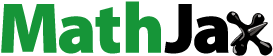
Abstract
A β-carbonic anhydrase (CA, EC 4.2.1.1) from the widespread bacterium Escherichia coli (EcoCAβ), encoded by the CynT2 gene, has been investigated for its catalytic properties and enzymatic activation by a panel of amino acids and amines. EcoCAβ showed a significant catalytic activity for the hydration of CO2 to bicarbonate and a proton, with a kinetic constant kcat of 5.3 × 105 s− and a Michaelis–Menten constant KM of 12.9 mM. The most effective EcoCAβ activators were L- and D-DOPA, L-Tyr, 4-amino-Phe, serotonin and L-adrenaline, with KAs from 2.76 to 10.7 µM. L-His, 2-pyridyl-methylamine, L-Asn and L-Gln were relatively weak activators (KAs from 36.0 to 49.5 µM). D-His, L- and D-Phe, L- and D-Trp, D-Tyr, histamine, dopamine, 2-(aminoethyl)pyridine/piperazine/morpholine, L-Asp, L- and D-Glu have KAs from 11.3 to 23.7 µM. Endogenous CA activators may play a role in bacterial virulence and colonisation of the host.
1. Introduction
The presence of carbonic anhydrase (CA, EC 4.2.1.1) in Escherichia coli was first reported in the early 90s by Guilloton et al.Citation1 The cyn operon present in the genome of this widespread bacterium encodes for at least three such enzymes all belonging to the β-CA genetic family, two with CO2 hydrase activity and one reported to act as a cyanase, i.e., catalysing the reaction of cyanate with bicarbonate to give ammonia and CO2Citation1. One of these β-CAs that is encoded by the CynT2 gene was crystallised by Cronk et al.Citation2,Citation3 which was one of the first representatives of this class of enzymes to be structurally characterised in detail. In the same study, the authors also qualitatively measured the CO2 hydrase activity for this enzyme, demonstrating the enzyme to be active at a pH 8.4, but devoid of any activity at lower, or even neutral pH values. However, the detailed kinetic/thermodynamic parameters or the catalytic efficacy of this enzyme has not reported in thatCitation2 or any other studies to date.
Why are CAs important for E. coliCitation4, or more generally for bacteriaCitation5? These enzymes are present in most organisms investigated to dateCitation5–9, with eight genetically distinct classes of CAs having been reported to date, the α-, β-, γ-, δ-, ζ-, η-, θ- and ι-classesCitation9–12. They all catalyse the simple but crucial interconversion reaction between CO2 and bicarbonate, with the concomitant generation of hydronium ions:
In addition to being involved in pH regulation in all organism and tissues in which they are presentCitation13,Citation14, CAs are also metabolic enzymesCitation15. Indeed, essential metabolic processes require either CO2 or bicarbonate as substrates for carboxylating reactions. Although both CO2 and bicarbonate spontaneously equilibrate in solution at the physiologic pH, the low concentration of CO2 in the air and its rapid diffusion from cells lead to an insufficient bicarbonate availability for in vivo metabolic and biosynthetic requirements in bacteria and other organismsCitation4. For example, Merlin et al.Citation4 calculated that in E. coli, the demand for bicarbonate is 1000–10,000-fold higher than the amount of this anion that is provided by uncatalyzed CO2 hydration. Thus, an enzymatic conversion of CO2 to bicarbonate is therefore strictly required for the growth of E. coli and many other bacteriaCitation5, which is likely why at least four CA genetic families are present in bacteria, the α-, β-, γ- and ι-CAsCitation5,Citation10,Citation12.
In fact, inhibition of CAs belonging to various classes and organisms is exploited pharmacologically for various applicationsCitation16–22. Many of the human isoforms (among the 15 presently known) are targets for diuretics, antiobesity, antiepileptic, antiglaucoma or antitumor agentsCitation16–20. Inhibition of such enzymes from pathogenic bacteria, fungi or protozoans was proposed as a new approach to develop antiinfective agents with novel mechanisms of action, devoid of the drug resistant problems of the currently used agentsCitation21,Citation22. Thus, a large number of drug design studies of CA inhibitors (CAIs) targeting both mammalian and pathogenic CAs are constantly being reportedCitation23,Citation24.
On the other hand, activation studies of various classes of CAs have progressed slowly compared to the inhibition studies. The CA activation mechanism was in fact explained at the molecular level only in 1997 with the report of the first X-ray crystallographic adduct of a CA-activator complex, more precisely the CA II complexed with histamineCitation25. Indeed, it has been demonstrated by Briganti et al.Citation25 and othersCitation8 that the CA activators (CAAs) participate directly in the enzyme catalytic cycle, which can be represented schematically by EquationEquations 1(1)
(1) and Equation2
(2)
(2) .
(1)
(1)
(2)
(2)
The metal hydroxide species of the enzyme (EZn2+-OH−) acts as a strong nucleophile (at physiologic pH) and converts CO2 to bicarbonate, which is subsequently coordinated to the catalytic metal ion [Step 1 in EquationEquation (1)(1)
(1) ]. This adduct is not very stable and its reaction with an incoming water molecule leads to liberation of bicarbonate in solution (Step 2 in EquationEquation (1)
(1)
(1) and generation of an acidic form of the enzyme incorporating a Zn2+(OH2) species at the metal centre, which is catalytically ineffective for the hydration of CO2.Citation6–8,Citation25 In order to regenerate the nucleophilic species, a proton transfer reaction occurs, which is rate determining for the catalytic cycle in many CAs [EquationEquation (2)
(2)
(2) ]. For many human isoforms, this step is assisted by a proton shuttle residue, which is His64 in most mammalian CAs. In the presence of an activator molecule ‘A’, EquationEquation (2)
(2)
(2) becomes EquationEquation (3)
(3)
(3) ; that is, in the enzyme-activator complex the proton transfer reaction is no longer intermolecular but intramolecular, and thus favouredCitation25–28:
(3)
(3)
The imidazole moiety of the key histidine residue, with a pKa of 6.0–7.5 (depending on the isoformCitation6) is an appropriate proton shuttling residue which transfers the proton from the metal coordinated water to the reaction medium, in the crucially important rate-determining step of the catalytic cycleCitation1–3. The process can also be assisted by endogenous molecules, which bind within the enzyme active site, as proven by X-ray crystallography and other techniques, which have been termed CAAsCitation25–28. Such activators facilitate the proton transfer reactions between the metal ion centre and the external medium by an alternative pathway to the proton shuttle residue.
