. As shown in , compound 3h significantly reduced NO, IL-6, and TNF-α levels in cell culture in a concentration-dependent manner. Based on the results above, 3h was an effective anti-inflammatory compound. Thus, compound 3h was selected as the title compound for further mechanism exploration.
Figure 4. Inhibition of the cytokines production by compound 3h. RAW 264.7 cells were pretreated with compound 3h at concentration of 10, 5, and 2.5 μM for 1 h and treated with LPS (1 μg/mL) for 24 h. (A) NO production was measured using nitrite and nitrate assay. (B and C) TNF-α and IL-6 levels in the medium were determined with an ELISA kit. ###p<.001 compared with control group; **p<.01 and ***p<.001 compared with LPS treated group.
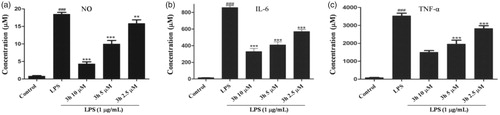
Mechanism exploration of anti-inflammatory activity
Compound 3h suppresses LPS-induced inflammatory response
NO is synthesised by nitric oxide synthase (NOS). NOS isoforms include neuronal nitric oxide synthase (nNOS), inducible nitric oxide synthase (iNOS), and endothelial nitric oxide synthase (eNOS)Citation18. Many inflammatory conditions are associated with production of large amounts of NO and iNOS. The findings have confirmed that iNOS inhibitors exert beneficial effects on osteoarthritisCitation19. Moreover, the cyclooxygenase-2 (COX-2) has been well-studied to play an important role in RA which is induced in inflammatory situations and highly expressed in synovial tissues of RA patientsCitation20,Citation21. Thus, the inhibitory effects of compound 3h on LPS-mediated expressions of iNOS and COX-2 were analysed by Western blotting. As shown in , the LPS (1 µg/mL) stimulation for 24 h could markedly augmented iNOS and COX-2 expression. Additionally, compound 3h could dose-dependently suppress LPS-induced iNOS and COX-2 expression. These results further demonstrated that compound 3h could prevent LPS-induced inflammatory response in macrophages.
Figure 5. Compound 3h inhibited the LPS-induced expression of iNOS and COX-2. RAW 264.7 cells were pretreated with compound 3h at concentration of 10, 5, and 2.5 μM for 1 h and treated with LPS (1 μg/mL) for 24 h. (A) Protein expression of iNOS and COX-2 was detected by Western blotting analysis. (B and C) Semi-quantitative statistical graph of iNOS and COX-2 protein expressions in various groups. Bay 11-7082 was positive control and β-actin was used as loading control. ###p<.001 compared with control group; ***p<.001 compared with LPS treated group.
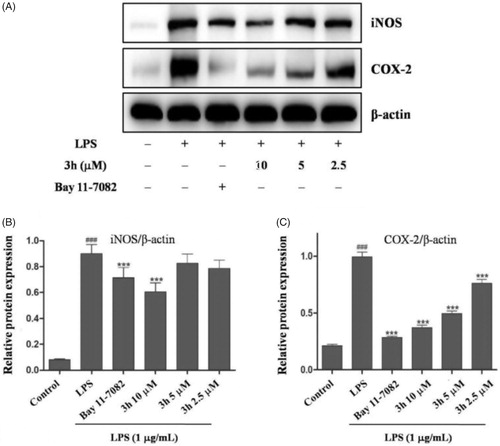
Inhibition of LPS-induced NF-κB signalling pathway activation
Nuclear factor-κB (NF-κB) is a principal transcription factor for amplifying expression of many cytokines that are involved in the pathogenesis of inflammatory diseasesCitation22. In patients with RA, activation of NF-κB signalling pathway results in production of inflammatory cytokines including TNF-α and IL-6Citation23. The NF-κB family includes NF-κB1, NF-κB2, P65, RelB, and c-Rel. Among NF-κB family, P65 plays the most important role in the development of inflammationCitation24. Many inflammation cytokines such as LPS, TNF-α, and IL-1β can stimulate the activation of IκB kinase (IKK). The activated IKK then phosphorylates IκB which regulates the nuclear translocation of NF-κBCitation25.
