Abstract
Coronavirus disease 2019 (COVID-19) is an infectious disease caused by severe acute respiratory syndrome coronavirus-2 (SARS-CoV-2). 3CLpro is a key enzyme in coronavirus proliferation and a treatment target for COVID-19. In vitro and in silico, compounds 1–3 from Glycyrrhiza uralensis had inhibitory activity and binding affinity for 3CLpro. These compounds decreased HCoV-OC43 cytotoxicity in RD cells. Moreover, they inhibited viral growth by reducing the amounts of the necessary proteins (M, N, and RDRP). Therefore, compounds 1–3 are inhibitors of 3CLpro and HCoV-OC43 proliferation.
Introduction
Glycyrrhiza uralensis (G. uralensis) belongs to the Leguminosae family, and its rhizomes are used as herbal medicines.Citation1 The main components of this plant include glycyrrhizin (a triterpenoid saponin) and other polyphenols (liquritin, liquirtin apioside, isoliqurititin aposide, licoisoflavone A, and glycyrol).Citation2,Citation3 Glycyrrhizin, an FDA-approved natural sweetening compound, has antioxidant, antimicrobial, antifungal, and antiviral activities.Citation4,Citation5 Licochalcone A, isoliquiritigen, and liquiritigenin at 20 µM increase UCP1 expression, thereby promoting 3T3-L1 adipocyte differentiation.Citation6 Moreover, several polyphenols at 10 µM increase Nrf2 expression; inhibit tyrosinase and PTP1B activity; and suppress NO production, NF-κB transcription, and the proliferation of HepG2, SW480, A549, and MCF7 cancer cells.Citation7
Coronavirus disease 2019 (COVID-19) is an infectious disease caused by severe acute respiratory syndrome coronavirus-2 (SARS-CoV-2).Citation8 COVID-19 is characterised by mild cold symptoms (fever, cough, tiredness, and loss of taste or smell; less commonly sore throat, headache, and diarrhea), and can be fatal, particularly in older people or those with underlying diseases.Citation9 As of 10 March 2023, there had been a total of 759 408 703 COVID-19 cases and 6,866,434 deaths globally.Citation9
SARS-CoV-2, of the Coronaviridae, is a positive-sense single-stranded RNA virus with a genome of ∼30,000 nucleotides and ∼ 89.1% similarity to SARS-CoV.Citation10 After entering the cell, the virus releases RNA, which is then translated into two polyproteins (pp1a and pp1ab).Citation11 These have two cysteine proteases, the papain-like protease (PLpro) and the chymotrypsin-like protease (3CLpro).Citation11 Especially, the latter is one of the most representative target enzymes for the development of SARS-CoV-2 inhibitors.Citation12 Polyproteins are cleaved into non-structure proteins by 3CLpro, which are key factors in virus propagation.Citation13,Citation14 Thus, 3CLpro is a target for the development of therapeutics.Citation15
We aimed to discover 3CLpro and HCoV-OC43 inhibitors from rhizomes of G. uralensis. Compounds 1–3 inhibited 3CLpro in vitro and in silico and affected HCoV-OC43 proliferation. Therefore, these compounds have potential as inhibitors of 3CLpro and HCoV-OC43.
Materials and methods
General experimental procedures
NMR was conducted using a Bruker DRX-400 spectrometer (Bruker, Germany), with a chemical shift referenced to residual solvent signals and using MeOH-d4 as the solvent. Thin-layer chromatography (TLC) was performed on silica-gel 60 F254 and RP-18 F254S plates (0.25 mm layer thickness; Merck, Darmstadt, Germany).Citation16 Purified compounds were visualised on two TLC plates at a wavelength of 254 nm. Silica gel (60 A, 70–230 or 230–400 mesh ASTM; Merck) and reversed-phase silica gel (ODS-A 12 nm S-150, S-75 µm; YMC Co., Kyoto, Japan) were used for open-column chromatography. SARS-CoV-2 3CLpro was purchased from Sigma Aldrich (St. Louis, MO, USA). DABCY-LKTSAVLQSGFRKME-EDANS was synthesised by Anygen (Gwangju, Republic of Korea [ROK]).Citation17
Plant material
Rhizomes of G. uralensis were harvested from a farm in Eumsung, Chungcheongbuk-do, ROK in October 2015 and identified by Dr. Jeonghoon Lee. A voucher specimen (GUR151000_1) was deposited at the herbarium of the Department of Herbal Crop Research, National Institute of Horticultural and Herbal Science, ROK.
