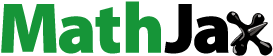
Abstract
Transarterial chemoembolisation (TACE) is used for unresectable hepatocellular carcinoma (HCC) treatment, but TACE-induced hypoxia leads to poor prognosis. The anti-cancer effects of soybean isoflavones daidzein derivatives 7,3′,4′-trihydroxyisoflavone (734THIF) and 7,8,4′-trihydroxyisoflavone (784THIF) were evaluated under hypoxic microenvironments. Molecular docking of these isomers with cyclooxygenase-2 (COX-2) and vascular endothelial growth factor receptor 2 (VEGFR2) was assessed. About 40 μM of 734THIF and 784THIF have the best effect on inhibiting the proliferation of HepG2 cells under hypoxic conditions. At a concentration of 40 μM, 784THIF significantly inhibits COX-2 expression in pre-hypoxia conditions compared to 734THIF, with an inhibition rate of 67.73%. Additionally, 40 μM 784THIF downregulates the expression of hypoxic, inflammatory, and metastatic-related proteins, regulates oxidative stress, and inhibits the expression of anti-apoptotic proteins. The uptake by HepG2 confirmed higher 784THIF level and slower degradation characteristics under post- or pre-hypoxic conditions. In conclusion, our results showed that 784THIF had better anti-cancer effects and cellular uptake than 734THIF.
Introduction
Liver cancer is the fourth leading cause of cancer-related deaths worldwide. Hepatocellular carcinoma (HCC), the most common form of primary liver cancer, is associated with chronic hepatitis virus infection, chronic alcoholism, and non-alcoholic fatty liver disease, accounting for approximately 80%−90% of the HCC cases.Citation1 The Barcelona Clinic Liver Cancer (BCLC) staging system divides HCC into five stages (0, A, B, C, and D) and provides guidelines for prognosis prediction and treatment.Citation2 Transarterial chemoembolisation (TACE), a palliative approach, is often used to treat patients with intermediate stage disease (BCLC B) in order to improve survival.Citation3 During TACE, high doses of cytotoxic drugs are administered selectively to the tumours and arterial embolisation is performed, thereby inducing ischaemic necrosis of the tumour tissue, leading to tumour hypoxia. However, TACE-induced hypoxia in tumour cells may promote metabolic alterations and adaptive gene expression, leading to a poor prognosis.Citation4
Hypoxic microenvironment conditions are linked to cancer progression. Hypoxia-inducible factor-1α (HIF-1α), a key transcription factor in the adaptive response to hypoxia, induces vascular endothelial growth factor (VEGF) expression and promotes neovascularisation in HCC under hypoxia, leading to tumour recurrence or metastasis.Citation5 In hypoxic malignant tumours, the activation and crosstalk between the signalling pathways, HIF-1α and NF-κB, induce the expression of pro-inflammatory factors cyclooxygenase-2 (COX-2), pro-survival gene B-cell lymphoma-2 (BCL-2), matrix metallopeptidase-9 (MMP-9), and VEGF, which promote cancer cell metastasis.Citation6 COX-2 is an established cancer marker, and HIF-1α can induce the expression of COX-2 in HCC cells, further promoting epithelial–mesenchymal transition (EMT), thereby enhancing the invasive and metastatic abilities of HCC, leading to a poor prognosis in TACE.Citation7 Nuclear factor erythroid 2-related factor 2 (NRF-2) is an important transcription factor that maintains intracellular oxidative homeostasis. Tumour hypoxia activates and enhances the interaction between the HIF-1 and NRF-2 signalling pathways, promoting cancer cell metastasis and chemoresistance.Citation8 NRF-2 acts as both a tumour suppressor and tumour promoter in different stages of cancer progression.Citation9 Therefore, proper regulation of NRF2 expression and inhibition of HIF-1α and COX-2 expression may improve hypoxia-induced tumour progression.
TACE combined with multikinase inhibitors such as sorafenib inhibited angiogenesis and improved the prognosis in TACE in clinical studies;Citation10 however, it has adverse effects, including hand-foot syndrome, high blood pressure, and diarrhoea.Citation11 Active pharmaceutical compounds derived from non-toxic natural sources, such as flavonoids and isoflavones, have fewer adverse effects.Citation12 Daidzein is one of the most common isoflavones, and 7,3′,4′-trihydroxyisoflavone (734THIF) and 7,8,4′-trihydroxyisoflavone (784THIF) are the oxidative metabolites of daidzein ().Citation13 734THIF and 784THIF are structural analogs, differing only by the position of one hydroxyl group on either the B or A ring of the flavonoid skeleton. Hence, the biological activities of these two positional isomers were compared. 734-THIF reduces intracellular ROS production, inhibits COX-2 expression, and exhibits anti-cancer activity.Citation14 784-THIF has antioxidant and anti-inflammatory activities.Citation15 However, research on the anti-HCC effects of 734THIF and 784THIF is limited, and the anti-cancer mechanism under hypoxic conditions remains unexplored.
In this study, we simulated molecular docking of 734THIF and 784THIF with hypoxia-induced proteins, COX-2 and VEGF receptor 2 (VEGFR2), and compared them with known inhibitors, in silico. The anti-cancer activities of the two analogues, 734THIF and 784THIF, were evaluated and compared using different hypoxic conditions.
Materials and methods
Molecular docking
Three-dimensional (3D) crystal structures of COX-2 and VEGFR2 were downloaded from the RCSB Protein Data Bank (PDB; https://www.rcsb.org/). The crystals of COX-2 (PDB code 3LN1) and VEGFR2 (PDB code 4ASD) contained active sites for the inhibitors celecoxib and sorafenib, respectively. The 3D conformations of Daidzein, 734THIF and 784THIF were downloaded from PubMed (https://pubchem.ncbi.nlm.nih.gov/). Discovery Studio (DS; BIOVIA, Dassault Systèmes, Vélizy-Villacoublay, Yvelines, France) was used to calculate the docking scores and binding energy of ligands and target proteins. The CDOCKER algorithmCitation16 in DS software was used to calculate the molecular docking scores.
