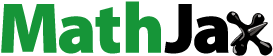
Abstract
A novel series of twelve aromatic bis-ureido-substituted benzenesulfonamides was synthesised by conjugation of aromatic aminobenzenesulfonamides with aromatic bis-isocyanates. The obtained bis-ureido-substituted derivatives were tested against four selected human carbonic anhydrase isoforms (hCA I, hCA II, hCA IX and hCA XII). Most of the new compounds showed an effective inhibitory profile against isoforms hCA IX and hCA XII, also having some selectivity with respect to hCA I and hCA II. The inhibition constants of these compounds against isoforms hCA IX and XII were in the range of 6.73–835 and 5.02–429 nM, respectively. Since hCA IX and hCA XII are important drug targets for anti-cancer/anti-metastatic drugs, these effective inhibitors reported here may be considered of interest for cancer related studies in which these enzymes are involved.
Introduction
Carbonic anhydrases (CAs, EC 4.2.1.1) are a superfamily of abundant metalloenzymes that catalyse a physiologically very important reaction, which is the reversible conversion of the carbon dioxide (CO2) to bicarbonate (HCO3−) and proton ions (H+). This reaction is also occurring under noncatalytic conditions but it is very slow for most life processes in which it is involvedCitation1–8. Up to now, eight genetically distinct CA families (α, β, γ, δ, ζ, η, θ, and more recently ι) were reported and they are present in all kingdoms of lifeCitation9–16. Among them, in humans, 16 different isozymes which are all belong to the α-CAs were discovered, possessing a diverse sub-cellular localisation, catalytic activity and organ/tissue distributionCitation17–24. These isoforms were classified according to their localisation, including the cytosolic forms (CA I, II, III, VII, and XIII), membrane-bound ones (CA IV, IX, XII, XIV, and XV), mitochondrial isoforms (CA VA and VB), and one secreted in saliva and milk (CA VI)Citation21–26. Among these isoforms, two of the membrane-bound ones (CA IX and CA XII), have been identified as tumour-associated enzymes and attracted much attention in the search of novel, potent and selective cancer drugs, in the last decadesCitation17–24. These isoforms have a crucial role as diagnostic tools for imaging hypoxic tumours but also in the metabolism, survival, migration and invasion of tumour cells, by regulating pH and other processes connected to tumorigenesis. Therefore, potent and selective inhibitors of these isoforms with new class of compounds might be a strategy to develop efficient antitumor/antimetastatic agentsCitation17–24.
Ureido-substituted primary benzenesulfonamides were extensively studied as potent and selective CA inhibitors (CAIs), and among them 4-[[(4-fluorophenyl) carbamoyl] amino] benzenesulfonamide (SLC-0111) () has reached to Phase Ib/II clinical trials for the management of advanced metastatic solid tumorsCitation12–14. Initially, ureido-substituted benzenesulfonamides were investigated as hCA I, hCA II, and bCA IV inhibitors possessing high activity and unexpectedly high selectivityCitation25. Later, other such ureido bearing compounds (benzenesulfonamides and benzenesulfamates) have been designed, synthesised and explored as potent and selective hCA IX and XII inhibitorsCitation12–14. On the other hand, more recently, secondary and tertiary benzenesulfonamides started to be also investigated as CAIs, some of which showed strong affinity against several important isozymes, although they were generally less effective, compared to primary counterpartsCitation26,Citation27.
In continuation of our recent efforts to develop effective and isoform selective CAIsCitation28–30, in the present study, we report bis-ureido-substituted primary benzenesulfonamides acting as potent human (h) hCA inhibitors using the design strategy summarised in . To the best of our knowledge, this is the first bis-ureido-substituted primary benzenesulfonamide study, which examined the inhibition profile of these compounds on selected hCAs, namely off-target cytosolic isoforms hCA I and II, and tumour-overexpressed membrane-bound isoforms hCA IX and XII.
Materials and methods
Chemistry
Unless otherwise noted, all the chemicals and anhydrous solvents were purchased from Sigma-Aldrich, Merck, Alfa Aesar and TCI and used without further purification. Melting points (mp) were determined with SMP20 melting point apparatus and are uncorrected. FT-IR spectra were obtained by using Perkin Elmer Spectrum 100 FT-IR spectrometer. Nuclear Magnetic Resonance (1H-NMR and 13C-NMR) spectra of compounds were recorded using a Bruker Advance III 300 Mhz spectrometer in DMSO-d6 as the solvent, and TMS as the internal standard operating at 300 Mhz for 1H-NMR and 75 Mhz for 13C-NMR. Chemical shifts are expressed in ppm relative to tetramethylsilane. Splitting patterns are designated as singlet (s), doublet (d), triplet (t), quartette (q), and multiplet (m). Thin layer chromatography (TLC) was carried out on Merck silica gel 60 F254 plates.
