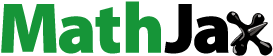
Abstract
A library of N-benzylpyridinium-based compounds, 7a-j and 8a-j, was designed and synthesised as potential acetylcholinesterase) AChE (inhibitors. An in vitro assay for the synthesised compounds showed that most compounds had significant AChE inhibitory activities at the nanomolar and submicromolar levels. The benzyl (8a) and fluoro (8b) derivatives were the most active, with IC50 values ≤56 nM. Compound 7f, which had a benzyl moiety, showed the highest potency among all the target compounds, with an IC50 value of 7.5 ± 0.19 nM against AChE, which was higher than that of the activities of tacrine (IC50 = 30 ± 0.2 nM) and donepezil (IC50 = 14 ± 0.12 nM). Compounds with vanillin moieties exhibited antioxidant activity. Among the tested compounds, four derivatives (7f, 7 g, 8f, and 8 g) exhibited superior AChE inhibitory activity, with Ki values of 6–16 nM, which were potent in the same range as the approved drug, donepezil. These compounds showed moderate antioxidant activities, as indicated by the results of the ABTS assay.
Introduction
Alzheimer’s disease (AD) is the most common form of dementia reported worldwide. In 2020 and 2021, AD was listed as the seventh leading cause of mortality in the world, making it one of the costliest illnesses in societyCitation1. Worldwide, there are only four approved drugs for AD, and they only treat the symptoms of moderate stages of the disease, not its cause and progression. Donepezil, rivastigmine, and galantamine treat AD by inhibiting cholinesterase (ChE), whereas memantine is an N-methyl-D-aspartate (NMDA) receptor antagonist. In June 2021, the U.S. Food and Drug Administration (USFDA) conditionally approved the antibody Aducanumab (Aduhelm™) as a therapy to address the underlying biology of AD. In January 2023, a new antibody, lecanemab, was approved by the USFDA. However, more studies addressing the efficacy and safety of these drugs are needed to confirm that they have more benefits than risksCitation2,Citation3. These antibodies target amyloid β (Aβ); nevertheless, there are other pathological factors of AD including the discrepancy of acetylcholine (ACh) in the hippocampus and cortex areas of the brain. According to the cholinergic hypothesis, the main pathological changes in AD are triggered by an extreme decline in AChCitation4 levels caused by the structural and functional loss of cholinergic neurons, which accelerates the neurodegenerative process. ACh is a neurotransmitter involved in signal transduction pathways related to memory and learning. Therefore, ACh deficiency leads to a progressive and significant loss of cognitive and behavioural functions in patients with ADCitation4. Human AChE is a prominent enzyme responsible for the degradation of ACh, which in turn blocks postsynaptic signal transmission. Additionally, it has been reported that AChE may function by accelerating Aβ formation and could play a role during amyloid deposition in the Alzheimer’s brainCitation5,Citation6. Hence, the approach of designing and synthesising novel AChE inhibitors is clinically valuable, especially if they can bind to both the catalytic active site (CAS) that is hydrophobic and the peripheral anionic site (PAS) of AChE because AChE interacts with β-amyloid through the PASCitation7.
Donepezil is the most potent approved drug for treating AD (1, ), and it significantly impacts several processes related to AD, such as cholinesterase activity, oxidative stress, and anti-Aβ aggregationCitation8. Therefore, many AChE inhibitors have been developed using the donepezil structure as a modelCitation7,Citation8 (2–5, ), a few of which are N-benzyl pyridinium compounds, such as compound 4Citation9 and compound 5Citation10.
In the donepezil and AChE-binding model, the benzyl piperidine moiety protonated at physiological pH can interact with CAS, and the indanone part of donepezil binds to PAS. Curcumin is a well-known natural product with many biological activities, including antioxidant and cognitive-enhancing activitiesCitation11. Curcumin has been reported to reduce amyloid formation and inhibit the formation of amyloid β aggregationsCitation12, and the curcumin part of the compound is thought to interact with the catalytic (hydrophobic) site of AChE. At the same time, the pyridinium moiety will go to the PAS. This is similar to the binding mode of donepezil to AChE, and since curcumin is a known antioxidant, we hypothesised that new analogous compounds derived from curcumin should be AChE inhibitors/antioxidant dual-acting compounds that target the multifactorial pathogenic nature of ADCitation13. This strategy is called multi-target directed ligands (MTDLs) and has been reported to be effective in treating ADCitation14. We developed a design strategy for the hybrid potential with the activity of the target compounds determined via an in vitro AChE inhibition assay using Ellman’s method and an ABTS antioxidant assay ().
Results and discussion
Chemistry
Scheme 1 shows the synthesis of the α,β-unsaturated carbonyl compounds 12a, 12b, 13a and 13b using the classical base-mediated Claisen–Schmidt condensation of (E)-4-phenylbut-3-en-2-one (9) or (E)-4–(4-hydroxy-3-methoxyphenyl)but-3-en-2-one (10) with pyridinecarboxaldehyde 11a or 11b. The target benzyl pyridinium salts 7a-j and 8a-j were then synthesised by the reaction of p-substituted benzyl pyridinium bromides with 12a, 12b, 13a, or 13b in refluxed acetonitrileCitation9 (Scheme 2).
In vitro AChE and BuChE inhibition
All target compounds, along with the known AD drugs tacrine and donepezil, were tested for their ability to inhibit acetylcholinesterase (eeAChE, from Electrophorus electricus) and butyrylcholinesterase (eqBChE, from equine serum) (). Both enzymes are widely used to screen compound series because they exhibit high amino acid sequence homology with human orthologsCitation15,Citation16 in addition to their stability and low cost in comparison with human enzymes. The IC50 values for the tested compounds were determined, and those with the lowest IC50 values were used in kinetic studies to determine the Ki values of their inhibition.
Table 1. AChE and BChE inhibition, ChEs selectivity, and Ki values for known and target compounds.
In addition to determining their inhibitory activities, the most powerful inhibitors, 7a, 7b, 7f, 7 g, 8a, 8b, 8f, and 8 g, were used for kinetic studies to determine their Ki values, and double reciprocal Lineweaver-Burk plots were produced (provided in the SI). shows the Lineweaver-Burk plot of compound 7a, illustrating that as the inhibitor concentration increased, the slopes and intercepts increased. This pattern indicated a mixed-type inhibition, suggesting that the inhibitor may be able to bind to either the free enzyme or the enzyme-substrate complex, similar to donepezilCitation17 and tacrineCitation18.
Figure 3. Lineweaver-Burk plot of the substrate-velocity curve of AChE activity with different substrate (ATC) concentrations (32–2 mM) in the absence and presence of compound 7a.
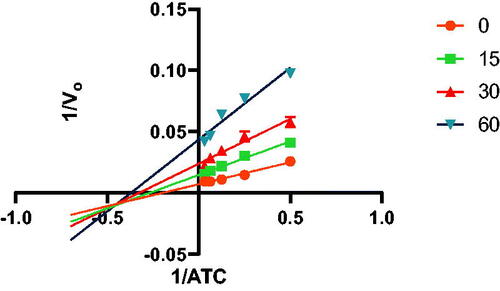
Structurally, compounds 7a-j and 8a-j were 4-pyridinium and 3-pyridinium derivatives, respectively. Derivatives 7f-j and 8j-f have curcumin residues in their structure. At the same time, the two basic 4-pyridinium (7a) and 3-pyridinium compounds (8a) were potent AChE inhibitors (IC50 = 32 nM and 56 nM, respectively). The potencies of the parent curcumin 4-pyridinium (7f) and curcumin 3-pyridinium compounds (8f) were even higher, with IC50 values of 7.5 and 17 nM, respectively. Derivative 7f had an unsubstituted benzyl moiety and was the most potent among all the target compounds, with an IC50 value of 7.5 ± 0.19 nM that was higher than that of tacrine (IC50 = 30 ± 0.2 nM) and donepezil (IC50 = 14 ± 0.12 nM). Substitution at the benzyl residue is disputable and can be attributed to the size of the substituent. Compound 7 g had the smallest substituent F and an IC50 value of 16 ± 0.18 nM, which was close to that of 7f. The same behaviour was observed for compounds 8f and 8 g. While derivatives with nitro, bromo, and methyl substituents show lower anti-AChE activity with IC50 values in the 0.22 to 1.5 µM range, these compounds were less potent inhibitors of AChE than those with H or F substituents.