Cell culture and hypoxic condition mode
The human HCC cell line HepG2 (Bioresource Collection and Research Center) was purchased from the Food Industry Research and Development Institute (Hsinchu, Taiwan). HepG2 cells were identified through STR profiling; the cell line was not contaminated. HepG2 cells were maintained in Dulbecco’s modified Eagle’s medium (DMEM; Himedia Laboratories, Mumbai, India) containing 10% foetal bovine serum (FBS; Hazelton Product, Denver, PA, USA) and 1% penicillin–streptomycin–amphotericin B solution (PSA; Biological Industries, Cromwell, CT, USA). The cells were cultured at 37 °C and 5% CO2.
The HepG2 hypoxia model is composed of three different experimental conditions: pre-hypoxia, post-hypoxia, and normoxia.Citation17 A hypoxic environment was established using an O2/CO2 cell culture incubator (MCO-5M-PT; Panasonic, OSAKA, Japan). Pre-hypoxia: depriving cells of oxygen up to 2% and then providing a normal concentration of oxygen; post-hypoxia: providing cells a normal concentration of oxygen for a period followed by hypoxia (2% oxygen); normoxia: same oxygen concentration as that used for maintaining cells. The duration of hypoxia and normal oxygen supply varied with the experiments.
Cell viability assay
HepG2 (1.5 × 104) cells were seeded in 96 wells and cultured under normal conditions for 24 h, and the DMEM was removed. 734THIF and 784THIF were provided by Prof. Chih-Hua Tseng (School of Pharmacy, College of Pharmacy, Kaohsiung Medical University, Kaohsiung, Taiwan); their purity was over 95%, as determined using HPLC analysis. They were solubilised in DMSO and diluted to 5, 10, 20, 40, and 80 μM in DMEM without FBS. The solution was added to 96 wells and incubated under the three conditions. The hypoxic period for pre-hypoxia and post-hypoxia was 6 h, and that for normoxia was 18 h. The control group (normoxia) was supplied a normal concentration of oxygen for 24 h. After treatment, the drug-containing DMEM was removed, and 100 μL of MTT solution (0.5 mg/mL) was added. After 4 h of incubation under normal conditions, the MTT solution was removed, and DMSO was added to dissolve the crystals. A microplate spectrophotometer was used for measuring absorbance at 550 nm.
Western blot analysis
To analyse protein expression, 1.5 × 106 HepG2 cells were seeded in six-well plates and incubated for 24 h under normal culture conditions. 734THIF and 784THIF were dissolved in DMSO and diluted to 40 μM in DMEM without FBS. The cell culture medium was removed and 100 μL of the sample and control solution were added. The cells were cultured under the three conditions. The hypoxic and normoxic periods for pre-hypoxia and post-hypoxia were 6 h. A normal oxygen concentration was supplied to the control group for 12 h. After treatment, all cells were lysed using RIPA buffer (Merck Millipore, Burlington, MA, USA). The samples were centrifuged at 12,000 rpm for 10 min and the supernatant was collected; protein concentration was quantified using a BCA protein assay kit (Thermo Fisher Scientific, Waltham, MA, USA). Proteins were separated using 10% sodium dodecyl sulphate-polyacrylamide gel electrophoresis (SDS-PAGE) and transferred onto a polyvinylidene fluoride (PVDF) membrane (Merck Millipore). The membrane was blocked using BlockPRO Blocking Buffer (Energenesis Biomedical Co., Ltd., Taipei, Taiwan) for 1 h at room temperature. After washing with Tris-buffered saline-Tween (TBST), the membrane was incubated overnight with the primary antibodies at 4 °C. Information on the primary antibodies is presented in . The membrane was washed with TBST and incubated with HRP-conjugated secondary antibodies for 1 h. Finally, the membrane was treated with enhanced chemiluminescence reagents (ECL; Thermo Fisher Scientific); protein signal was detected using Touch Imager (e-BLOT; Shanghai, China), and protein expression was quantified using ImageJ software.
Table 1. Primary antibodies used.
In vitro wound healing assay
A two-well culture insert (Blossom Biotechnologies Inc., Taipei, Taiwan) was placed in a 6-cm dish, and HepG2 cells were seeded in the culture insert. When the cells were confluent, the culture-insert was removed, and each dish was washed with PBS; 40 μM of 734THIF, 784THIF, or control DMEM solution was added. The cells were first incubated under hypoxic conditions for 6 h, followed by normoxic conditions. Images were captured at 0, 6, 24, 48, and 72 h. The percentage of wound healing area was quantified using ImageJ software. The percentage of wound healing area was calculated as follows
where x h is the area at different time points.
Measurement of 734THIF and 784THIF levels in HepG2 cells
HepG2 cells were seeded in a 12-well plate (5 × 105/well) for 24 h and then cultured with 40 μM 734THIF or 784THIF for various periods under normoxic and hypoxic conditions in FBS-free medium. We collected HepG2 cells under normoxic (6 h and 12 h), hypoxic (6 h), post-hypoxic (6 h of normoxia followed by 6 h of hypoxia), and pre-hypoxic conditions (6 h of hypoxia followed by 6 h of normoxia). Harvested cells were lysed with 0.5% SDS, washed with methanol, and sonicated for 30 min. The levels of 734THIF and 784THIF in all cell samples passed through a 0.45-μm filter was determined using HPLC. The HPLC system (Chromaster system, Hitachi, Tokyo, Japan) contained a 5410 ultraviolet (UV) detector, 5210 auto sampler, 5110 pump, and a column (250 × 4.6 mm, 5 μm; Mightysil RP-18 GP, Kanto Chemical Co., Inc., Tokyo, Japan) was used to elute the compound. The mobile phase contained 10 mM KH2PO4 with acetonitrile (65:35, v/v; pH 2.8). The detection wavelength was 262 nm and the flow rate and injection volume were 1 ml/min and 20 μL, respectively. A standard curve of 734THIF or 784THIF generated using HPLC was used to calculate the concentration of each sample.