Extraction and isolation
Rhizomes of G. uralensis (5 kg) were extracted thrice with 95% ethanol for 2 weeks. The solution was evaporated under reduced pressure to give an ethanol extract (∼ 240 g). The concentrated extract was suspended in distilled water and divided into chloroform (∼ 80 g) and ethyl acetate (∼ 85 g) fractions. The chloroform fraction was subjected to silica gel column chromatography using a gradient solvent system of chloroform and methanol (10:0 to 0:10) to yield four fractions (1–4). Fraction 1 was chromatographed on a C-18 column with a solvent system of water and methanol (60:40 to 20:80) to produce compound 1 (∼5 mg). Fraction 2 was chromatographed on a silica gel column with a solvent system of chloroform and methanol (9:1 to 1:9) to yield three fractions (21–23). Compounds 2 (∼ 50 mg) and 3 (∼20 mg) were purified from fraction 22 by C-18 column chromatography with a solvent system of water and methanol (60:40 to 20:80).
3CLpro inhibition assay
This assay was performed as described previously with modification.Citation16,Citation18 3CLpro (140 µL; 13.3 µg/mL) in 20 mM Tris-HCl buffer (pH 7.5) was mixed with 10 µL of MeOH or compound in MeOH (1.56–100 µM) in a 96-well plate, and 50 µL of 100 µM substrate was added. After starting the reaction at 37 °C, the product was quantified using a fluorescence spectrophotometer at emission and excitations wavelengths of 530 and 340 nm, respectively, with a gain of 85 for 30 min.Citation17
Molecular docking
This study was performed as described by Kim et al.Citation17 The three-dimensional (3D) structures of the compounds were constructed and minimised using Chem3D Pro (CambridgeSoft, Cambridge, MA, USA). The 3D structure of SARS CoV-2 3CLpro coded in 7END was downloaded from the RCSB Protein Data Bank. Water and ligand (J7R) were excluded from the 3D structure. Hydrogen was added to the protein using AutoDockTools (Scripps Research, La Jolla, CA, USA); the Gasteiger charge model was then applied.Citation16 Flexible ligand docking was achieved using a torsion tree, with the detection of the torsion root and rotatable bonds. The grid box was set to a size of 60 × 60 × 60 at 0.375 Å for docking the ligand into the active site. Molecular docking was achieved via a Lamarckian genetic algorithm with the maximum number of evaluations. The resulting values were calculated and represented using Chimaera 1.14 (San Francisco, CA, USA), and LIGPLOT (European Bioinformatics Institute, Hinxton, UK).Citation16,Citation17
Molecular dynamics
Molecular dynamics analysis was performed as described previously.Citation17 The Gromacs 4.6.5 package was used to simulate the complex of 3CLpro with polyphenols. The complex was charged by a Gromos96 54a6 force field. Ligand topology was generated by The GlycoBioChem PRODRG Server. The charged complex was dissolved in water in a cubic box using the simple point charge water model and ionised with sodium. mdp files were generated following the instructions for GROMACS.Citation19 The mdp files were minimised to a maximal force of 10 kJ/mol using the steepest-descent method. The product was further equilibrated by 300 K NVT in 1 bar NPT for 100 ps. Finally, a molecular dynamics simulation was conducted for 30 ns. The results were analysed using g_utility. The data were visualised using SigmaPlot (San Jose, CA, USA) and Chimaera (San Francisco, CA, USA).Citation16,Citation17,Citation19
Cell culture and plaque formation assay
