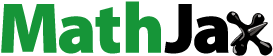
Abstract
New dipeptide–dihydroquinolinone derivatives were successfully synthesised by benzotriazole mediated nucleophilic acyl substitution reaction and their structures were elucidated by spectroscopic and analytic techniques. The carbonic anhydrase (CA, EC 4.2.1.1) inhibitory activity of the new compounds was determined against four human (h) isoforms, hCA I, hCA II, hCA IX and hCA XII. While all compounds showed moderate to good in vitro CA inhibitory properties against hCA IX and hCA XII with inhibition constants in the micromolar level (37.7–86.8 and 2.0–8.6 µM, respectively), they did not show inhibitory activity against hCA I and hCA II up to 100 µM concentration. The antioxidant capacity of the peptide–dihydroquinolinone conjugates was determined using 1,1-diphenyl-2-picrylhydrazyl (DPPH) radical scavenging method. Most of the synthesised compounds showed low antioxidant activities compared to the control antioxidant compounds BHA and α-tocopherol.
1. Introduction
2-Quinolinones derivatives constitute a privilege class of heterocyclic compounds for their wide range of important biological properties such as such as antibacterialCitation1,Citation2, antimalarialCitation3, antitumorCitation4, carbonic anhydrase inhibitorCitation5,Citation6, antioxidant, anti-tuberculosisCitation7, antiparasiticCitation8 and anti-hepatit C and B viruses activityCitation9. For example, the 3,4-dihydro-2-quinolinone structure is found in a number of biologically active and FDA approved medicine such as cilostazol, carteolol and aripiprazole. Compounds containing the 3,4-dihydro-2(1H)-quinolone moiety also exhibit a variety of activities in both the peripheral and central tissues, which includes phosphodiesterase inhibition, blocking of β-adrenergic receptors, antagonism of vasopressin receptors and interaction with serotonin and dopamine receptorsCitation10. Since heterocyclic compounds containing peptide have been of particular interest because their biocompatibility of the peptide parts play a crucial role in transporting into mammalian tissue of these type drug candidatesCitation11. The carbonic anhydrase enzymes play a role in many physiological events, such as acid base balance, regulation of cardiovascular tone, digestion, ion exchange between cell sections and providing the necessary bicarbonate for different enzymatic reactionsCitation12–14. The emergence of possible relationships between carbonic anhydrase enzyme and cancer in recent years has increased the interest in carbonic anhydrase enzyme inhibitorsCitation15,Citation16.
Encouraged by the above literature information and our interest in the biological and chemical properties of such compounds, synthesis and carbonic anhydrase and antioxidant properties of mono and dipeptide containing dihydroquinolinone derivatives have been studied.
2. Material and methods
2.1. Chemistry
The starting materials and reagents used in the reactions were supplied commercially by Across, Aldrich or Merck Chemical Co. 1H NMR (400.13 MHz) and 13C NMR (100.62 MHz) spectra were obtained using Bruker Advance 400 Ultra shield high performance digital FT NMR spectrometer. Infra-red spectra were recorded with ATR equipment in the range 4000–200 cm−1 on a Perkin-Elmer FT-IR spectrophotometer. Elemental analyses were performed by LECO CHNS-932 elemental analyser. Melting points were recorded using an electrothermal-9200 melting point apparatus and are uncorrected. Positive or negative-ion electrospray ionization (ESI) mass spectra were recorded on a double-focusing Finnigan MAT 95 instrument with BE geometry. All microwave-assisted reactions were carried out in a microwave oven system manufactured by Milestone (Milestone Start S Microwave Labstation for Synthesis). Benzotriazole derivatives of N-protected amino acids (I–V)Citation3,Citation17,Citation18 and dipeptide (VI)Citation19,Citation20 were prepared according to literature procedure.
2.2. General procedure for the synthesis of mono or dipeptide–dihydroquinolinone conjugates, 1–6
A mixture of equivalent amounts of the appropriate N-protected aminoacylbenzotriazole and 7-amino-3,4-dihydroquinolin-2(1H)-one was subjected to microwave irradiation (100 W, 70 °C) in anhydrous THF (5 ml) for 30 min. On completion of the reaction followed by TLC, all volatiles were removed by rotavapor and the obtained crude product was crystallised from methanol.