Most of the target compounds exhibited high inhibitory activities against eeAChE, with IC50 values in the nano- and low-micromolar ranges. All the target compounds showed distinct AChE selectivity over BChE, considering that donepezil exhibited higher selectivity for AChE over BChE, whereas tacrine was more selective for BChECitation19. Furthermore, it has been noticed that 3- and 4-pyridinium derivatives with similar substituents (R) have similar potency against AChE. The dose-response curves and hillslope values of all tested compounds are provided in the Supporting Information.
The slopes of the Lineweaver-Burk reciprocal plots against the concentrations of the compound (inhibitor) were plotted to obtain the Ki values for the prepared compounds (). The value at which the line intersects the x-axis is -Ki. The plot of slopes of the Lineweaver-Burk plots versus inhibitor 7a concentrations is shown in (-Ki = −39 nM).
In vitro antioxidant activity
The ABTS assay was performed to determine the antioxidant activity of the most potent AChE inhibitors that were synthesised. The radical scavenging activities of the target compounds were compared to the reference antioxidants, trolox and α-tocopherol, at the same concentration and expressed as a percentage of inhibition against ABTS (2,2′-azino-bis(3-ethylbenzothiazoline-6-sulfonic acid). Compounds with a curcumin moiety exhibited good-to-moderate antioxidant activity in a concentration-dependent manner (). The radical scavenging activity of compound 7f at 0.5 mg/mL was 55.3%. In contrast, compounds 8f and 8 g had approximately 59% activity at the same concentration. Trolox and α-tocopherol exhibited 93.9 and 90.2% scavenging activity, respectively, at the same concentration of the target compounds.
Table 2. ABTS antioxidant activity.
As can be concluded from the results of both Ellman’s and ABTS assays, there was a good correlation between the inhibitory activity of the target compounds and their radical scavenging activity, which supported the multi-target behaviour of these compounds. In other words, they can simultaneously target the cholinergic system and oxidative stress in the brain.
Docking study with AChE
The results of the in vitro AChE inhibition assay for the synthesised target compounds motivated us to investigate and confirm their molecular interactions with AChE. Therefore, molecular docking techniques were applied to a few of the target compounds to investigate the intermolecular forces involved in the recognition process, using the interaction between donepezil and AChE for comparison. Two binding sites comprise the AChE structure: the peripheral anionic site (PAS) at the enzyme entrance and the catalytic active site (CAS) at the bottom of the gorgeCitation10. Donepezil’s docking results showed that it interacted with both CAS and PAS ().
Figure 5. Superposition of the X-ray (blue) and docked (light green) structures of donepezil within the active site of AChE.
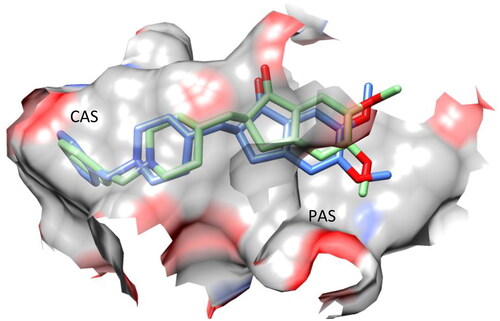
The most potent compound in our library was 7f, which had a binding environment similar to that of donepezil (IC50 = 19 nM)Citation19, with similar binding positions observed in both the CAS and PAS structures ().
Figure 6. Superposition of the top-scored structure of target compound 7f (pink) and the X-ray structure of donepezil (blue) within the active site of AChE.
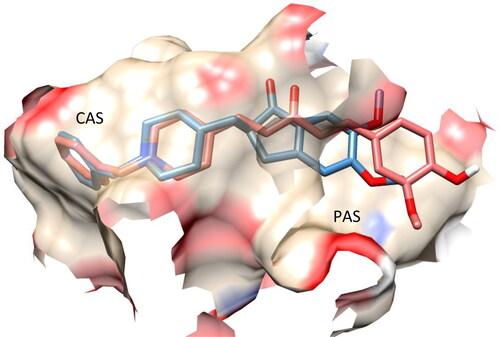
The amino acids TRP84 and PHE330 were close to the bottom of the gorge. They exhibited positive interactions with compound 7f, as observed from the docked structure of compound 7f with AChE (). TRP84 was stacked with the benzene aromatic ring of compound 7f over a 3.7 Å-ring-to-ring distance. The quaternary nitrogen of the pyridinium ring in compound 7f interacted with the phenyl group of PHE330 at a distance of 4.0 Å. At a distance of 4.5 Å, an aromatic interaction was observed between the pyridinium ring of 7f and the phenyl group of PHE330. In the PAS site, the vanillin moiety of compound 7f interacted with the indole ring of TRP279 via a stacking interaction with a distance of 4.0. These results illustrated that compound 7f could simultaneously interact with the CAS and PAS of AChE.
The AChE inhibitory activity assay for target compound 7e demonstrated a noticeable decrease in inhibitory affinity, with an IC50 of 0.8 μM compared to 7.5 nM for 7f. Therefore, a molecular docking study of the 4-nitrobenzyl analog compound 7e was performed to understand this behaviour. The superposition of the top-scoring structures for compounds 7e and 7f showed that compound 7e could interact with the surrounding amino acids similar to that of compound 7f (). The superimposition of the two docked structures showed that the orientation of compound 7e shifted, affecting the strength of its interaction with the amino acids of AChE. Additionally, the close proximity of the nitro group in compound 7e to the carboxylate group of GLU199 induced an orientational change in the benzyl moiety in compound 7f. This may explain the reduced activity of compound 7e compared to that of compound 7f.
Figure 8. (a) Superposition of the top-scored structures for compounds 7e (green) and 7f (pink) within the active site of AChE. (b) Interaction mode of compound 7e in the active site of AChE.
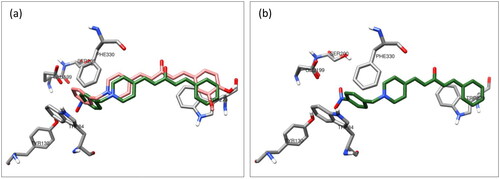
The effect of the size of the four-substituent group on the binding affinity towards the CAS in AChE may explain the reduction in AChE inhibitory activity of all bulky nitro, bromo, and methyl groups versus para H and/or F analogs as a result of this docking study.
Experimental
Chemistry
All chemicals were purchased from commercial sources: Sigma-Aldrich Co. and Acros. Melting points of the compounds were uncorrected and measured in capillary tubes, using Krüss Optronic melting point metre M3000. 1H and 13C-NMR spectral data were obtained from a Bruker Avance III spectrometer (500 and 125 MHz, respectively) with TMS as an internal standard. Spectra were acquired in deuterated dimethyl sulfoxide-d6 (DMSO-d6) or Methanol-d4 (CD3OD). 1H-NMR and 13C-NMR spectra of all compounds are given in SI (Figure S1-S48). High-resolution mass spectra (HRMS) were measured (in positive ion mode) using electrospray ion trap (ESI) technique by collision-induced dissociation on a Bruker APEX-IV (7 Tesla) instrument. TLC was performed on silica gel on aluminium foils with fluorescent indicator 254 nm (Sigma-Aldrich). For column chromatography, silica gel 60, 230–400 mesh (Macherey-Nagel) was used.
General procedure for the synthesis of compounds 12a and 12b
To a mixture of (E)-4-phenylbut-3-en-2-one (9) (20 mmol) and pyridinecarboxaldehyde 11a or 11b (20 mmol) in toluene (10 ml), 7–10 drops of piperidine were added. Then, the reaction mixture was refluxed for 24 h (overnight). After completion of the reaction (monitored by TLC using a 1:1 n-hexane/ethyl acetate eluent system), the mixture was cooled and then concentrated under vacuum. The residue was subjected to column chromatography using n-hexane/ethyl acetate (1:1) as the eluent system to give a yellow solid product (12a or 12b).