General procedure for preparation of bis-ureido substituted primary benzenesulfonamide derivatives (8-19)
4-aminobenzenesulfonamide/3-aminobenzenesulfonamide/4(2-aminoethyl)benzenesulfonamide (2 mmol) was dissolved in acetonitrile (10-15 ml) and then treated with subsequent bis-isocyanates (1.1 mmol) (1,4-Phenylene diisocyanide for Y1, 4,4′-Methylenebis (phenyl isocyanate) for Y2, 3,3′-Dimethyl-4,4′-biphenylene diisocyanate for Y3, 4-Methyl-1,3-phenylene diisocyanate for Y4). The mixture was stirred at room temperature for 3h, and then heated at 50 °C until completion (TLC monitoring). The obtained precipitate was filtered off, washed with diethyl ether (50 ml) and water, and dried in vacuo. The obtained products (compounds 8-19) were characterised in detail by spectroscopic and analytic methods (FT-IR, 1H-NMR, 13C-NMR, and melting points) (Scheme 1).
Scheme 1. General synthetic route for the synthesis of the primary benzenesulfonamides incorporating bis-ureido moieties 8–19.
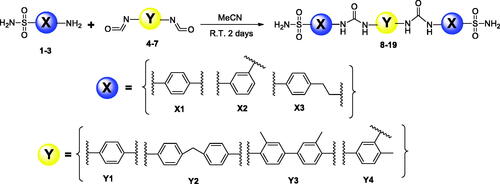
4,4'-(((1,4-Phenylenebis(azanediyl))bis(carbonyl))bis(azanediyl))dibenzenesulfonamide (8)
Yield: 88%; Colour: white solid; Melting Point: >300 °C; FT-IR (cm−1): 3387, 3331, 3222 (NH), 1689 (C=O), 1306, 1154 (symmetric) (S=O); 1H-NMR (DMSO-d6, 300 MHz, δ ppm): 9.02 (s, 2H, -NH-), 8.69 (s, 2H, -NH-), 7.73 (d, J = 7.5 Hz, 4H, Ar-H), 7.61 (d, J = 7.5 Hz, 4H, Ar-H), 7.40 (s, 4H, -SO2NH2), 7.21 (s, 4H, Ar-H); 13C-NMR (DMSO-d6, 75 MHz, δ ppm): 150.65, 141.31, 135.00, 132.30, 125.16, 117.57, 115.61.
4,4'-((((Methylenebis(4,1-phenylene))bis(azanediyl))bis(carbonyl))bis(azanediyl))dibenzenesulfonamide (9)
Yield: 82%; Colour: white solid; Melting Point: 293–294 °C; FT-IR (cm−1): 3374, 3324, 3262 (NH), 1656 (C=O), 1328, 1151 (symmetric) (S=O); 1H-NMR (DMSO-d6, 300 MHz, δ ppm): 9.05 (s, 2H, -NH-), 8.76 (s, 2H, -NH-), 7.75 (d, J = 8.4 Hz, 4H, Ar-H), 7.63 (d, J = 8.7 Hz, 4H, Ar-H), 7.40 (d, J = 8.4 Hz, 4H, Ar-H), 7.25 (s, 4H, -SO2NH2), 7.15 (d, J = 8.7 Hz, 4H, Ar-H), 3.84 (s, 2H, Ph-CH2-Ph); 13C-NMR (DMSO-d6, 75 MHz, δ ppm): 152.74, 143.39, 137.64, 137.18, 135.87, 129.46, 127.31, 119.11, 118.82, 117.85.
4,4'-((((3,3'-Dimethyl-[1,1'-biphenyl]-4,4'-diyl)bis(azanediyl))bis(carbonyl))bis(azanediyl))dibenzenesulfonamide (10)
Yield: 76%; Colour: white solid; Melting Point: >300 °C; FT-IR (cm−1): 3412, 3348, 3292 (NH), 1693 (C=O), 1304, 1148 (symmetric) (S=O); 1H-NMR (DMSO-d6, 300 MHz, δ ppm): 9.45 (s, 2H, -NH-), 8.13 (s, 2H, -NH-), 7.99–7.95 (m, 4H, Ar-H), 7.77 (d, J = 8.4 Hz, 4H, Ar-H), 7.66 (d, J = 8.4 Hz, 4H, Ar-H), 7.52–7.45 (m, 4H, Ar-H), 7.25 (s, 4H, -SO2NH2), 2.30 (s, 6H, -CH3); 13C-NMR (DMSO-d6, 75 MHz, δ ppm): 152.76, 146.07, 143.43, 137.11, 136.16, 134.18, 128.56, 127.60, 124.20, 121.99, 117.31, 18.61.