2.2.1. Tert-butyl (R)-(1-oxo-1-((2-oxo-1,2,3,4-tetrahydroquinolin-7-yl)amino)propan-2-yl)carbamate, 1
Cream solid (77%); mp 197–198 °C; 1H NMR (DMSO-d6, 400 MHz), δ 10.17 (s, 1H, NHlactam), 9.91 (s, 1H,NH), 7.28 (d, 1H, N–H, J = 4 Hz), 7.17–7.09 (m, 3 H, Ar–H) , 4.16–4.13 (m, 1H, CHNH), 2.86 (t, 2H, COCH2, J = 8 Hz), 2.47 (t, 2H, COCH2CH2, J = 8 Hz), 1.44 (s, 9H, (OCH(CH3)3), 1.29 (d, 3H, CHCH3, J = 8 Hz). 13C NMR (DMSO-d6, 400 MHz), δ 171.8 (COCH2), 170.8 (COCH), 155.5 (COOCH2Ph), 138.93, 138.51, 128.21, 118.82, 113.29, 106.71 (Ar–C), 78.5 (OCH(CH3)3), 50.8 (CHNH), 31.1 (COCH2CH2), 28.7 (COCH2CH2), 24.8 (OCH(CH3)3), 18.5 (CHCH3). ν(C = O)carbamate: 1605 cm−1, ν(C = O)amide: 1672, 1687 cm−1, ν(N–H)amine: 3200, 3344 cm−1. Elemental analysis: C17H23N3O4 required C, 61.25; H, 6.95; N, 12.60; S, 6.74, found C, 61.02; H, 6.94; N, 12.63. HRMS m/z for C17H23N3O4 [M + Na]+calcd. 356.1586, found 356.2000.
2.2.2. Tert-butyl (R)-(1-oxo-1-((2-oxo-1,2,3,4-tetrahydroquinolin-7-yl)amino)-3-phenylpropan-2-yl)carbamate, 2
Cream solid (87%); mp 180–181 °C; 1H NMR (DMSO-d6, 400 MHz), δ 10.14 (s, 1H, NHlactam), 9.99 (s, 1H,NH), 7.33–7.07 (m, 9 H, Ar–H + NH) , 4.34–4.29 (m, 1H, CHNH), 2.99–2.95 (m, 1H, CH2Ph), 2.83–2.79 (m, 3H, COCH2 + CH2Ph), 2.42 (t, 2H, COCH2CH2), 1.32 (s, 9H, OCH(CH3)3). 13C NMR (DMSO-d6, 400 MHz), δ 171.1 (COCH2), 170.8 (COCH), 155.8 (COOC(CH3)3), 138.9, 138.4, 138.32, 129.7, 128.5, 128.2, 126.6, 1189.0, 113.5, 106.8 (Ar–H),78.7 (OCH(CH3)3), 56.9 (CHNH), 37.9 (CHCH2), 31.1 (COCH2CH2), 28.6 (COCH2CH2), 24.8 (OCH(CH3)3). ν(C = O)carbamate: 1604 cm−1, ν(C = O)amide: 1623, 1671 cm−1, ν(N–H)amine: 3326 cm−1. Elemental analysis: C23H27N3O4 required C, 67.46; H, 6.65; N, 10.26, found C, 67.11; H, 6.56; N, 10.30.HRMS m/z for C23H27N3O4 [M − H]+calcd. 408.1923, found 408.2000.
2.2.3. Tert-butyl (S)-(4-(methylthio)-1-oxo-1-((2-oxo-1,2,3,4-tetrahydroquinolin-7-yl)amino)butan-2-yl)carbamate, 3
Beige solid (86%); mp 171–172 °C; 1H NMR (DMSO-d6, 400 MHz), δ 10.12 (s, 1H, NHlactam), 9.93 (s, 1H,NH), 7.23 (d, 1H, N–H, J = 4 Hz), 7.13–7.06 (m, 3 H, Ar–H) , 4.15–4.10 (m, 1H, CHNH), 2.80 (t, 2H, COCH2, J = 8 Hz), 2.48–2.40 (m, 4H, COCH2CH2 + CHCH2CH2S), 2.06 (s, 3H, CH3), 1.88–1.85 (m, 2H, CHCH2CH2S), 1.38 (s, 9H, OC(CH3)3). 13C NMR (DMSO-d6, 400 MHz), δ 171.2 (COCH2), 170.9 (COCH), 156.0 (COOC(CH3)3), 138.9, 138.3, 128.2, 119.0, 113.5, 106.9 (Ar–H), 78.7 (OCH(CH3)3), 65.4 (CHNH), 54.8 (COCH2CH2), 31.1 (CHCH2CH2S), 30.2 (COCH2CH2), 28.6 (SCH3), 24.8 (CHCH2CH2S), 15.1 (OCH(CH3)3). ν(C = O)carbamate: 1613 cm−1, ν(C = O)amide: 1669, cm−1, ν(N–H)amine: 3224 cm−1. Elemental analysis: C19H27N3O4 required: C, 57.99; H, 6.92; N, 10.68; S, 8.15, found: C, 57.89; H, 6.86; N, 10.67; S, 8.18. HRMS m/z for C19H27N3O4S [M − H]+ calcd. 392.1644, found 392.1000; [M + Na]+ calcd. 416.1620, found 416.3000.
