Abstract
A small library of coumarin and their psoralen analogues EMAC10157a-b-d-g and EMAC10160a-b-d-g has been designed and synthesised to investigate the effect of structural modifications on their inhibition ability and selectivity profile towards carbonic anhydrase isoforms I, II, IX, and XII. None of the new compounds exhibited activity towards hCA I and II isozymes. Conversely, both coumarin and psoralen derivatives were active against tumour associated isoforms IX and XII in the low micromolar or nanomolar range of concentration. These data further corroborate our previous findings on analogous derivatives, confirming that both coumarins and psoralens are interesting scaffolds for the design of isozyme selective hCA inhibitors.
Introduction
The development of cancer is a complex multifactorial process, involving many cellular adaptations and signal transduction pathwaysCitation1–4. In solid tumours, cancer cells must survive in a low oxygen concentration environment, due to the rapid cellular proliferation and to the impossibility to promptly supply an adequate vascularisationCitation5,Citation6. Indeed, many pathways are involved in the hypoxia survival mechanismCitation7–11 and they all concur in helping cancer cells to escape from apoptosis. These pathways have been investigated in depth and might be inhibited by relatively new classes of anticancer drugs, to contrast the angiogenesis process, such as VEGFR (sunitinib, sorafenib), VEGF directed monoclonal antibodies (bevacizumab), and mTOR (everolimus, temsirolimus) inhibitorsCitation12–17. In this contest, the key role of human carbonic anhydrases, a class of metalloproteins that catalyse the reversible conversion of carbon dioxide to bicarbonate and protonsCitation18–20, has been outlined. In particular, two membrane isoforms, namely hCA IX and XII, are mainly involved in cancer proliferation and invasionCitation21–31. Not surprisingly, several inhibitors of membrane bounded hCA isozymes, with diverse structures and mechanisms of action, have been designed and investigated so far. In this respect, both synthetic and natural coumarin derivatives have already demonstrated to possess high selectivity and activity towards specific hCA isozymesCitation32–36. Moreover, their interaction and binding mode on this class of metalloenzymes have been investigated in depthCitation37,Citation38. Besides, it should be considered that coumarins have been reported to interact with several cancer druggable targets. In particular, coumarin derivatives have shown a variety of biological activities such as CK2 inhibitorsCitation39, EGFRCitation40, PI3K-AKT-mTOR signalling inhibitorsCitation41–43. Furthermore, their anticancer potential, tumour targets, diverse mechanisms of action as well as their advantages and disadvantages have been recently reviewedCitation44. In continuation with our previous work and prompted by these considerations, to further explore the influences of structural modifications on the coumarin and psoralene core on the activity and selectivity towards membrane-bound hCA isozyme, we have designed and synthesised a small library of methyl-2-[4-methyl-2-oxo-7–(2-oxo-2-arylethoxy)-8-propylchromen-3-yl]acetate and 2–(5-methyl-7-oxo-3-aryl-9-propyl-7H-furo[3,2-g]chromen-6-yl)acetic acid derivatives were a propyl group in the position 8 or 9 has been introduced compared to the previously synthesised derivatives.
Methods
Materials and apparatus
Starting materials and reagents were obtained from commercial suppliers and were used without purification. All melting points were determined on a Stuart SMP11 melting points apparatus and are uncorrected. Melting points, the yield of reactions, and analytical data of derivatives EMAC10157a-b-d-g and EMAC10160a-b-d-g are reported in .
Table 1. Chemical, analytical, and physical data of derivatives EMAC10157 a-b-d-g and EMAC10160 a-b-d-g.
1H-NMR and 13C-NMR spectra () were registered on a Bruker AMX 400 MHz (chemical shifts in δ values) operating at 400 MHz and 100 MHz, respectively. All samples were measured in DMSO. Chemical shifts are reported referenced to the solvent in which they were measured. Coupling constants J are expressed in hertz (Hz). Elemental analyses were obtained on a Perkin–Elmer 240 B microanalyser. Analytical data of the synthesised compounds are in agreement within ±0.4% of the theoretical values. TLC chromatography was performed using silica gel plates (Merck F 254), spots were visualised by UV light.