To explore the anti-inflammatory mechanism of compound 3h, we examined the effect of compound 3h on the markers of the NF-κB pathway. The Western blotting analyses demonstrated that the expression levels of p-IκB and p-P65 were suppressed in compound 3h group compared with untreated group, indicating that compound 3h could decrease the IκB phosphorylation level and degradation (). Meanwhile, it could also inhibit NF-κB P65 translocate into the nucleus.
Figure 6. Compound 3h inhibited LPS-induced activation of NF-κB signalling pathway in RAW 264.7 cells. RAW 264.7 cells were pretreated with compound 3h at concentration of 10, 5, and 2.5 μM for 1 h and treated with LPS (1 μg/mL) for 24 h. (A) Proteins expression were detected by Western blotting analysis. (B and C) Semi-quantitative statistical graph of p-IκB, IκB and p-P65 protein expressions in various groups. Bay 11-7082 was positive control and β-actin was used as loading control. ###p<.001 compared with control group; ***p<.001 compared with LPS treated group.
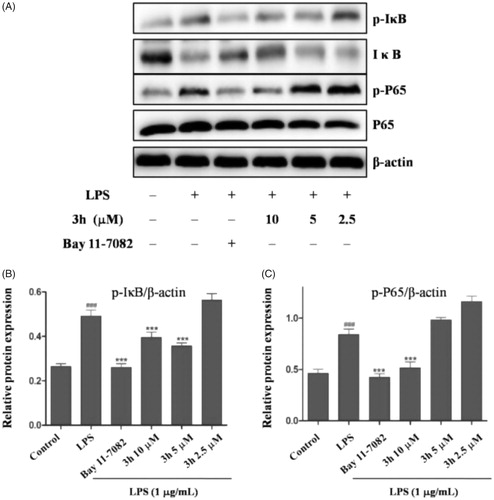
Compound 3h inhibits LPS-induced ERK signalling activation
Mitogen-activated protein kinases (MAPKs) are the key signalling pathway that plays an important role in RACitation26. To further investigate the anti-inflammatory mechanism of compound 3h on CFA-induced arthritis, the inflammation-related signalling pathway of MAPK was studied. As shown in , the expression levels of p-ERK, p-P38, and p-JNK were upregulated in rats after exposure to CFA. Treatment with compound 3h effectively downregulated the protein levels of p-ERK in a dose-dependent manner. The three major kinase cascades in MAPK signalling pathway include extracellular signal regulated kinase (ERK), C-Jun-terminal kinase (JNK), and P38 MARK. In the current study, compound 3h treatment caused significant inhibition of the phosphorylation of ERK. The results indicated that compound 3h exert its anti-inflammatory action by inhibiting the activation of ERK/MAPK pathway.
Figure 7. Compound 3h inhibited LPS-induced MAPKs signalling pathway. RAW 264.7 cells were pretreated with compound 3h at concentration of 10, 5, and 2.5 μM for 1 h and treated with LPS (1 μg/mL) for 24 h. (A) Proteins expression were detected by Western blotting analysis. (B, C and D) Semi-quantitative statistical graph of p-ERK, p-P38 and p-JNK protein expressions in various groups. ###p<.001 compared with control group; ***p<.001 compared with LPS treated group.
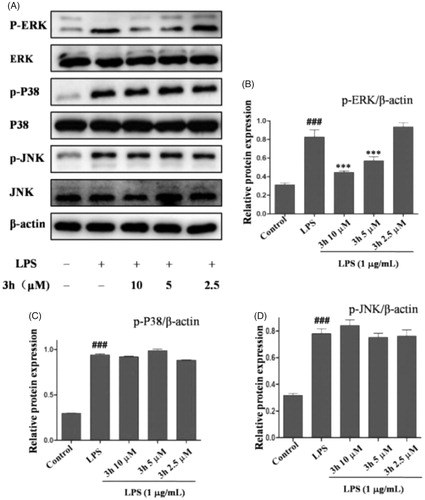
In vivo anti-inflammatory activity of compound 3h
The CFA-induced arthritis (AIA) is a widely used model to study RACitation27. In this study, we established the rat AIA model by intradermal injection of CFA into the right hind pawCitation14. We observed that 80–90% of rats exhibited obvious hind paw swelling and body weight loss within 14–28 days after CFA injection. AIA rats were given intragastric administration of compound 3h once a day from day 14 to 28, aspirin was given intragastrically as the positive control. The results are shown in .