CAAs were recently demonstrated to have potential pharmacologic applications, as the activation of mammalian enzymes was shown to enhance cognition and memory in experimental animalsCitation29, whereas its inhibition had the opposite effectCitation13,Citation14. The activation of CAs from pathogenic bacteria may also be relevant for understanding the factors governing virulence and colonisation of the host, because pH in the tissues surrounding the pathogens likely plays a key role in such processesCitation5,Citation10,Citation12. Considering such evidence, in this study we report the first activation study with amines and amino acids (compounds 1–24, ) of one of the β-CAs reported to be present in the model organism E. coli.
2. Materials and methods
2.1. Enzyme production and purification
The protocol described in Ref.Citation3 has been used to obtain purified recombinant EcoCAβ.
2.2. Ca activity/activation measurements
An Sx.18Mv-R Applied Photophysics (Oxford, UK) stopped-flow instrument has been used to assay the catalytic activity of various CA isozymes for CO2 hydration reactionCitation30. Phenol red (at a concentration of 0.2 mM) was used as indicator, working at the absorbance maximum of 557 nm, with 10 mM Hepes (pH 7.5, for α-CAs)Citation31–34 or TRIS (pH 8.3, for β-CAs)Citation35–38 as buffers, 0.1 M NaClO4 (for maintaining constant ionic strength), following the CA-catalyzed CO2 hydration reaction for a period of 10 s at 25 °C. The CO2 concentrations ranged from 1.7 to 17 mM for the determination of the kinetic parameters and inhibition constants. For each activator, at least six traces of the initial 5–10% of the reaction have been used for determining the initial velocity. The uncatalyzed rates were determined in the same manner and subtracted from the total observed rates. Stock solutions of activators (at 0.1 mM) were prepared in distilled-deionised water and dilutions up to 1 nM were made thereafter with the assay buffer. Enzyme and activator solutions were pre-incubated together for 15 min prior to assay, in order to allow for the formation of the enzyme–activator complexes. The activation constant (KA), defined similarly with the inhibition constant KI, can be obtained by considering the classical Michaelis–Menten equation [EquationEquation (4)(4)
(4) ], which has been fitted by non-linear least squares by using PRISM 3:
(4)
(4)
where [A]f is the free concentration of activator.
Working at substrate concentrations considerably lower than KM ([S] ≪ KM), and considering that [A]f can be represented in the form of the total concentration of the enzyme ([E]t) and activator ([A]t), the obtained competitive steady-state equation for determining the activation constant is given by EquationEquation (5)(5)
(5) :
(5)
(5)
where v0 represents the initial velocity of the enzyme-catalyzed reaction in the absence of activatorCitation35–39. This type of approach to measuring enzyme-ligand interactions is in excellent agreement with recent results from native mass spectrometry measurementsCitation40.
2.3. Reagents
Amines and amino acid derivatives 1–24 were obtained in the highest purity that was available commercially from Sigma-Aldrich (Milan, Italy).
3. Results and discussion
A mentioned in the introduction, the crystal structure of EcoCAβ was reported in 2001Citation2 (), although no kinetic characterisation of the enzyme was reported.
Figure 2. Structure of EcoCAβ (PDB code 1I6OCitation2) (A) Ribbon representation of the dimer. (B) View of the active site. The Zn(II) ion is represented as a magenta sphere. Chain A and chain B are coloured white and green, respectively. Amino acids in the active site are labelled with one letter symbols (blue for chain A and black for chain B): A, Ala; C, Cys; D, Asp; H, His; I, Ile; K, Lys; L, Leu; R, Arg; V, Val; W, Trp; Y, Tyr.
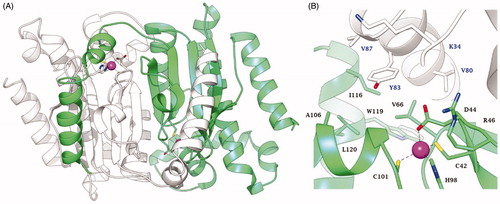
As many other β-CAs have been characterised to date by X-ray crystallographyCitation41, EcoCAβ is a tetrameric enzyme, more precisely a dimer of homodimers. The dimer which constitutes the fundamental element for the structure and functioning of this enzyme is shown in , with the catalytic Zn(II) ion situated at the bottom of a rather long and narrow active siteCitation2. However, when the detailed coordination geometry of the active site was inspected (), a rather surprising situation emerged: the metal ion was observed to be coordinated by four amino acid residues (Cys42, His98, Cys101 and Asp44), with no water molecule coordinated to the Zn2+ to form the zinc-hydroxide nucleophile in the CO2 hydration reaction. Thus, for a rather long period of time the catalytic mechanism of this type of β-CAs (thereafter denominated “closed active site” or “type II β-CAs”) was poorly understood, with various hypothesises being proposed, including that a water molecule acts as the fifth zinc ligand (but was unobserved in the crystal structures), which may eventually be used to form the nucleophileCitation2,Citation42. This mystery has been resolved in a very elegant study by Covarrubias et al.Citation43, who used a β-CA from Mycobacterium tuberculosis, which was crystallised at various pH values. For pH values >8.3, the Zn(II) ion was coordinated by a His residue, two Cys residues and a water molecule/hydroxide ion, whereas at pH values <8, the coordination was as the one shown in , with an Asp replacing the water moleculeCitation43. The active site at higher pH was thus entitled the “open” and that at lower pH was considered ‘closed’Citation43. Furthermore, the mechanism by which the closed active site is opened has also been elucidated: a conserved catalytic dyad comprising an Asp and an Arg residue (Asp44–Arg46 in ) is present in all β-CAs. In the closed active site enzymes, the Asp of the dyad is coordinated to the Zn(II) ion at pH values <8. At higher pH values, the carboxylate moiety of this Asp residue is involved in a strong ionic interaction with the guanidinium moiety of the Arg from the dyad and thus, liberating the coordination position around the zinc ion to allow coordination of a water molecule; that is, the key zinc-hydroxide nucleophile can be formed by the enzyme during catalysis from the open but not the closed active siteCitation43. For this reason, the type II β-CAs are usually catalytically active only at pH values >8.