Table 2. 1H NMR and 13C NMR data of derivatives EMAC10157a-b-d-g and EMAC10160a-b-d-g.
General procedure for the synthesis of compound EMAC10157 a-b-d-g and EMAC10160 a-b-d-g
Synthesis of methyl 2–(7-hydroxy-4-methyl-2-oxo-8-propyl-2H-chromen-3-yl)acetate
A mixture of propylresorcinol (1 eq.), dimethylacetylsuccinate (1 eq.) and sulphuric acid 98% (2.8 eq.) was vigorously stirred at room temperature. The progression of the reaction was monitored by TLC, using ethyl acetate/n-hexane 2:1. After 30 min a homogeneous sticky solid was obtained which was dissolved in methanol and poured into ice water. The mixture was stirred until ice melting and then filtered off to obtain a light yellow solid. The crude product was washed with ethyl ether giving a white powder that was crystallised from methanol.
Synthesis of methyl-2-[4-methyl-2-oxo-7–(2-oxo-2-arylethoxyi)-8-propylchromen-3-yl]acetate (EMAC 10157 a-b-d-g)
A hot solution of methyl 2–(7-hydroxy-4-methyl-2-oxo-8-propyl-2H-chromen-3-yl)acetate (1 eq.) in dry acetone was treated with K2CO3 (2.5 eq.), stirred vigorously, and treated with the appropriate α-haloketone (1 eq.). The reaction mixture was heated to reflux and stirred for 1–5 h (course of the reaction monitored by TLC using ethyl acetate/n-hexane 5:1). When the reaction was completed, it was cooled at 0 °C and the solution acidified with HCl conc. The resulting precipitate was filtered off and crystallised if necessary.
Synthesis of 2–(5-methyl-7-oxo-3-aryl-9-propyl-7H-furo[3,2-g]chromen-6-yl)acetic acid (EMAC10160 a-b-d-g)
A solution or suspension of coumarin (1 eq., EMAC10157 a-b-d-g) in propan-2-ol was treated with NaOH solution (4 eq., 1 N). The reaction mixture was heated for 3–4 h, obtaining a dark solution. The solution was cooled to room temperature and poured into ice water. Concentrated HCl was added to the solution, obtaining a suspension that was filtered and crystallised.
Analytical and spectral data of compounds EMAC10157 a-b-d-g and EMAC10160 a-b-d-g are reported in and . 1H-NMR and 13C-NMR spectra are reported in Supplementary material (Figures S1–S16).
Biological activity
Carbonic anhydrase inhibition assay
The CA catalysed CO2 hydration/inhibition was measured by using a stopped-flow instrument (Applied Photophysics, Oxford, U.K.) as the method described earlierCitation45. Initial rates of the CA-catalysed CO2 hydration reaction were followed for 10–100 s. The CO2 concentrations ranged from 1.7 to 17 mM for the determination of the inhibition constants. For each inhibitor, at least six traces of the initial 5–10% of the reaction were used for assessing the initial velocity. The uncatalyzed rates were subtracted from the total observed rates. Stock solutions of inhibitors (10 mM) and dilutions up to 0.01 nM were prepared in distilled-deionized water. Inhibitor and enzyme solutions were preincubated together for 15 min at room temperature prior to assay, in order to allow for the formation of the E–I complex. The inhibition constants were obtained by non-linear least-squares methods using PRISM 3 as reported earlier, and represent the mean from at least three different determinationsCitation46–48. hCA I, hCA II, hCA IX (catalytic domain), and hCA XII (catalytic domain) were recombinant proteins produced in-house using our standardised protocol and their concentration in the assay system was in the range of 3–10 nM (and even lower for highly effective, sub-nanomolar inhibitors)Citation46–48.