Figure 8. (A) Effect of compound 3h on paw swelling in rats with adjuvant arthritis (AA); (B) Effect of compound 3h on weight in rats with adjuvant arthritis. Data are the mean ± SD, n = 5 per group. Aspirin was the positive control: ###p<.01 compared with control; ∗∗∗p<.001, ∗p<.05 vs. AA group.
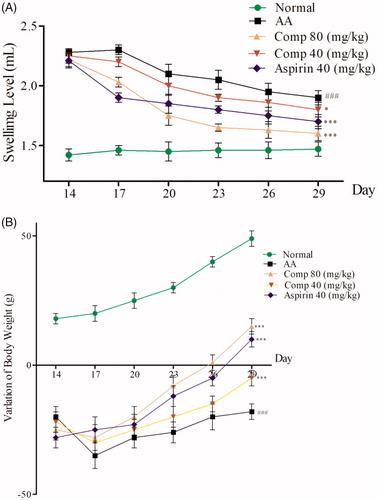
Histopathological analysis of knee joints was conducted at the end of the experiment to evaluate the level of inflammation and changes of the tissue. Representative histological photographs of the tissue sections are presented in . No inflammation was seen in normal rats (. AIA rats exhibited extensive inflammatory cells infiltration, synovial hyperplasia, and cartilage destruction (. Administration of compound 3h at dose of 40 mg/kg significantly reduced the infiltration of inflammatory cells and improved synovial hyperplasia and cartilage destruction (. Escalating the dose of compound 3h (80 mg/kg) resulted in a better therapeutic effect on AIA rats (. Taken together, the results of histopathological evaluation suggested that compound 3h was able to attenuate inflammation of AIA rats.
Conclusions
In summary, nineteen 4-ferrocenyl-3,4-dihydrocoumarin derivatives were designed and synthesised to discover novel agents with anti-inflammatory activity. The preliminary screening results indicated that most target compounds exhibited significant NO inhibitory activity with low toxicity against RAW 264.7 cells. Based on the SAR, the introduction of coplanar groups like naphthalenyl and benzodioxol bridging to the dihydrocoumarin ring is beneficial to the NO inhibitory activity. Furthermore, the compound 3h was found to be able to inhibit the secretion of IL-6, NO, and TNF-α in a dose-dependent manner. The preliminary mechanism investigation indicated that compound 3h could significantly suppress LPS-induced expressions of iNOS, COX-2, IL-6, TNF-α, and NO via inactivating the NF-κB and ERK/MAPK signalling pathways. The further in vivo anti-inflammatory study revealed that compound 3h effectively relieved the histological changes in knee joints of AIA rats.
Supplemental Material
Download PDF (1.6 MB)Disclosure statement
No potential conflict of interest was reported by the authors.
Additional information
Funding
References
- Guo Q, Wang Y, Xu D, et al. Rheumatoid arthritis: pathological mechanisms and modern pharmacologic therapies. Bone Res 2018;6:15.
- Zeb A, Qureshi OS, Yu CH, et al. Enhanced anti-rheumatic activity of methotrexate-entrapped ultradeformable liposomal gel in adjuvant-induced arthritis rat model. Int J Pharm 2017;525:92–100.
- Miwatashi S, Arikawa Y, Kotani E, et al. Novel inhibitor of p38 MAP kinase as an anti-TNF-alpha drug: discovery of N-[4-[2-ethyl-4-(3-methylphenyl)-1,3-thiazol-5-yl]-2-pyridyl]benzamide (TAK-715) as a potent and orally active anti-rheumatoid arthritis agent. J Med Chem 2005;48:5966–79.
- Safina BS, Baker S, Baumgardner M, et al. Discovery of novel PI3-kinase delta specific inhibitors for the treatment of rheumatoid arthritis: taming CYP3A4 time-dependent inhibition. J Med Chem 2012;55:5887–900.
- Alam J, Jantan I, Bukhari S. Rheumatoid arthritis: recent advances on its etiology, role of cytokines and pharmacotherapy. Biomed Pharmacother 2017;92:615–33.
- Steffan RJ, Matelan E, Ashwell MA, et al. Synthesis and activity of substituted 4-(indazol-3-yl)phenols as pathway-selective estrogen receptor ligands useful in the treatment of rheumatoid arthritis. J Med Chem 2004;47:6435–8.