4,4'-((((4-Methyl-1,3-phenylene)bis(azanediyl))bis(carbonyl))bis(azanediyl))dibenzenesulfonamide (11)
Yield: 63%; Colour: white solid; Melting Point: 279–281 °C; FT-IR (cm−1): 3391, 3309, 3190 (NH), 1701 (C=O), 1310, 1154 (symmetric) (S=O); 1H-NMR (DMSO-d6, 300 MHz, δ ppm): 9.45 (s, 1H, -NH-), 8.94 (s, 1H, -NH-), 8.80 (d, J = 7.5 Hz, 2H, Ar-H), 8.44 (s, 1H, -NH-), 8.29 (s, 1H, -NH-), 8.04 (s, 2H, Ar-H), 7.78–7.59 (m, 5H, Ar-H), 7.23 (s, 4H, -SO2NH2), 7.18–7.08 (m, 2H, Ar-H), 2.21 (s, 3H, -CH3); 13C-NMR (DMSO-d6, 75 MHz, δ ppm): 152.74, 152.38, 147.11, 143.42, 138.20, 137.83, 137.40, 130.61, 127.21, 121.89, 117.63, 115.96, 111.67, 104.71, 17.63.
3,3'-(((1,4-Phenylenebis(azanediyl))bis(carbonyl))bis(azanediyl))dibenzenesulfonamide (12)
Yield: 92%; Colour: white solid; Melting Point: >300 °C; FT-IR (cm−1): 3362, 3322, 3267 (NH), 1638 (C=O), 1338, 1153 (symmetric) (S=O); 1H-NMR (DMSO-d6, 300 MHz, δ ppm): 8.96 (s, 2H, -NH-), 8.61 (s, 2H, -NH-), 8.07 (s, 2H, Ar-H), 7.55 (d, J = 7.2 Hz, 2H, Ar-H), 7.46 (t, 2H, Ar-H), 7.42–7.40 (m, 6H, Ar-H and -SO2NH2), 7.36 (s, 4H, Ar-H); 13C-NMR (DMSO-d6, 75 MHz, δ ppm): 152.96, 145.43, 140.78, 134.47, 129.87, 121.43, 119.67, 119.17, 115.44.
3,3'-((((Methylenebis(4,1-phenylene))bis(azanediyl))bis(carbonyl))bis(azanediyl))dibenzenesulfonamide (13)
Yield: 82%; Colour: white solid; Melting Point: 293–295 °C; FT-IR (cm−1): 3357, 3322, 3268 (NH), 1644 (C=O), 1339, 1154 (symmetric) (S=O); 1H-NMR (DMSO-d6, 300 MHz, δ ppm): 8.99 (s, 2H, -NH-), 8.68 (s, 2H, -NH-), 8.09 (s, 2H, Ar-H), 7.54 (d, J = 7.5 Hz, 2H, Ar-H), 7.50–7.41 (m, 8H, Ar-H), 7.38 (s, 4H, -SO2NH2), 7.15 (d, J = 8.4 Hz, 4H, Ar-H), 3.84 (s, 2H, Ph-CH2-Ph); 13C-NMR (DMSO-d6, 75 MHz, δ ppm): 152.88, 145.14, 140.71, 137.78, 135.80, 129.88, 129.44, 121.46, 119.23, 119.04, 115.43.
3,3'-((((3,3'-Dimethyl-[1,1'-biphenyl]-4,4'-diyl)bis(azanediyl))bis(carbonyl))bis(azanediyl))dibenzenesulfonamide (14)
Yield: 80%; Colour: white solid; Melting Point: >300 °C; FT-IR (cm−1): 3367, 3306, 3284 (NH), 1639 (C=O), 1302, 1151 (symmetric) (S=O); 1H-NMR (DMSO-d6, 300 MHz, δ ppm): 9.42 (s, 2H, -NH-), 8.13 (s, 2H, -NH-), 8.06 (s, 2H, Ar-H), 7.96 (d, J = 8.4 Hz, 2H, Ar-H), 7.60 (d, J = 7.5 Hz, 2H, Ar-H), 7.52–7.46 (m, 8H, Ar-H), 7.40 (s, 4H, -SO2NH2), 2.34 (s, 6H, -CH3); 13C-NMR (DMSO-d6, 75 MHz, δ ppm): 153.04, 145.20, 140.78, 136.71, 134.75, 130.01, 128.46, 124.47, 121.73, 121.28, 119.24, 115.27, 18.51.
3,3'-((((4-Methyl-1,3-phenylene)bis(azanediyl))bis(carbonyl))bis(azanediyl))dibenzenesulfonamide (15)
Yield: 76%; Colour: white solid; Melting Point: 233–234 °C; FT-IR (cm−1): 3388, 3322, 3272 (NH), 1692, 1645 (C=O), 1308, 1150 (symmetric) (S=O); 1H-NMR (DMSO-d6, 300 MHz, δ ppm): 9.42 (s, 1H, -NH-), 8.88 (s, 1H, -NH-), 8.77 (s, 1H, -NH-), 8.17 (s, 1H, Ar-H), 8.10 (d, J = 7.8 Hz, 2H, Ar-H), 7.98 (s, 1H, Ar-H), 7.56–7.45 (m, 6H, Ar-H), 7.39 (s, 5H, -SO2NH2 and Ar-H), 7.18 (d, J = 7.2 Hz, 1H, Ar-H), 7.10 (d, J = 8.4 Hz, 1H, Ar-H), 2.21 (s, 3H, -CH3); 13C-NMR (DMSO-d6, 75 MHz, δ ppm): 152.87, 152.78, 145.19, 140.76, 138.07, 137.83, 130.79, 130.03, 129.89, 121.44, 121.31, 121.21, 119.21, 115.33, 113.53, 111.38, 17.71.