Statistical analysis
The experimental data were analysed and compared using Microsoft 365 Excel software and SPSS software version 19. A one-way ANOVA was used to compare data from multiple groups, and Tukey’s test was used to determine significant differences. Data are presented as mean ± SD, and results with p < 0.05 were considered significant.
Results
Molecular docking of 734THIF and 784THIF within COX-2 and VEGFR2
Owing to a lack of potential target protein studies for 734THIF and 784THIF, these two compounds were evaluated using hypoxia-related COX-2 and VEGFR2 active site docking assays. The crystal structures of COX-2 were used; a known COX-2 selective inhibitor, celecoxib, was used as the reference. Daidzein was used as the control. Similar to celecoxib, 734THIF and 784THIF can form hydrogen bonds (HB) with HIS 75 of COX-2 (). Daidzein did not form the same HB with celecoxib and COX-2. Daidzein formed HB with SER 339, TYR 371 and SER 516, and showed hydrophobic interactions with VAL 335, LEU338, SER 339, VAL 509, and GLY 512 (). In addition, 734THIF formed HB with TYR 341 and SER 516 and showed hydrophobic interactions with VAL 335, ALA 502, VAL 509, and ALA 513 (). 784THIF formed HB with SER 339 and SER 516 and showed hydrophobic interactions with VAL 335, LEU 338, ALA 502, VAL 509, and ALA 513 (). Daidzein, 734THIF and 784THIF have similar docking scores of −31.44, −31.20, and −31.43 kcal/mol, respectively (). In addition, the minimum binding energy of these compounds was −30.91, −41.16 and −41.69 kcal/mol, respectively. The docking score and minimum binding energy of celecoxib were −20.62 and −32.44 kcal/mol, respectively ().
Figure 2. Three-dimensional (3D) and two-dimensional (2D) schematic diagrams of the docking poses of celecoxib (A), daidzein (B), 734THIF (C), and 784THIF (D) within the COX-2 active site (PDB code 3LN1). Green structures in the 3D plot: the analysed compounds.
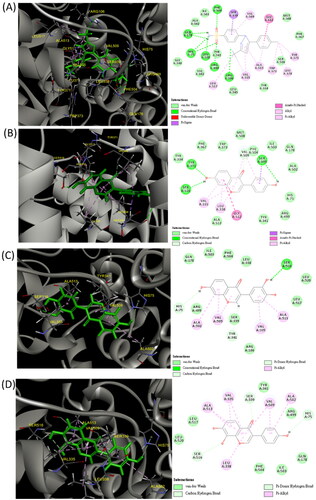
Table 2. Docking score and binding energy of daidzein, 734THIF, 784THIF, celecoxib, and sorafenib with COX-2 and VEGFR2.
In the docking assay with VEGFR2, sorafenib was used as the reference (). Daidzein, 734THIF and 784THIF formed HB with GLU 885 and CYS 919 of VEGFR2, and 784THIF additionally formed hydrogen bonds with ASP 1046 (). Sorafenib also interacted through HB with these three residues (). In addition, daidzein, 734THIF and 784THIF showed similar interactions with VEGFR2 residues (), hydrophobic interactions (LEU 840, VAL 848, ALA 866, VAL 899, VAL 916, and LEU 1035), electrostatic interactions (LYS 868), and Pi-sulphur (CYS 1045); the hydrophobic interactions were the same as that in sorafenib (). The docking score of daidzein, 734THIF and 784THIF was −25.65, −28.96, and −26.61 kcal/mol, respectively; the minimum binding energy was −44.02, −63.37, and −60.23 kcal/mol, respectively (). The docking score and minimum binding energy of sorafenib were −45.01 and −94.20 kcal/mol, respectively. These results showed that daidzein has lower binding affinity for both COX-2 and VEGFR2 than 734THIF and 784THIF. Therefore, subsequent in vitro studies will focus on comparing the differences between these two structural isomers.
Effects of 734THIF and 784THIF on cell viability in human HepG2 cells under hypoxia
The effects of 734THIF and 784THIF on the viability of HepG2 cells under the following conditions were evaluated: normoxia, post-hypoxia, and pre-hypoxia. Under normoxia and pre-hypoxia conditions, 734THIF showed significant effects compared to the control group at all doses, while under post-hypoxia condition, the significant effects started from 20 µM. 784THIF showed significant effects compared to the control group under normoxia and post-hypoxia conditions starting from 10 µM, while under pre-hypoxia condition, it started from 20 µM. (). 734THIF inhibited the growth of HepG2 cells greater under post-hypoxic conditions than under the other conditions, inhibiting cell viability to 62.9% at 40 μM (). 784THIF inhibited the growth of HepG2 cells more under normoxic conditions than under the other conditions, with an inhibition rate of 67.7% at 40 μM (). In the same oxygen conditions (normoxia, post-hypoxia, or pre-hypoxia), 40 and 80 μM of 734THIF, and 784THIF have no significant effect on cell viability. Therefore, 40 μM 734THIF or 784THIF was used in the subsequent experiments.