(1E,4E)-1-Phenyl-5-(pyridin-4-yl)penta-1,4-dien-3-one (12a)
Yellow solid, Yield: 21%. 1H- NMR (500 MHz, Methanol-d4) δ: 8.56 (d, J = 5.1 Hz, 2H), 7.81 (d, J = 16.0 Hz, 1H; H-aliphatic), 7.68 (m, 5H), 7.48 (d, J = 16.1 Hz, 1H; H-aliphatic), 7.41 (m, 3H), 7.21 (d, J = 16.1 Hz, 1H; H-aliphatic) ppm. 13C-NMR (125 MHz, Methanol-d4) δ: 189.3 (C = O), 149.5 (CH), 144.6 (CH), 143.4, 139.5 (CH), 134.5, 130.6 (CH), 129.5 (CH), 128.7 (CH), 128.3 (CH), 124.9 (CH), 122.5 (CH) ppm. HRMS (ESI) m/z: calcd for C16H14NO, [M + H]+: 236.10307, found: 236.10606.
(1E,4E)-1-phenyl-5-(pyridin-3-yl)penta-1,4-dien-3-one (12b)
Yellow solid, Yield: 15%. 1H NMR (500 MHz, DMSO-d6) δ: 8.91 (d, J = 2.2 Hz, 1H), 8.57 (m, 1H), 8.19 (m, 1H), 7.77 (m, 4H), 7.57 (m, 1H), 7.48 (m, 2H), 7.43 (m, 2H) 7.29 (d, J = 16.2 Hz, 1H, H-aliphatic) ppm. 13C NMR (125 MHz, DMSO-d6) δ: 188.8 (C = O), 151.4 (CH), 150.5 (CH), 143.7 (CH), 139.8 (CH), 135.2 (CH), 135.1, 131.1 (CH), 131.0, 129.4 (CH), 129.0 (CH), 127.6 (CH), 126.1 (CH), 124.4 (CH) ppm. HRMS (ESI) m/z: calcd for C16H14NO, [M + H]+: 236.10699, found: 236.10780.
General procedure for the synthesis of compounds 13a and 13b
(E)-4–(4-hydroxy-3-methoxyphenyl)but-3-en-2-one (10) (5.2 mmol) and pyridinecarboxaldehyde 11a or 11b (20 mmol) were dissolved in 1-propanol (15 ml). The resulting solution was stirred at room temperature for 10 min; then the reaction mixture was cooled in an ice-bath and 1 M NaOH (3 mmol) was slowly added. The stirring continued at room temperature for about 2 h. After completion of the reaction as illustrated by TLC (using 1:1 ethyl acetate/n-hexane eluent system), an appropriate amount of ice-cooled water (25 ml) was added, and the pH was adjusted to about 5–6 using 5% HCl solutionCitation10. The yellow precipitate was filtered and washed with diethyl ether furnishing 13a or 13b.
(1E,4E)-1–(4-Hydroxy-3-methoxyphenyl)-5-(pyridin-4-yl)penta-1,4-dien-3-one (13a)
Yellow solid, Yield: 92%. 1H-NMR (500 MHz, DMSO-d6) δ: 9.75 (s, 1H, OH), 8.65 (m, 2H), 7.79 (d, J = 16.0 Hz, 1H), 7.72 (m, 2H), 7.65 (d, J = 16.0 Hz, 1H), 7.59 (d, J = 16.0 Hz, 1H), 7.39 (d, J = 2.0 Hz, 1H), 7.24 (m, 1H), 7.14 (d, J = 15.9 Hz, 1H), 6.86 (m, 1H), 3.85 (s, 3H, OCH3) ppm. 13C-NMR (125 MHz, DMSO-d6) δ: 188.61 (C = O), 150.8 (CH), 150.3, 148.4, 145.1 (CH), 142.5, 139.4 (CH), 129.9 (CH), 126.5, 124.2 (CH), 123.3 (CH), 122.6 (CH), 116.2 (CH), 112.0 (CH), 56.1 (OCH3) ppm. HRMS (ESI) m/z: calcd for C17H16NO3, [M + H]+: 282.11247, found: 282.11414.
(1E,4E)-1–(4-Hydroxy-3-methoxyphenyl)-5-(pyridin-3-yl)penta-1,4-dien-3-one (13b)
Yellow solid, Yield: 90%. 1H-NMR (500 MHz, DMSO-d6) δ: 9.68 (s, 1H, OH), 8.90 (d, J = 2.2 Hz, 1H), 8.56 (m, 1H), 8.18 (m, 1H), 7.74 (d, J = 16.0 Hz, 1H, H-aliphatic), 7.68 (d, J = 16.1 Hz, 1H, H-aliphatic), 7.47 (m, 1H), 7.43 (m, 1H), 7.34 (d, J = 2.0 Hz, 1H), 7.18 (m, 1H), 7.08 (d, J = 16.0 Hz, 1H, H-aliphatic), 6.81 (d, J = 8.1 Hz, 1H), 3.81 (s, 3H, OCH3) ppm. 13C-NMR (125 MHz, DMSO-d6) δ: 188.5 (C = O), 151.2 (CH), 150.4 (CH), 150.2 (CH), 148.4 (CH), 144.7 (CH), 138.8 (CH), 135.1, 131.1 (CH), 127.6, 126.6 (CH), 124.4, 124.0 (CH), 123.4 (CH), 116.1 (CH), 112.0 (CH), 56.1 (OCH3) ppm. HRMS (ESI) m/z: calcd for C17H16NO3, [M + H]+: 282.11247, found: 282.11444.
General procedure for the synthesis of pyridinium salt derivatives (7a-j, 8a-j)Citation10
Compound 12a, 12b, 13a or 13b (0.3 mmol) was dissolved in acetonitrile (5–6 ml) upon heating. Then, benzyl bromide or substituted benzyl bromide (0.6 mmol) was added dropwise. The reaction mixture was heated under reflux for 2–4 h. After completion of the reaction as indicated by TLC using 10:1 dichloromethane/methanol as an eluent system, the resulting yellow solid was filtered and washed with diethyl ether to afford the targeted pyridinium salts (7a-j, 8a-j).
1-Benzyl-4-(1E,4E)-3-oxo-5-phenylpenta-1,4-dien-1-yl)pyridin-1-ium bromide (7a)
Yellow solid, Yield: 73%, mp 191–193 0C. 1H-NMR (500 MHz, DMSO-d6) δ: 9.24 (d, J = 6.4 Hz, 2H, H-pyridine), 8.49 (d, J = 6.3 Hz, 2H, H-pyridine), 7.94 (dd, J = 16.2 Hz, 2H, H-aliphatic), 7.79 (m, 3H), 7.52 (m, 2H), 7.43 (m, 6H), 7.27 (d, J = 16.2 Hz, 1H, H-aliphatic), 5.81 (s, 2H, N-CH2) ppm. 13C-NMR (125 MHz, DMSO-d6) δ: 188.7 (C = O), 151.5, 145.5 (CH), 145.5 (CH), 136.3 (CH), 135.2 (CH), 134.8 (CH), 134.8, 131.5 (CH), 129.8 (CH), 129.7 (CH), 29.6 (CH), 129.2 (CH), 129.2 (CH), 126.9 (CH), 126.2 (CH), 63.4 (N-CH2) ppm. HRMS (ESI) m/z: calcd for C23H20NO+, [M]+: 326.15394, found: 326.15558.
1–(4-Fluorobenzyl)-3-(1E,4E)-3-oxo-5-phenylpenta-1,4-dien-1-yl)pyridin-1-ium bromide (7b)
Yellow solid, Yield: 76% and mp 119–122 0C. 1H-NMR (500 MHz, DMSO-d6) δ: 9.25 (m, 2H, H-pyridine), 8.49 (m, 2H, H-pyridine), 7.95 (d, J = 16.2 Hz, 2H, H-aliphatic), 7.80 (m, 4H), 7.64 (m, 2H), 7.45 (m, 3H), 7.25 (m, 2H), 5.81 (s, 2H, N-CH2) ppm. 13C-NMR (125 MHz, DMSO-d6) δ: 188.7 (C = O), 163.0 (d, J = 246.68 Hz, C-F), 151.5, 145.6 (CH), 145.4 (CH), 136.3, 135.2 (CH), 134.8 (CH), 131.9 (d, J = 8.7 Hz), 131.5 (CH), 131.0 (d, J = 3.2 Hz), 129.5 (CH), 129.2 (CH), 127.0 (CH), 126.2 (CH), 116.6 (d, J = 22.68 Hz,), 62.5 (CH2) ppm. HRMS (ESI) m/z: calcd for C23H19FNO+, [M]+: 344.14452, found: 344.14651.