2.2.4. Benzyl (R)-(1-oxo-1-((2-oxo-1,2,3,4-tetrahydroquinolin-7-yl)amino)-3-(phenylthio)propan-2-yl)carbamate, 4
Cream solid (74%); mp 150–151 °C; 1H NMR (DMSO-d6, 400 MHz), δ 10.24 (s, 1H, NHlactam), 10.20 (s, 1H, NH), 7.88 (d, 1H, N–H, J = 8 Hz), 7.45–7.12 (m, 13 H, Ar–H) , 5.11 (s, 2H, CH2Ph), 4.44–4.43 (m, 1H, CHNH), 3.42–3.39 (m, 1H, CH2S), 3.27–3.22 (m, 1H, CH2S), 2.86 (t, 2H, COCH2, J = 8 Hz), 2.48 (m, 4H, COCH2CH2, J = 8 Hz). 13C NMR (DMSO-d6, 400 MHz), δ 170.8 (COCH2), 169.0 (COCH), 156.4 (COOCH2Ph), 138.9, 138.1, 137.3, 136.3, 129.6, 128.8, 128.8, 128.3, 128.2, 126.4, 119.3, 113.7, 107.1 (Ar–C), 66.1 (CH2Ph), 55.3 (CHNH), 35.3 (CHCH2S), 31.1 (COCH2CH2), 24.8 (COCH2CH2). ν(C = O)carbamate: 1606 cm−1, ν(C = O)amide: 1659, 1680 cm−1, ν(N–H)amine: 3288 cm−1. Elemental analysis: C26H25N3O4S required: C, 65.67; H, 5.30; N, 8.84; S, 6.74, found C, 65.39; H, 5.04; N, 8. 71; S, 6.63. HRMS m/z for C26H25N3O4S [M + H]+ calcd. 476.1644, found 476.3000.
2.2.5. Benzyl (S)-(4-(methylthio)-1-oxo-1-((2-oxo-1,2,3,4-tetrahydroquinolin-7-yl)amino)butan-2-yl)carbamate, 5
Cream solid (78%); mp 137–138 °C; 1H NMR (DMSO-d6, 400 MHz), δ 10.19 (s, 1H, NHlactam), 10.08 (s, 1H, NH), 7.70 (d, 1H, N–H, J = 8 Hz), 7.51–7.12 (m, 8 H, Ar–H) , 5.09 (s, 2H, CH2Ph), 4.31–4.25 (m, 1H, CHNH), 2.86 (t, 2H, COCH2, J = 8 Hz), 2.60–2.39 (m, 4H, CHCH2CH2 + COCH2CH2), 2.10 (s, 3H, CH3), 1.99–1.91 (m, 2H, CHCH2CH2SCH3). 13C NMR (DMSO-d6, 400 MHz), δ 170.9 (COCH2), 170.9 (COCH), 156.6 (COOCH2Ph), 138.9, 138.3, 137.4, 128.8, 128.3, 128.2, 119.1, 113.5, 108.5, 106.9 (Ar–C), 66.0 (CH2Ph), 55.2 (CHNH), 32.0 (COCH2CH2), 31.1 (CHCH2CH2), 30.2 (COCH2CH2), 24.8 (CH3), 15.1 (CH2CH2S). ν(C = O)carbamate: 1604 cm−1, ν(C = O)amide: 1654, 1684 cm−1, ν(N–H)amine: 3283 cm−1. Elemental analysis: C22H25N3O4S required C, 61.81; H, 5.89; N, 9.83; S, 7.50, found C, 61. 72; H, 5.81; N, 9.62; S, 7.51. HRMS m/z for C22H25N3O4S [M + H]+ calcd. 427.1644, found 428.2000.