Expression of hypoxia-related proteins in HepG2 cells treated with 734THIF and 784THIF under hypoxic conditions
Under post-hypoxic conditions, 784THIF significantly inhibited hypoxia-induced HIF-1α expression (). In contrast, HIF-1α expression was not induced under pre-hypoxic conditions but was significantly induced by 734THIF treatment (). Under hypoxic conditions, 734THIF and 784THIF significantly inhibited hypoxia-induced VEGF expression (), whereas under pre-hypoxic conditions, 784THIF only slightly inhibited VEGF expression (). Caveolin-1 (CAV-1), the major caveolae component regulated by HIF-1α, is associated with cancer malignancy.Citation18 CAV-1 expression remained unaltered under post-hypoxic conditions and slightly induced under pre-hypoxic conditions (). 784THIF significantly inhibited the expression of CAV-1 under pre-hypoxic conditions (). Therefore, the expression of hypoxia-related proteins was inhibited by 784THIF in HepG2 cells.
Figure 5. Expression of hypoxia-related proteins in HepG2 cells. (A), (C), and (E) indicate the expression of HIF1a, VEGF, and CAV-1, respectively, under post-hypoxic conditions; (B), (D), and (F) indicate the expression of HIF1a, VEGF, and CAV-1, respectively, under pre-hypoxic conditions. n = 3 in each group; #P < 0.05 compared with normoxic control; * P < 0.05 compared with hypoxic control; $P < 0.05 734THIF compared with 784THIF.

734THIF and 784THIF inhibited the expression of a pro-inflammatory protein and regulated the expression of oxidative homeostasis-related proteins
734THIF and 784THIF significantly inhibited hypoxia-induced COX-2 expression under both post- and pre-hypoxic conditions (). NRF-2 was significantly induced under post-hypoxic conditions, and both 734THIF and 784THIF significantly reduced the expression of NRF-2 (). In contrast, NRF-2 expression was significantly decreased under pre-hypoxic conditions, whereas 734THIF and 784THIF restored and enhanced NRF-2 expression (). Sirtuin-1 (SIRT-1), a class III histone deacetylase, maintains the balance of intracellular ROS by indirectly regulating cellular antioxidant factors.Citation19 Under post-hypoxic conditions, SIRT-1 expression was downregulated; it was significantly rescued by 784THIF treatment (). The expression of SIRT-1 was downregulated under pre-hypoxic conditions; however, the expression was restored after treatment with 734THIF and 784THIF (). Therefore, both compounds could inhibit the hypoxia-induced expression of COX-2 and regulate the expression of intracellular oxidative homeostasis-related proteins, NRF-2 and SIRT-1.
Figure 6. Expression of a pro-inflammatory protein and oxidative homeostasis-related proteins in HepG2 cells. (A), (C), and (E) indicate the expression of COX-2, NRF-2, and SIRT-1, respectively, under post-hypoxic conditions; (B), (D), and (F) indicate the expression of COX-2, NRF-2, and SIRT-1, respectively, under pre-hypoxic conditions. n = 3 in each group; #P < 0.05 compared with normoxic control; * P < 0.05 compared with hypoxic control; $P < 0.05 734THIF compared with 784THIF.
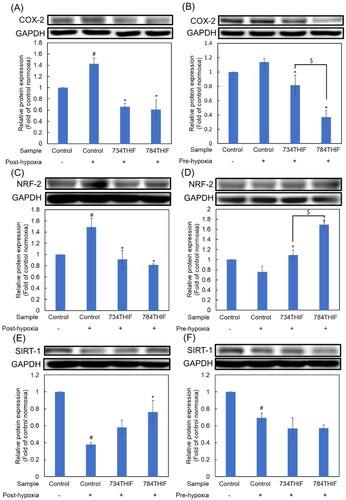
Treatment of HepG2 cells with 734THIF and 784THIF under hypoxic conditions altered epithelial–mesenchymal transition-related protein expression
Under both post-hypoxic and pre-hypoxic conditions, the expression of the epithelial marker E-cadherin (E-cad) was slightly decreased (). 734THIF and 784THIF significantly upregulated E-cadherin expression under hypoxic conditions (). N-Cadherin (N-cad) and vimentin are mesenchymal cell markers. Under hypoxic conditions, 734THIF and 784THIF significantly inhibited hypoxia-induced N-cad expression (). In addition, 734THIF and 784THIF significantly inhibited N-cad expression under pre-hypoxic conditions (). Vimentin expression was elevated under post-hypoxic conditions and was reduced under pre-hypoxic conditions (). However, 784THIF significantly reduced vimentin expression under both conditions. Therefore, 734THIF and 784THIF may reduce the expression of mesenchymal markers in HepG2 cells; 784THIF was more effective.
Figure 7. Expression of EMT makers in HepG2 cell. (A), (C), and (E) indicate the expression of E-cad, N-cad, and vimentin, respectively, under post-hypoxic conditions; (B), (D), and (F) indicate the expression of E-cad, N-cad, and vimentin, respectively, under pre-hypoxic conditions. n = 3 in each group; #P < 0.05 compared with normoxic control; * P < 0.05 compared with hypoxic control; $P < 0.05 734THIF compared with 784THIF.