1–(4-Bromobenzyl)-4-(1E,4E)-3-oxo-5-phenylpenta-1,4-dien-1-yl)pyridin-1-ium bromide (7c)
Yellow solid, Yield: 61% and mp 100–102 0C. 1H-NMR (500 MHz, DMSO-d6) δ: 9.21 (d, J = 6.3 Hz, 2H, H-pyridine), 8.48 (d, J = 6.3 Hz, 2H, H-pyridine), 7.93 (dd, J = 16.1 Hz, 2H, aliphatic-H), 7.76 (m, 3H), 7.64 (d, J = 8.4 Hz, 2H), 7.47 (m, 5H), 7.27 (d, J = 16.2 Hz, 1H, aliphatic-H), 5.79 (s, 2H, N-CH2) ppm. 13C-NMR (125 MHz, DMSO-d6) δ: 188.1 (C = O), 162.2, 151.0, 145.0 (CH), 144.9 (CH), 135.7 (CH), 134.6 (CH), 134.2, 133.4, 132.0, 131.0 (CH), 129.0 (CH), 128.6 (CH), 126.4 (CH), 125.6 (CH), 122.7 (CH), 61.9 (N-CH2) ppm. HRMS (ESI) m/z: calcd for C23H19BrNO+, [M]+: 404.06445, 406.06241, found: 404.06282 (79Br), 406.06098 (81Br).
1–(4-Methylbenzyl)-4-(1E,4E)-3-oxo-5-phenylpenta-1,4-dien-1-yl)pyridin-1-ium bromide (7d)
Yellow solid, Yield: 76%, decomposed at 195–197 °C. 1H NMR (500 MHz, DMSO-d6) δ: 9.25 (d, J = 6.3 Hz, 2H, pyridine-H), 8.50 (d, J = 6.3 Hz, 2H, pyridine-H), 7.99 (d, J = 2.9 Hz, 1H), 7.95 (d, J = 3.1 Hz, 1H), 7.80 (m, 3H), 7.44 (m, 5H), 7.27 (d, J = 16.2 Hz, 1H, aliphatic-H), 7.22 (d, J = 7.8 Hz, 2H), 5.78 (s, 2H, N-CH2), 2.26 (s, 3H, CH3) ppm. 13C NMR (125 MHz, DMSO-d6) δ: 188.8 (C = O), 151.4, 145.6 (CH), 145.3 (CH), 139.4, 136.3 (CH), 135.1 (CH), 134.8, 131.8, 131.5 (CH), 130.2 (CH), 129.5 (CH), 129.3 (CH), 129.2 (CH), 126.9 (CH), 126.2 (CH), 63.1 (N-CH2), 21.2 (CH3) ppm. HRMS (ESI) m/z: calcd for C24H22NO+, [M]+: 340.16959, found: 340.16795.
1–(4-Nitrobenzyl)-4-(1E,4E)-3-oxo-5-phenylpenta-1,4-dien-1-yl)pyridin-1-ium bromide (7e)
Yellow solid, Yield: 72% and decomposed at 210 0C. 1H-NMR (500 MHz, DMSO-d6) δ: 9.27 (d, J = 6.3 Hz, 2H, pyridine-H), 8.54 (d, J = 6.3 Hz, 2H, pyridine-H), 8.26 (d, J = 8.4 Hz, 2H), 7.97 (dd, J = 15.5 Hz, 2H, aliphatic-H), 7.83 (d, J = 16.0 Hz, 1H, aliphatic-H), 7.77 (m, 4H), 7.45 (m, 3H), 7.27 (d, J = 16.2 Hz, 1H, aliphatic-H), 5.99 (s, 2H, N-CH2) ppm. 13C-NMR (125 MHz, DMSO-d6) δ: 188.1 (C = O), 151.2, 147.8, 145.3 (CH), 145.0 (CH), 141.1, 135.7 (CH), 134.7 (CH), 134.2, 131.0 (CH), 129.9 (CH), 129.0 (CH), 128.6 (CH), 126.5 (CH), 125.6 (CH), 124.0 (CH), 61.6 (N-CH2) ppm. HRMS (ESI) m/z: calcd for C23H19N2O3+, [M]+: 371.13902, found: 371.14106.
1-Benzyl-4-(1E,4E)-5–(4-hydroxy-3-methoxyphenyl)-3-oxopenta-1,4-dien-1-yl)pyridin-1-ium bromide (7f)
Orange solid, Yield: 78% and decomposed at 182–183 0C. 1H-NMR (500 MHz, DMSO-d6) δ: 9.80 (s, 1H, OH), 9.22 (d, J = 6.3 Hz, 2H, pyridine-H), 8.47 (d, J = 6.3 Hz, 2H, pyridine-H), 7.94 (d, J = 16.0 Hz, 1H, aliphatic-H), 7.86 (d, J = 16.1 Hz, 1H, aliphatic-H), 7.74 (d, J = 15.9 Hz, 1H, aliphatic-H), 7.52 (m, 2H), 7.38 (m, 4H), 7.21 (m, J = 8.2 Hz, 1H), 7.09 (d, J = 16.1 Hz, 1H), 6.82 (d, J = 8.1 Hz, 1H), 5.80 (s, 2H, N-CH2), 3.81 (s, 3H, OCH3) ppm. 13C-NMR (125 MHz, DMSO-d6) δ: 188.2 (C = O), 151.6, 150.7, 148.5, 146.5 (CH), 145.4 (CH), 135.5 (CH), 135.4 (CH), 134.8, 129.8 (CH), 129.7 (CH), 129.2 (CH), 126.9 (CH), 126.3, 124.5 (CH), 123.4 (CH), 116.2 (CH), 112.0 (CH), 63.3 (N-CH2), 56.2 (OCH3) ppm. HRMS (ESI) m/z: calcd for C24H22NO3+, [M]+: 372.15942, found: 372.16075.
1–(4-Fluorobenzyl)-4-(1E,4E)-5–(4-hydroxy-3-methoxyphenyl)-3-oxopenta-1,4-dien-1-yl)pyridin-1-ium bromide (7 g)
Maroon solid, Yield: 77% and decomposed at 222–224 0C. 1H-NMR (500 MHz, DMSO-d6) δ: 9.80 (s, 1H, OH), 9.20 (d, J = 6.3 Hz, 2H, pyridine-H), 8.46 (d, J = 6.3 Hz, 2H, pyridine-H), 7.85 (d, J = 16.1 Hz, 1H, aliphatic-H), 7.73 (d, J = 15.9 Hz, 1H, aliphatic-H), 7.62 (m, 2H), 7.35 (d, J = 2.0 Hz, 1H,), 7.32 (s, 1H), 7.24 (m, 3H), 7.09 (d, J = 16.0 Hz, 1H, aliphatic-H), 6.82 (d, J = 8.1 Hz, 1H), 5.78 (s, 2H, N-CH2), 3.81 (s, 3H, O-CH3) ppm. 13C-NMR (125 MHz, DMSO-d6) δ: 188.2 (C = O) 163.0 (d, J = 247.19 Hz, C-F), 151.7, 150.7, 146.5 (CH), 145.4 (CH), 135.5 (d, J = 13.8 Hz, CH), 131.8 (d, J = 8.7 Hz), 131.0, 126.9 (CH), 126.3, 124.5 (CH), 123.4 (CH), 116.6 (d, J = 21.7 Hz, CH), 116.2 (CH), 112.0 (CH), 62.5 (N-CH2), 56.2 (O-CH3) ppm. HRMS (ESI) m/z: calcd for C24H21FNO3+, [M]+: 390.15000, found: 390.14826.