Thus, we measured the catalytic activity of EcoCAβ at a pH of 8.3 and determined its kinetic constants (kcat and KM) for comparison to those of the thoroughly studied human (h) CA isoforms hCA I and II, belonging to the α-CA class () as well as a recently investigated pathogenic, fungal β-CA, from Malassezia restrica, MreCACitation44. The experiments were also performed in the presence of 10 µM L-Trp as an activator () or in the presence of a sulphonamide inhibitor (data not shown).
Table 1. Activation of human carbonic anhydrase (hCA) isozymes I, II, MreCA and EcoCAβ with L-Trp, at 25 °C, for the CO2 hydration reactionCitation25.
The catalytic activity of EcoCAβ is substantial for the hydration of CO2 to bicarbonate, with a kinetic constant kcat of 5.3 × 105s−1 and a Michaelis–Menten constant KM of 12.9 mM. These kinetic parameters are in fact comparable to those of other α- or β-CAs (). In fact, EcoCAβ has an activity comparable to the human isoform hCA I. The bacterial enzyme has a kcat/Km of 4.10 × 107 M−1 s−1, whereas hCA I has nearly the same ratio (5.0 × 107 M−1 s−1), indicating that the two enzymes have moderate activity overall. Acteazolamide, a sulphonamide standard CAI, inhibited this catalytic activity with a KI of 227 nM (hCA I is inhibited by this compound with a KI of 250 nMCitation6).
The data in also indicates that the presence of L-Trp as an activator does not change the KM for either of the two enzymes belonging to the α-class (hCA I/II) as well as for MreCA and EcoCAβ, a situation also observed for all CA classes for which CA activators have been investigated so farCitation8,Citation31–38. In fact, as proven by kinetic and crystallographic dataCitation3,Citation20, the activator binds in a different region of the active site than the site of substrate binding. Thus, the activator does not influence KM but has an effect only on kcat. Indeed, a 10 µM concentration of L-Trp leads to a 3.4-fold enhancement of the kinetic constant of EcoCAβ compared to the same parameter in the absence of the activator (). For hCA I and II, the enhancement of the kinetic constant in the presence of L-Trp was rather modest, as these enzymes have a weaker affinity for this activator (). On the other hand, L-Trp has a low micromolar affinity for EcoCAβ which explains its more effective activating effect on this enzyme.
Thus, we proceeded with the investigation of activators 1–24 () belonging to the amino acid and amine chemotypes for understanding their ability to activate EcoCAβ. In , the activation constants of these compounds against the target enzyme EcoCAβ as well as hCA II and II (α-CA enzymes) and MreCA (a fungal β-CA) are shown. The following structure-activity relationship (SAR) was observed for the activation of EcoCAβ:
Table 2. Activation constants of hCA I, hCA II and the fungal enzyme MreCA from M. resticta and EcoCAβ (E. coli) with amino acids and amines 1–24, by a stopped-flow CO2 hydrase assayCitation25.
(i) A rather weak activation was observed with L-His, 2-pyridyl-methylamine 15, L-Asn and L-Gln, which had activation constants KAs from 36.0 to 49.5 µM.
(ii) Medium potency activating effects were observed for the following derivatives: D-His, L- and D-Phe, L- and D-Trp, D-Tyr, histamine, dopamine, 2-(aminoethyl)pyridine/piperazine/morpholine (compounds 16–18), L-Asp, L- and D-Glu. These derivatives have KAs from 11.3 to 23.7 µM (). These activators belong to the heterogeneous classes of amines and amino acids, with both aromatic, heterocyclic and aliphatic representatives in both series. Thus, the SAR is rather challenging to delineate definitively.
(iii) The most effective EcoCAβ activators were L- and D-DOPA, L-Tyr, 4-amino-Phe 11, serotonin and L-adrenaline, which had KAs from 2.76 to 10.7 µM. The most effective compounds of this subseries were serotonin (KA of 2.76 µM) and D-DOPA (KA of 3.14 µM). In addition, for the enantiomers of this amino acid, the D-enantiomer was 3.4-times more effective as an activator than the L-enantiomer.
The mechanism of action of these CAAs probably involves the facilitation of the rate-determining step of the catalytic cycle [EquationEquation (3)(3)
(3) ], as for the α-CAsCitation8. However, no X-ray crystal structures of the activator-enzyme complexes have been characterised so far for the β-CAs. The proton shuttling residue for this class of enzymes is yet to be definitively established, but as shown in , the long channel which constitutes the active site of β-CAs would easily accommodate such small molecules as the amines and amino acids investigated here, providing in this way a more efficient proton shuttling between the zinc-coordinated water molecule and the aqueous environment.