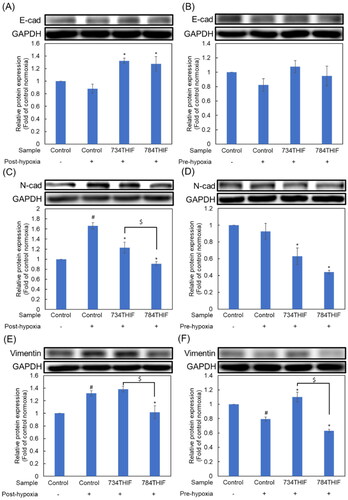
734THIF and 784THIF suppressed the expression of the metastatic protein MMP-9 in HepG2 cells and their migration ability under hypoxia
MMP-9 expression was significantly induced by hypoxia under post-hypoxic conditions, and both 734THIF and 784THIF significantly inhibited the expression of MMP-9 (). Under pre-hypoxic conditions, MMP-9 expression was not induced, and 734THIF and 784THIF reduced MMP-9 expression, but there was no statistical difference (). The wound healing assay showed that both 734THIF and 784THIF significantly inhibited the migration of HepG2 cells from 24 to 72 h (). Compared with that in the hypoxic control group, 734THIF and 784THIF inhibited the cell migration rate by up to 42.7% and 62.3%, respectively (). Thus, both compounds significantly inhibited the mobility of HepG2 cells under hypoxic conditions; 784THIF treatment was more effective.
Figure 8. Expression of MMP-9 in and migration rate of HepG2 cells treated with 734THIF and 784THIF. The expression of MMP-9 under post-hypoxic (A) and pre-hypoxic conditions (B), respectively. Wound healing assay (C) and its closure percentage at different time points (D). n = 3 in each group; #P < 0.05: compared with normoxic control; *P < 0.05: compared with hypoxic control; $P < 0.05: 734THIF compared with 784THIF.
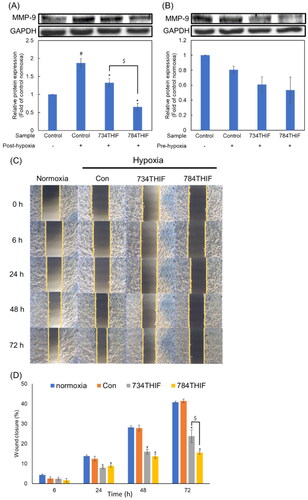
734THIF and 784THIF upregulated the expression of mitogen-activated protein kinases (MAPKs) and inhibited the expression of anti-apoptosis proteins under hypoxia
Under both post-hypoxic and pre-hypoxic conditions, the expression of phospho-ERK (p-ERK) and phospho-p38 (p-p38) significantly increased in HCC cells treated with 734THIF and 784THIF (). Interestingly, the increase in p-ERK expression was significantly higher in 734THIF treatment compared with that in 784THIF treatment, under both hypoxic conditions (). The expression of BCL-2 significantly increased under post-hypoxic conditions; however, 734THIF and 784THIF significantly reduced the expression of BCL-2 under both hypoxic conditions (). Therefore, 734THIF and 784THIF significantly increased the expression of MAPKs and inhibited anti-apoptotic protein expression under both hypoxic conditions.
Figure 9. Expression of MAPKs (p-ERK and p-p38) and anti-apoptosis proteins in HepG2 cells. (A), (C), and (E) indicate the expression of p-ERK, p-p38, and BCL-2, respectively, under post-hypoxic conditions; (B), (D), and (F) indicate the expression of p-ERK, p-p38, and BCL-2, respectively, under pre-hypoxic conditions. n = 3 in each group; #P < 0.05 compared with normoxic control; * P < 0.05 compared with hypoxic control; $P < 0.05 734THIF compared with 784THIF.
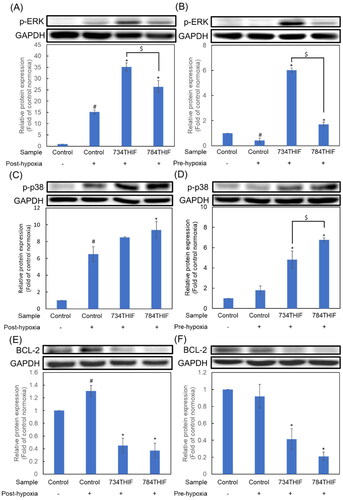
Inhibition of hypoxia-induced protein expression rate by 734THIF and 784THIF in hypoxic conditions
In , we extracted all western blot data and analysed the percentage of induced protein expression inhibition by 734THIF and 784THIF under post-hypoxia and pre-hypoxia conditions. The results showed that 784THIF had a better inhibition rate than 734THIF in almost all proteins and hypoxia conditions. 784THIF showed significantly higher inhibition rates than 734THIF against N-cadherin and MMP-9 in post-hypoxia, and against CAV-1 and COX-2 in pre-hypoxia.
Table 3. Percentage of protein expression inhibition by 734THIF and 784THIF under different hypoxic conditions.
Tumour hypoxic microenvironment affects cellular drug uptake
The uptake of 734THIF by HepG2 cells was greater than that of 784THIF after 6 h under normoxic or hypoxic conditions (). However, under post-hypoxic, pre-hypoxic, and 12-h normoxic conditions, the level of 784THIF in HepG2 cells was higher than that of 734THIF. In addition, the intracellular levels of 734THIF and 784THIF under post-hypoxic conditions was 66.85% and 29.47% lower, respectively, than that under 6-h normoxic conditions. The intracellular levels of 734THIF and 784THIF under pre-hypoxic conditions were 84.06% and 32.60% lower than that under 6-h hypoxic conditions. Therefore, 784THIF exhibits better cellular uptake and slower degradation than 734THIF under post- and pre-hypoxic conditions.
Table 4. 734THIF and 784THIF levels in HepG2 cells under normoxic and different hypoxic conditions.