4,4'-((((1,4-Phenylenebis(azanediyl))bis(carbonyl))bis(azanediyl))bis(ethane-2,1-diyl))dibenzenesulfonamide (16)
Yield: 88%; Colour: white solid; Melting Point: >300 °C; FT-IR (cm−1): 3361, 3333, 3266 (NH), 1626 (C=O), 1333, 1156 (symmetric) (S=O); 1H-NMR (DMSO-d6, 300 MHz, δ ppm): 8.28 (s, 2H, -CH2CH2NH-CO-NH-), 7.77 (d, J = 8.4 Hz, 4H, Ar-H), 7.43 (d, J = 8.4 Hz, 4H, Ar-H), 7.33 (s, 4H, -SO2NH2), 7.23 (s, 4H, Ar-H), 6.05 (t, 2H, -CH2CH2NH-CO-NH-), 3.35 (q, 4H, -CH2CH2NH-CO-NH-), 2.82 (t, 4H, -CH2CH2NH-CO-NH-); 13C-NMR (DMSO-d6, 75 MHz, δ ppm): 155.76, 144.37, 142.47, 134.73, 129.65, 126.19, 118.88, 40.76, 36.11.
4,4'-(((((Methylenebis(4,1-phenylene))bis(azanediyl))bis(carbonyl))bis(azanediyl))bis(ethane-2,1-diyl))dibenzenesulfonamide (17)
Yield: 86%; Colour: white solid; Melting Point: 270–272 °C; FT-IR (cm−1): 3361, 3335, 3268 (NH), 1636 (C=O), 1341, 1161 (symmetric) (S=O); 1H-NMR (DMSO-d6, 300 MHz, δ ppm): 8.40 (s, 2H, -CH2CH2NH-CO-NH-), 7.78 (d, J = 8.4 Hz, 4H, Ar-H), 7.42 (d, J = 8.4 Hz, 4H, Ar-H), 7.33 (s, 4H, -SO2NH2), 7.28 (d, J = 8.7 Hz, 4H, Ar-H), 7.04 (d, J = 8.4 Hz, 4H, Ar-H), 6.10 (t, 2H, -CH2CH2NH-CO-NH-), 3.75 (s, 2H, Ph-CH2-Ph), 3.35 (q, 4H, -CH2CH2NH-CO-NH-), 2.83 (t, 4H, -CH2CH2NH-CO-NH-); 13C-NMR (DMSO-d6, 75 MHz, δ ppm): 155.63, 144.53, 142.53, 138.79, 134.76, 129.64, 129.23, 126.19, 118.24, 40.70, 36.04.
4,4'-(((((3,3'-Dimethyl-[1,1'-biphenyl]-4,4'-diyl)bis(azanediyl))bis(carbonyl))bis(azanediyl))bis(ethane-2,1-diyl))dibenzenesulfonamide (18)
Yield: 85%; Colour: white solid; Melting Point: >300 °C; FT-IR (cm−1): 3365, 3330, 3276 (NH), 1629 (C=O), 1334, 1151 (symmetric) (S=O); 1H-NMR (DMSO-d6, 300 MHz, δ ppm): 7.89 (d, J = 8.4 Hz, 2H, Ar-H), 7.80 (d, J = 8.1 Hz, 4H, Ar-H), 7.73 (s, 2H, -CH2CH2NH-CO-NH-), 7.46 (d, J = 8.4 Hz, 4H, Ar-H), 7.40–7.35 (m, 8H, Ar-H and -SO2NH2), 6.62 (t, 2H, -CH2CH2NH-CO-NH-), 3.40 (q, 4H, -CH2CH2NH-CO-NH-), 2.86 (t, 4H, -CH2CH2NH-CO-NH-), 2.24 (s, 6H, -CH3); 13C-NMR (DMSO-d6, 75 MHz, δ ppm): 155.82, 144.40, 142.51, 137.55, 133.95, 129.69, 128.17, 127.55, 126.21, 124.22, 121.27, 40.75, 36.08, 18.57.
4,4'-(((((4-Methyl-1,3-phenylene)bis(azanediyl))bis(carbonyl))bis(azanediyl))bis(ethane-2,1-diyl))dibenzenesulfonamide (19)
Yield: 79%; Colour: white solid; Melting Point: 245–247 °C; FT-IR (cm−1): 3344, 3309, 3261 (NH), 1627 (C=O), 1330, 1152 (symmetric) (S=O); 1H-NMR (DMSO-d6, 300 MHz, δ ppm): 8.41 (s, 1H, -CH2CH2NH-CO-NH-), 7.78 (dd, J1 = 8.4 Hz, J2 = 1.5 Hz, 5H, Ar-H), 7.59 (s, 1H, Ar-H), 7.43 (dd, J1 = 8.4 Hz, J2 = 2.4 Hz, 4H, Ar-H), 7.34 (s, 4H, -SO2NH2), 7.13 (d, J = 8.7 Hz, 1H, Ar-H), 6.95 (d, J = 8.7 Hz, 1H, Ar-H), 6.59 (t, 1H, -CH2CH2NH-CO-NH-), 6.02 (t, 1H, -CH2CH2NH-CO-NH-), 3.38(q, 4H, -CH2CH2NH-CO-NH-), 2.85 (t, 4H, -CH2CH2NH-CO-NH-), 2.01 (s, 3H, -CH3); 13C-NMR (DMSO-d6, 75 MHz, δ ppm): 155.75, 155.64, 144.37, 142.45, 138.95, 138.55, 130.44, 129.67, 126.21, 119.83, 112.10, 110.70, 40.73, 36.10, 17.70.