RD cells were maintained in MEM (Welgene, Seoul, ROK) containing 10% foetal bovine serum (FBS, Thermo Fisher Scientific, Waltham, MA, USA) and 1% penicillin-streptomycin solution (Welgene).Citation17,Citation20 RD cells were infected with HCoV-OC43 at the indicated dilutions of medium from virus-infected cells, and the infected cells were maintained in MEM containing 2% FBS and 1% penicillin-streptomycin. Cell viability was measured by 3–(4,5-dimethylthiazol-2-yl)-2,5-diphenyltetrazolium bromide (MTT) assay. HCoV-OC43 virus was obtained from the ATCC (Rockville, MD, USA) and RD cells from the Korean Cell Line Bank (KCLB, Seoul, ROK). MTT was purchased from USB Corporation (Cleveland, OH, USA). Plaque formation assay was performed to enumerate the virus.Citation16,Citation20 RD cells were seeded in a 12-well plate 1 day prior to infection. Medium-containing coronavirus was diluted 10-fold in MEM (Welgene) with 2% FBS (Thermo Fisher Scientific) and 1% penicillin-streptomycin (Welgene). A diluted medium was added to RD cells, which were subsequently incubated for 1 h at 33 °C in 5% CO2. An overlay medium containing 0.6% agarose was added to each well and incubated for 3 days. Next, the cells were fixed in 10% formaldehyde and stained with 1% crystal violet to visualise plaques.Citation16,Citation20
Western blot and quantitative RT-PCR
For western blotting, cells and medium were added to the buffer (150 mM NaCl, 50 mM HEPES [pH 7.5], and 1% NP40) containing a protease inhibitor cocktail (Roche, Basel, Switzerland). Equal amounts of samples were resolved by SDS-PAGE and transferred to PVDF membranes (Bio-Rad, Hercules, CA, USA). Viral proteins were detected using a primary anti-HCoV-OC43 antibody (1:5000 dilution) and an ECL system (Dogen, Seoul, ROK). Images were acquired using a Chemidoc (Bio-Rad).Citation16,Citation20
For quantitative RT-PCR, cells and media were collected and RNA was extracted using TRIzol (Thermo Fisher Scientific) in accordance with the manufacturer’s instructions. RT-PCR was performed using the StepOnePlus™ Real-Time PCR System (Thermo Fisher Scientific). The HCoV-OC43 RdRp gene was amplified using the forward primer 5′-GAGTGTAGATGCCCGTCTCG-3′ and reverse primer 5′-TGTGGCACACGACTACCTTC-3′. The HCoV-OC43 M gene was amplified using the forward primer 5′-ACGGTCACAATAATACGCGGT-3′ and reverse primer 5′-GGGTTGATGGCAGTCG GTAA-3′. The HCoV-OC43 N gene was amplified using the forward primer 5′-AGGATGCCACCAAACCTCAG-3′ and reverse primer 5′-TGGGGAACTGTGGGTCACT A-3′.Citation16,Citation20
Statistical analysis
All measurements were performed in triplicate across three independent experiments, and the results are shown as mean ± standard error of the mean (SEM). The results of MTT were evaluated by a 2-tailed Student’s t-test using Excel software (Microsoft, Redmond, WA, USA). The results were analysed using Sigma Plot (Systat Software Inc., San Jose, CS, USA).
Results
Isolation and identification of compounds 1–3 from G. uralensis
Ethanol extract from rhizomes of G. uralensis was divided into chloroform, ethyl acetate, and water fractions. The chloroform fraction was purified by open-column chromatography to yield compounds 1–3. Their chemical structures were elucidated by 1H- and 13C-nuclear magnetic resonance and compared with prior reports. The compounds were identified as 2′-methoxyisoliquiritigenin (1)Citation21, glycycoumarin (2)Citation22, and isoangustone A (3)Citation23 ().
The inhibitory activity of compounds 1–3 on SARS-CoV-2 3CLpro
The inhibitory activities of compounds 1–3 towards SARS-CoV-2 3CLpro were evaluated. The amount (increased fluorescence intensity) of the substrate (DABCYL-KTSAVLQVSGFRKME-EDANS) hydrolysed by 3CLpro was determined. The three compounds inhibited enzyme activity in a dose-dependent manner (IC50 values 8.2 ± 1.5, 0.6 ± 0.2, and 5.2 ± 0.9 µM, respectively; and ).