1–(4-Bromobenzyl)-4-(1E,4E)-5–(4-hydroxy-3-methoxyphenyl)-3-oxopenta-1,4-dien-1-yl)pyridin-1-ium bromide (7h)
Maroon solid, Yield: 89% and decomposed at 234–235 0C. 1H- NMR (500 MHz, DMSO-d6) δ: 9.79 (s, 1H, OH), 9.21 (d, J = 6.4 Hz, 2H, pyridine-H), 8.48 (d, J = 6.4 Hz, 2H, pyridine-H), 7.96 (d, J = 16.0 Hz, 1H, aliphatic-H), 7.87 (d, J = 16.0 Hz, 1H, aliphatic-H), 7.74 (d, J = 15.9 Hz, 1H, aliphatic-H), 7.63 (d, J = 8.2 Hz, 2H), 7.49 (d, J = 8.2 Hz, 2H), 7.36 (d, J = 2.0 Hz, 1H), 7.21 (m, 1H), 7.09 (d, J = 16.1 Hz, 1H), 6.83 (d, J = 8.1 Hz, 1H), 5.78 (s, 2H, N-CH2), 3.81 (s, 3H, O-CH3) ppm. 13C-NMR (125 MHz, DMSO-d6) δ: 188.23 (C = O), 151.7, 150.7, 148.5, 146.6 (CH), 145.5 (CH), 135.5 (CH), 135.5 (CH), 134.0, 132.6 (CH), 131.5 (CH), 126.9 (CH), 126.3, 124.5 (CH), 123.5 (CH), 123.3, 116.2 (CH), 112.0 (CH), 62.5 (N-CH2), 56.2 (O-CH3) ppm. HRMS (ESI) m/z: calcd for C24H21BrNO3+, [M]+: 450.06993, 452.06788, found: 450.07152 (79Br), 452.06980 (81Br).
4-(1E,4E)-5–(4-Hydroxy-3-methoxyphenyl)-3-oxopenta-1,4-dien-1-yl)-1–(4-methylbenzyl)pyridin-1-ium bromide (7i)
Reddish orange solid, Yield: 81% and decomposed at 213–214 0C. 1H-NMR (500 MHz, DMSO-d6) δ: 9.79 (s, 1H, OH), 9.20 (d, J = 6.3 Hz, 2H, pyridine), 8.46 (d, J = 6.4 Hz, 2H, pyridine), 7.94 (d, J = 16.0 Hz, 1H, aliphatic-H), 7.86 (d, J = 16.0 Hz, 1H, aliphatic-H), 7.74 (d, J = 16.0 Hz, 1H, aliphatic-H), 7.42 (d, J = 7.8 Hz, 2H), 7.36 (d, J = 2.0 Hz, 1H), 7.21 (m, 3H), 7.09 (d, J = 16.0 Hz, 1H), 6.83 (d, J = 8.2 Hz, 1H), 5.75 (s, 2H, N-CH2), 3.81 (s, 3H, O-CH3), 2.26 (s, 3H, CH3) ppm. 13C-NMR (125 MHz, DMSO-d6) δ: 187.6 (C = O), 151.0, 150.1, 147.9, 145.9 (CH), 144.7 (CH), 138.8, 135.0 (CH), 134.8 (CH), 131.2, 129.6 (CH), 128.7 (CH), 126.2 (CH), 125.7, 123.9 (CH), 122.8 (CH), 115.6 (CH), 111.5 (CH), 62.6 (N-CH2), 55.6 (O-CH3), 20.6 (CH3) ppm. HRMS (ESI) m/z: calcd for C25H24NO3+, [M]+: 386.17507, found: 386.17672.
4-(1E,4E)-5–(4-Hydroxy-3-methoxyphenyl)-3-oxopenta-1,4-dien-1-yl)-1–(4-nitrobenzyl)pyridin-1-ium bromide (7j)
Reddish orang solid, Yield: 79% and decomposed at 170–172 0C. 1H-NMR (500 MHz, DMSO-d6) δ: 9.81 (s, 1H, OH), 9.22 (d, J = 6.3 Hz, 2H, pyridine-H), 8.50 (d, J = 6.3 Hz, 2H, pyridine-H), 8.26 (d, J = 8.3 Hz, 2H), 7.96 (d, J = 16.0 Hz, 1H, aliphatic-H), 7.86 (d, J = 16.0 Hz, 1H, aliphatic-H), 7.75 (m, 3H), 7.35 (d, J = 2.1 Hz, 1H), 7.21 (m, 1H), 7.09 (d, J = 16.1 Hz, 1H, aliphatic-H), 6.83 (d, J = 8.1 Hz, 1H), 5.95 (s, 2H, N-CH2), 3.81 (s, 3H, CH3) ppm. 13C-NMR (125 MHz, DMSO-d6) δ: 188.2 (C = O), 152.0, 150.7, 148.5, 148.3, 146.6 (CH), 145.8 (CH), 141.7, 135.6 (CH), 135.5 (CH), 130.4 (CH), 127.0 (CH), 126.3, 124.6 (CH), 124.6 (CH), 123.5 (CH), 116.2 (CH), 112.0 (CH), 62.2 (CH2), 56.2 (O-CH3) ppm. HRMS (ESI) m/z: calcd for C24H21N2O5+, [M]+: 417.14450, found: 417.14296.
1-Benzyl-3-(1E,4E)-3-oxo-5-phenylpenta-1,4-dien-1-yl)pyridin-1-ium bromide (8a)
Yellow solid, Yield: 70% and mp 223–225 0C. 1H-NMR (500 MHz, DMSO-d6) δ: 9.51 (s, 1H, pyridine-H), 8.99 (d, J = 6.0 Hz, 1H, pyridine-H), 8.93 (d, J = 8.2 Hz, 1H, pyridine-H), 8.12 (m, 1H), 7.91 (d, J = 16.1 Hz, 1H, aliphatic-H), 7.79 (d, J = 16.0 Hz, 1H, aliphatic-H), 7.72 (m, 3H), 7.55 (m, 2H), 7.45 (m, 6H), 7.19 (d, J = 16.1 Hz, 1H), 5.87 (s, 2H, N-CH2) ppm. 13C-NMR (125 MHz, DMSO-d6) δ: 188.4, 145.3 (CH), 144.3 (CH), 144.2 (CH), 143.5 (CH), 136.6, 134.5, 134.1 (CH), 133.0, 131.3 (CH), 130.8 (CH), 129.7 (CH), 129.3 (CH), 128.7 (CH), 128.7 (CH), 128.4 (CH), 128.3 (CH), 125.0 (CH), 64.6 (N-CH2) ppm. HRMS (ESI) m/z: calcd for C23H20NO+, [M]+: 326.15394, found: 326.15487.
1–(4-Fluorobenzyl)-3-(1E,4E)-3-oxo-5-phenylpenta-1,4-dien-1-yl)pyridin-1-ium bromide (8b)
Pale yellow solid, Yield: 69% and mp 157–159 °C. 1H-NMR (500 MHz, DMSO-d6) δ: 9.89 (s, 1H, pyridine-H), 9.19 (d, J = 6.1 Hz, 1H, pyridine-H), 9.00 (d, J = 8.2 Hz, 1H, pyridine-H), 8.21 (m, 1H), 8.02 (d, J = 16.3 Hz, 1H, aliphatic-H), 7.92 (d, J = 16.1 Hz, 1H, aliphatic-H), 7.80 (m, 3H), 7.73 (m, 2H), 7.43 (m, 3H), 7.25 (m, 3H), 5.90 (s, 2H, N-CH2) ppm. 13C-NMR (125 MHz, DMSO-d6) δ: 188.7 (C = O), 163.1 (d, J = 246.5 Hz, C-F), 145.2 (CH), 145.02 (CH), 144.9 (CH), 144.3 (CH), 136.0, 135.4 (CH), 134.9, 132.1 (d, J = 8.6 Hz, CH), 131.4 (CH), 131.1 (CH), 130.7 (d, J = 3.2 Hz), 129.5 (CH), 129.2 (CH), 128.9 (CH), 126.2 (CH), 116.5 (d, J = 21.7 Hz, CH), 63.0 (N-CH2) ppm. HRMS (ESI) m/z: calcd for C23H19FNO+, [M]+: 344.14452, found: 344.14623.
