Abstract
A novel class of benzamide-hydroxypyridinone (HPO) derivatives were innovatively designed, synthesised, and biologically evaluated as potential multitargeting candidates for the treatment of Alzheimer's disease (AD) through pharmacophores-merged approaches based on lead compounds 18d, benzyloxy phenyl analogs, and deferiprone (DFP). These hybrids possessed potent Monoamine oxidase B (MAO-B) inhibition as well as excellent iron chelation, with pFe3+ values ranging from 18.13 to 19.39. Among all the compounds, 8g exhibited the most potent selective MAO-B inhibitor (IC50 = 68.4 nM, SI = 213). Moreover, 8g showed favourable pharmacokinetic properties and had great potential to penetrate the BBB in silico and PAMPA-BBB assay. Molecular modelling showed that 8g could adopt an extended conformation and have more enhanced interactions with MAO-B than 18d. In vitro and in vivo assays demonstrated that 8g remarkably resisted Aβ-induced oxidation and ameliorated cognitive impairment induced by scopolamine. Taken collectively, these results suggest that compound 8g is a potential multifunctional candidate for anti-AD treatment.
1. Introduction
With the development of society and the improvement of living standards, human life expectancy has been extended and population ageing has swept the world. The prevalence of dementia is increased with ageing and longevity, especially Alzheimer’s disease (AD). AD is an irrevocable progressive neurodegenerative disorder characterised by a progressive deterioration in memory, incoherent language, cognitive impairments, and behavioural abnormalitiesCitation1. In recent years, it has affected about 50 million people worldwide and it is expected to increase four times by 2050Citation2. The incidence rate will also continue to rise, placing a heavy burden on families and societies. Therefore, AD has become a major socio-economic and healthcare concern which has led to an urgent need to develop novel and more efficient anti-AD drugs.
The pathogenesis of AD is still enigmatic and complicated. Many factors, such as loss of acetylcholine (ACh)Citation3,Citation4, aggregation of AβCitation5, hyperphosphorylation of tau proteinCitation6, disturbance of biometallic homeostasisCitation7, oxidative stressCitation8, neuroinflammation, and activation of microglia cellsCitation9, are all considered to play a pivotal role in the pathogenesis of AD and possess complicated interconnections. The recognised multifactorial nature of AD and its consequent complexity is thought to account for the absence of effective drugs based on a single target. Therefore, the multitarget-directed ligands (MTDLs) can simultaneously intervene in more than two AD pathogenesis and may achieve better therapeutic outcomes when the mechanisms of action are complimentaryCitation10,Citation11.
An elevated level of iron has been demonstrated to be associated with a variety of pathogenesis of AD. The higher iron levels in AD patients will stimulate the expression of amyloid protein precursor (APP) gene and tan protein, which leads to binding to Aβ and tau protein, further promoting Aβ aggregation and tau hyperphosphorylationCitation12. The excess iron ions can also activate microglia cells to produce reactive oxygen species (ROS), causing mitochondrial dysfunction, oxidative stress, and neuronal deathCitation13. In addition, the hydrogen peroxide produced by the oxidation of neurotransmitters was catalysed by MAO-B, which will further participate in the free radical reaction catalysed by iron and then aggravate oxidative stressCitation14,Citation15. Therefore, we believed that combining two major functions (MAO-B inhibition and metal chelation) into a single molecule may afford a promising multifunctional therapeutic strategy for AD therapy (metal chelation, MAO-B inhibition, Aβ aggregation inhibition, and antioxidant activity).
Deferiprone (DFP), a typical orally active hydroxypyridinone (HPO) iron chelator, has been widely used clinically for the treatment of thalassaemiaCitation16. It has also been involved in many clinical trials to treat AD and Parkinson’s disease (PD) because of their ability to remove excess iron from the brainCitation17,Citation18. HPOs have high selectivity and affinity for iron which can form steady neutral 3:1 iron complexes at physiological pH, enabling these complexes to easily penetrate cell membranes through simple diffusion and facilitate iron removal from iron-overload cellsCitation19,Citation20. Many studies have reported HPO derivatives as MTDLs with potential efficacy in the treatment of ADCitation21–24.