Figure 2. The inhibitory activity of compounds on SARS-CoV-2 3CLpro (A). The best pose (B) and docking results (C–E) between inhibitors (1: red, 2: yellow, 3: green) and 3CLpro (ID: 7END).
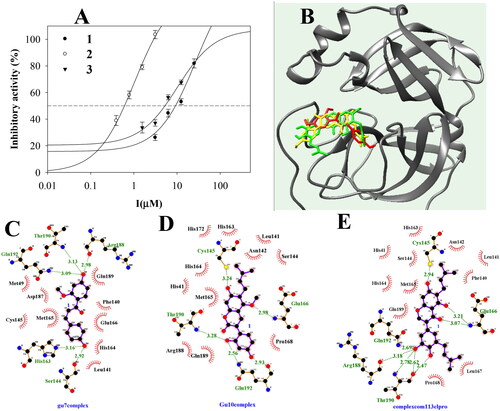
Table 1. The inhibitory activity and docking results of inhibitors towards SARS-CoV-2 3CLpro.
The predicted binding site of inhibitors with 3CLpro
To assess the predicted interactions of the compounds with SARS-CoV-2 3CLpro, a molecular docking analysis was performed. Flexible ligands were stably docked in similar positions of the active site with Autodock scores of −7.31, −9.62, and −9.83 kcal/mol, respectively ( and ). Compound 1 had five hydrogen bonds with His162 (3.16), Ser144 (2.92 Å), Arg188 (2.98 Å), Thr190 (3.13 Å), and Gln192 (3.09 Å). Compound 2 had five hydrogen bonds with Cys145 (3.24 Å), Glu166 (2.98), Thr190 (3.28 Å), and Gln192 (2.56 Å, 2.93 Å). Compound 3 formed eight hydrogen bonds with five amino acids–Cys145 (2.94 Å), Glu166 (3.07 Å, 3.21 Å), Arg188 (3.18 Å), Thr190 (2.47 Å, 2.62 Å, 2.78 Å), and Gln192 (2.69 Å).
Molecular dynamics study of the inhibitors with SARS-CoV-2 3CLpro
To evaluate inhibitor (I)–enzyme (E) interactions, I + E complexes with ions and water were subjected to molecular dynamics analysis. Compounds 1–3 moved to the active site according to the fluid movement of SARS-CoV-2 3CLpro (). Compounds 1, 2, and 3 showed protein-based root-mean-square deviation (RMSD) values of 0.5, 0.4, and 0.3 nm, respectively (), and their root-mean-square deviation (RMSF) values had similar shapes (). The compounds consisted of 1–2 (1), 1–4 (2), and 1–2 (3) hydrogen bonds (). Compounds 1 and 2 maintained a ∼ 3.5 Å distance from Glu166, Thr190, and Gln192. Inhibitor 3 showed movement at a ∼ 4 Å distance with two Glu166 and Gln192 ().
Figure 3. The superposition of inhibitors 1–3 with sEH for the 30 ns (red: 0 ns, orange: 3 ns, yellow: 6 ns, green: 9 ns, cyan: 12 ns, blue: 15 ns, conflower blue: 18 ns, purple: 21 ns, hot pink: 24 ns, magenta: 27 ns, black: 30 ns) (A-C). The RMSD (D), RMSF (E), and hydrogen bonds (F–H) of the simulation. The distance of key residues (I) with inhibitors 10 with sEH(J–L).