2.2.6. Benzyl ((R)-1-oxo-1-(((S)-1-oxo-1-((2-oxo-1,2,3,4-tetrahydroquinolin-6-yl)amino)-3-phenylpropan-2-yl)amino)-3-phenylpropan-2-yl)carbamate, 6
Cream solid (62%); mp 197–198 °C; 1H NMR (DMSO-d6, 400 MHz), δ 10.15 (s, 1H, NHlactam), 10.06 (s, 1H, NH), 8.30 (d, 1H, N–H, J = 8 Hz), 7.47 (d, 1H, N–H, J = 8 Hz), 7.33–7.09 (m, 18 H, Ar–H) , 4.95 (s, 2H, CH2Ph), 4.73–4.68 (m, 1H, CHNH), 4.32–4.26 (m, 1H, CHNH), 3.10–3.06 (m, 1H, CH2Ph), 2.98–2.91 (m, 2H, CH2Ph), 2.81 (t, 2H, COCH2, J = 8 Hz), 2.73–2.66 (m, 1H, 1H, CH2P), 2.42 (t, 2H, COCH2CH2, J = 8 Hz). 13C NMR (DMSO-d6, 400 MHz), δ 171.9 (COCH2), 170.8, 170.3 (COCH), 156.2 (COOCH2Ph), 138.9, 138.4, 138.2, 137.8, 137.5, 129.7, 129.6, 128.8, 128.6, 128.5, 128.2, 128.1, 127.9, 126.9, 126.7, 119.1, 113.5, 106.9 (Ar–C), 65.7 (OCH2Ph), 56.5 (CHNH), 55.2 (CHNH), 38.3 (CHCH2), 38.0 (CH2CH2), 31.1 (CHCH2), 24.8 (CH2CH2). ν(C = O)carbamate: 1608 cm−1, ν(C = O)amide: 1647, 1680 cm−1, ν(N–H)amine: 3282 cm−1. Elemental analysis: C35H34N4O5 required C, 71.17; H, 5.80; N, 9.49, found C, 71. 05; H, 5.78; N, 9.21. HRMS m/z for C35H34N4O5 [M + H]+ calcd. 591.2607, found 593.4000; [M + Na]+ calcd. 613.2427, found 613.3000.
2.3. Ca inhibition
An Applied Photophysics Stopped-Flow instrument has been used for assaying the CA catalysed CO2 hydration activity by using method of KhalifahCitation21. Phenol red (at a concentration of 0.2 mM) has been used as indicator, working at the absorbance maximum of 557 nm, with 20 mM HEPES (pH 7.5) as buffer and 20 mM Na2SO4 (for maintaining constant the ionic strength), following the initial rates of the CA-catalysed CO2 hydration reaction for a period of 10–100 s. The CO2 concentrations ranged from 1.7 to 17 mM for the determination of the kinetic parameters and inhibition constants. For each inhibitor at least six traces of the initial 5–10% of the reaction have been used for determining the initial velocity. The uncatalysed rates were determined in the same manner and subtracted from the total observed rates. Stock solutions of inhibitor (0.1 mM) were prepared in distilled–deionised water and dilutions up to 0.01 nM were done thereafter with the assay buffer. Inhibitor and enzyme solutions were pre-incubated together for 15 min at room temperature prior to assay, in order to allow for the formation of the E–I complex. The inhibition constants were obtained by non-linear least-square methods using PRISM (www.graphpad.com), and non-linear least squares methods, values representing the mean of at least three different determinations, as described earlier by usCitation22–27.
2.3. Antioxidant testing
2.3.1. DPPH radical scavenging activity
Antioxidant activity was determined based on the ability of the antioxidants to act as radical scavengers towards the stable free radical, 1,1-diphenyl-2-picrylhydrazyl (DPPH). As detailed by Yang et alCitation28, 1 ml of antioxidant solution (solubilised in ethanol) was added to 3 ml of a 0.1 mM ethanolic solution of DPPH. After 30 min at ambient temperature in darkness, absorbance readings were taken at 517 nm. Inhibition (%) was calculated using the equation
where As was the absorbance reading for samples containing antioxidant, Ao was the absorbance of the antioxidant in pure methanol and Ab corresponded to the absorbance of the DPPH solution.
3. Results and discussion
3.1. Synthesis and characterization of the new mono and dipeptide–dihydroquinolinone derivatives
New N-protected monopeptide and dipeptide–dihydroquinolinone conjugates (1–6) were synthesised by the reaction of 7-amino-3,4-dihydroquinolin-2(1H)-one with N-protected aminoacylbenzotriazole under microwave heating at 70 °C for 30 min with good yields of 62–87%. The synthesis of the N-protected mono and dipeptide–dihydroquinolinone conjugates 1–6 is summarised in Scheme 1.
Scheme 1. Synthesis pathways of the new dihydroquinolinone conjugates of N-protected amino acids and dipeptide. Conditions and reagents: (i) r.t., 2 h in THF; 70 °C, 30 min in THF.