Molecular modelling
Ligand preparation
The ligands were built using the Maestro GUICitation49. The most stable conformation has been determined by molecular mechanics conformational analysis performed by Macromodel software version 9.2Citation50. using the Merck Molecular Force Fields (MMFFs)Citation51 and GB/SA water implicit solvation modelCitation52, Polak–Ribier Conjugate Gradient (PRCG) method, 5000 iterations, and a convergence criterion of 0.05 kcal/(mol Å). All the other parameters were left as default.
Protein preparation
The coordinates for hCA isoforms enzymes were taken from the RCSB Protein Data BankCitation53 (PDB codes 3k34Citation54, for isoform II; 5fl4Citation55, for isoform IX and 5msa, for isoform XII). The proteins were prepared by using the Maestro Protein Preparation WizardCitation49. Original water molecules were removed.
Docking protocol
Molecular docking studies were performed using QMPL workflow protocolCitation56. Grids were defined around the refined structure by centring on crystallised ligands. The other settings were left as default.
Post docking protocol
To better consider, the induced fit phenomena, the most energy favoured generated complexes were fully optimised with the OPLS2005 force field in GB/SA implicit water. The optimisation process was performed setting 10,000 steps interactions up to the derivative convergence criterion equal to 0.05 kJ/(molÅ). The resulting complexes were considered for the binding modes graphical analysis with PymolCitation57 and Maestro.
Results and discussion
As a continuation of our ongoing research in the field of carbonic anhydrase and anticancer agentsCitation58–62 we have synthesised a new series of methyl-2-[4-methyl-2-oxo-7–(2-oxo-2-arylethoxyi)-8-propylchromen-3-yl]acetate and 2–(5-methyl-7-oxo-3-aryl-9-propyl-7H-furo[3,2-g]chromen-6-yl)acetic acid to evaluate their activity and selectivity towards hCA isozymes and to gain information on their structure-activity relationships. The compounds are reported as EMAC10157 a-b-d-g and EMAC10160 a-b-d-g and their structures are illustrated in .
All the synthesised compounds show a propyl substituent in the position 8 or 9 of the coumarin and psoralen nucleus, respectively. As in previously reported derivatives, a methylene carboxylic group was placed in position 3 of the chromene which may lead to the formation of a bidentate chelator of the Zn2+ ion in the catalytic pocket, due to the hCA esterase activityCitation32,Citation34,Citation63 on the dihydropyranone ring, as illustrated in .
Figure 2. (a) Esterase activity of carbonic anhydrase on compound EMAC10157gCitation34. (b, c) Oral bioavailability radar profile.
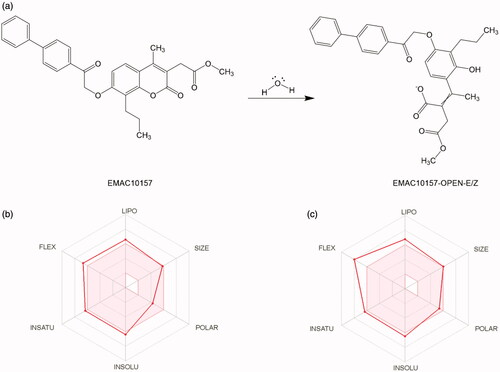
The synthesis of the new derivatives is reported in Scheme 1. It consists of the H2SO4 mediated Pechman condensation of dimethylacetylsuccinate and 2-propylresorcinol at room temperature in solvent-free conditions, followed by a Williamson reaction of the phenolic group in the position 7 of the chromene ring with the appropriate α-haloketone in dry acetone and K2CO3, to generate the asymmetric ether which will lead to the furocoumarin formation by intramolecular electrophilic substitution, mediated by refluxing in NaOH 1 N water solution.
Scheme 1. Synthetic pathway to compounds EMAC10157 a-b-d-g and EMAC10160 a-b-d-g. Reagents and conditions: (i) dimethylacetylsuccinate, H2SO4 98% R.T.; (ii) α-halogeno arylketone, dry acetone, K2CO3, reflux; (iii) NaOH 1 N, reflux.