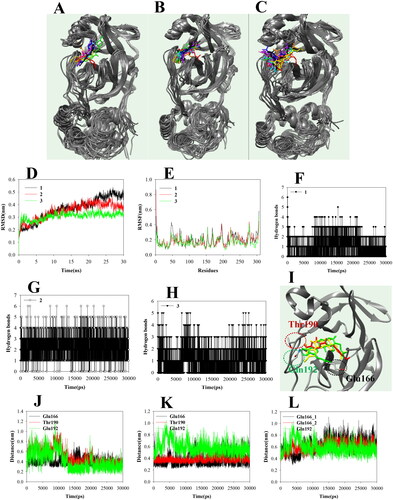
The decreases of coronavirus-induced cytotoxicity by compounds 1–3
Because compounds 1–3 inhibited SARS-CoV-2 3CLpro, we examined their effects on coronavirus replication and their cytotoxicity. Compounds 1–3 at 20 µM decreased cell viability, but at 1.25–10 µM did not show significant cytotoxicity (). Therefore, we used compounds 1–3 at ≤10 µM. HCoV-OC43-infected cells showed a viability rate of ∼60% that of uninfected cells. Compounds 1–3 reduced coronavirus-induced cytotoxicity in a dose-dependent manner (). Compound 2 at 5 µM resulted in a ∼ 90% viability rate but was cytotoxic at 10 µM. Therefore, compounds 1–3 suppressed coronavirus-induced cytotoxicity.
Figure 4. Compounds 1–3 treatment decreased HCoV-OC43-induced cytotoxicity. The cytotoxicity of compounds 1–3 was evaluated by MTT assay (A–C). RD cells were incubated with the indicated concentration of compounds 1–3 for 24 h. Compounds 1–3 treatment reduces the cytotoxicity of HCoV-OC43 infections (D–F). RD cells were infected with mock or HCoV-OC43 and cells were incubated with the indicated concentration of compound. Cell viability was evaluated with MTT assay.
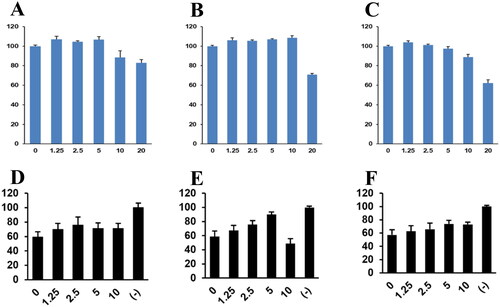
The inhibition and reduced plaque formation of coronavirus replication by compounds 1–3
The 3D-structure of 3CLpro of HCOV-OC43 was derived through molecular homology technology. As a result of docking the compounds 1–3 to the predictive model, a value similar to those of SARS-CoV-2 3CLpro was confirmed (Figure S4, S5, Table S1).
Figure 5. Compounds 1–3 inhibited the replication of HCoV-OC43 coronavirus. (A) Compounds 1–3 treatment decreased the expression of HCoV-OC43 protein in cells and media. RD cells were incubated with compounds 1–3 and infected with HCoV-OC43 (1 0 −3 dilution of conditioned media). Three days after infection, an equal amount of cell lysates (upper panel) and conditioned media (lower panel) were probed with anti-HCoV-OC43 antibody. (B) Compounds 1–3 treatment decreased the RNA level of HCoV-OC43 in media. RD cells were infected HCoV-OC43, and RNA was purified from the infected cell and media. The RNA level of RdRp gene, M gene and N gene was evaluated by quantitative RT-PCR.
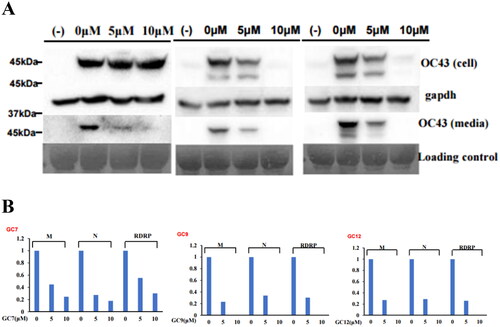
Because compounds 1–3 suppressed coronavirus-induced cytotoxicity, we hypothesised that they would inhibit coronavirus replication. Western blotting using an anti-OC43 antibody showed that compounds 1–3 decreased coronavirus protein levels in a dose-dependent manner (). Interestingly, compound 1 decreased the viral protein level in the conditioned medium but did not affect that in cell lysate, suggesting interference with the viral release (). Quantitative RT-PCR showed that the mRNA levels of the M, N, and RDRP proteins of HCoV-OC43 were decreased by compounds 1–3 (). Therefore, compounds 1–3 inhibited coronavirus replication. Because they inhibited coronavirus replication, we examined their effects on plaque formation. Compounds 1–3 decreased the number of plaques, indicating inhibition of coronavirus replication ().