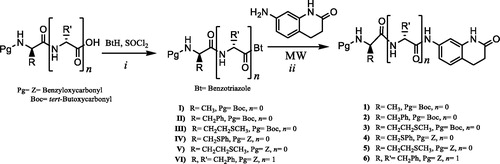
The structures of N-protected mono and dipeptide–dihydroquinolinone conjugates (1–6) were elucidated by 1H NMR, 13C NMR, IR, mass and elemental analyses. The characteristic NH resonances of the lactam moiety of the mono or dipeptide–dihydroquinolinone conjugates in the 1H NMR spectra of compounds (1–6) were observed at 10.12–10.24 ppm region as singlet peak. The NH peaks at position 7 of the quinolinone part of the mono and dipeptide–dihydroquinolinone conjugates were observed at 9.91–9.20 ppm region as a singlet peak in the 1H NMR spectra. The carbamate NH proton signals for the protected group of the conjugates 1–6 were observed as doublet at 7.23–7.77 ppm, except compound 2 which resonated together with aromatic protons. Other NH peak for compound 6 was observed as doublet at 8.30 ppm. All NH protons were confirmed by deuterium exchange by D2O. Carbonyl resonances of the lactam carbonyl, amide carbonyl and carbamate carbonyl for monopeptide–dihydroquinolinone conjugates were observed around 170.8–171.9, 169.0–170.9 and 155.5–156.6 ppm, respectively. Carbonyl resonances of dipeptide–dihydroquinolinone conjugates 6 were appeared at 171.9, 170.8, 170.3 and 156.2 ppm, respectively. All other aliphatic and aromatic protons and carbons for mono and dipeptide–dihydroquinolinone conjugates observed at expected regions and were in accordance with the assumed structures. The IR spectra of mono and dipeptide–dihydroquinolinone conjugates, 1–6, showed characteristic lactam or amide carbonyl peaks around between 1687 and 1623 cm−1, whereas the carbamate carbonyl peaks around between 1613 and 1604 cm−1.
It was observed that in the mass spectra of all compounds 1–6, there were corresponding molecular ion peaks for assumed structures.
3.2. Carbonic anhydrase inhibition
Among the biological activities, human carbonic anhydrase (hCA, EC 4.2.1.1) inhibition has been the subject of several investigations since the discovery of the biological importance of this enzyme in several living organismsCitation29. Since many heterocyclic compounds exhibit CA inhibitor propertiesCitation6,Citation30,Citation31, we synthesised novel type mono and dipeptide–dihydroquinolinone conjugates to explore their possible carbonic anhydrase enzyme inhibition capacities against human carbonic anhydrase hCA I, II, IX and XII.
In order to explore the inhibitory capacity of all the prepared new mono and dipeptide–dihydroquinolinone conjugates (1–6) have been evaluated by means of a stopped flow CO2 hydrase assay against four human (h) CA isoforms (hCA I, hCA II, hCA IX and hCA XII). Inhibition results of the compounds are reported in , along with those referred to acetazolamide (AAZ), used as standard inhibitor. When the results in are analysed, the following structure–activity relationships (SAR) can be obtained.
Table 1. Inhibition data of hCA I, hCA II, hCA IX and hCA XII with compounds 1–6 and the standard sulphonamide inhibitor acetazolamide (AAZ) by a stopped flow CO2 hydrase assay.
Table 2. Antioxidant activities of the synthesised mono and dipeptide–dihydroquinolinone conjugates.
All compounds were found to be ineffective up to 100 µM concentration against hCA I and hCA II compared to AAZ, which has an inhibition value of 0.250 µM ().
All the synthesised mono and dipeptide–dihydroquinolinone conjugates exhibited weak inhibitory properties against hCA IX, with Ki values among the series, ranging from 37.7 to 86.8 µM (). Among the compounds, it was found that those containing methionine (compounds 2 and 5) had a stronger inhibition capacity with 37.7 and 41.2 K values than others in the series.
As for the tumour associated isoform CA XII, it revealed to be moderately inhibited by all compounds with Ki values among the series, ranging from 2.0 to 8.6 µM (). However, the results are still lower than the Ki value of standard compound AAZ.
3.3. Antioxidant testing
3.3.1. DPPH radical scavenging activity
The antioxidant activity of the compounds was determined based on the ability of the antioxidants to act as radical scavengers towards the stable free radical, 1,1-diphenyl-2-picrylhydrazyl (DPPH)Citation28.
Monopeptide–dihydroquinolinone derivatives, 1–5, from synthesised compounds generally did not show antioxidant activity compared to standard antioxidant compounds α-tocopherol and BHA. Only the dipeptide, 6, showed some antioxidant activity at a concentration of 125 μg/ml ().