In our previous work, some coumarin-HPO derivatives were designed and biologically evaluated as multitargeted iron chelatorsCitation25–27. As a continuation of this research, we present the design, synthesis, and biological evaluation of a class of novel benzamide-HPO derivatives as multitargeting iron chelators with potent anti-AD effects here.
The main rational design for these novel benzamide-HPO hybrids was through pharmacophores-merged approaches based on lead compound 18dCitation27, benzyloxy acetophenone, and DFP. According to our previous research, most of the coumarin-HPO hybrids possess certain MAO-B inhibitory activity and excellent iron-chelating activityCitation27. The structure-activity relationship (SAR) shows that C-2 substituted HPOs and C-7 benzyloxy or alkoxyl substituted coumarins were optimal, and the amido bond enhanced the interaction with MAO-B. Compound 18d was demonstrated to be a promising MTDL. However, these compounds have disadvantages of poor solubility, poor permeability, and relatively low Log P and Log BB values, which may be due to the lactone ring of coumarin. Moreover, benzyloxy phenyl and its analogs have been reported to possess preferable MAO-B inhibition with high selectivity and potent inhibition, such as safinamideCitation28–30. Therefore, to improve the activities and physicochemical properties, the structure was simplified and optimised based on 18d. A novel class of benzamide-HPO hybrids was innovatively designed and synthesised based on pharmacophores-merged approaches ().
2. Results and discussion
2.1. Chemistry
The synthetic strategies for the benzamide-HPO hybrids 8a–y and 11a–c are presented in Schemes 1–4. According to previous research, the biological activities of 2-methyl substituted HPOs are optimal. For the protective group of 3-hydroxyl on HPOs, 4-methoxybenzyl was more easily removed than the benzyl groupCitation27. Therefore, to reduce the difficulty of selective deprotection, 4-methoxylbenzyl chloride was used to protect the 3-hydroxyl group of commercially available maltol 1 (Scheme 1). Then compound 2 was reacted with ethylenediamine to produce intermediates 3 under alkaline conditionsCitation27.
Scheme 1. Synthetic route of compound 3.a aReaction conditions: (i) 4-methoxylbenzyl chloride, K2CO3, DMF, 80 °C, 2 h. (ii) Ethylenediamine, NaOH, ethanol: water = 1.1:1 (v/v), 70 °C, 1.5 h.
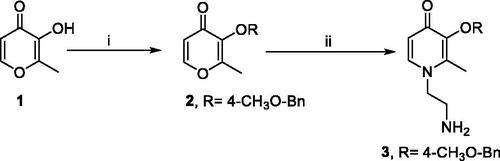
Scheme 4. Synthetic route of compounds 11.a aReaction conditions: (i) (1) 9a–c, DCC, DMAP, 2-mercaptothiazoline, DCM, r.t., 24 h; (2) 3, DCM, r.t., 24 h. (ii) BCl3, anhydrous DCM, −48 °C to r.t., 12 h.
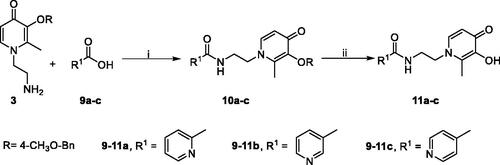
The synthesis of alkoxy and benzyloxy substituted benzoic acids was shown in Scheme 2. The O-alkylated or O-benzylated products 5a–r with moderate yields were obtained by the reaction of o-, m-, and p-hydroxybenzoic acids 4a–c with benzyl bromides or alkyl bromides in ethanol/water with KOHCitation31.
Scheme 2. Synthetic route of compounds 5.a aReaction conditions: (i) alkyl or benzyl bromides, KOH, ethanol: water = 2:1 (v/v), reflux, 5–30 h.