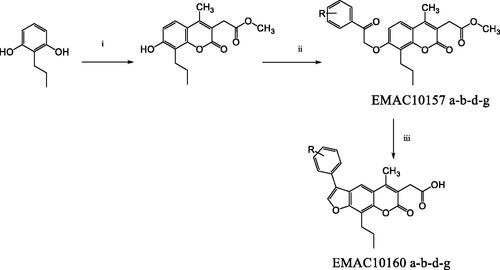
EMAC10157 a-b-d-g-and EMAC10160 a-b-d-g were characterised employing analytical and spectroscopic methods and the results are summarised in and . Compounds were then submitted to enzymatic evaluation towards hCA I, II, IX, and XII. The results are reported in . Interestingly, none of the new EMAC derivatives exhibited any inhibition activity towards hCA I and II isozymes. On the contrary, all of them are submicromolar inhibitors of the hCA membrane isoforms IX and XII. This behaviour is in agreement with our previous findings and with the generally observed selectivity profile of coumarin derivativesCitation32,Citation33,Citation35,Citation37,Citation38,Citation63.
Table 3. Inhibition data towards hCA I, II, IX, and XII of compounds EMAC10157a-b-d-g and EMAC10160 a-b-d-g.
Within the tested compounds, EMAC10157 series was the most active towards the hCA IX and XII isoforms. Therefore, its mechanism of action was investigated in more detail by docking experiments followed by energy minimisation of the obtained complexes.
In particular, considering that an interesting hCA esterase mediated inhibition mechanism was recently reported for coumarin derivativesCitation32,Citation33,Citation63, we firstly investigated this aspect. Hence, the most promising compound, EMAC10157g, was submitted to docking experiments to evaluate if the dihydropyranone ring of the chromene moiety could reach the bottom of the catalytic cavity of hCA II, IX and XII and, therefore, be hydrolysed by the Zn2+ activated water molecule, which acts as a very potent nucleophile ().
Confirming the selectivity profile already observed in previously investigated analogues docking experiment in hCA II enzyme showed that the EMAC10157g tail is too bulky to access hCA II cavity, while, in isoforms IX and XII, the dihydropyranone portion of EMAC10157g was able to bind close enough to the Zn2+ ion ( and ) cofactor to undergo the esterase reaction.
Figure 3. 3D representation of the putative binding mode obtained by docking experiments. (a) hCA-IX – EMAC10157g, (b) hCA-IX – EMAC10157g-openE, and (c) hCA-IX – EMAC10157g-openZ and the relative 2D representation of the complexes stabilising interactions with the binding site residues represented with different colour depending on their chemical-physical properties: green, hydrophobic; cyan, polar; violet, positive; red, negative charged residues; grey, metal atoms. Instead, magenta arrows indicate the formation of a hydrogen bond between protein and ligand, while grey lines indicate the interaction with the complexed ion.
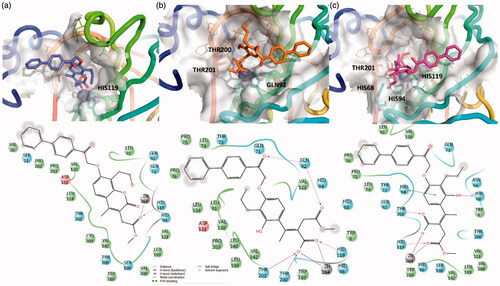
Hence, the two open cinnamic acid derivative () configurations (E/Z) were subjected to docking experiments to predict the putative binding mode of the hydrolysed forms in hCA IX and hCA XII ( and .
Figure 4. 3D representation of the putative binding mode obtained by docking experiments. (a) hCA-XII – EMAC10157g, (b) hCA-XII – EMAC10157g-openE, and (c) CA-XII- EMAC10157g-openZ and the relative 2D representation of the complexes stabilising interactions with the binding site residues.