4. Conclusions
Mono and dipeptide–dihydroquinolinone derivatives synthesised within the scope of this study were synthesised by benzotriazole-mediated method with good yields. The synthesised compounds were found to be ineffective up to 100 µM concentration against hCA I and hCA II, whereas it was found to be effective against hCA IX and hCA XII at the studied concentrations. The antioxidant activity of the synthesised compounds were generally found to be ineffective at concentrations of 12.5–125 µg/ml. Only the dipeptide–dihydroquinolinone compound 6 showed an activity at a concentration of 125 µg/ml, close to half the antioxidant values shown by standard antioxidants, α-tocopherol and BHA.
Disclosure statement
No potential conflict of interest was reported by the author(s).
Additional information
Funding
References
- Feng LS, Liu ML, Wang S, et al. Synthesis of naphthyridone derivatives containing 8-alkoxyimino-1,6-dizaspiro[3.4]octane scaffolds. Tetrahedron 2011;67:8264–70.
- Hu YQ, Zhang S, Xu Z, et al. 4-Quinolone hybrids and their antibacterial activities. Eur J Med Chem 2017;141:335–45.
- Panda SS, Ibrahim MA, Küçükbay H, et al. Synthesis and antimalarial bioassay of quinine–peptide conjugates. Chem Biol Drug Des 2013;82:361–6.
- Katariya KD, Shah SR, Reddy D. Anticancer, antimicrobial activities of quinoline based hydrazone analogues: synthesis, characterization and molecular docking. Bioorg Chem 2020;94:103406.
- Vullo D, Isik S, Bozdag M, et al. 7-Amino-3,4-dihydro-1H-quinolin-2-one, a compound similar to the substituted coumarins, inhibits α-carbonic anhydrases without hydrolysis of the lactam ring. J Enzyme Inhib Med Chem 2015;30:773–7.
- Küçükbay FZ, Küçükbay H, Tanc M, Supuran CT. Synthesis and carbonic anhydrase inhibitory properties of amino acid – coumarin/quinolinone conjugates incorporating glycine, alanine and phenylalanine moieties. J Enzyme Inhib Med Chem 2016;31:1198–202.
- Liu B, Li F, Zhou T, et al. Quinoline derivatives with potential activity against multidrug-resistant tuberculosis. J Heterocycl Chem 2018;55:1863–73.
- Ibrahim MA, Hassanin HM, Abass M, Badran S. Synthesis of substituted quinolinone ketones derived with some five, six, and seven-membered heterocycles. J Turk Chem Soc 2016; 3:27–45.
- Cheng P, Zhang Q, Ma YB, et al. Synthesis and in vitro anti-hepatitis B virus activities of 4-aryl-6-chloro-quinolin-2-one and 5-aryl-7-chloro-1,4-benzodiazepine derivatives. Bioorganic Med Chem Lett 2008;18:3787–9.
- Meiring L, Petzer JP, Petzer A. A review of the pharmacological properties of 3,4-dihydro-2(1H)-quinolinones. Mini Rev Med Chem 2018;18:828–36.
- Panda SS, Hall CD, Scriven E, Katritzky AR. Aminoacyl benzotriazolides: versatile reagents for the preparation of peptides and their mimetics and conjugates. Aldrichimica Acta 2014;46:43–58.
- (a) Supuran CT. Exploring the multiple binding modes of inhibitors to carbonic anhydrases for novel drug discovery. Expert Opin Drug Discov in press.; (b) Supuran CT. Structure and function of carbonic anhydrases. Biochem J 2016; 473:2023–32; (c) Supuran CT. Advances in structure-based drug discovery of carbonic anhydrase inhibitors. Expert Opin Drug Discov 2017;12:61–88; (d) Nocentini A, Supuran CT. Advances in the structural annotation of human carbonic anhydrases and impact on future drug discovery. Expert Opin Drug Discov 2019; 14:1175–97.
- (a) Supuran CT. Carbonic anhydrases: novel therapeutic applications for inhibitors and activators. Nat Rev Drug Discov 2008;7:168–81; (b) Neri D, Supuran CT. Interfering with pH regulation in tumours as a therapeutic strategy. Nat Rev Drug Discov 2011;10:767–77; (c) Supuran CT. How many carbonic anhydrase inhibition mechanisms exist? J Enzyme Inhib Med Chem 2016;31:345–60; (d) Supuran CT. Carbonic anhydrases and metabolism. Metabolites 2018;8:25.