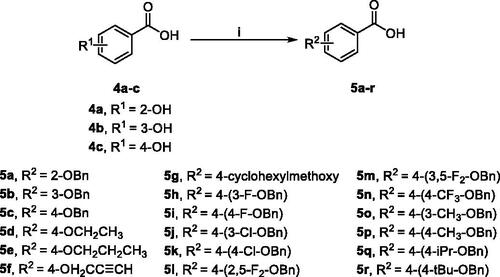
Scheme 3. Synthetic route of compounds 8.a aReaction conditions: (i) (1) 5a-r/6a-d, DCC, DMAP, 2-mercaptothiazoline, DCM, r.t., 24 h; (2) 3, DCM, r.t., 24 h. (ii) BBr3 or BCl3, anhydrous DCM, −78 to −48 °C to r.t., 12 h.
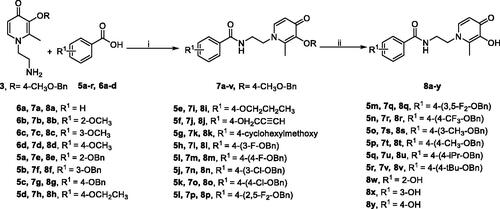
Subsequently, amide derivatives 7a–v and 10a–c were formed by activation of carboxyl groups of benzoic acids 5a–r/6a–d and pyridinecarboxylic acids 9a–c, which using dicyclohexylcarbodiimide (DCC), 2-mercaptothiazoline, and 4-(dimethylamino)pyridine (DMAP). Selective deprotection of 7a–v and 10a–c was achieved by appropriate equivalent BCl3, providing the designed compounds 8a–v and 11a–c as white solids in excellent yields. However, to obtain compounds 8w–y, the methoxy groups on compound 7b–d were removed by BBr3 (Schemes 3 and 4).
2.2. Iron-chelating activity test
3-Hydroxypyridin-4-ones have high affinity and selectivity for Fe3+. Because of the competition effect in aqueous solutions at different pH values, the superior selectivity and affinity for Fe3+ derived from the extensive delocalisation of electrons in its resonance structures (Scheme 5)Citation20. In biological conditions, the pFe3+ value is a more useful parameter than the traditional stability constant in assessing the affinity of ligands for Fe3+. It was defined as the negative logarithm of the concentration of free Fe3+ in solution at pH 7.4 ([Fe3+]total = 10−6 M, [ligand]total = 10−5 M)Citation20. Therefore, the pKa values of compounds and their affinity constants for Fe3+ were measured ()Citation32.
Table 1. The pKa values of all compounds and their affinity constants for Fe3+.a
Similar to the previous research, because of the amide bond, all compounds were fitting to three pKa values by spectrophotometric and speciation plot analysis, such as compounds 8a and 8g ()Citation27. There was no doubt that the pKa1 (<3.40) was attributed to the protonation of the 4-carbonyl oxygen group, the pKa2 (9.55–9.99) was belonging to the dissociation of the 3-OH group. These compounds were all determined in 0.1 M KCl aqueous solution, indicating that they have good water solubility. The spectrophotometric titration yielded two main pKa values for all compounds over the pH range 2.0–10.5, such as 8a and 8g, which are 3.43, 9.81 and 3.38, 9.69, respectively. It could be seen that the ionisation equilibrium of compounds is pH-dependent and they possess uncharged property in the pH range of 6.0–8.0 (). Obviously, the pKa1 values of compounds were almost all lower than the corresponding value of DFP. This is because that the substitutional groups on 1-nitrogen affect the negative charge delocalisation of 4-carbonyl oxygen.
Figure 2. pH dependence of UV spectra of compounds 8a and 8g in 0.1 M KCl over the pH range 2.0–10.5 at 25 °C. (A-1/B-1) The 2D titration spectra of compound 8a/8g. (A-2/B-2) Speciation plots of compound 8a/8g.