1–(4-Bromobenzyl)-3-(1E,4E)-3-oxo-5-phenylpenta-1,4-dien-1-yl)pyridin-1-ium bromide (8c)
Off-white solid, Yield: 78% and mp 203–205 0C. 1H-NMR (500 MHz, DMSO-d6) δ 9.74 (s, 1H, pyridine-H), 9.15 (d, J = 6.1 Hz, 1H, pyridine-H), 8.99 (d, J = 8.2 Hz, 1H, pyridine-H), 8.22 (m, 1H), 7.95 (d, J = 16.1 Hz, 1H, aliphatic-H), 7.80 (m, 4H), 7.64 (d, J = 8.1 Hz, 2H), 7.55 (d, J = 8.1 Hz, 2H), 7.45 (m, 3H), 7.24 (d, J = 16.1 Hz, 1H, aliphatic-H), 5.85 (s, 2H, N-CH2) ppm. 13C-NMR (125 MHz, DMSO-d6) δ: 188.0 (C = O), 144.6 (CH), 144.5 (CH), 144.5 (CH), 143.6 (CH), 135.4, 134.8 (CH), 134.3, 133.1, 132.0 (CH), 131.2 (CH), 130.8 (CH), 130.7 (CH), 128.9 (CH), 128.6 (CH), 128.4 (CH), 125.5, 122.8, 62.6 (CH2) ppm. HRMS (ESI) m/z: calcd for C23H19BrNO+, [M]+: 404.06445, 406.06241, found: 404.06353 (79Br), 406.06219 (81Br).
1–(4-Methylbenzyl)-3-(1E,4E)-3-oxo-5-phenylpenta-1,4-dien-1-yl)pyridin-1-ium bromide (8d)
Off-white solid, Yield: 73% and mp 205–206 0C. 1H-NMR (500 MHz, DMSO-d6) δ: 9.82 (s, 1H, pyridine-H), 9.15 (d, J = 6.1 Hz, 1H, pyridine-H), 8.99 (d, J = 8.2 Hz, 1H, pyridine-H), 8.20 (m, 1H), 7.99 (d, J = 16.2 Hz, 1H, aliphatic-H), 7.88 (d, J = 16.1 Hz, 1H, aliphatic-H), 7.80 (m, 3H), 7.50 (m, 2H), 7.44 (m, 3H), 7.23 (m, 3H), 5.83 (s, 2H, N-CH2), 2.26 (s, 3H, CH3) ppm. 13C-NMR (125 MHz, DMSO-d6) δ: 188.7 (C = O), 145.1, 145.0 (CH), 144.9 (CH), 144.1 (CH), 139.5, 135.9, 135.4 (CH), 134.9, 131.5, 131.4 (CH), 131.2 (CH),130.2 (CH), 129.5 (CH), 129.5 (CH), 129.1 (CH), 128.9 (CH), 126.2 (CH), 63.8 (CH2), 21.2 (CH3) ppm. HRMS (ESI) m/z: calcd for C24H22NO+, [M]+: 340.16959, found: 340.17090.
1–(4-Nitrobenzyl)-3-(1E,4E)-3-oxo-5-phenylpenta-1,4-dien-1-yl)pyridin-1-ium bromide (8e)
Off-white solid, Yield: 76% and mp 145–147 0C. 1H-NMR (500 MHz, DMSO-d6) δ: 9.85 (s, 1H, pyridine-H), 9.21 (d, J = 6.1 Hz, 1H, pyridine-H), 9.03 (d, J = 8.1 Hz, 1H, pyridine-H), 8.26 (m, 3H), 7.99 (d, J = 16.3 Hz, 1H, aliphatic-H), 7.83 (m, 6H), 7.44 (m, 3H), 7.23 (d, J = 16.3 Hz, 1H, aliphatic-H), 6.06 (s, 2H, N-CH2) ppm. 13C-NMR (125 MHz, DMSO-d6) δ: 188.1 (C = O), 147.8, 144.9 (CH), 144.8 (CH), 144.6 (CH), 144.0 (CH), 140.7, 135.5, 134.8 (CH), 134.3, 130.8 (CH), 130.6 (CH), 130.2 (CH), 128.9 (CH), 128.6 (CH), 128.4 (CH), 125.6 (CH), 124.0 (CH), 62.2 (N-CH2) ppm. HRMS (ESI) m/z: calcd for C23H19N2O3+, [M]+: 371.13902, found: 371.14040.
1-Benzyl-3-(1E,4E)-5–(4-hydroxy-3-methoxyphenyl)-3-oxopenta-1,4-dien-1-yl)pyridin-1-ium bromide (8f)
Yellow solid, Yield: 91% and mp 196–198 0C. 1H-NMR (500 MHz, DMSO-d6) δ: 9.87 (s, 1H, pyridine-H), 9.75 (s, 1H, OH), 9.17 (d, J = 5.8 Hz, 1H, pyridine-H), 9.00 (d, J = 6.6 Hz, 1H, pyridine-H), 8.21 (m, 1H), 7.91 (m, 2H), 7.76 (dd, J = 16.1 Hz, 1H, aliphatic-H), 7.60 (m, 2H), 7.41 (m, 4H), 7.24 (m, 1H), 7.07 (dd, J = 16.2 Hz, 1H, aliphatic-H), 6.83 (m, 1H), 5.90 (d, J = 5.2 Hz, 2H, N-CH2), 3.82 (d, J = 5.3 Hz, 3H, O-CH3) ppm. 13C-NMR (125 MHz, DMSO-d6) δ: 188.2 (C = O), 150.5, 148.5, 146.1 (CH), 144.9 (CH), 144.1 (CH), 136.2, 134.5 (CH), 134.5, 131.4 (CH), 129.9 (CH), 129.6 (CH), 129.4(CH), 128.9 (CH), 126.4, 124.4 (CH), 123.4 (CH), 116.2 (CH), 112.1 (CH), 63.9 (CH), 56.2 (CH2) ppm. HRMS (ESI) m/z: calcd for C24H22NO3+, [M]+: 372.15942, found: 372.16107.
1–(4-Fluorobenzyl)-3-(1E,4E)-5–(4-hydroxy-3-methoxyphenyl)-3-oxopenta-1,4-dien-1-yl)pyridin-1-ium bromide (8 g)
Yellow solid, Yield: 90% and mp 214–216 0C. 1H-NMR (500 MHz, DMSO-d6) δ: 9.85 (s, 1H, pyridine-H), 9.75 (s, 1H, OH), 9.16 (d, J = 6.1 Hz, 1H, pyridine-H), 8.99 (d, J = 8.2 Hz, 1H, pyridine-H), 8.20 (m, 1H), 7.90 (dd, J = 16.0 Hz, 2H, aliphatic-H), 7.72 (m, 3H), 7.39 (d, J = 2.0 Hz, 1H), 7.25 (m, 3H), 7.06 (d, J = 16.1 Hz, 1H, aliphatic-H), 6.82 (d, J = 8.1 Hz, 1H), 5.88 (s, 2H, N-CH2), 3.81 (s, 3H, O-CH3) ppm. 13C-NMR (125 MHz, DMSO-d6) δ: 188.2 (C = O), 163.1 (d, J = 246.7 Hz, C-F), 150.5, 148.5, 146.1 (CH), 144.8 (CH), 144.1 (CH), 136.1, 134.5 (CH), 132.1 (d, J = 8.6 Hz, CH), 131.4 (CH), 130.7 (d, J = 3.1 Hz), 128.9 (CH), 126.4, 124.4 (CH), 123.4 (CH), 116.5 (d, J = 21.9 Hz, CH), 116.2 (CH), 112.1 (CH), 63.0 (CH2), 56.2 (O-CH3) ppm. HRMS (ESI) m/z: calcd for C24H21FNO3+, [M]+: 390.15000, found: 390.15171.