4. Conclusions
The β-CA from the widespread bacterium Escherichia coli EcoCAβ has been investigated here for the first time for its catalytic properties as well as for enzymatic activation by a panel of amino acids and amines. EcoCAβ has substantial catalytic activity for the hydration of CO2 to bicarbonate, with a kinetic constant kcat of 5.3 × 105 s−1 and a Michaelis–Menten constant KM of 12.9 mM. The most effective EcoCAβ activators were L- and D-DOPA, L-Tyr, 4-amino-Phe, serotonin and L-adrenaline (KAs from 2.76 to 10.7 µM). Weak activation was observed for L-His, 2-pyridyl-methylamine, L-Asn and L-Gln, with activation constants KAs in the range of 36.0–49.5 µM. D-His, L- and D-Phe, L- and D-Trp, D-Tyr, histamine, dopamine, 2-(aminoethyl)pyridine/piperazine/morpholine, L-Asp, L- and D-Glu showed KAs in the range of 11.3–23.7 µM. CA activators may play a role in bacterial virulence and colonisation of the host, although no detailed studies in this area are available to date.
Acknowledgements
WAD and CTS thank the Australian Research Council for funding.
Disclosure statement
The authors have no relevant affiliations of financial involvement with any organisation or entity with a financial interest in or financial conflict with the subject matter or materials discussed in the manuscript.
Additional information
Funding
References
- a) Guilloton MB, Korte JJ, Lamblin AF, et al. Carbonic anhydrase in Escherichia coli. A product of the cyn operon. J Biol Chem 1992;267:3731–4. b) Guilloton MB, Lamblin AF, Kozliak EI, et al. A physiological role for cyanate-induced carbonic anhydrase in Escherichia coli. J Bacteriol 1993;175:1443–51.
- Cronk JD, Endrizzi JA, Cronk MR, et al. Crystal structure of E. coli beta-carbonic anhydrase, an enzyme with an unusual pH-dependent activity. Protein Sci 2001;10:911–22.
- Cronk JD, Rowlett RS, Zhang KY, et al. Identification of a novel noncatalytic bicarbonate binding site in eubacterial beta-carbonic anhydrase. Biochemistry 2006;45:4351–61.
- Merlin C, Masters M, McAteer S, Coulson A. Why is carbonic anhydrase essential to Escherichia coli? J Bacteriol 2003;185:6415–24.
- Capasso C, Supuran CT. An overview of the alpha-, beta- and gamma-carbonic anhydrases from Bacteria: can bacterial carbonic anhydrases shed new light on evolution of bacteria?. J Enzyme Inhib Med Chem 2015;30:325–32. b) Smith KS, Jakubzick C, Whittam TS, Ferry JG. Carbonic anhydrase is an ancient enzyme widespread in prokaryotes. Proc Natl Acad Sci USA 1999;96:15184–9.
- a) Supuran CT. Carbonic anhydrases: novel therapeutic applications for inhibitors and activators. Nat Rev Drug Discov 2008;7:168–81. b) Nocentini A, Supuran CT. Advances in the structural annotation of human carbonic anhydrases and impact on future drug discovery. Expert Opin Drug Discov 2019;14:1175–97.
- a) Supuran CT. How many carbonic anhydrase inhibition mechanisms exist?. J Enzyme Inhib Med Chem 2016;31:345–60. b) Supuran CT. Advances in structure-based drug discovery of carbonic anhydrase inhibitors. Expert Opin Drug Discov 2017;12:61–88. c) Supuran CT. Structure and function of carbonic anhydrases. Biochem J 2016;473:2023–32.
- a) Supuran CT. Carbonic anhydrase activators. Future Med Chem 2018;10:561–73. b) Temperini C, Scozzafava A, Supuran CT. Carbonic anhydrase activation and the drug design. Curr Pharm Des 2008;14:708–15. c) Akocak S, Supuran CT. Activation of α-, β-, γ- δ-, ζ- and η- class of carbonic anhydrases with amines and amino acids: a review. J Enzyme Inhib Med Chem 2019;34:1652–9. d) Angeli A, Vaiano F, Mari F, et al. Psychoactive substances belonging to the amphetamine class potently activate brain carbonic anhydrase isoforms VA, VB, VII, and XII. J Enzyme Inhib Med Chem 2017;32:1253–9.
- a) Zoccola D, Innocenti A, Bertucci A, et al. Coral carbonic anhydrases: regulation by ocean acidification. Mar Drugs 2016;14:109. b) Bertucci A, Moya A, Tambutté S, et al. Carbonic anhydrases in anthozoan corals-a review. Bioorg Med Chem 2013;21:1437–50. c) Del Prete S, Vullo D, Zoccola D, et al. Activation profile analysis of CruCA4, an α-carbonic anhydrase involved in skeleton formation of the Mediterranean red coral, Corallium rubrum. Molecules 2017;23:66. d) Dathan NA, Alterio V, Troiano E, et al. Biochemical characterization of the chloroplastic β-carbonic anhydrase from Flaveria bidentis (L.) "Kuntze"”. J Enzyme Inhib Med Chem 2014;29:500–4.
- a) Pander B, Harris G, Scott D, et al. The carbonic anhydrase of Clostridium autoethanogenum represents a new subclass of β-carbonic anhydrases. Appl Microbiol Biotechnol 2019;103:7275–86. b) Vullo D, Kumar RSS, Scozzafava A, et al. Sulphonamide inhibition studies of the β-carbonic anhydrase from the bacterial pathogen Clostridium perfringens. J Enzyme Inhib Med Chem 2018;33:31–6.