CA inhibition
An SX.18 MV-R Applied Photophysics (Oxford, UK) stopped-flow instrument has been used to assay the catalytic/inhibition of various CA isozymesCitation31. Phenol Red (at a concentration of 0.2 mM) has been used as an indicator, working at the absorbance maximum of 557 nm, with 10 mM Hepes (pH 7.4) as a buffer, 0.1 M Na2SO4 or NaClO4 (for maintaining constant the ionic strength; these anions are not inhibitory in the used concentration), following the CA-catalyzed CO2 hydration reaction for a period of 5–10 s. Saturated CO2 solutions in water at 25 °C were used as substrate. Stock solutions of inhibitors were prepared at a concentration of 10 mM (in DMSO-water 1:1, v/v) and dilutions up to 0.01 nM done with the assay buffer mentioned above. At least 7 different inhibitor concentrations have been used for measuring the inhibition constant. Inhibitor and enzyme solutions were pre-incubated together for 10 min at room temperature prior to assay, in order to allow for the formation of the E-I complex. Triplicate experiments were done for each inhibitor concentration, and the values reported throughout the paper is the mean of such results. The inhibition constants were obtained by nonlinear least-squares methods using the Cheng-Prusoff equation, as reported earlierCitation32–37, and represent the mean from at least three different determinationsCitation38–45. All CA isozymes used here were recombinant proteins obtained as reported earlier by our group and their concentrations were in the range of 6–14 nMCitation38–Citation45.
Results and discussion
General synthesis and design strategy of the compounds
In recent studies, CAIs having bifunctional pharmacophores in their structures were reported, in order to improve biological activity and selectivity of compounds by ditopic interactions on the active site of the same or different enzyme(s)Citation46–50. In the context of the bis-substituted CAI design approach, we focussed on the development of a new bis-ureido-substituted benzenesulfonamides. Our aim was to produce molecules which have two binding groups in their structure and the ureido linker in between them to investigate isoform selectivity and potency of compounds by a potentially synergistic/multivalent effect. On the other hand, we aimed to find the relationship between the effect of the zinc binding group (primary sulphonamide part) and the linker position and length (ureido part) against various CA isozyme of pharmacologic relevance. To achieve this, we have used three well known primary benzenesulfonamide pharmacophores namely, sulphanilamide, metanilamide and 4-(2-aminoethyl)benzenesulfonamide which have been converted to aromatic bis-ureido derivatives (8-19). Hence, these primary benzenesulfonamide derivatives were condensed with four different aromatic bis-isocyanate moieties under mild conditions to produce twelve novel bis-ureido-substituted benzenesulfonamides derivatives. The general synthetic route is shown in the Scheme 1. Briefly, acetonitrile was used as a solvent and the reaction temperature was from room temperature to 50°C, overnight.
Carbonic anhydrase inhibition
All the newly synthesised bis-ureido-substituted primary benzenesulfonamide derivatives were evaluated for their CA inhibition properties against two cytosolic off-target isoforms (hCA I and II) and two membrane-bound isoforms (hCA IX and XII) by using a stopped-flow assay.The well-known CAI drug acetazolamide (AAZ) was used as a standard for comparison, and all the obtained results are summarised in . The following structure-activity relationship (SAR) can be drawn from obtained carbonic anhydrase inhibition studies as shown in the .
Table 1. Inhibition data of human CA isoforms hCA I, hCA II, hCA IX and hCA XII with bis-ureido-substituted primary benzenesulfonamide derivatives (8–19) reported here and standard sulphonamide inhibitor Acetazolamide (AAZ) by a stopped flow CO2 hydrase assay.
In general, our designed compounds were less prone to inhibit the cytosolic off-target isoform hCA I, having Ki values of 68.1 to 9174 nM. The most potent compound was 11, which is structurally different from other linkers by having substitution on 1,3- position in the phenyl ring although other ones have 1,4-substitution. Compound 19 showed good inhibition which is also have same linker with compound 11 by having Ki value of 95.4 nM. The other off-target isoform hCA II was effectively to moderately inhibited by the synthesised bis-ureido-substituted primary benzenesulfonamides. Interestingly, the most potent compound against hCA II was also compound 11 and 19, as in hCA I inhibition, with a Ki values of 4.4 and 25.8 nM, respectively. Compound 11 showed the best inhibition value that observed in the present study against hCA II with Ki value of 4.4 nM.