Discussion
TACE is a key treatment option for unresectable HCC; however, hypoxia could lead to a poor prognosis and tumour recurrence. Following TACE treatment, the increased VEGF level in blood samples could induce considerable neovascularisation.Citation4 Most HCC cases are associated with chronic inflammation caused by hepatitis virus infection or alcohol intake; a pro-inflammatory environment promotes tumour development.Citation1 Therefore, in this study, we aimed to identify drugs that can simultaneously inhibit hypoxia-induced angiogenesis and inflammation. In silico, 734THIF and 784THIF formed hydrogen bonds and other non-covalent bonds with the active site residues of COX-2 and VEGFR2. Flavonoids with good molecular docking results with COX-2 proteins have been reported.Citation20 734THIF and 784THIF had better docking score and binding energy than celecoxib and inhibited COX-2 expression. VEGFR2 is a receptor tyrosine kinase activated by VEGF to promote angiogenesis and metastasis-related pathways.Citation21 734THIF and 784THIF have a similar flavonoid backbone and a hydroxyl substitution at position 6 (A ring); therefore, they are potential candidates for anti-angiogenic agents.Citation22 734THIF and 784THIF had lower binding affinity to VEGFR2 than sorafenib () but showed certain inhibitory effects on angiogenesis and metastasis ( and ), with 784THIF being more effective. This finding could be attributed to the higher number of hydroxyl substitutions on the A ring, which can form more hydrogen bonds.
In our previous study, we established a biological activity platform to evaluate the anti-cancer effect of natural products under hypoxic conditions,Citation17 simulating the situation of TACE treatment for HCC combined with sorafenib.Citation10 The first mode is post-hypoxia, in which the therapeutic is applied to HCC cells before hypoxia, and the second mode is pre-hypoxia, in which it is applied along with the application of hypoxic conditions. In cell viability analysis, 734THIF was more effective in suppressing HCC cell growth in post-hypoxic conditions, while 784THIF was more effective in suppressing HCC cells under normal oxygen conditions (). Therefore, we further analysed the molecular mechanisms of these two analogues under different hypoxic conditions. HCC inflammation and TACE-induced hypoxic environment induce HIF-1α and COX-2 expression to promote tumour angiogenesis and EMT processes.Citation7 COX-2 is an important mediator of the inflammatory pathways and is highly expressed in various cancers.Citation23 784THIF significantly inhibited hypoxia-induced HIF-1α and COX-2 expression under hypoxic conditions, whereas 734THIF did not inhibit HIF-1α expression. In addition, the COX-2 expression results were consistent with the molecular docking results (). HIF-1α and COX-2 induce the expression of pro-angiogenic factors, such as VEGF, in tumours and promote angiogenesis.Citation5,Citation24 784THIF and 734THIF significantly inhibited hypoxia-induced VEGF expression under post-hypoxic conditions, whereas 784THIF had a better inhibitory effect than 734THIF under pre-hypoxic conditions. Liu et al. have shown that CAV-1 overexpression is a potential prognostic marker for HCC patients after curative resection.Citation25 HIF-1α induces CAV-1 transcription under hypoxia, which enhances HCC cell invasion and metastasis. Suppressing CAV-1 expression effectively reduces HIF-1α expression and improves hypoxia.Citation26 784THIF can inhibit CAV-1 expression in both hypoxic conditions (), with a significantly higher inhibition rate in pre-hypoxia than 734THIF (). EMT is involved in the transformation of primary epithelial cancer cells into invasive and metastatic mesenchymal cell types. The molecular mechanism of EMT involves decreased expression of the epithelial marker E-cadherin and increased expression of mesenchymal markers, such as N-cadherin, vimentin, and fibronectin.Citation27 784THIF significantly increased E-cadherin expression under post-hypoxic conditions and decreased N-cadherin and vimentin expression under both hypoxic conditions (). Therefore, 784THIF ameliorates the malignant potential of HepG2 cells under hypoxic conditions by inhibiting hypoxia-related inflammation, angiogenesis, and EMT processes.
Hypoxia increases oxidative stress in cancer cells and promotes cancer cell adaptation and progression.Citation28 NRF-2 and SIRT-1 are important proteins that maintain oxidative homeostasis in cells. NRF-2, which maintains intracellular oxidative homeostasis, is activated during hypoxia; it coordinates with the HIF-1 pathway to stimulate angiogenesis, chemoresistance, and metabolic remodelling, thereby contributing to tumour survival and progression.Citation28 734THIF and 784THIF significantly inhibited NRF-2 expression under hypoxic conditions. Interestingly, NRF-2 was not induced under pre-hypoxic conditions; however, 734THIF and 784THIF significantly increased NRF-2 expression. Therefore, normoxic conditions after 6 h of hypoxia restored cancer cells to a normal state, whereas 734THIF and 784THIF could suppress inflammatory responses such as COX-2 by upregulating NRF-2 expression.Citation29 NRF-2 binds to BCL-2 antioxidant response elements (AREs) and upregulates BCL-2 expression.Citation30 Irrespective of how 734THIF and 784THIF regulated NRF-2, they still inhibited the expression of BLC-2 in HepG2 cells under both hypoxic conditions. SIRT-1 expression is downregulated upon hypoxia in cancer cells, whereas its activation inhibits hypoxia-promoted cancer cell migration.Citation8 784THIF significantly restored hypoxia-suppressed SIRT-1 expression under hypoxic conditions and inhibited HepG2 cell migration.
Activation of COX-2 further increases resistance to cancer cell apoptosis by upregulating BCL-2 expression; upregulation of MMP2 and MMP9 expression promotes metastasis.Citation31 Here, 784THIF inhibited hypoxia-induced MMP9 expression and HepG2 cell migration more than 734THIF. The activation of p38 and ERK in the MAPK family reduces the expression of the anti-apoptotic protein BCL-2 and induces apoptosis in HCC cells.Citation32 Here, both 734THIF and 784THIF significantly increased MAPK expression and decreased BCL-2 expression under both hypoxic conditions (). Therefore, 734THIF and 784THIF could effectively regulate intracellular oxidative stress-related proteins under hypoxic conditions. In addition, they could induce apoptosis and inhibit the migration of HepG2 cells.