- a) Supuran CT, Capasso C. The η-class carbonic anhydrases as drug targets for antimalarial agents. Expert Opin Ther Targets 2015;19:551–63. b) Capasso C, Supuran CT. Bacterial, fungal and protozoan carbonic anhydrases as drug targets. Expert Opin Ther Targets 2015;19:1689–704. c) Supuran CT, Capasso C. Biomedical applications of prokaryotic carbonic anhydrases. Expert Opin Ther Pat 2018;28:745–54.
- Del Prete S, Nocentini A, Supuran CT, Capasso C. Bacterial ι-carbonic anhydrase: a new active class of carbonic anhydrase identified in the genome of the Gram-negative bacterium Burkholderia territorii. J Enzyme Inhib Med Chem 2020;35:1060–8.
- a) Supuran CT. Applications of carbonic anhydrases inhibitors in renal and central nervous system diseases. Expert Opin Ther Pat 2018;28:713–21. b) Supuran CT. Carbonic anhydrase inhibitors and their potential in a range of therapeutic areas. Expert Opin Ther Pat 2018;28:709–12. c) Supuran CT. Carbonic anhydrase inhibitors as emerging agents for the treatment and imaging of hypoxic tumors. Expert Opin Investig Drugs 2018;27:963–70. d) Nocentini A, Supuran CT. Carbonic anhydrase inhibitors as antitumor/antimetastatic agents: a patent review (2008–2018). Expert Opin Ther Pat 2018;28:729–40. e) Dogné JM, Hanson J, Supuran C, Pratico D. Coxibs and cardiovascular side-effects: from light to shadow. Curr Pharm Des 2006;12:971–5.
- a) Ozensoy Guler O, Capasso C, Supuran CT. A magnificent enzyme superfamily: carbonic anhydrases, their purification and characterization. J Enzyme Inhib Med Chem 2016;31:689–94. b) De Simone G, Supuran CT. (In)organic anions as carbonic anhydrase inhibitors. J Inorg Biochem 2012;111:117–29. c) Supuran CT. Carbonic anhydrase inhibition and the management of hypoxic tumors. Metabolites 2017;7:E48. d) Bertol E, Vaiano F, Mari F, et al. Advances in new psychoactive substances identification: the U.R.I.To.N. consortium. J Enzyme Inhib Med Chem 2017;32:841–9. e) Boztaş M, Çetinkaya Y, Topal M, et al. Synthesis and carbonic anhydrase isoenzymes I, II, IX, and XII inhibitory effects of dimethoxybromophenol derivatives incorporating cyclopropane moieties. J Med Chem 2015;58:640–50.
- a) Supuran CT. Carbonic anhydrases and metabolism. Metabolites 2018;8:25. b) Supuran CT. Exploring the multiple binding modes of inhibitors to carbonic anhydrases for novel drug discovery. Expert Opin Drug Discov 2020;15:671–86.
- a) Alterio V, Esposito D, Monti SM, et al. Crystal structure of the human carbonic anhydrase II adduct with 1-(4-sulfamoylphenyl-ethyl)-2,4,6-triphenylpyridinium perchlorate, a membrane-impermeant, isoform selective inhibitor. J Enzyme Inhib Med Chem 2018;33:151–7. b) Innocenti A, Vullo D, Scozzafava A, et al. Carbonic anhydrase inhibitors: inhibition of mammalian isoforms I-XIV with a series of substituted phenols including paracetamol and salicylic acid. Bioorg Med Chem 2008;16:7424–8. c) Nocentini A, Bonardi A, Gratteri P, et al. Steroids interfere with human carbonic anhydrase activity by using alternative binding mechanisms. J Enzyme Inhib Med Chem 2018;33:1453–9. d) Tars K, Vullo D, Kazaks A, et al. Sulfocoumarins (1,2-benzoxathiine-2,2-dioxides): a class of potent and isoform-selective inhibitors of tumor-associated carbonic anhydrases. J Med Chem 2013;56:293–300.
- a) Alterio V, Di Fiore A, D’Ambrosio K, et al. Multiple binding modes of inhibitors to carbonic anhydrases: how to design specific drugs targeting 15 different isoforms? Chem Rev 2012;112:4421–68. b) Briganti F, Pierattelli R, Scozzafava A, Supuran CT. Carbonic anhydrase inhibitors. Part 37. Novel classes of carbonic anhydrase inhibitors and their interaction with the native and cobalt-substituted enzyme: kinetic and spectroscopic investigations. Eur J Med Chem 1996;31:1001–10. c) Neri D, Supuran CT. Interfering with pH regulation in tumours as a therapeutic strategy. Nat Rev Drug Discov 2011;10:767–77. d) Supuran CT, Vullo D, Manole G, et al. Designing of novel carbonic anhydrase inhibitors and activators. Curr Med Chem Cardiovasc Hematol Agents 2004;2:49–68.
- a) McDonald PC, Chia S, Bedard PL, et al. A phase 1 study of SLC-0111, a novel inhibitor of carbonic anhydrase IX, in patients with advanced solid tumors. Am J Clin Oncol 2020 (in press). b) Lou Y, McDonald PC, Oloumi A, et al. Targeting tumor hypoxia: suppression of breast tumor growth and metastasis by novel carbonic anhydrase IX inhibitors. Cancer Res 2011;71:3364–76. c) Pacchiano F, Aggarwal M, Avvaru BS, et al. Selective hydrophobic pocket binding observed within the carbonic anhydrase II active site accommodate different 4-substituted-ureido-benzenesulfonamides and correlate to inhibitor potency. Chem Commun (Camb) 2010;46:8371–3. d) Köhler K, Hillebrecht A, Schulze Wischeler J, et al. Saccharin inhibits carbonic anhydrases: possible explanation for its unpleasant metallic aftertaste. Angew Chem Int Ed Engl 2007;46:7697–9.