Discussion
Four coronaviruses (229E, OC43, NL63, HKU1) are responsible for 10–30% of common cold cases, SARS-CoV in 2002, MERS-CoV in 2013, and SARS-CoV-2 in 2019.Citation24 Although the COVID-19 outbreak in 2019 had a ∼ 0.9% fatality rate, it has killed millions of people because of rapid SARS-CoV-2 transmission.Citation9 Despite the development of vaccines and drugs, these viruses continue to threaten public health.Citation9,Citation25 For this reason, phytochemists have focused on developing antiviral drugs from natural products.Citation26 Glycyrrhizin and glycyrrhetinic acid of Glycyrrhiza glabra suppress the proliferation and infection of RNA and DNA viruses.Citation27 We focused on polyphenols rather than triterpenoids in rhizomes of G. uralensis. Compounds 1–3 inhibited substrate cleavage by 3CLpro with IC50 values of 8.2 ± 1.5, 0.6 ± 1.2, and 5.2 ± 0.9 µM, respectively. Also, the three compounds showed similar binding forces at the active site. Compounds 1–3 had similar numbers of hydroxyl, ketone, and methyl groups, which can participate in hydrogen bonding. Molecular dynamics can provide insight into flexible drug-target interactions.Citation28 A force field based on Newton’s physics is responsible for a role calculating the atom forces and the energy of the complex.Citation28 The results showed that three (Glu166, Thr190, and Gln192) or two (Glu166 and Gln192) residues maintain a continuous distance during the binding of the inhibitor with 3CLpro in a fluid state. Glu166 participated in hydrogen bonding to the three compounds. The inhibition of 3CLpro catalysis plays an important role in inhibitiing coronavirus replication.Citation13–Citation15 . It was confirmed through homology modelling that SARS-CoV-2 and HCoV-OC43, which are beta coronaviruses, have similar 3CLpro.Citation29 We evaluated the effects of compounds 1–3 on viral cytotoxicity and replication in HCoV-OC43-infected cells. RD cells were protected from infected HCoV-OC43 when treated with compounds 1 and 3 at 10 µM, and 2 at 5 µM. Compounds 2 and 3 reduced the HCoV-OC43 protein level in cells and media, and compound 1 only in the medium. Therefore, compound 1 is more effective in releasing viruses than inhibiting viral replication in infected cells. Moreover, the three compounds at 5 and 10 µM not only reduced the levels of M, N, and RDRP genes that were relicated by proteins cleaved from polyprotein by 3CLpro but also inhibited HCoV-OC43 proliferation, an effect confirmed by the results of plaque assays.
Methoxyisoliquiritigenin (1), glycycoumarin (2), and isoangustone A (3) from rhizomes of G. uralensis inhibited 3CLpro with IC50 values of 8.2 ± 1.5, 0.6 ± 0.2, and 5.2 ± 0.9 µM, respectively. In silico, compounds 1–3 stably bonded with Glu166, Thr190, and Gln192 and inhibited the production of M, N, and RDRP proteins during HCoV-OC43 proliferation. Finally, we confirmed the antiviral effects of these compounds by measuring viral protein expression, RNA expression, and plaque formation with the coronavirus model system ( and ). In conclusion, compounds 1–3 have potential as candidates or lead compounds for the development of therapies for coronavirus infection.
Supplemental Material
Download PDF (846.2 KB)Disclosure statement
No potential conflict of interest was reported by the author(s).
Additional information
Funding
References
- Guo D, Yin X, Wu D, Chen J, Ye X. Natural polysaccharides from Glycyrrhiza uralensis residues with typical glucan structure showing inhibition on α-glucosidase activities. Int J Biol Macromol. 2023; 224:776–785.