Hypoxia in the tumour microenvironment leads to altered metabolism of cancer cells through the activation of HIF, resulting in hypoxia-induced drug resistance, further inhibiting drug delivery, uptake, and efficacy.Citation33 Therefore, the identification of hypoxia-resistant drugs is important. The 734THIF level in HepG2 cells was higher than 784THIF in the first 6 h under hypoxic and normoxic conditions. However, 734THIF was degraded faster than 784THIF under pre- or post-hypoxic conditions, and the intracellular level of 734THIF was also lower than that of 784THIF (). In addition, the 784THIF level in cells was not significantly different under normoxic and hypoxic conditions. 784THIF had better cellular uptake under both post-and pre-hypoxic conditions than 734THIF. In addition, 784THIF is less susceptible to hypoxic microenvironments and exhibits slower degradation than 734THIF. Pre-treatment with sorafenib before TACE could improve prognosis because the drug could be homogeneously distributed within the tumour and inhibit the expression of HIF-1α-induced proteins such as VEGF.Citation10 This result may be related to the hypoxic conditions and cellular drug uptake during TACE treatment. The hypoxia tolerance and natural medicinal properties of 784THIF may ameliorate the limitations of TACE and TACE combined with sorafenib in the treatment of HCC.
We analysed the docking of 734THIF and 784THIF with COX-2 and VEGFR2 using an in silico assay; these two compounds had better docking scores than the known COX-2 inhibitor. 784THIF could improve the regulation of HepG2 growth under hypoxia by downregulating hypoxia-induced inflammation and metastasis-related protein expression, regulating oxidative stress, inducing cancer cell apoptosis, and inhibiting cell proliferation. 784THIF exhibited better cellular uptake than 734THIF under both post-hypoxic and pre-hypoxic conditions; it was not affected by hypoxia in terms of intracellular drug level. Therefore, 784THIF has the potential to be a candidate drug for the treatment of hypoxic HCC, but further clinical studies are still needed in the future.
Author contribution
Wen-Sheng Tzeng: Conceptualisation and Writing - Original Draft. Wei-Lin Teng: Methodology, Validation, Investigation, and Writing - Original Draft. Pao-Hsien Huang: Validation and Investigation. Feng-Lin Yen: Conceptualisation, Project administration, Writing- Reviewing and Editing. Yow-Ling Shiue: Conceptualisation. All authors provided the final approval of the version to be published and all authors agree to be accountable for all aspects of the work.
Acknowledgements
The authors thank Professor Chih-Hua Tseng who provided 7,3′,4′-trihydroxyisoflavone and 7,8,4′-trihydroxyisoflavone..
Disclosure statement
The authors report there are no competing interests to declare.
Data availability statement
The data that support the findings of this study are available from the corresponding author on reasonable request. Some data may not be made available because of privacy or ethical restrictions.
Additional information
Funding
References
- Li X, Ramadori P, Pfister D, Seehawer M, Zender L, Heikenwalder M. The immunological and metabolic landscape in primary and metastatic liver cancer. Nat Rev Cancer. 2021;21(9):541–557.
- Reig M, Forner A, Rimola J, Ferrer-Fàbrega J, Burrel M, Garcia-Criado Á, Kelley RK, Galle PR, Mazzaferro V, Salem R, et al. BCLC strategy for prognosis prediction and treatment recommendation: The 2022 update. J Hepatol. 2022;76(3):681–693.
- Chang Y, Jeong SW, Young Jang J, Kim JY. Recent updates of transarterial chemoembolilzation in hepatocellular carcinoma. Int J Mol Sci. 2020;21(21):1–20.
- Lin Z-H, Jiang J-R, Ma X-K, Chen J, Li H-P, Li X, Wu X-Y, Huang M-S, Lin Q. Prognostic value of serum HIF-1alpha change following transarterial chemoembolization in hepatocellular carcinoma. Clin Exp Med. 2021;21(1):109–120.
- Liu K, Min XL, Peng J, Yang K, Yang L, Zhang XM. The changes of HIF-1alpha and VEGF expression after TACE in patients with hepatocellular carcinoma. J Clin Med Res. 2016;8(4):297–302.
- Korbecki J, Siminska D, Gassowska-Dobrowolska M, Listos J, Gutowska I, Chlubek D, et al. Chronic and cycling hypoxia: drivers of cancer chronic inflammation through HIF-1 and NF-kappaB activation: a review of the molecular mechanisms. Int J Mol Sci. 2021;22(19):10701.
- Huang M, Wang LONG, Chen J, Bai M, Zhou C, Liu S, Lin QU. Regulation of COX-2 expression and epithelial-to-mesenchymal transition by hypoxia-inducible factor-1alpha is associated with poor prognosis in hepatocellular carcinoma patients post TACE surgery. Int J Oncol. 2016;48(5):2144–2154.
- Yu S, Zhou R, Yang T, Liu S, Cui Z, Qiao Q, Zhang J. Hypoxia promotes colorectal cancer cell migration and invasion in a SIRT1-dependent manner. Cancer Cell Int. 2019;19(1):116.
- Wu S, Lu H, Bai Y. Nrf2 in cancers: A double-edged sword. Cancer Med. 2019;8(5):2252–2267.
- Kudo M, Ueshima K, Ikeda M, Torimura T, Tanabe N, Aikata H, Izumi N, Yamasaki T, Nojiri S, Hino K, et al. Randomised, multicentre prospective trial of transarterial chemoembolisation (TACE) plus sorafenib as compared with TACE alone in patients with hepatocellular carcinoma: TACTICS trial. Gut. 2020;69(8):1492–1501.
- Li Y, Gao ZH, Qu XJ. The adverse effects of sorafenib in patients with advanced cancers. Basic Clin Pharmacol Toxicol. 2015;116(3):216–221.