- a) Krall N, Pretto F, Decurtins W, et al. A small-molecule drug conjugate for the treatment of carbonic anhydrase IX expressing tumors. Angew Chem Int Ed Engl 2014;53:4231–5. b) Abo-Ashour MF, Eldehna WM, Nocentini A, et al. Novel synthesized SLC-0111 thiazole and thiadiazole analogues: Determination of their carbonic anhydrase inhibitory activity and molecular modeling studies. Bioorg Chem 2019;87:794–802.
- a) Del Prete S, Vullo D, Scozzafava A, et al. Cloning, characterization and anion inhibition study of the δ-class carbonic anhydrase (TweCA) from the marine diatom Thalassiosira weissflogii. Bioorg Med Chem 2014;22:531–7. b) Vullo D, Del Prete S, Osman SM, et al. Sulfonamide inhibition studies of the δ-carbonic anhydrase from the diatom Thalassiosira weissflogii. Bioorg Med Chem Lett 2014;24:275–9. c) Del Prete S, Vullo D, De Luca V, et al. Biochemical characterization of the δ-carbonic anhydrase from the marine diatom Thalassiosira weissflogii, TweCA. J Enzyme Inhib Med Chem 2014;29:906–11.
- a) Supuran CT, Capasso C. An overview of the bacterial carbonic anhydrases. Metabolites 2017;7:56. b) Supuran CT. Carbon- versus sulphur-based zinc binding groups for carbonic anhydrase inhibitors? J Enzyme Inhib Med Chem 2018;33:485–95. c) Rahman MM, Tikhomirova A, Modak JK, et al. Antibacterial activity of ethoxzolamide against Helicobacter pylori strains SS1 and 26695. Gut Pathog 2020;12:20.
- a) Del Prete S, Vullo D, Fisher GM, et al. Discovery of a new family of carbonic anhydrases in the malaria pathogen Plasmodium falciparum-the η-carbonic anhydrases. Bioorg Med Chem Lett 2014;24:4389–96. b) De Simone G, Di Fiore A, Capasso C, Supuran CT. The zinc coordination pattern in the η-carbonic anhydrase from Plasmodium falciparum is different from all other carbonic anhydrase genetic families. Bioorg Med Chem Lett 2015;25:1385–9. c) Hewitson KS, Vullo D, Scozzafava A, et al. Molecular cloning, characterization, and inhibition studies of a β-carbonic anhydrase from Malassezia globosa, a potential antidandruff target. J Med Chem 2012;55:3513–20. d) De Prete S, Vullo D, Osman SM, et al. Anion inhibitors of the β-carbonic anhydrase from the pathogenic bacterium responsible of tularemia, Francisella tularensis. Bioorg Med Chem 2017;25:4800–4. e) Bua S, Bozdag M, Del Prete S, et al. Mono- and di-thiocarbamate inhibition studies of the δ-carbonic anhydrase TweCAδ from the marine diatom Thalassiosira weissflogii. J Enzyme Inhib Med Chem 2018;33:707–13. f) Vullo D, Del Prete S, Di Fonzo P, et al. Comparison of the sulfonamide inhibition profiles of the β- and γ-carbonic anhydrases from the pathogenic bacterium Burkholderia pseudomallei. Molecules 2017;22:421. g) Del Prete S, Vullo D, Di Fonzo P, et al. Sulfonamide inhibition profile of the γ-carbonic anhydrase identified in the genome of the pathogenic bacterium Burkholderia pseudomallei the etiological agent responsible of melioidosis. Bioorg Med Chem Lett 2017;27:490–5. h) Vullo D, Lehneck R, Donald WA, et al. Sulfonamide inhibition studies of the β-class carbonic anhydrase CAS3 from the filamentous Ascomycete Sordaria macrospora. Molecules 2020;25:1036. i) Vullo D, Lehneck R, Donald WA, et al. Anion inhibition studies of the β-class carbonic anhydrase CAS3 from the filamentous Ascomycete Sordaria macrospora. Metabolites 2020;10:93.
- a) Akocak S, Lolak N, Bua S, et al. Discovery of novel 1,3-diaryltriazene sulfonamides as carbonic anhydrase I, II, VII, and IX inhibitors. J Enzyme Inhib Med Chem 2018;33:1575–80. b) Milite C, Amendola G, Nocentini A, et al. Novel 2-substituted-benzimidazole-6-sulfonamides as carbonic anhydrase inhibitors: synthesis, biological evaluation against isoforms I, II, IX and XII and molecular docking studies. J Enzyme Inhib Med Chem 2019;34:1697–710. c) Distinto S, Meleddu R, Ortuso F, et al. Exploring new structural features of the 4-[(3-methyl-4-aryl-2,3-dihydro-1,3-thiazol-2-ylidene)amino]benzenesulphonamide scaffold for the inhibition of human carbonic anhydrases. J Enzyme Inhib Med Chem 2019;34:1526–33.
- a) Rotondi G, Guglielmi P, Carradori S, et al. Design, synthesis and biological activity of selective hCAs inhibitors based on 2-(benzylsulfinyl)benzoic acid scaffold. J Enzyme Inhib Med Chem 2019;34:1400–13. b) Gitto R, De Luca L, Mancuso F, et al. Seeking new approach for therapeutic treatment of cholera disease via inhibition of bacterial carbonic anhydrases: experimental and theoretical studies for sixteen benzenesulfonamide derivatives. J Enzyme Inhib Med Chem 2019;34:1186–92. c) Nocentini A, Osman SM, Almeida IA, et al. Appraisal of anti-protozoan activity of nitroaromatic benzenesulfonamides inhibiting carbonic anhydrases from Trypanosoma cruzi and Leishmania donovani. J Enzyme Inhib Med Chem 2019;34:1164–71.
- Briganti F, Mangani S, Orioli P, et al. Carbonic anhydrase activators: X-ray crystallographic and spectroscopic investigations for the interaction of isozymes I and II with histamine. Biochemistry 1997;36:10384–92.