The tumour-overexpressed isoforms hCA IX and XII were efficiently inhibited, in general, by our novel compounds. More specifically, compound 11 showed a great affinity against these isoforms with Ki values of 6.73 and 7.39 nM, respectively. On the other hand, another compound from the same linker series compound 19, was also shown great inhibition potency with Ki values of 8.92 and 5.02 nM, respectively. However, a better selectivity was observed for compound 19 against off target isoforms hCA I and hCA II. The remaining compounds in the present series also displayed great inhibition properties with Ki values ranging from 57.5 to 835 nM for hCA IX, and 27.7 to 429 nM for hCA XII.
Overall, the SAR results demonstrate that 4-aminobenzenesulfonamide scaffold is a better primary sulphonamide as compared to other counterparts in combination with the bis-isocyanate (Y4) which has 1,3-substitution among others.
Conclusions
A series of novel primary benzenesulfonamides incorporating bis-ureido moieties were synthesised through the conjugation of sulphonamides with aromatic bis-isocyanates under mild conditions using SLC-0111 as lead compound. These bifunctional CAI design strategy was applied to obtain potent and possibly selective inhibitors incorporating the ureido linker. The inhibition properties of the obtained compounds were evaluated against four selected CA isoforms, including cytosolic ones (hCA I and II), as well as the tumour-associated membrane-bound isoforms (hCA IX and XII). In general, these compounds showed weak hCA I inhibition with Kis ranging from 68.1 to 9174 nM and effective to moderate inhibition against another cytosolic isoform hCA II with Kis of 4.4 to 792 nM. The tumour-associated membrane-bound isoforms hCA IX and XII were effectively inhibited by some of these compounds with Kis in the range of 6.73–835 and 5.02–429 nM, respectively. Interestingly, compounds 11 and 19 were found to be potent derivatives against these isoforms in which both of them have the same ureido linker (1,3-substituted phenyl ring) in their structure. However, compound 19 was more selective against the off-target isoforms hCA I and II. As a result, the potent inhibition properties of these novel compounds against tumour-associated isoforms hCA IX and XII making these bis-ureido substituted compounds of interest for antimetastatic drug design research.
Supplemental Material
Download PDF (603.2 KB)Acknowledgement
TT is thankful to Gazi University for the support of her PhD studies. The authors are grateful to Gazi University BAP [Gazi University Scientific Research Projects Coordination Department grant no: FDK-2022-7551] for the financial support of the current work.
Disclosure statement
All authors except CTS report no conflict of interest. CT Supuran is Editor-in-Chief of the Journal of Enzyme Inhibition and Medicinal Chemistry. He was not involved in the assessment, peer review, or decision-making process of this paper. The authors have no relevant affiliations of financial involvement with any organisation or entity with a financial interest in or financial conflict with the subject matter or materials discussed in the manuscript. This includes employment, consultancies, honoraria, stock ownership or options, expert testimony, grants or patents received or pending, or royalties.
References
- Alterio V, Di Fiore A, D'Ambrosio K, Supuran CT, De Simone G. Multiple binding modes of inhibitors to carbonic anhydrases: how to design specific drugs targeting 15 different isoforms? Chem Rev 2012;112(8):4421–4468.
- Neri D, Supuran CT. Interfering with pH regulation in tumours as a therapeutic strategy. Nat Rev Drug Discov. 2011;10(10):767–777.
- Supuran CT. Carbonic anhydrases: novel therapeutic applications for inhibitors and activators. Nat Rev Drug Discov. 2008;7:168–181.
- Supuran CT. Advances in structure-based drug discovery of carbonic anhydrase inhibitors. Expert Opin. Drug Discov. 2017;12(1):61–88.
- Supuran CT, Scozzafava A. Carbonic anhydrase inhibitors and their therapeutic potential. Expert Opin Ther Pat. 2000;10:575–600.
- Supuran CT. Carbonic anhydrase inhibitors and their potential in a range of therapeutic areas. Expert Opin Ther Pat. 2018;28:709–712.
- Nocentini A, Supuran CT. Carbonic anhydrase inhibitors as antitumor/antimetastatic agents: a patent review (2008-2018). Expert Opin Ther Pat. 2018;28:729–740.
- Shabana AM, Mondal UK, Alam R, et al. pH-Senstive multiligand gold nanoplatform targeting carbonic anhydrase IX enhances the delivery of Doxorubicin to hypoxic tumor spheroids and overcomes the hypoxia-induced chemoresistance. ACS Appl Mater Interfaces. 2018;10:17792–17808.
- Akocak S, Ilies MA. Next-Generation Primary Sulfonamide Carbonic Anhydrase Inhibitors. In: Supuran CT, Cappasso C, editors. Targeting carbonic anhydrases. London: Future Science; 2014. p. 35–51.
- Supuran CT. How many carbonic anhydrase inhibition mechanisms exist? J Enzym Inhib Med Chem. 2016;31(3):345–360.