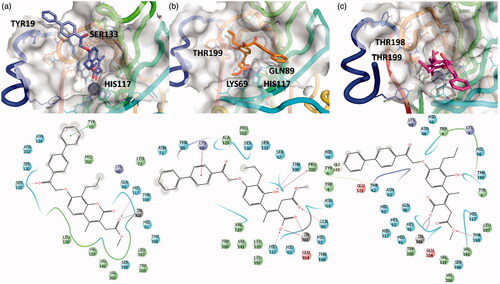
The predicted affinity of the open compounds was estimated to be better than that of the closed ones. What seems important is the Zn2+ chelation and the interactions between the newly formed carboxylate moiety and the methyl ester with the surrounding residues in the catalytic site.
The Y shape of the compound, with propyl and -biphenyl-2oxoethoxy moiety being the arms, helps to maintain the selectivity but, ultimately, decreases the activity, compared to previously investigated psoralensCitation32.
Biphenyl moiety accommodation is well tolerated due to the wide cavity exposed to the solvent, as shown by other hCA inhibitors with bulky tails. In fact, the interactions between this group with adjacent hydrophobic residues stabilise the complexes.
In summary, the computational methods helped to rationalise the good activity of the investigated compound towards the hCA IX and hCA XII isoform and to suggest a reasonable mechanism of action that could be further investigated to be confirmed. If verified, this compound can be considered as a new prodrug candidate with acceptable oral bioavailability properties (), and with good drug-like properties. Nevertheless, considering the encouraging predicted ADME propertiesCitation64 and the activity data, we are further optimising this scaffold.
Conclusions
We have designed and synthesised a series of methyl-2-[4-methyl-2-oxo-7–(2-oxo-2-arylethoxyi)-8-propylchromen-3-yl]acetate and 2–(5-methyl-7-oxo-3-aryl-9-propyl-7H-furo[3,2-g]chromen-6-yl)acetic acid, and evaluate their activity on hCA I, II, IX, and XII isozymes. As a confirmation of the literature reported chromene derivatives selectivity profile, none of the investigated compounds was able to inhibit the off-target I and II isoforms of hCA. On the contrary, they all inhibit the membrane isozymes hCA IX and XII and further corroborate the reported data on chromene derivatives. Considering the acceptable ADME prediction profile and the high potential of coumarin derivatives, not only as hCA selective inhibitors but also as potential multitarget anticancer agents, these data prompted us to further investigate this scaffold to optimise both the activity and the isozyme selectivity.
Supplemental Material
Download PDF (986.8 KB)Acknowledgements
The authors wish to acknowledge the “Ufficio Valorizzazione dei Risultati della Ricerca” of Sardegna Ricerche Technological Park, Pula (CA) – Italy. The authors also acknowledge the PRIN 2017 research project “Novel anticancer agents endowed with multi-targeting mechanism of action” (201744BN5T).
Disclosure statement
No potential conflict of interest was reported by the author(s).
References
- Li S-S, Lian Y-F, Huang Y-L, et al. Overexpressing PLOD family genes predict poor prognosis in gastric cancer. J Cancer 2020;11:121–31.
- Hoxhaj G, Manning BD. The PI3K-AKT network at the interface of oncogenic signalling and cancer metabolism. Nat Rev Cancer 2020;20:74–88.
- Li Z, You Q, Zhang X. Small-molecule modulators of the hypoxia-inducible factor pathway: development and therapeutic applications. J Med Chem 2019;62:5725–49.
- Ferreira BI, Lie MK, Engelsen AST, et al. Adaptive mechanisms of resistance to anti-neoplastic agents. MedChemComm 2017;8:53–66.
- Krzykawska-Serda M, Miller RC, Elas M, et al. Correlation between hypoxia proteins and EPR-detected hypoxia in tumors. Adv Exp med Biol 2017;977:319–325.
- Huang Y, Lin D, Taniguchi CM. Hypoxia inducible factor (HIF) in the tumor microenvironment: friend or foe? Sci China Life Sci 2017;60:1114–24.