- a) Temperini C, Scozzafava A, Vullo D, Supuran CT. Carbonic anhydrase activators. Activation of isozymes I, II, IV, VA, VII, and XIV with l- and d-histidine and crystallographic analysis of their adducts with isoform II: engineering proton-transfer processes within the active site of an enzyme. Chemistry 2006;12:7057–66. b) Temperini C, Scozzafava A, Vullo D, Supuran CT. Carbonic anhydrase activators. Activation of isoforms I, II, IV, VA, VII, and XIV with L- and D-phenylalanine and crystallographic analysis of their adducts with isozyme II: stereospecific recognition within the active site of an enzyme and its consequences for the drug design. J Med Chem 2006;49:3019–27. c) Temperini C, Innocenti A, Scozzafava A, Supuran CT. Carbonic anhydrase activators: kinetic and X-ray crystallographic study for the interaction of D- and L-tryptophan with the mammalian isoforms I-XIV. Bioorg Med Chem 2008;16:8373–8. d) Temperini C, Innocenti A, Scozzafava A, et al. Carbonic anhydrase activators: L-Adrenaline plugs the active site entrance of isozyme II, activating better isoforms I, IV, VA, VII, and XIV. Bioorg Med Chem Lett 2007;17:628–35.
- a) Akocak S, Lolak N, Vullo D, et al. Synthesis and biological evaluation of histamine Schiff bases as carbonic anhydrase I, II, IV, VII, and IX activators. J Enzyme Inhib Med Chem 2017;32:1305–12. b) Akocak S, Lolak N, Bua S, et al. α-Carbonic anhydrases are strongly activated by spinaceamine derivatives. Bioorg Med Chem 2019;27:800–4. c) Akocak S, Lolak N, Bua S, et al. Activation of human α-carbonic anhydrase isoforms I, II, IV and VII with bis-histamine Schiff bases and bis-spinaceamine substituted derivatives. J Enzyme Inhib Med Chem 2019;34:1193–8. d) Dave K, Scozzafava A, Vullo D, et al. Pyridinium derivatives of histamine are potent activators of cytosolic carbonic anhydrase isoforms I, II and VII. Org Biomol Chem 2011;9:2790–800. e) Dave K, Ilies MA, Scozzafava A, et al. An inhibitor-like binding mode of a carbonic anhydrase activator within the active site of isoform II. Bioorg Med Chem Lett 2011;21:2764–8.
- a) Bhatt A, Mondal UK, Supuran CT, et al. Crystal structure of carbonic anhydrase II in complex with an activating ligand: implications in neuronal function. Mol Neurobiol 2018;55:7431–7. b) Temperini C, Scozzafava A, Supuran CT. Carbonic anhydrase activators: the first X-ray crystallographic study of an adduct of isoform I. Bioorg Med Chem Lett 2006;16:5152–6. c) Temperini C, Scozzafava A, Puccetti L, Supuran CT. Carbonic anhydrase activators: X-ray crystal structure of the adduct of human isozyme II with L-histidine as a platform for the design of stronger activators. Bioorg Med Chem Lett 2005;15:5136–41. d) Clare BW, Supuran CT. Carbonic anhydrase activators. 3: structure-activity correlations for a series of isozyme II activators . J Pharm Sci 1994;83:768–73.
- a) Canto de Souza L, Provensi G, Vullo D, et al. Carbonic anhydrase activation enhances object recognition memory in mice through phosphorylation of the extracellular signal-regulated kinase in the cortex and the hippocampus. Neuropharmacology 2017;118:148–56. b) Sanku RKK, John JS, Ilies MA, Walker EA. Potential learning and memory disruptors and enhancers in a simple, 1-day operant task in mice. Behav Pharmacol 2018;29:482–92. c) Wang X, Schröder HC, Schlossmacher U, et al. Modulation of the initial mineralization process of SaOS-2 cells by carbonic anhydrase activators and Polyphosphate. Calcif Tissue Int 2014;94:495–509.
- Khalifah RG. The carbon dioxide hydration activity of carbonic anhydrase. I. Stop-flow kinetic studies on the native human isoenzymes B and C. J Biol Chem 1971;246:2561–73.
- a) Vullo D, Innocenti A, Nishimori I, et al. Carbonic anhydrase activators: activation of the human isoforms VII (cytosolic) and XIV (transmembrane) with amino acids and amines. Bioorg Med Chem Lett 2007;17:4107–12. b) Pastorekova S, Vullo D, Nishimori I, et al. Carbonic anhydrase activators: Activation of the human tumor-associated isozymes IX and XII with amino acids and amines. Bioorg Med Chem 2008;16:3530–6. c) Innocenti A, Hilvo M, Parkkila S, et al. Carbonic anhydrase activators. Activation of the membrane-associated isoform XV with amino acids and amines . Bioorg Med Chem Lett 2009;19:3430–3.
- a) Innocenti A, Zimmerman SA, Scozzafava A, et al. Carbonic anhydrase activators: activation of the archaeal beta-class (Cab) and gamma-class (Cam) carbonic anhydrases with amino acids and amines. Bioorg Med Chem Lett 2008;18:6194–8. b) Vullo D, Del Prete S, Capasso C, Supuran CT. Carbonic anhydrase activators: activation of the β-carbonic anhydrase from Malassezia globosa with amines and amino acids. Bioorg Med Chem Lett 2016;26:1381–5. c) Isik S, Kockar F, Aydin M, et al. Carbonic anhydrase activators: activation of the beta-carbonic anhydrase Nce103 from the yeast Saccharomyces cerevisiae with amines and amino acids . Bioorg Med Chem Lett 2009;19:1662–5.