- van Dinteren S, Meijerink J, Witkamp R, van Ieperen B, Vincken J-P, Araya-Cloutier C. Valorisation of liquorice (Glycyrrhiza) roots: antimicrobial activity and cytotoxicity of prenylated (iso)flavonoids and chalcones from liquorice spent (G. glabra, G. inflata, and G. uralensis). Food Funct. 2022;13(23):12105–12120.
- Qiao X, Ji S, Yu S-w, Lin X-h, Jin H-w, Duan Y-k, Zhang L-r, Guo D-a, Ye M. Identification of key licorice constituents which interact with cytochrome P450: Evaluation by LC/MS/MS cocktail assay and metabolic profiling. Aaps J. 2014;16(1):101–113.
- Muthu SA, Sharma R, Qureshi A, Parvez S, Ahmad B. Mechanistic insights into monomer level prevention of amyloid aggregation of lysozyme by glycyrrhizic acid. Int J Biol Macromol. 2023;227:884–895.
- Langer D, Mlynarczyk DT, Dlugaszewska J, Tykarska E. Potential of glycyrrhizic and glycyrrhetinic acids against influenza type A and B viruses: a perspective to develop new anti-influenza compounds and drug delivery systems. Eur J Med Chem. 2023;246:114934.
- Lee HE, Yang G, Han S-H, Lee J-H, An T-J, Jang J-K, Lee JY. Anti-obesity potential of Glycyrrhiza uralensis and licochalcone A through induction of adipocyte browning. Biochem Biophys Res Commun. 2018;503(3):2117–2123.
- Li K, Ji S, Song W, Kuang Y, Lin Y, Tang S, Cui Z, Qiao X, Yu S, Ye M. Glycybridins A − K, bioactive phenolic compounds from Glycyrrhiza glabra. J Nat Prod. 2017;80(2):334–346.
- Keretsu S, Bhujbal SP, Cho SJ. Rational approach toward COVID‑19 main protease inhibitors via molecular docking, molecular dynamics simulation and free energy calculation. Sci Rep. 2020;10(1):17716.
- World Health Organization, Coronavirus disease (COVID-19). https://www.who.int/health - topics/coronavirus#tab=tab_1. (acessed data: 10 March 2023.
- Eberle RJ, Olivier DS, Amaral MS, Gering I, Willbold D, Arni RK, Coronado MA. The repurposed drugs suramin and quinacrine cooperatively inhibit SARS-CoV-2 3CLpro in vitro. Viruses. 2021;13:873.
- Citarella A, Scala A, Piperno A, Micale N. SARS-CoV Mpro: a potential target for peptidominetics and small-molecule inhibitors. Biomolecules. 2021;11:607.
- Xia Z, Sacco M, Hu Y, Ma C, Meng X, Zhang F, Szeto T, Xiang Y, Chen Y, Wang J. Rational design of hybrid SARS-CoV-2 main protease inhibitors guided by the superimposed cocrystal structures with the peptidomimetic inhibitors GC-376, Telaprevir, and Boceprevir. ACS Pharmacol Transl Sci. 2021;4(4):1408–1421.
- Melo-Filho CC, Bobrowski T, Martin H-J, Sessions Z, Popov KI, Moorman NJ, Baric RS, Muratov EN, Tropsha A. Conserved coronavirus proteins as target of broad-spectrum antivirals. Antiviral Res. 2022;204:105360.
- V’kovski P, Kratzel A, Steiner S, Stalder Thiel V. Coronavirus biology and replication: implications for SARS-CoV-2. Nat Rev. 2021;19:155–170.
- Nand M, Maiti P, Joshi T, Chandra S, Pande V, Kuniyal JC, Ramakrishnan MA. Virtual screening of anti‑HIV1 compounds against SARS‑CoV‑2: machine learning modeling, chemoinformatics and molecular dynamics simulation based analysis. Sci Rep. 2020;10(1):20397.