- Zamanova S, Shabana AM, Mondal UK, Ilies MA. Carbonic anhydrase as disease markers. Expert Opin Ther Targets. 2019;29:509–533.
- Lou Y, McDonald PC, Oloumi A, et al. Targeting tumor hypoxia: suppression of breast tumor growth and metastasis by novel carbonic anhydrase IX inhibitors. Cancer Res. 2011;71(9):3364–3376.
- Pacchiano F, Aggarwal M, Avvaru BS, Robbins AH, Scozzafava A, McKenna R, Supuran CT. Selective hydrophobic pocket binding observed within the carbonic anhydrase II active site accommodate different 4-substituted-ureido-benzenesulfonamides and correlate to inhibitor potency. Chem Commun (Camb)). 2010;46(44):8371–8373.
- Pacchiano F, Carta F, McDonald PC, et al. Ureido-substituted benzenesulfonamides potently inhibit carbonic anhydrase IX and show antimetastatic activity in a model of breast cancer metastasis. J Med Chem. 2011;54(6):1896–1902.
- Supuran CT, Capasso C. The η-class carbonic anhydrases as drug targets for antimalarial agents. Expert Opin Ther Targets. 2015;19:551–563.
- Capasso C, Supuran CT. Bacterial, fungal and protozoan carbonic anhydrases as drug targets. Expert Opin Ther Targets. 2015;19:1689–1704.
- Supuran CT, Capasso C. Biomedical applications of prokaryotic carbonic anhydrases. Expert Opin Ther Pat. 2018;28:745–754.
- Akocak S, Supuran CT. Activation of α-, β-, γ- δ-, ζ- and η- class of carbonic anhydrases with amines and amino acids: a review. J Enzy Inhib Med Chem. 2019;34(1):1652–1659.
- Del Prete S, Vullo D, Scozzafava A, et al. Cloning, characterization and anion inhibition study of the δ-class carbonic anhydrase (TweCA) from the marine diatom Thalassiosira weissflogii. Bioorg Med Chem. 2014;22:531–537.
- Vullo D, Del Prete S, Osman SM, et al. Sulfonamide inhibition studies of the δ-carbonic anhydrase from the diatom Thalassiosira weissflogii. Bioorg Med Chem Lett. 2014;24:275–279.
- Del Prete S, Vullo D, Del Luca V, et al. Biochemical characterization of the δ-carbonic anhydrase from the marine diatom Thalassiosira weissflogii. TweCA. J Enzy Inhib Med Chem. 2014;29:906–911.
- Supuran CT, Capasso C. An overview of the bacterial carbonic anhydrases. Metabolites. 2017;7:56.
- Del Prete S, Vullo D, Fisher GM, et al. Discovery of new family of carbonic anhydrases in the malaria pathogen Plasmodium falciparum- the η-carbonic anhydrases. Bioorg Med Chem. 2014;24:4389–4396.
- De Simone G, Di Fiore A, Capasso C, Supuran CT. The zinc coordination pattern in the η-carbonic anhydrase from Plasmodium falciparum is different from all other carbonic anhydrase genetic families. Bioorg Med Chem Lett. 2015;25:1385–1389.
- Supuran CT, Scozzafava A, Jurca BC, Ilies MA. Carbonic anhydrase inhibitors - Part 49: Synthesis of substituted ureido and thioureido derivatives of aromatic/heterocyclic sulfonamides with increased affinities for isozyme I. Eur J Med Chem. 1998;33:83–93.
- Mishra CB, Kumari S, Angeli A, et al. Design, synthesis and biological evaluation of N-(5-methyl-isoxazol-3-yl/1,3,4-thiadiazol-2-yl)-4-(3-substitutedpheylureido) benzenesulfonamides as human carbonic anhydrase isoenzymes I, II, VII and XII inhibitors. J Enzy Inhib Med Chem. 2016;31(52):174–179.
- Moeker J, Peat TS, Bornaghi LF, Vullo D, Supuran CT, Poulsen S-A. Cyclic secondary sulfonamides: unusually good inhibitors of cancer-related carbonic anhydrase enzymes. J Med Chem. 2014;57(8):3522–3531.
- Akocak S, Alam MR, Shabana AM, et al. PEGylated Bis-Sulfonamide Carbonic Anhydrase Inhibitors Can Efficiently Control the Growth of Several Carbonic Anhydrase IX-Expressing Carcinomas. J Med Chem. 2016;59(10):5077–5088.
- Akocak S, Lolak N, Nocentini A, et al. Synthesis and biological evaluation of novel aromatic and heterocyclic bis-sulfonamide Schiff bases as carbonic anhydrase I, II,VII, and IX inhibitors. Bioorg Med Chem. 2017;25:3093–3097.
- Lolak N, Akocak S, Turkes C, et al. Synthesis, characterization,inhibition effects, and molecular docking studies as acetylcholinesterase, α-glycosidase, and carbonic anhydrase inhibitors of novel benzenesulfonamides incorporating 1,3,5-triazine structural motifs. Bioorg Chem. 2019;100:103897.