- (a) Supuran CT, Briganti F, Menabuoni L, et al. Carbonic anhydrase inhibitors – Part 78. Synthesis of water-soluble sulfonamides incorporating β-alanyl moieties, possessing long lasting- intraocular pressure lowering properties via the topical route. Eur J Med Chem 2000;35:309–21; (b) Supuran CT, Capasso C. An overview of the bacterial carbonic anhydrases. Metabolites 2017;7:56; (c) Supuran CT, Capasso C. Biomedical applications of prokaryotic carbonic anhydrases. Expert Opin Ther Pat 2018;28:745–54; (d) Supuran CT. Carbonic anhydrase inhibitors and their potential in a range of therapeutic areas. Expert Opin Ther Pat 2018;28:709–12.
- (a) Supuran CT, Alterio V, Di Fiore A, et al. Inhibition of carbonic anhydrase IX targets primary tumors, metastases, and cancer stem cells: three for the price of one. Med Res Rev 2018;38:1799–836; (b) Supuran CT. Carbonic anhydrase inhibitors as emerging agents for the treatment and imaging of hypoxic tumors. Expert Opin Investig Drugs 2018;27:963–70; (c) Nocentini A, Supuran CT. Carbonic anhydrase inhibitors as antitumor/antimetastatic agents: a patent review (2008–2018). Expert Opin Ther Pat 2018;28:729–40.
- (a) Supuran CT. Applications of carbonic anhydrases inhibitors in renal and central nervous system diseases. Expert Opin Ther Pat 2018;28:713–21; (b) Supuran CT, Altamimi ASA, Carta F. Carbonic anhydrase inhibition and the management of glaucoma: a literature and patent review 2013–2019. Expert Opin Ther Pat 2019;29:781–92; (c) Supuran CT. The management of glaucoma and macular degeneration. Expert Opin Ther Pat 2019;29:745–7.
- El Khatib M, Jauregui L, Tala SR, et al. Solution-phase synthesis of chiral O-acyl isodipeptides. Medchemcomm 2011;2:1087.
- Bugday N, Kucukbay FFZ, Apohan E, et al. Synthesis and evaluation of novel benzimidazole conjugates incorporating amino acids and dipeptide moieties. Lett Org Chem 2017;14:198–206.
- (a) Küçükbay H, Buğday N, Küçükbay FZ, et al. Bioorganic chemistry synthesis and carbonic anhydrase inhibitory properties of novel 4-(2-aminoethyl)benzenesulfonamide–dipeptide conjugates. Bioorg Chem 2019;83:414–23; (b) Supuran CT. Carbon- versus sulphur-based zinc binding groups for carbonic anhydrase inhibitors? J Enzyme Inhib Med Chem 2018;33:485–95; (c) Nocentini A, Bonardi A, Gratteri P, et al. Steroids interfere with human carbonic anhydrase activity by using alternative binding mechanisms. J Enzyme Inhib Med Chem 2018;33:1453–9.
- (a) Buğday N, Küçükbay FZ, Küçükbay H, et al. Synthesis of novel dipeptide sulfonamide conjugates with effective carbonic anhydrase I, II, IX, and XII inhibitory properties. Bioorg Chem 2018;81:311–8; (b) Innocenti A, Vullo D, Scozzafava A, et al. Carbonic anhydrase inhibitors. Interactions of phenols with the 12 catalytically active mammalian isoforms (CA I–XIV). Bioorg Med Chem Lett 2008;18:1583–7; (c) Innocenti A, Vullo D, Scozzafava A, et al. Carbonic anhydrase inhibitors. Inhibition of mammalian isoforms I–XIV with a series of substituted phenols including paracetamol and salicylic acid. Bioorg Med Chem 2008;16:7424–8; (d) Bayram E, Senturk M, Kufrevioglu OI, et al. In vitro effects of salicylic acid derivatives on human cytosolic carbonic anhydrase isozymes I and II. Bioorg Med Chem 2008;16:9101–5.
- Khalifah RG. The carbon dioxide hydration activity of carbonic anhydrase. I. Stop-flow kinetic studies on the native human isoenzymes B and C. J Biol Chem 1971;246:2561–73.