- Park Y-I, Kim JH, Lee S, Lee IS, Park J. Eupatin, a flavonoid, inhibits coronavirus 3CL protease and replication. Inter J Mol Sci. 2023;24:9221.
- Kim JH, Park Y-I, Hur M, Park WT, Moon Y-H, Koo SC, Her Y-C, Lee IS, Park J. The inhibitory activity of methoxyl flavonoids derived from Inula britannica flowers on SARS-CoV-2 3CLpro. Int J Biol Macromol. 2022;222(Pt B):2098–2104.
- Jo S, Kim S, Kim DY, Kim M-S, Shin DH. Flavonoids with inhibitory activity against SARS-CoV-2 3CLpro. J Enzyme Inhib Med Chem. 2020;35(1):1539–1544.
- Kim JH, Park JS, Lee YJ, Choi S, Kim YH, Yang SY. Inhibition of soluble epoxide hydrolase by phytochemical constituents of the root bark of Ulmus davidiana var. japonica. J Enzyme Inhib Med Chem. 2021;36(1):1049–1055.
- Jang M, Park R, Park Y-I, Cha Y-E, Yamamoto A, Lee JI, Park J. EGCC, a green tea polyphenol, inhibits human coronavirus replication in vitro. Biochem Biophys Res Commun. 2021;547:23–28.
- Ryu YB, Kim JH, Park S-J, Chang JS, Rho M-C, Bae KH, Park KH, Lee WS. Inhibition of neuraminidase activity by polyphenol compounds isolated from the roots of Glycyrrhiza uralensis. Bioorg Med Chem Lett. 2010;20(3):971–974.
- Gafner S, Bergeron C, Villinski JR, Godejohann M, Kessler P, Cardellina JH, Ferreira D, Feghali K, Grenier D. Isoflavonoids and coumarins from Glycyrrhiza uralensis: antibacterial activity against oral pathogens and conversion of isoflavans into isoflavan-quinones during purification. J Nat Prod. 2011;74(12):2514–2519.
- Seon MR, Lim SS, Choi HJ, Park SY, Cho HJ, Kim J-K, Kim J, Kwon DY, Yoon Park JH. Isoangustone A present in hexane/ethanol extract of Glycyrrhiza uralensis induces apoptosis in DU145 human prostate cancer cells via the activation of DR4 and intrinsic apoptosis pathway. Mol Nutr Food Res. 2010;54(9):1329–1339.
- Yang H, Rao Z. Structural biology of SARS-CoV-2 and implications for therapeutic development. Nat Rev Microbiol. 2021;19(11):685–700.
- Kumari M, Lu R-M, Li M-C, Huang J-L, Hsu F-F, Ko S-H, Ke F-Y, Su S-C, Liang K-H, Yuan JP-Y, Chiang H-L, Sun C-P, Lee I-J, Li W-S, Hsieh H-P, Tao M-H, Wu H-C. A critical overview of current progress for COVID-19: development of vaccines, antiviral drugs, and therapeutic antibodies. J Biomed Sci. 2022;29(1):68.
- Bachar SC, Mazumder K, Bachar R. Aktar A, Al Mahtab M. A review of medicinal plants with antiviral activity available in Bangladesh and mechanistic insight into their bioactive metabolites on SARS-CoV-2, HIV and HBV. Frontiers Pharm. 2021;122:732891.
- Diomede L, Beeg M, Gamba A, Fumagalli O, Gobbi M, Salmona M. Can antiviral activity of licorice help fight COVID-19 infection? Biomolecules. 2021;11:855.
- de Vivo M, Masett M, Bottegoni G, Cavalli A. Role of molecular dynamics and related methods in drug discovery. J Med Chem. 2016;59(9):4035–4061.
- Milani M, Donalisio M, Bonotto RM, Schneider E, Arduino I, Boni F, Lembo D, Marcello A, Mastrangelo E. Combined in silico and in vitro approaches identified the antipsychotic drug lurasidone and the antiviral drug elbasvir as SARS-CoV2 and HCoV-OC43 inhibitors. Antiviral Res. 2021;189:105055.