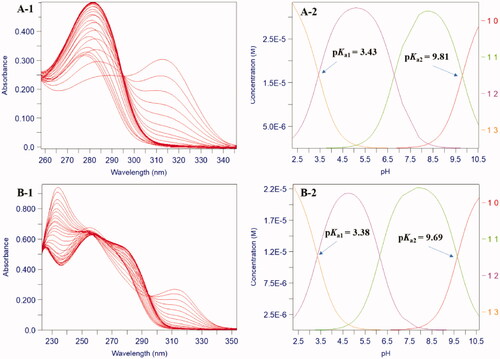
The affinity constants for Fe3+ (log β1, β2, and β3) were also analysed according to the absorption spectra of speciation between Fe3+ with ligands at different pH solutions. The pFe3+ values were then calculated based on the pKa values and the above three affinity constants. All compounds exhibited excellent pFe3+ values (18.13–19.39) (). Compounds 8g, 8l, and 8u exhibited the most potent iron chelation with pFe3+ values of 19.31, 19.39, and 19.35, respectively, which were higher than that of DFP (pFe3+ = 18.24) under the same experimental conditions. As found with the speciation plot of compound 8g, the neutral 3:1 complexes dominated over the pH range 6–9 ().
Figure 3. pH dependence of UV–Vis spectra of compound 8g in 1.0 μM Fe3+ at 25 °C. (A) The spectra of compound 8g (1.1 μM) over the pH range 1.113–2.183 in DMSO: 0.1 M KCl = 1:9 (v/v). (B) The spectra of compound 8g (5.0 μM) over the pH range 2.534–9.069 in DMSO: 0.1 M KCl = 2:3 (v/v). (C) Speciation plot of Fe3+ and compound 8g.
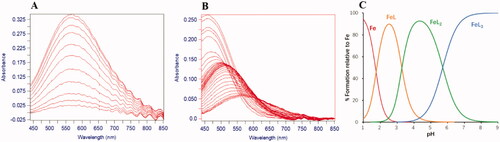
SAR showed that the 4-carbonyl and 3-hydroxyl groups on the HPO ring were necessary for iron chelation. The para- and meta- substitutions on the benzene ring showed better iron chelation than ortho-substitutions. Compounds with 4-bulky alkoxy, 4-benzyloxy, and 4-benzyloxy substituted by electron-withdrawing groups on the benzene ring exhibited good iron chelation. While the benzene ring replaced by pyridine ring exhibited relatively poor iron chelation. In general, all benzamide-HPO hybrids show favourable iron-chelating ability.
2.3. Human MAO-B inhibition assay
The MAO-B inhibitory ability of all benzamide-HPO hybrids was measured. As shown in , the inhibitory rate at the concentration of 1 μM and 100 nM were firstly screened using pargyline as the positive control. Most compounds displayed remarkable MAO-B inhibition with an inhibitory rate ranging from 60 to 80% at 1 μM. Compound 8l showed more than 80% inhibitory effect but was still weaker than pargyline. When tested at 100 nM, most compounds exhibited MAO-B inhibitory rate between 40 and 50%. Five compounds (8a, 8g, 8i, 8l, and 8m) displayed inhibitory effects over 50%, which is superior to pargyline.
Table 2. MAO-B inhibitory rate of all compounds.
The IC50 values of 20 compounds with favourable MAO-B inhibition were subsequently measured ( and ). Most of the compounds exerted IC50 values between 100 and 200 nM. There was no doubt that five compounds (8a, 8g, 8i, 8l, and 8m) mentioned above also possessed much more potent MAO-B inhibition than pargyline, with IC50 values below 100 nM. Compound 8g exhibited the highest inhibition and good selectivity for MAO-B (IC50 = 68.4 nM, SI = 213), which was demonstrated to be the most potent one. However, it is interesting that the compounds without benzyloxy phenyl motif (8a, 8i) still showed available inhibition of MAO-B, with IC50 values very similar to that of compound 8g, suggesting that benzyl may not be the key pharmacophore. This may provide useful guidance for us to design more concise and efficient compounds in the future.
Table 3. The IC50 values against MAO-B of the selected compounds.
Table 4. The IC50 values of compound 8g against MAO-A and MAO-B.