- Lee P, Chandel NS, Simon MC. Cellular adaptation to hypoxia through hypoxia inducible factors and beyond. Nat Rev Mol Cell Biol 2020;21:268–83.
- Xu D, Li DW, Xie J, et al. Effect and mechanism of survivin on hypoxia-induced multidrug resistance of human laryngeal carcinoma cells. BioMed Res Int 2019;2019:1–6.
- Saleh R, Taha RZ, Nair VS, et al. PD-L1 blockade by atezolizumab downregulates signaling pathways associated with tumor growth, metastasis, and hypoxia in human triple negative breast cancer. Cancers 2019;11:1050.
- Maruggi M, Layng FI, Lemos R, Jr., et al. Absence of HIF1A leads to glycogen accumulation and an inflammatory response that enables pancreatic tumor growth. Cancer Res 2019;79:5839–48.
- Chipurupalli S, Kannan E, Tergaonkar V, et al. Hypoxia induced ER stress response as an adaptive mechanism in cancer. Int J Mol Sci 2019;20:749.
- Sanfilippo R, Jones RL, Blay J-Y, et al. Role of chemotherapy, VEGFR inhibitors, and mTOR inhibitors in advanced perivascular epithelioid cell tumors (PEComas). Clin Cancer Res 2019;25:5295–300.
- Saxenaa AK, Bhuniab SS. Development of VEGFR inhibitors as antiangiogenic agents. Med Chem Rev 2016;51:297–310.
- Berretta M, Rinaldi L, Di Benedetto F, et al. Angiogenesis inhibitors for the treatment of hepatocellular carcinoma. Front Pharmacol 2016;7:428–38.
- Paiva TF, Fonseca de Jesus VH, Marques RA, et al. Angiogenesis-related protein expression in bevacizumab-treated metastatic colorectal cancer: NOTCH1 detrimental to overall survival. BMC Cancer 2015;15:643–12.
- Mishra GP, Doddapaneni BS, Nguyen D, et al. Antiangiogenic effect of docetaxel and everolimus as individual and dual-drug-loaded micellar nanocarriers. Pharm Res 2014;31:660–9.
- Bianco R, Garofalo S, Rosa R, et al. Inhibition of mTOR pathway by everolimus cooperates with EGFR inhibitors in human tumours sensitive and resistant to anti-EGFR drugs. Br J Cancer 2008;98:923–30.
- Mishra CB, Tiwari M, Supuran CT. Progress in the development of human carbonic anhydrase inhibitors and their pharmacological applications: where are we today? Med Res Rev 2020;40:2485–565.
- Domsic JF, Avvaru BS, Kim CU, et al. Entrapment of carbon dioxide in the active site of carbonic anhydrase II. J Biol Chem 2008;283:30766–71.
- Duda DM, Tu C, Fisher SZ, et al. Human carbonic anhydrase III: structural and kinetic study of catalysis and proton transfer. Biochemistry 2005;44:10046–53.
- Nocentini A, Supuran CT. Carbonic anhydrase inhibitors as antitumor/antimetastatic agents: a patent review (2008-2018). Expert Op Therap Patents 2018;28:729–40.
- Supuran CT. Structure and function of carbonic anhydrases. Biochem J 2016;473:2023–32.
- Supuran CT, Winum JY. Carbonic anhydrase IX inhibitors in cancer therapy: an update. Future Med Chem 2015;7:1407–14.
- Supuran CT. Inhibition of carbonic anhydrase IX as a novel anticancer mechanism. World J Clin Oncol 2012;3:98–103.
- Neri D, Supuran CT. Interfering with pH regulation in tumours as a therapeutic strategy. Nature Rev Drug Disc 2011;10:767–77.
- De Simone G, Supuran CT. Carbonic anhydrase IX: biochemical and crystallographic characterization of a novel antitumor target. Biochim Biophys Acta 2010;1804:404–9.
- Swietach P, Vaughan-Jones RD, Harris AL. Regulation of tumor pH and the role of carbonic anhydrase 9. Cancer Metast Rev 2007;26:299–310.