- Liao C-Y, Lee C-C, Tsai C-c, Hsueh C-W, Wang C-C, Chen I-H, Tsai M-K, Liu M-Y, Hsieh A-T, Su K-J, et al. Novel investigations of flavonoids as chemopreventive agents for hepatocellular carcinoma. Biomed Res Int. 2015;2015:840526–840542.
- Roh C. Microbial transformation of bioactive compounds and production of ortho-dihydroxyisoflavones and glycitein from natural fermented soybean paste. Biomolecules. 2014;4(4):1093–1101.
- Huang PH, Tseng CH, Lin CY, Lee CW, Yen FL. Preparation, characterizations and anti-pollutant activity of 7,3',4'-trihydroxyisoflavone nanoparticles in particulate matter-induced HaCaT keratinocytes. Int J Nanomedicine. 2018;13:3279–3293.
- Kim E, Kang YG, Kim JH, Kim YJ, Lee TR, Lee J, et al. The antioxidant and anti-inflammatory activities of 8-hydroxydaidzein (8-HD) in activated macrophage-like RAW264.7 cells. Int J Mol Sci. 2018;19(7):1828.
- Wu G, Robertson DH, Brooks CL, 3rd, Vieth M. Detailed analysis of grid-based molecular docking: A case study of CDOCKER-A CHARMm-based MD docking algorithm. J Comput Chem. 2003;24(13):1549–1562.
- Tzeng WS, Teng WL, Huang PH, Lin TC, Yen FL, Shiue YL. Pterostilbene nanoparticles downregulate hypoxia-inducible factors in hepatoma cells under hypoxic conditions. Int J Nanomed. 2021;16:867–879.
- Castillo Bennett J, Silva P, Martinez S, Torres VA, Quest AFG. Hypoxia-induced caveolin-1 expression promotes migration and invasion of tumor cells. Curr Mol Med. 2018;18(4):199–206.
- Singh CK, Chhabra G, Ndiaye MA, Garcia-Peterson LM, Mack NJ, Ahmad N. The role of sirtuins in antioxidant and redox signaling. Antioxid Redox Signal. 2018;28(8):643–661.
- Dash R, Uddin MM, Hosen SMZ, Rahim ZB, Dinar A, Kabir M, Sultan RA, Islam A, Hossain MK. Molecular docking analysis of known flavonoids as duel COX-2 inhibitors in the context of cancer. Bioinformation. 2015;11(12):543–549.
- Chen L, Lin G, Chen K, Liang R, Wan F, Zhang C, Tian G, Zhu X. VEGF promotes migration and invasion by regulating EMT and MMPs in nasopharyngeal carcinoma. J Cancer. 2020;11(24):7291–7301.
- Rajesh G, Harshala S, Dhananjay G, Jadhav A, Vikram G. Effect of hydroxyl substitution of flavone on angiogenesis and free radical scavenging activities: a structure-activity relationship studies using computational tools. Eur J Pharm Sci. 2010;39(1-3):37–44.
- Gandhi J, Khera L, Gaur N, Paul C, Kaul R. Role of modulator of inflammation cyclooxygenase-2 in gammaherpesvirus mediated tumorigenesis. Front Microbiol. 2017;8:538.
- Toomey DP, Murphy JF, Conlon KC. COX-2, VEGF and tumour angiogenesis. Surgeon. 2009;7(3):174–180.
- Liu W-R, Jin L, Tian M-X, Jiang X-F, Yang L-X, Ding Z-B, Shen Y-H, Peng Y-F, Gao D-M, Zhou J, et al. Caveolin-1 promotes tumor growth and metastasis via autophagy inhibition in hepatocellular carcinoma. Clin Res Hepatol Gastroenterol. 2016;40(2):169–178.
- Mao X, Wong SYS, Tse EYT, Ko FCF, Tey SK, Yeung YS, Man K, Lo RC-L, Ng IO-L, Yam JWP, et al. Mechanisms through which hypoxia-induced caveolin-1 drives tumorigenesis and metastasis in hepatocellular carcinoma. Cancer Res. 2016;76(24):7242–7253.
- Tam SY, Wu VWC, Law HKW. Hypoxia-induced epithelial-mesenchymal transition in cancers: HIF-1alpha and beyond. Front Oncol. 2020;10:486.
- Toth RK, Warfel NA. Strange bedfellows: nuclear factor, erythroid 2-like 2 (Nrf2) and hypoxia-inducible factor 1 (HIF-1) in tumor hypoxia. Antioxidants (Basel). 2017;6(2):27.
- Ahmed SM, Luo L, Namani A, Wang XJ, Tang X. Nrf2 signaling pathway: pivotal roles in inflammation. Biochim Biophys Acta Mol Basis Dis. 2017;1863(2):585–597.
- Niture SK, Jaiswal AK. Nrf2 protein up-regulates antiapoptotic protein Bcl-2 and prevents cellular apoptosis. J Biol Chem. 2012;287(13):9873–9886.
- Hashemi Goradel N, Najafi M, Salehi E, Farhood B, Mortezaee K. Cyclooxygenase-2 in cancer: a review. J Cell Physiol. 2019;234(5):5683–5699.
- Zhang G, He J, Ye X, Zhu J, Hu X, Shen M, Ma Y, Mao Z, Song H, Chen F, et al. beta-Thujaplicin induces autophagic cell death, apoptosis, and cell cycle arrest through ROS-mediated Akt and p38/ERK MAPK signaling in human hepatocellular carcinoma. Cell Death Dis. 2019;10(4):255.
- Ho YJ, Thao DT, Yeh CK. Overcoming hypoxia-induced drug resistance via promotion of drug uptake and reoxygenation by acousto-mechanical oxygen delivery. Pharmaceutics. 2022;14(5):902.