The SAR study indicated that the para- and ortho-substitutions on the benzene ring exhibited better MAO-B inhibition than meta-substitutions. When substitutions were all on the para-benzene ring, compounds with saturated alkoxy with long chains, benzyloxy, and benzyloxy substituted by single electron-withdrawing groups at para-phenyl ring exhibited better inhibition effect on MAO-B. Moreover, poor MAO-B inhibition was obtained when the benzene ring was replaced by a pyridine ring.
2.4. Prediction of drug-like properties and BBB permeability
To further understand the drug-likeness, the molecular properties of these new hybrids were predicted and performed by molinspiration (http://www.molinspiration.com). It was found that miLog p-values of HPOs were closer to the experimentally measured values than those calculated by other programsCitation33. All the compounds were in accordance with Lipinski’s rules and Veber’s rules. They also had appropriate topological polar surface area (TPSA) values except for 8a because low TPSA (<75 Å2) may increase the risk of non-specific toxicityCitation34. Subsequently, the BBB permeability is very critical for anti-AD compounds. Log BB was calculated with Clark’s equation while compounds with a Log BB value <−1.0 are not likely to enter the brain (Table S1)Citation35. Therefore, 19 compounds (8b, 8e–8v) possessed preferable drug-likeness with appropriate solubility and permeability. 8g was found to be the optimum compound (miLog P = 1.87, Log BB = −0.77) when simultaneously possessed good iron chelation and MAO-B inhibition.
Another two in silico methods (ADMETlab and admetSAR) were also applied to predict the BBB permeability of compound 8gCitation36,Citation37. This reliable classification model was built by machine learning ways and resampling methodsCitation38. As shown in , compound 8g was classified as BBB + with a probability of 0.631 and 0.8164, respectively.
Table 5. The BBB permeability of compound 8g is predicted by ADMETlab and admetSAR.
Certainly, the parallel artificial membrane permeation assay (PAMPA) was carried out to assess the capacity of 8g to penetrate into the brainCitation39. We have identified the effective permeability (Pe) for seven commercial drugs with known CNS penetration as well as for the compound 8g (). The standard concentration-absorbance curve for each compound was shown in the Supporting Information (Table S1). According to the BBB permeation limits defined by Di et al.Citation39, compounds with Pe > 4.0 were possessing high permeation, with Pe < 2.0 were showing low permeation, and with 2.0 < Pe < 4.0 were displaying uncertain permeability. Compound 8g showed Pe values above 4.0, suggesting that compound 8g has a high potential to cross the BBB by passive diffusion.
Table 6. The permeability (Pe, 10–6 cm/s) results of compound 8g and commercial drugs in the PAMPA-BBB assay and their prediction of CNS penetrability.
2.5. Molecular modelling
The potential binding sites of optimum compound 8g with MAO-B (PDB: 2V5Z) was shown in , which was investigated by molecular docking. The ligand formed an extended conformation between the substrate and entrance cavities. The benzyloxyphenyl of 8g has formed some lipophilic binding interactions with Tyr 326, Ile 316, Ile 199, Leu 164, Pro 104, Leu 171, and Cys 172 in the hydrophobic entrance cavity. The carbonyl oxygen of amide forms strong hydrogen bond interaction with Cys 172, NH forms strong hydrogen bond interaction with Leu 171, and also interacts with Gln 206 and Tyr 435 to some extent, indicating that the introduction of amide bond enhanced the interaction with enzyme and result in its good inhibitory activity against MAO-B. Furthermore, the hydroxyl of HPO forms Pi-sulfur interaction with Lys 296 and forms a hydrogen bond with the FAD cofactor. The methyl of HPO forms Pi-alkyl interaction with Tyr 398, which indicated that HPO also works as a critical segment for interacting with MAO-B. Therefore, all these interactions may explain the preferable activity of compound 8g.