- Pastorekova S, Parkkila S, Zavada J. Tumor‐associated carbonic anhydrases and their clinical significance. Adv Clin Chem. 2006;42:167–216.
- Cecchi A, Hulikova A, Pastorek J, et al. Carbonic anhydrase inhibitors. Design of fluorescent sulfonamides as probes of tumor-associated carbonic anhydrase IX that inhibit isozyme IX-mediated acidification of hypoxic tumors. J Med Chem 2005;48:4834–41.
- Parks SK, Chiche J, Pouysségur J. Disrupting proton dynamics and energy metabolism for cancer therapy. Nat Rev Cancer 2013;13:611–23.
- Angeli A, Carta F, Nocentini A, et al. Carbonic anhydrase inhibitors targeting metabolism and tumor microenvironment. Metabolites 2020;10:412.
- Melis C, Distinto S, Bianco G, et al. Targeting tumor associated carbonic anhydrases IX and XII: highly isozyme selective coumarin and psoralen inhibitors. ACS Med Chem Lett 2018;9:725–9.
- Fois B, Distinto S, Meleddu R, et al. Coumarins from Magydaris pastinacea as inhibitors of the tumour-associated carbonic anhydrases IX and XII: isolation, biological studies and in silico evaluation. J Enzyme Inhibition Med Chem 2020;35:539–48.
- Supuran CT. Coumarin carbonic anhydrase inhibitors from natural sources. J Enzyme Inhibition Med Chem 2020;35:1462–70.
- Mancuso F, De Luca L, Angeli A, et al. Synthesis, computational studies and assessment of in vitro inhibitory activity of umbelliferon-based compounds against tumour-associated carbonic anhydrase isoforms IX and XII. J Enzyme Inhibition Med Chem 2020;35:1442–9.
- De Luca L, Mancuso F, Ferro S, et al. Inhibitory effects and structural insights for a novel series of coumarin-based compounds that selectively target human CA IX and CA XII carbonic anhydrases. Euro J Med Chem 2018;143:276–82.
- Temperini C, Innocenti A, Scozzafava A, et al. The coumarin-binding site in carbonic anhydrase accommodates structurally diverse inhibitors: the antiepileptic lacosamide as an example and lead molecule for novel classes of carbonic anhydrase inhibitors. J Med Chem 2010;53:850–4.
- Maresca A, Temperini C, Vu H, et al. Non-zinc mediated inhibition of carbonic anhydrases: coumarins are a new class of suicide inhibitors. J Am Chem Soc 2009;131:3057–62.
- Cozza G, Gianoncelli A, Bonvini P, et al. Urolithin as a converging scaffold linking ellagic acid and coumarin analogues: design of potent protein kinase CK2 inhibitors. ChemMedChem 2011;6:2273–86.
- Yang EB, Zhao YN, Zhang K, et al. Daphnetin, one of coumarin derivatives, is a protein kinase inhibitor. Biochem Biophys Res Commun 1999;260:682–5.
- Liu H, Wang Y, Sharma A, et al. Derivatives containing both coumarin and benzimidazole potently induce caspase-dependent apoptosis of cancer cells through inhibition of PI3K-AKT-mTOR signaling. Anti-Cancer Drugs 2015;26:667–77.
- Mi C, Ma J, Wang KS, et al. Imperatorin suppresses proliferation and angiogenesis of human colon cancer cell by targeting HIF-1α via the mTOR/p70S6K/4E-BP1 and MAPK pathways. J Ethnopharmacol 2017;203:27–38.
- Park H, Choe H, Hong S. Virtual screening and biochemical evaluation to identify new inhibitors of mammalian target of rapamycin (mTOR). Bioorg Med Chem Lett 2014;24:835–8.
- Wu Y, Xu J, Liu Y, et al. A review on anti-tumor mechanisms of coumarins. Front Oncol 2020;10:592853.
- Khalifah RG. The carbon dioxide hydration activity of carbonic anhydrase. I. Stop-flow kinetic studies on the native human isoenzymes B and C. J Biol Chem 1971;246:2561–73.