- Khalifah RG. The carbon dioxide hydration activity of carbonic anhydrase. I. Stop-flow kinetic studies on the native human isoenzymes B and C. J Biol Chem. 1971;246(8):2561–2573.
- Draghici B, Vullo D, Akocak S, Walker EA, Supuran CT, Ilies MA. Ethylene bis-imidazoles are highly potent and selective activators for isozymes VA and VII of carbonic anhydrase, with a potential nootropic effect. Chem Commun. 2014;50(45):5980–5983.
- Akocak S, Lolak N, Vullo D, Durgun M, Supuran CT. Synthesis and biological evaluation of histamine Schiff bases as carbonic anhydrase I, II, IV, VII and IX activators. J Enzy Inhib Med Chem. 2017;32:1305–1312.
- Akocak S, Lolak N, Bua S, et al. α-Carbonic anhydrases are strongly activated by spinaceamine derivatives. Bioorg Med Chem. 2019;27:800–804.
- Akocak S, Lolak N, Bua S, Nocentini A, Supuran CT. Activation of human α-carbonic anhydrase isoforms I, II, IV, and VII with bis-histamine Schiff bases and bis-spinaceamine substituted derivatives. J Enzy Inhib Med Chem. 2019;34(1):1193–1198.
- Akocak S, Lolak N, Bua S, Turel I, Supuran CT. Synthesis and biological evaluation of novel N,N′-Diaryl Cyanoguanidines acting as potent and selective carbonic anhydrase II inhibitors. Bioorg Chem. 2018;77:245–251.
- Lolak N, Akocak S, Bua S, Supuran CT. Design, synthesis and biological evaluation of ureido benzenesulfonamides incorporating 1,3,5-triazine moieties as potent carbonic anhydrase IX inhibitors. Bioorg Chem. 2019;82:117–122.
- Lolak N, Akocak S, Bua S, et al. Discovery of new ureido benzenesulfonamides incorporating 1,3,5-triazine moieties as carbonic anhydrase I, II, IX and inhibitors. Bioorg Med Chem. 2019;27(8):1588–1594.
- Lolak N, Akocak S, Bua S, Koca M, Supuran CT. Design and synthesis of novel 1,3-diaryltriazene-substituted sulfonamides as potent and selective carbonic anhydrase II inhibitors. Bioorg Chem. 2018;77:542–547.
- Akocak S, Lolak N, Bua S, Supuran CT. Discovery of novel 1,3-diaryltriazene sulfonamides as carbonic anhydrase I, II, VII and IX inhibitors. J Enzy Inhib Med Chem. 2018;33:1575–1580.
- Kucukbay H, Gonul Z, Kucukbay FZ, et al. Synthesis of new 7-amino-3,4-dihydroquinolin-2(1H)-one-peptide derivatives and their carbonic anhydrase enzyme inhibition, antioxidant, and cytotoxic activities. Arch Pharm. 2021;354(11):2100122.
- Kucukbay H, Parladi FM, Kucukbay FZ, et al. Synthesis, antioxidant and carbonic anhydrase inhibitory properties of monopetide-anthraquinone conjugates. Org Commun. 2021;14(3):255–269.
- Onyilmaz M, Koca M, Bonardi A, Degirmenci M, Supuran CT. Isocoumarins: a new class of selective carbonic anhydrase IX and XII inhibitors. J Enzy Inhib Med Chem. 2022;37(1):743–748.
- Durgun M, Turkes C, Isik M, et al. Synthesis, characterisation, biological evaluation and in silico studies of sulphonamide Schiff bases. J Enzy Inhib Med Chem. 2020;35(1):950–962.
- Ilies MA, Vullo D, Pastorek J, et al. Carbonic anhydrase inhibitors. Inhibition of tumor-associated isozyme IX by halogenosulfanilamide and halogenophenylaminobenzolamide derivatives. J Med Chem. 2003;46(11):2187–2196.
- Larcher A, Nocentini A, Supuran CT, et al. Bis-benzoxaboroles: Design, synthesis, and biological evaluation as carbonic anhydrase inhibitors. ACS Med Chem Lett. 2019;10(8):1205–1210.
- Mondal UK, Doroba K, Shabana AM, et al. PEG linker length strongly affects tumor cell killing by PEGylated carbonic anhydrase inhibitors in hypoxic carcinomas expressing carbonic anhydrase IX. Int J Mol Sci. 2021;22(3):1120.
- Kurt BZ, Dag A, Dogan B, et al. Synthesis, biological activity and multiscale molecular modeling studies of bis-coumarins as selective carbonic anhydrase IX and XII inhibitors with effective cytotoxicity against hepatocellular carcinoma. Bioorg Chem. 2019;87:838–850.
- Carta F, Dumy P, Supuran CT, Winum JY. Multivalent carbonic anhydrase inhibitors. Int J Mol Sci. 2019;20(21):5352.
- Lolak N, Akocak S, Durgun M, et al. Novel bis‑ureido‑substituted sulfaguanidines and sulfisoxazoles as carbonic anhydrase and acetylcholinesterase inhibitors. Mol Divers. 2022:1–15.