2.6. Antioxidant activity assays
Aβ deposition and peroxidation are also important clinical features of AD. Therefore, we subsequently assessed the antioxidation of compound 8g, using 2′,7′-dichlorofluorescin diacetate (DCFH-DA) to detect intracellular ROS generation on the PC12 cell line derived from neural pheochromocytoma. Based on our previous research, the concentration of 10 μM of compound 8g was chosen in this test. As shown in and , the fluorescence intensity of cells containing Aβ1–42 was much higher than normal cells, indicating that Aβ deposition would cause oxidative stress to a certain extent. When compound 8g was adopted, it was surprisingly found that the Aβ1–42-induced intracellular ROS levels were significantly reduced (33.32% vs. model group 44.58%). The ROS fluorescence intensity also showed a significant decrease, indicating that 8g had the ability to resist Aβ-induced oxidation.
2.7. Cognitive and memory assays in vivo
The anti-AD effect of compound 8g on the scopolamine-induced cognitive dysfunction ICR mice model was assessed by Morris water maze (MWM) testCitation27. Each group of mice was intraperitoneally injected with compound 8g (15 mg/kg), pargyline (15 mg/kg), or PBS, and then injection with scopolamine (15 mg/kg) or PBS 30 min later once a day for 15 consecutive days. The MWM test was performed at the last 5 days including two days of memory and learning training, three days of cognitive assessment. On the fifth day, a probe training trial was performed and analysed. To make a contrast with lead compound 18d, we still chose pargyline (15 mg/kg) as the positive drug. However, the dosage of scopolamine was increased from 5 mg/kg to 15 mg/kg to achieve a better modelling effect.
The data for the last day was shown in , the latency (12.54 ± 2.71 vs. 41.40 ± 5.87 s, ###p < 0.001) and the distance (2.40 ± 0.49 vs. 7.74 ± 1.23 m, ##p < 0.01) of mice treated with scopolamine were remarkably more prolonged than the control group. Moreover, the entries to the target were also significantly decreased (5.75 ± 0.86 vs. 2.00 ± 0.50, ##p < 0.01), demonstrating that the mice model of cognitive dysfunction has been well-established. Treatment with 8g markedly reduced the latency (16.03 ± 2.76 s, **p < 0.01) and the distance to the target (2.64 ± 0.52 m, **p < 0.01), which a little better than the pargyline group (13.96 ± 2.94 s, ***p < 0.001) (2.89 ± 0.69 m, **p < 0.01). Compound 8g (4.12 ± 0.40, **p < 0.01) also worked better than pargyline (3.85 ± 0.48, *p < 0.05) in increasing the entries to target. The representative trajectories () also showed that the model group was very lengthy and disorganised, while compound 8g was clearer than pargyline, which demonstrated that the scopolamine-induced spatial learning and memory deficits were remarkably ameliorated by compound 8g.
Figure 7. Effect of compound 8g and pargyline on scopolamine-induced cognitive deficit ICR mice determined by MWM test. (A) Latency to the target. (B) Distance to the target. (C) Entries to the target.(n = 8, mean ± SEM; *p < 0.05, **p < 0.01, ***p < 0.001, 8g or pargyline group vs. model group; ##p < 0.01, ###p < 0.001, Control group vs. model group).
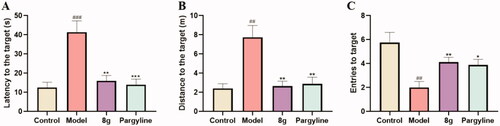
3. Conclusion
In conclusion, we reported a class of novel benzamide-HPO hybrids as potential anti-AD candidates with multiple biochemical properties based on the MTDLs strategy. All compounds possessed excellent iron chelation activity and showed promising MAO-B inhibition. Among them, compound 8g was proved to be the most potent iron chelator (pFe3+ = 19.31) and the most effective selective MAO-B inhibitor (IC50 = 68.4 nM, SI = 213). In silico drug-likeness predictions and PAMPA-BBB assay demonstrated that 8g possessed acceptable BBB permeability. Molecular modelling showed that 8g could form an extended conformation and have more enhanced interactions with MAO-B than 18d. In vitro assay indicated that compound 8g significantly reduced the Aβ-induced intracellular ROS levels and remarkedly reversed the cognitive deficit in the MWM test. All results indicated that hybrid 8g is an interesting and promising multifunctional agent with the potential to be a therapeutic candidate against AD.