- Ruan BF, Ge WW, Cheng HJ, et al. Resveratrol-based cinnamic ester hybrids: synthesis, characterization, and anti-inflammatory activity. J Enzyme Inhib Med Chem 2017;32:1282–90.
- Chen LZ, Yao L, Jiao MM, et al. Novel resveratrol-based flavonol derivatives: synthesis and anti-inflammatory activity in vitro and in vivo. Eur J Med Chem 2019;175:114–28.
- Lippert R, Shubina TE, Vojnovic S, et al. Redox behavior and biological properties of ferrocene bearing porphyrins. J Inorg Biochem 2017;171:76–89.
- Spiller F, Oliveira Formiga R, Fernandes da Silva Coimbra J, et al. Targeting nitric oxide as a key modulator of sepsis, arthritis and pain. Nitric Oxide 2019;89:32–40.
- Yao RS, Lu XQ, Guan QX, et al. Synthesis and biological evaluation of some novel resveratrol amide derivatives as potential anti-tumor agents. Eur J Med Chem 2013;62:222–31.
- Shi JB, Chen LZ, Wang BS, et al. Novel pyrazolo[4,3-d]pyrimidine as potent and orally active inducible nitric oxide synthase (iNOS) dimerization inhibitor with efficacy in rheumatoid arthritis mouse model. J Med Chem 2019;62:4013–31.
- Huh JR, Englund EE, Wang H, et al. Identification of potent and selective diphenylpropanamide RORgamma inhibitors. ACS Med Chem Lett 2013;4:79–84.
- Chen D, Bi A, Dong X, et al. Luteolin exhibits anti-inflammatory effects by blocking the activity of heat shock protein 90 in macrophages. Biochem Biophys Res Commun 2014;443:326–32.
- Ouyang L, Dan Y, Shao Z, et al. Effect of umbelliferone on adjuvant-induced arthritis in rats by MAPK/NF-κB pathway. Drug Des Dev Ther 2019;13:1163–70.
- Gou KJ, Zeng R, Ren XD, et al. Anti-rheumatoid arthritis effects in adjuvant-induced arthritis in rats and molecular docking studies of Polygonum orientale L. extracts. Immunol Lett 2018;201:59–69.
- Mateen S, Zafar A, Moin S, et al. Understanding the role of cytokines in the pathogenesis of rheumatoid arthritis. Clin Chim Acta 2016;455:161–71.
- Xue Q, Yan Y, Zhang R, Xiong H. Regulation of iNOS on immune cells and its role in diseases. Int J Mol Sci 2018;19:3085.
- Cuzzocrea S. Role of nitric oxide and reactive oxygen species in arthritis. Curr Pharm Des 2006;12:3551–70.
- Huang QC, Huang RY. The cyclooxygenase-2/thromboxane A2 pathway: a bridge from rheumatoid arthritis to lung cancer? Cancer Lett 2014;354:28–32.
- Hoxha M. A systematic review on the role of eicosanoid pathways in rheumatoid arthritis. Adv Med Sci 2018;63:22–9.
- Timothy SB, John WC. The role of nuclear factor-kB in cytokine gene regulation. Am J Respir Cell Mol Biol 1997;17:3–9.
- Ivanenkov YA, Balakin KV, Lavrovsky Y. Small molecule inhibitors of NF-kB and JAK/STAT signal transduction pathways as promising anti-inflammatory therapeutics. Mini Rev Med Chem 2011;11:55–78.
- Tak PP, Firestein GS. NF-kappaB: a key role in inflammatory diseases. J Clin Invest 2001;107:7–11.
- Thummuri D, Jeengar MK, Shrivastava S, et al. Thymoquinone prevents RANKL-induced osteoclastogenesis activation and osteolysis in an in vivo model of inflammation by suppressing NF-KB and MAPK signalling. Pharmacol Res 2015;99:63–73.
- Sujitha S, Rasool M. MicroRNAs and bioactive compounds on TLR/MAPK signaling in rheumatoid arthritis. Clin Chim Acta 2017;473:106–15.
- Shah SU, Ashraf N, Soomro ZH, et al. The anti-arthritic and anti-oxidative effect of NBD (6-nitro-1,3-benzodioxane) in adjuvant-induced arthritis (AIA) in rats. Inflamm Res 2012;61:875–87.