- (a) Mincione F, Starnotti M, Menabuoni L, et al. Carbonic anhydrase inhibitors: 4-sulfamoyl-benzenecarboxamides and 4-chloro-3-sulfamoyl-benzenecarboxamides with strong topical antiglaucoma properties. Bioorganic Med Chem Lett 2001;11:1787–91; (b) Tars K, Vullo D, Kazaks A, et al. Sulfocoumarins (1,2-benzoxathiine-2,2-dioxides): a class of potent and isoform-selective inhibitors of tumor-associated carbonic anhydrases. J Med Chem 2013;56:293–300; (c) D'Ambrosio K, Carradori S, Monti SM, et al. Out of the active site binding pocket for carbonic anhydrase inhibitors. Chem Commun 2015;51:302–5; (d) Güzel-Akdemir Ö, Angeli A, Demir K, et al. Novel thiazolidinone-containing compounds, without the well-known sulphonamide zinc-binding group acting as human carbonic anhydrase IX inhibitors. J Enzyme Inhib Med Chem 2018;33:1299–308.
- (a) Del Prete S, Vullo D, De Luca V, et al. Biochemical characterization of recombinant β-carbonic anhydrase (PgiCAb) identified in the genome of the oral pathogenic bacterium Porphyromonas gingivalis. J Enzyme Inhib Med Chem 2015;30:366–70; (b) Ammazzalorso A, Carradori S, Angeli A, et al. Fibrate-based N-acylsulphonamides targeting carbonic anhydrases: synthesis, biochemical evaluation, and docking studies. J Enzyme Inhib Med Chem 2019;34:1051–61.
- (a) Supuran CT, Scozzafava A. Carbonic anhydrases as targets for medicinal chemistry. Bioorganic Med Chem 2007;15:4336–50; (b) De Simone G, Supuran CT. (In)organic anions as carbonic anhydrase inhibitors. J Inorg Biochem 2012;111:117–29; (c) Nocentini A, Supuran CT, Winum JY. Benzoxaborole compounds for therapeutic uses: a patent review (2010–2018). Expert Opin Ther Pat 2018;28:493–504.
- (a) Maresca A, Vullo D, Scozzafava A, et al. Inhibition of the β-class carbonic anhydrases from Mycobacterium tuberculosis with carboxylic acids. J Enzyme Inhib Med Chem 2013;28:392–6; (b) Köhler K, Hillebrecht A, Schulze Wischeler J, et al. Saccharin inhibits carbonic anhydrases: possible explanation for its unpleasant metallic aftertaste. Angew Chem Int Ed Engl 2007;46:7697–9.
- (a) Scozzafava A, Passaponti M, Supuran CT, Gülçin I. Carbonic anhydrase inhibitors: guaiacol and catechol derivatives effectively inhibit certain human carbonic anhydrase isoenzymes (hCA I, II, IX and XII). J Enzyme Inhib Med Chem 2015;30:586–91; (b) Pustenko A, Stepanovs D, Žalubovskis R, et al. 3H-1,2-benzoxathiepine 2,2-dioxides: a new class of isoform-selective carbonic anhydrase inhibitors. J Enzyme Inhib Med Chem 2017;32:767–75.
- (a) Küçükbay H, Buğday N, Küçükbay FZ, et al. Synthesis and human carbonic anhydrase I, II, VA, and XII inhibition with novel amino acid–sulphonamide conjugates. J Enzyme Inhib Med Chem 2020;35:489–97; (b) Supuran CT. Carbonic anhydrase activators. Future Med Chem 2018;10:561–73.
- Yang J, Guo J, Yuan J. In vitro antioxidant properties of rutin. LWT – Food Sci Technol 2008;41:1060–6.
- (a) Supuran C. Carbonic anhydrases an overview. Curr Pharm Des 2008;14:603–14; (b) Supuran CT. Acetazolamide for the treatment of idiopathic intracranial hypertension. Expert Rev Neurother 2015;15:851–6; (c) Supuran CT. Drug interaction considerations in the therapeutic use of carbonic anhydrase inhibitors. Expert Opin Drug Metab Toxicol 2016;12:423–31.
- (a) Küçükbay FZ, Buğday N, Küçükbay H, et al. Synthesis, characterization and carbonic anhydrase inhibitory activity of novel benzothiazole derivatives. J Enzyme Inhib Med Chem 2016;31:1221–5; (b) Alterio V, Esposito D, Monti SM, et al. Crystal structure of the human carbonic anhydrase II adduct with 1-(4-sulfamoylphenyl-ethyl)-2,4,6-triphenylpyridinium perchlorate, a membrane-impermeant, isoform selective inhibitor. J Enzyme Inhib Med Chem 2018;33:151–7.
- Payaz DÜ, Küçükbay FZ, Küçükbay H, et al. Synthesis carbonic anhydrase enzyme inhibition and antioxidant activity of novel benzothiazole derivatives incorporating glycine, methionine, alanine, and phenylalanine moieties. J Enzyme Inhib Med Chem 2019;34:343–9.