Supplemental Material
Download PDF (6.6 MB)Disclosure statement
The authors declare no competing financial interest and the target compounds were protected in a patent by the authors (CN 111995567).
Additional information
Funding
References
- Scheltens P, Blennow K, Breteler MM, et al. Alzheimer's disease. Lancet 2016;388:505–17.
- Association AS. 2019 Alzheimer's disease facts and figures. Alzheimers Dement 2019;15:321–87.
- Wang H, Zhang HY. Reconsideration of anticholinesterase therapeutic strategies against Alzheimer's disease. ACS Chem Neurosci 2019;10:852–62.
- Hampel H, Mesulam MM, Cuello AC, et al. The cholinergic system in the pathophysiology and treatment of Alzheimer's disease. Brain 2018;141:1917–33.
- Wolfe MS. Secretase targets for Alzheimer's disease: identification and therapeutic potential. J Med Chem 2001;44:2039–60.
- Chu D, Liu F. Pathological changes of Tau related to Alzheimer's disease. ACS Chem Neurosci 2019;10:931–44.
- Ayton S, Lei P, Bush AI. Metallostasis in Alzheimer's disease. Free Radic Biol Med 2013;62:76–89.
- Losada-Barreiro S, Bravo-Diaz C. Free radicals and polyphenols: the redox chemistry of neurodegenerative diseases. Eur J Med Chem 2017;133:379–402.
- Walker KA, Ficek BN, Westbrook R. Understanding the role of systemic inflammation in Alzheimer's disease. ACS Chem Neurosci 2019;10:3340–2.
- Zhou JT, Jiang XY, He SY, et al. Rational design of multitarget-directed ligands: strategies and emerging paradigms. J Med Chem 2019;62:8881–914.
- Oset-Gasque MJ, Marco-Contelles J. Alzheimer's disease, the “one-molecule, one-target” paradigm, and the multitarget directed ligand approach. ACS Chem Neurosci 2018;9:401–3.
- Liu JL, Fan YG, Yang ZS, et al. Iron and Alzheimer's disease: from pathogenesis to therapeutic implications. Front Neurosci 2018;12:632–14.
- Liu Y, Nguyen M, Robert A, et al. Metal ions in Alzheimer's disease: a key role or not? Acc Chem Res 2019;52:2026–35.
- Mangiatordi GF, Alberga D, Pisani L, et al. A rational approach to elucidate human monoamine oxidase molecular selectivity. Eur J Pharm Sci 2017;101:90–9.
- Edmondson DE, Binda C, Mattevi A. Structural insights into the mechanism of amine oxidation by monoamine oxidases A and B. Arch Biochem Biophys 2007;464:269–76.
- Hider RC. Recent developments centered on orally active iron chelators. Thalassemia Rep 2014;4:2261–9.
- Martin-Bastida A, Ward RJ, Newbould R, et al. Brain iron chelation by deferiprone in a phase 2 randomised double-blinded placebo controlled clinical trial in Parkinson's disease. Sci Rep 2017;7:1398–406.
- Crichton RR, Ward RJ, Hider RC. The efficacy of iron chelators for removing iron from specific brain regions and the pituitary-ironing out the brain. Pharmaceuticals 2019;12:138–50.
- Hider RC, Roy S, Ma YM, et al. The potential application of iron chelators for the treatment of neurodegenerative diseases. Metallomics 2011;3:239–49.
- Cilibrizzi A, Abbate V, Chen YL, et al. Hydroxypyridinone journey into metal chelation. Chem Rev 2018;118:7657–701.
- Jiang XY, Zhou T, Bai RR, et al. Hydroxypyridinone-based iron chelators with broad-ranging biological activities. J Med Chem 2020;63:14470–501.