- Berrino E, Angeli A, Zhdanov DD, et al. Azidothymidine “Clicked” into 1,2,3-triazoles: first report on carbonic anhydrase-telomerase dual-hybrid inhibitors. J Med Chem 2020;63:7392–409.
- Pacchiano F, Carta F, McDonald PC, et al. Ureido-substituted benzenesulfonamides potently inhibit carbonic anhydrase IX and show antimetastatic activity in a model of breast cancer metastasis. J Med Chem 2011;54:1896–902.
- Bilginer S, Gonder B, Gul HI, et al. Novel sulphonamides incorporating triazene moieties show powerful carbonic anhydrase I and II inhibitory properties. J Enzyme Inhibition Medi Chem 2020;35:325–9.
- Schrödinger LLC.: New York N, USA, 2018.
- Mohamadi F, Richards NGJ, Guida WC, et al. Macromodel—an integrated software system for modeling organic and bioorganic molecules using molecular mechanics. J Comput Chem 1990;11:440–67.
- Halgren TA. Merck molecular force field. II. MMFF94 van der Waals and electrostatic parameters for intermolecular interactions. J Comput Chem 1996;17:520–52.
- Kollman PA, Massova I, Reyes C, et al. Calculating structures and free energies of complex molecules: combining molecular mechanics and continuum models. Acc Chem Res 2000;33:889–97.
- Berman HM, Westbrook J, Feng Z, et al. The protein data bank. Nucleic Acids Res 2000;28:235–42.
- Behnke CA, Le Trong I, Godden JW, et al. Atomic resolution studies of carbonic anhydrase II. Acta Crystallogr Sect D, Biol Crystallogr 2010;66:616–27.
- Leitans J, Kazaks A, Balode A, et al. Efficient expression and crystallization system of cancer-associated carbonic anhydrase isoform IX. J Med Chem 2015;58:9004–9.
- Chung JY, Hah JM, Cho AE. Correlation between performance of QM/MM docking and simple classification of binding sites. J Chem Info Model 2009;49:2382–7.
- Schrödinger LLC. The PyMOL molecular graphics system. Version 1.7. New York (NY): Schrödinger LLC.
- Meleddu R, Petrikaite V, Distinto S, et al. Investigating the anticancer activity of isatin/dihydropyrazole hybrids. ACS Med Chem Lett 2019;10:571–6.
- Meleddu R, Distinto S, Cottiglia F, et al. Tuning the dual inhibition of carbonic anhydrase and cyclooxygenase by dihydrothiazole benzensulfonamides. ACS Med Chem Lett 2018;9:1045–50.
- Melis C, Meleddu R, Angeli A, et al. Isatin: a privileged scaffold for the design of carbonic anhydrase inhibitors. J Enzyme Inhib Med Chem 2017;32:68–73.
- Bianco G, Meleddu R, Distinto S, et al. N-acylbenzenesulfonamide dihydro-1,3,4-oxadiazole hybrids: seeking selectivity toward carbonic anhydrase isoforms. ACS Med Chem Lett 2017;8:792–6.
- Meleddu R, Maccioni E, Distinto S, et al. New 4-[(3-cyclohexyl-4-aryl-2,3-dihydro-1,3-thiazol-2-ylidene)amino]benzene-1-sulfonamides, synthesis and inhibitory activity toward carbonic anhydrase I, II, IX, XII. Bioorg Med Chem Lett 2015;25:3281–4.
- Maresca A, Supuran CT. Coumarins incorporating hydroxy- and chloro-moieties selectively inhibit the transmembrane, tumor-associated carbonic anhydrase isoforms IX and XII over the cytosolic ones I and II. Bioorg Med Chem Lett 2010;20:4511–4.
- Daina A, Michielin O, Zoete V. SwissADME: a free web tool to evaluate pharmacokinetics, drug-likeness and medicinal chemistry friendliness of small molecules. Sci Rep 2017;7:42717.