1–(4-Bromobenzyl)-3-(1E,4E)-5–(4-hydroxy-3-methoxyphenyl)-3-oxopenta-1,4-dien-1-yl)pyridin-1-ium bromide (8h)
Yellow solid, Yield: 91% and mp 226–228 0C. 1H-NMR (500 MHz, DMSO-d6) δ: 9.79 (s, 1H, pyridine-H), 9.75 (s, 1H, OH), 9.14 (d, J = 6.1 Hz, 1H, pyridine-H), 8.99 (d, J = 8.1 Hz, 1H, pyridine-H), 8.21 (m, 1H), 7.88 (dd, J = 16.1 Hz, 2H, aliphatic-H), 7.74 (d, J = 16.1 Hz, 1H, aliphatic-H), 7.63 (d, J = 8.2 Hz, 2H), 7.56 (d, J = 8.3 Hz, 2H), 7.38 (d, J = 1.9 Hz, 1H), 7.22 (m, 1H), 7.06 (d, J = 16.2 Hz, 1H, aliphatic-H), 6.82 (d, J = 8.1 Hz, 1H), 5.86 (s, 2H, N-CH2), 3.81 (s, 3H, O-CH3) ppm. 13C-NMR (125 MHz, DMSO-d6) δ: 188.2 (C = O), 150.5, 148.5, 146.1 (CH), 145.0 (CH), 144.2 (CH), 136.2, 134.5 (CH), 133.7, 132.5 (CH), 131.7 (CH), 131.4 (CH), 128.9 (CH), 126.4, 124.4 (CH), 123.4, 123.4 (CH), 116.2, 112.0, 63.1 (N-CH2), 56.2 (O-CH3) ppm. HRMS (ESI) m/z: calcd for C24H21BrNO3+, [M]+: 450.06993, 452.06788, found: 450.07190 (79Br), 452.07010 (81Br).
3-(1E,4E)-5–(4-Hydroxy-3-methoxyphenyl)-3-oxopenta-1,4-dien-1-yl)-1–(4-methylbenzyl)pyridin-1-ium bromide (8i)
Yellow solid, Yield: 90% and mp 200–203 0C. 1H- NMR (500 MHz, DMSO-d6) δ: 9.79 (s, 1H, pyridine-H), 9.75 (s, 1H, OH), 9.13 (d, J = 6.1 Hz, 1H, pyridine-H), 8.98 (d, J = 8.1 Hz, 1H, pyridine-H), 8.19 (m, 1H), 7.87 (dd, J = 16.0 Hz, 2H, aliphatic-H), 7.75 (d, J = 16.0 Hz, 1H, aliphatic-H), 7.49 (d, J = 7.8 Hz, 2H), 7.38 (s, 1H), 7.22 (d, J = 7.7 Hz, 3H), 7.06 (d, J = 16.0 Hz, 1H, aliphatic-H), 6.82 (d, J = 8.1 Hz, 1H), 5.82 (s, 2H, N-CH2), 3.81 (s, 3H, O-CH3), 2.26 (s, 3H, CH3) ppm. 13C-NMR (125 MHz, DMSO-d6) δ: 188.2 (C = O), 150.5, 148.5, 146.0 (CH), 144.8 (CH), 144.0 (CH), 139.5, 136.1, 134.6 (CH), 131.5, 131.4 (CH), 130.2 (CH), 129.4 (CH), 128.9 (CH), 126.4, 124.4 (CH), 123.4 (CH), 116.2 (CH), 112.0 (CH), 63.8 (N-CH2), 56.2 (O-CH3), 21.2 (CH3) ppm. HRMS (ESI) m/z: calcd for C25H24NO3+, [M]+: 386.17507, found: 386.17650.
3-(1E,4E)-5–(4-Hydroxy-3-methoxyphenyl)-3-oxopenta-1,4-dien-1-yl)-1–(4-nitrobenzyl)pyridin-1-ium bromide (8j)
Yellow solid, Yield: 93% and mp 190–192 0C. 1H- NMR (500 MHz, DMSO-d6) δ: 9.84 (s, 1H, pyridine), 9.75 (s, 1H, OH), 9.19 (d, J = 6.1 Hz, 1H, pyridine), 9.03 (d, J = 8.2 Hz, 1H, pyridine), 8.25 (m, 3H), 7.87 (m, 4H), 7.75 (d, J = 16.0 Hz, 1H, aliphatic-H), 7.38 (s, 1H), 7.23 (d, J = 8.2 Hz, 1H), 7.06 (d, J = 16.1 Hz, 1H, aliphatic-H), 6.82 (d, J = 8.1 Hz, 1H), 6.06 (s, 2H, N-CH2), 3.81 (s, 3H, O-CH3) ppm. 13C-NMR (125 MHz, DMSO-d6) δ: 188.2 (C = O), 150.5, 148.5, 148.4, 146.1 (CH), 145.3 (CH), 144.4 (CH), 141.3, 136.3, 134.5 (CH), 131.4 (CH), 130.7 (CH), 129.0 (CH), 126.4, 124.5 (CH), 124.4 (CH), 123.4 (CH), 116.2 (CH), 112.0 (CH), 62.8 (CH2), 56.2 (O-CH3) ppm. HRMS (ESI) m/z: calcd for C24H21N2O5+, [M]+: 417.14450, found: 417.14644.
Biology
Acetyl- and butyrylcholinesterase inhibition assays (IC50)
The AChE and BChE inhibitory activity assays were performed using a BioTek Epoch 2 microplate spectrophotometer in clear, flat-bottom 96-well plates. Compounds 7a-j and 8a-j were dissolved in 1:1–1:1.5 water/methanol, depending on their solubility. To each well, 295 µL phosphate buffer pH 8.0, 10 µL enzyme solution (AChE/BChE), and 10 µL target compound solutions were added together and allowed to stand for 4 min at room temperature. Then, 10 µL of 0.01 M solution freshly prepared Ellman’s reagent 5,5′-dithiobis(2-nitrobenzoic acid) (DTNB) was added to each well. The reaction in the wells was started by the addition of 2 µL of 0.075 M solution freshly prepared substrate (ATCh (acetylthiocholine iodide)/BTCh (S-butyrylthiocholine iodide)) solution. The solution was mixed immediately and incubated for 2:30 min for AChE or for 5 min for BChE inside the microplate reader at 26 °C. The absorbance was measured at 412 nm, and the assay was conducted in triplicate; each concentration was in duplicate on each plateCitation20. The IC50 values were calculated graphically by nonlinear regression analysis of inhibition curves that were obtained by plotting the logarithm of the inhibitor concentrations versus the percentage of inhibition (log(inhibitor) vs. normalised response) using GraphPad Prism 8 software (See Supplementary Information for details).
Enzyme inhibition constant (Ki) determination
The rates of hydrolysis of the ATCh mean velocity (V) for the most potent compounds were measured spectrophotometrically using the kinetic assay protocol available in Gen5 software. The changes in absorbance in each well at 405 nm and 26 °C were measured using the microplate reader at 48-s intervals over 10 min. The assay was performed in triplicate using different inhibitor concentrations. Compounds 7a-j and 8a-j concentrations were prepared according to the IC50 value of each inhibitor using [IC50/2], [IC50], and [2 × IC50] as the final concentrations. The assay was carried out in clear, flat-bottom 96-well plates, to which 5 µL distilled water was added to all wells of columns 2–3, rows B-F. Then, 5 µL of the lowest concentration of inhibitor solution was added to the wells of columns 4–5, 5 µL of the median concentration of inhibitor solution was added to the wells of columns 6–7, and 5 µL of the highest concentration of inhibitor solution was added to the wells of columns 8–9. Next, 275 μL enzyme solution was added to the wells, and the plates were incubated for 4 min. Then, 10 μL 2.5 mM DTNB was added to the wells, followed by 10 μL of 32, 16, 8, 4, or 2 mM ATCh solutions to initiate the reactions. The plates were placed in a microplate reader for direct kinetic measurements.
The inhibition constant (Ki) for each inhibitor was calculated by averaging the ATCh hydrolysis rates from three independent experiments. Using GraphPad Prism 8 software, nonlinear regression was used to calculate the Km and Vmax values of the Michaelis-Menten kinetics by plotting [substrate] versus velocity. For the Lineweaver-Burk plots, linear regression was used to plot [1/ATCh] versus [substrate]. Finally, the Ki values were obtained by plotting the slopes against [inhibitor] Citation20.