- Chaves S, Piemontese L, Hiremathad A, et al. Hydroxypyridinone derivatives: a fascinating class of chelators with therapeutic applications – an update. Curr Med Chem 2018;25:97–112.
- Santos MA, Chand K, Chaves S. Recent progress in multifunctional metal chelators as potential drugs for Alzheimer's disease. Coord Chem Rev 2016;327–328:287–303.
- Hiremathad A, Chand K, Tolayan L, et al. Hydroxypyridinone-benzofuran hybrids with potential protective roles for Alzheimer's disease therapy. J Inorg Biochem 2018;179:82–96.
- Mi ZS, Gan B, Yu SH, et al. Dual-target anti-Alzheimer's disease agents with both iron ion chelating and monoamine oxidase-B inhibitory activity. J Enzyme Inhib Med Chem 2019;34:1489–97.
- Zhang CJ, Yang K, Yu SH, et al. Design, synthesis and biological evaluation of hydroxypyridinone-coumarin hybrids as multimodal monoamine oxidase B inhibitors and iron chelates against Alzheimer's disease. Eur J Med Chem 2019;180:367–82.
- Jiang XY, Guo JN, Lv YJ, et al. Rational design, synthesis and biological evaluation of novel multitargeting anti-AD iron chelators with potent MAO-B inhibitory and antioxidant activity. Bioorg Med Chem 2020;28:115550.
- Gealageas R, Devineau A, So PPL, et al. Development of novel monoamine oxidase-B (MAO-B) inhibitors with reduced blood-brain barrier permeability for the potential management of noncentral nervous system (CNS) diseases. J Med Chem 2018;61:7043–64.
- Wang Z, Wu J, Yang X, et al. Neuroprotective effects of benzyloxy substituted small molecule monoamine oxidase B inhibitors in Parkinson's disease. Bioorg Med Chem 2016;24:5929–40.
- Legoabe LJ, Petzer A, Petzer JP. 2-Acetylphenol analogs as potent reversible monoamine oxidase inhibitors. Drug Des Dev Ther 2015;9:3635–44.
- Giancotti G, Cancellieri M, Balboni A, et al. Rational modifications on a benzylidene-acrylohydrazide antiviral scaffold, synthesis and evaluation of bioactivity against Chikungunya virus. Eur J Med Chem 2018;149:56–68.
- Xie YY, Lu ZD, Kong XL, et al. Systematic comparison of the mono-, dimethyl- and trimethyl 3-hydroxy-4(1H)-pyridones – attempted optimization of the orally active iron chelator, deferiprone. Eur J Med Chem 2016;115:132–40.
- Chen WT, Yuan X, Li Z, et al. CN128: A new orally active hydroxypyridinone iron chelator. J Med Chem 2020;63:4215–26.
- Prasanna S, Doerksen RJ. Topological polar surface area: a useful descriptor in 2D-QSAR. Curr Med Chem 2009;16:21–41.
- Clark DE, Pickett SD. Computational methods for the prediction of ‘drug-likeness’. Drug Discov Today 2000;5:49–58.
- Dong J, Wang NN, Yao ZJ, et al. ADMETlab: a platform for systematic ADMET evaluation based on a comprehensively collected ADMET database. J Cheminform 2018;10:29.
- Cheng FX, Li WH, Zhou YD, et al. admetSAR: a comprehensive source and free tool for assessment of chemical ADMET properties. J Chem Inf Model 2012;52:3099–105.
- Wang Z, Yang HB, Wu ZR, et al. In silico prediction of blood-brain barrier permeability of compounds by machine learning and resampling methods. ChemMedChem 2018;13:2189–201.
- Di L, Kerns EH, Fan K, et al. High throughput artificial membrane permeability assay for blood-brain barrier. Eur J Med Chem 2003;38:223–32.
- Lemes LFN, de Andrade Ramos G, de Oliveira AS, et al. Cardanol-derived AChE inhibitors: towards the development of dual binding derivatives for Alzheimer's disease. Eur J Med Chem 2016;108:687–700.