- Innocenti A, Leewattanapasuk W, Manole G, et al. Carbonic anhydrase activators: activation of the beta-carbonic anhydrase from the pathogenic yeast Candida glabrata with amines and amino acids. Bioorg Med Chem Lett 2010;20:1701–4.
- a) Angeli A, Del Prete S, Osman SM, et al. Activation studies of the α- and β-carbonic anhydrases from the pathogenic bacterium Vibrio cholerae with amines and amino acids. J Enzyme Inhib Med Chem 2018;33:227–33. b) Angeli A, Del Prete S, Donald WA, et al. The γ-carbonic anhydrase from the pathogenic bacterium Vibrio cholerae is potently activated by amines and amino acids. Bioorg Chem 2018;77:1–5. c) Angeli A, Del Prete S, Osman SM, et al. Activation studies with amines and amino acids of the β-carbonic anhydrase encoded by the Rv3273 gene from the pathogenic bacterium Mycobacterium tuberculosis. J Enzyme Inhib Med Chem 2018;33:364–9.
- a) Angeli A, Del Prete S, Pinteala M, et al. The first activation study of the β-carbonic anhydrases from the pathogenic bacteria Brucella suis and Francisella tularensis with amines and amino acids. J Enzyme Inhib Med Chem 2019;34:1178–85. b) Stefanucci A, Angeli A, Dimmito MP, et al. Activation of β- and γ-carbonic anhydrases from pathogenic bacteria with tripeptides. J Enzyme Inhib Med Chem 2018;33:945–50. c) Bua S, Haapanen S, Kuuslahti M, et al. Activation studies of the β-carbonic anhydrase from the pathogenic protozoan Entamoeba histolytica with amino acids and amines. Metabolites 2019;9:26–33.
- a) Vullo D, Del Prete S, Osman SM, et al. Burkholderia pseudomallei γ-carbonic anhydrase is strongly activated by amino acids and amines. Bioorg Med Chem Lett 2017;27:77–80. b) Vullo D, Del Prete S, Osman SM, et al. Comparison of the amine/amino acid activation profiles of the β- and γ-carbonic anhydrases from the pathogenic bacterium Burkholderia pseudomallei. J Enzyme Inhib Med Chem 2018;33:25–30.
- a) Angeli A, Del Prete S, Osman SM, et al. Activation studies of the γ-carbonic anhydrases from the Antarctic marine bacteria Pseudoalteromonas haloplanktis and Colwellia psychrerythraea with amino acids and amines. Marine Drugs 2019;17:238–46. b) Angeli A, Alasmary FAS, Del Prete S, et al. The first activation study of a δ-carbonic anhydrase: TweCAδ from the diatom Thalassiosira weissflogii is effectively activated by amines and amino acids. J Enzyme Inhib Med Chem 2018;33:680–5. c) Angeli A, Buonanno M, Donald WA, et al. The zinc - but not cadmium - containing ζ-carbonic from the diatom Thalassiosira weissflogii is potently activated by amines and amino acids . Bioorg Chem 2018;80:261–5.
- a) Angeli A, Del Prete S, Alasmary FAS, et al. The first activation studies of the η-carbonic anhydrase from the malaria parasite Plasmodium falciparum with amines and amino acids. Bioorg Chem 2018;80:94–8. b) Angeli A, Donald WA, Parkkila S, Supuran CT. Activation studies with amines and amino acids of the β-carbonic anhydrase from the pathogenic protozoan Leishmania donovani chagasi. Bioorg. Chem 2018;78:406–10.
- a) Capasso C, Supuran CT, Protozoan, fungal and bacterial carbonic anhydrases targeting for obtaining antiinfectives. In: Supuran CT, Capasso C, eds. Targeting carbonic anhydrases. London: Future Science Ltd. 2014:133–141. b) Schlicker C, Hall RA, Vullo D, et al. Structure and inhibition of the CO2-sensing carbonic anhydrase Can2 from the pathogenic fungus Cryptococcus neoformans. J Mol Biol 2009;385:1207–20.
- a) Nguyen GTH, Tran TN, Podgorski MN, et al. Nanoscale ion emitters in native mass spectrometry for measuring ligand-protein binding affinities. ACS Cent Sci 2019;5:308–18. b) Nguyen GTH, Nocentini A, Angeli A, et al. Perfluoroalkyl substances of significant environmental concern can strongly inhibit human carbonic anhydrase isozymes. Anal Chem 2020;92:4614–22.
- a) Ferraroni M, Del Prete S, Vullo D, et al. Crystal structure and kinetic studies of a tetrameric type II β-carbonic anhydrase from the pathogenic bacterium Vibrio cholerae. Acta Crystallogr D Biol Crystallogr 2015;71:2449–56. b) Lehneck R, Neumann P, Vullo D, et al. Crystal structures of two tetrameric β-carbonic anhydrases from the filamentous ascomycete Sordaria macrospora. Febs J 2014;281:1759–72. c) Huang S, Hainzl T, Grundstrom C, et al. Structural studies of β-carbonic anhydrase from the green alga Coccomyxa: inhibitor complexes with anions and acetazolamide. PLoS One 2011;6:e28458.
- Mitsuhashi S, Mizushima T, Yamashita E, et al. X-ray structure of beta-carbonic anhydrase from the red alga, Porphyridium purpureum, reveals a novel catalytic site for CO(2) hydration. J Biol Chem 2000;275:5521–6.
- Covarrubias AS, Bergfors T, Jones TA, Högbom M. Structural mechanics of the pH-dependent activity of beta-carbonic anhydrase from Mycobacterium tuberculosis. J Biol Chem 2006;281:4993–9.
- Angeli A, Prete SD, Ghobril C, et al. Activation studies of the β-carbonic anhydrases from Malassezia restricta with amines and amino acids. J Enzyme Inhib Med Chem 2020;35:824–30.