Antioxidant activity assay (ABTS)
The antioxidant activity was determined using a radical cation (ABTS.+) decolorisation assay, as described by Xiao et al.Citation21 with a few modifications. The assay used a BioTek Epoch 2 microplate spectrophotometer (Agilent, USA) with clear, flat-bottom 96-well plates. The 7 mmol/L ABTS.+ stock solution was prepared by dissolving 96.02 mg ABTS in acetic acid buffer (pH 4.5) and to a final volume of 25 ml, which was kept in the dark at 0–4 °C. A 2.45 mmol/L potassium persulfate (K2S2O8) stock solution was prepared by dissolving 66.24 mg K2S2O8 in acetic acid buffer solution with pH 4.5, and then diluted to 100 ml and kept in the dark at 0–4 °C. The ABTS working solution was prepared by mixing similar quantities of 7 mmol/L ABTS and 2.45 mmol/L K2S2O8. This solution was stored in a dark cabinet for 16 h before use, when it was diluted with distilled water to obtain an absorbance of 0.70 at 734 nm. In a clear, flat-bottom 96-well plate, 200 μL of ABTS working solution and 10.0 μL sample solution at different concentrations (0.0312–0.5 mg/mL) were mixed well and incubated in the dark for 7 min before the absorbance was measured at 734 nm using a microplate reader. A blank was run for each assay, and all measurements were performed after 7 min. The ABTS scavenging capacity of inhibitors was compared with that of trolox and α-tocopherol. The percentage of inhibition was calculated as follows:
where AC is the absorbance of the blank, and AS is the absorbance in the presence of the inhibitor.
Molecular docking study
The crystallographic structure of AChE complexed with donepezil (PDB entry: 1EVE) was obtained from the RCSB Protein Data Bank. Chain A of the crystal structure was used with a grid box of 25 × 25 × 25 Å, appropriate for covering the interaction region between the ligand and enzyme. The ligand flexible docking study was performed using the docking program AutoDock Vina (1.5.6) Citation22. The exhaustiveness parameter was set to 100, whereas the default parameters were used in all other cases. The docked structures were visualised using the UCSF Chimaera program (1.14)Citation23.
Conclusion
To ensure a good quality of life and health for people, we aimed to develop anti-AD agents. Herein, we report synthesising a series of potent AChE inhibitors bearing an N-benzylpyridinium moiety. In vitro assays indicated the potency of the prepared compounds, especially those containing a vanillin moiety, in additon to their antioxidant activity. Eight of the prepared compounds exhibited AChE inhibitory activity in the nanomolar range. Docking studies explained the potency and binding of these compounds to the active site of AChE. Overall, the results of this study confirmed the promising potential of the synthesised compounds as AD inhibitors. However, further in vivo studies are required to confirm this hypothesis.
Consent for publication
The authors accept the final version of the paper submitted to the journal.
Supplemental Material
Download PDF (4.8 MB)Disclosure statement
No potential conflict of interest was reported by the author(s).
Additional information
Funding
References
- 2023 Alzheimer’s disease facts and figures. Alzheimers Dement. 2023;19(4):1598–1695.
- Rahman A, Hossen MA, Chowdhury MFI, Bari S, Tamanna N, Sultana SS, Haque SN, Al Masud A, Saif-Ur-Rahman KM. Aducanumab for the treatment of Alzheimer’s disease: a systematic review. Psychogeriatrics. 2023;23(3):512–522.
- van Dyck CH, Swanson CJ, Aisen P, Bateman RJ, Chen C, Gee M, Kanekiyo M, Li D, Reyderman L, Cohen S, et al. Lecanemab in early Alzheimer’s disease. N Engl J Med. 2023;388(1):9–21.
- Chen ZR, Huang JB, Yang SL, Hong FF. Role of cholinergic signaling in Alzheimer’s disease. Molecules. 2022;27(6):1816. doi:10.3390/molecules27061816.
- Inestrosa NC, Alvarez A, Pérez CA, Moreno RD, Vicente M, Linker C, Casanueva OI, Soto C, Garrido J. Acetylcholinesterase accelerates assembly of amyloid-beta-peptides into alzheimer’s fibrils: Possible role of the peripheral site of the enzyme. Neuron. 1996;16(4):881–891.
- Carvajal FJ, Inestrosa NC. Interactions of ache with aβ aggregates in Alzheimer’s brain: Therapeutic relevance of idn 5706. Front Mol Neurosci. 2011;4(:19.
- Kareem RT, Abedinifar F, Mahmood EA, Ebadi AG, Rajabi F, Vessally E. The recent development of donepezil structure-based hybrids as potential multifunctional anti-Alzheimer’s agents: highlights from 2010 to 2020. RSC Adv. 2021;11(49):30781–30797.
- Eissa KI, Kamel MM, Mohamed LW, Kassab AE. Development of new Alzheimer’s disease drug candidates using donepezil as a key model. Arch Pharm. 2023;356(1):2200398.
- Mostofi M, Mohammadi Ziarani G, Mahdavi M, Moradi A, Nadri H, Emami S, Alinezhad H, Foroumadi A, Shafiee A. Synthesis and structure-activity relationship study of benzofuran-based chalconoids bearing benzylpyridinium moiety as potent acetylcholinesterase inhibitors. Eur J Med Chem. 2015;103(:361–369.
- Wang C, Wu Z, Cai H, Xu S, Liu J, Jiang J, Yao H, Wu X, Xu J. Design, synthesis, biological evaluation and docking study of 4-isochromanone hybrids bearing n-benzyl pyridinium moiety as dual binding site acetylcholinesterase inhibitors. Bioorg Med Chem Lett. 2015;25(22):5212–5216.
- Jabir NR, Khan FR, Tabrez S. Cholinesterase targeting by polyphenols: a therapeutic approach for the treatment of Alzheimer’s disease. CNS Neurosci Ther. 2018;24(9):753–762.
- Yang F, Lim GP, Begum AN, Ubeda OJ, Simmons MR, Ambegaokar SS, Chen PP, Kayed R, Glabe CG, Frautschy SA, et al. Curcumin inhibits formation of amyloid beta oligomers and fibrils, binds plaques, and reduces amyloid in vivo. J Biol Chem. 2005;280(7):5892–5901.
- Ramalakshmi N, R SR, C NN. Multitarget directed ligand approaches for Alzheimer’s disease: a comprehensive review. Mini Rev Med Chem. 2021;21(16):2361–2388.
- Maramai S, Benchekroun M, Gabr MT, Yahiaoui S. Multitarget therapeutic strategies for Alzheimer’s disease: review on emerging target combinations. Biomed Res Int. 2020;2020(:5120230–27.
- Yücel YY, Tacal O, Ozer I. Comparative effects of cationic triarylmethane, phenoxazine and phenothiazine dyes on horse serum butyrylcholinesterase. Arch Biochem Biophys. 2008;478(2):201–205.
- Ganeshpurkar A, Singh R, Shivhare S, Kumar D, Gutti G, Singh R, Kumar A, Singh , SK. Divya Improved machine learning scoring functions for identification of electrophorus electricus’s acetylcholinesterase inhibitors. Mol Divers. 2022;26(3):1455–1479.
- Sugimoto H, Ogura H, Arai Y, Limura Y, Yamanishi Y. Research and development of donepezil hydrochloride, a new type of acetylcholinesterase inhibitor. Jpn J Pharmacol. 2002;89(1):7–20.
- Ahmed M, Rocha JBT, Corrêa M, Mazzanti CM, Zanin RF, Morsch ALB, Morsch VM, Schetinger MRC. Inhibition of two different cholinesterases by tacrine. Chem Biol Interact. 2006;162(2):165–171.
- Liston DR, Nielsen JA, Villalobos A, Chapin D, Jones SB, Hubbard ST, Shalaby IA, Ramirez A, Nason D, White WF. Pharmacology of selective acetylcholinesterase inhibitors: implications for use in alzheimer’s disease. Eur J Pharmacol. 2004;486(1):9–17.
- Darras FH, Pang Y-P. On the use of the experimentally determined enzyme inhibition constant as a measure of absolute binding affinity. Biochem Biophys Res Commun. 2017;489(4):451–454.
- Xiao F, Xu T, Lu B, Liu R. Guidelines for antioxidant assays for food components. Food Frontiers. 2020;1(1):60–69.
- Trott O, Olson AJ. Autodock vina: Improving the speed and accuracy of docking with a new scoring function, efficient optimization, and multithreading. J Comput Chem. 2010;31(2):455–461.
- Pettersen EF, Goddard TD, Huang CC, Couch GS, Greenblatt DM, Meng EC, Ferrin TE. Ucsf chimera—a visualization system for exploratory research and analysis. J Comput Chem. 2004;25(13):1605–1612.