ABSTRACT
Introduction: Adjuvants are used to improve vaccine immunogenicity and efficacy by enhancing antigen presentation to antigen-specific immune cells with the aim to confer long-term protection against targeted pathogens. Adjuvants have been used in vaccines for more than 90 years. Combinations of immunostimulatory molecules, such as in the Adjuvant System AS01, have opened the way to the development of new or improved vaccines.
Areas covered: AS01 is a liposome-based vaccine adjuvant system containing two immunostimulants: 3-O-desacyl-4ʹ-monophosphoryl lipid A (MPL) and the saponin QS-21. Here we describe studies investigating the mode of action of AS01, and consider the role of AS01 in enhancing specific immune responses to the antigen for selected candidate vaccines targeting malaria and herpes zoster. The effects of AS01 are rapid and transient, being localized to the injected muscle and draining lymph node. AS01 is efficient at promoting CD4+ T cell-mediated immune responses and is an appropriate candidate adjuvant for inclusion in vaccines targeting viruses or intracellular pathogens.
Expert commentary: AS01 activity to enhance adaptive responses depends on synergistic activities of QS-21 and MPL. AS01 adjuvantation shows good prospects for use in new vaccines targeted to populations with challenging immune statuses and against diseases caused by complex pathogens.
1. Introduction
Vaccination is one of the most successful public health interventions ever undertaken. Underpinning the success of many of the vaccines developed and licensed for use since the early 1900s is the ability of the vaccine antigens to induce a humoral immune response, combined with the susceptibility of the target pathogens to antibody-mediated killing. Despite the enormous success of vaccines in preventing morbidity and mortality due to pathogens of global importance, there are notable exceptions, such as malaria, tuberculosis, and human immunodeficiency virus (HIV). Disease due to these complex pathogens may require additional features of the immune response beyond antibodies, such as cell-mediated responses that result in the activation of effector CD4+ and CD8+ T cells. CD4+ T cells secrete cytokines that activate macrophages to destroy phagocytosed pathogens and limit pathogen survival and spreading. CD4+ T cells also provide help to antibody-producing B cells to facilitate the development of high-affinity antibodies and memory B cells. CD8+ effector cells secrete cytotoxic factors that kill pathogen-infected or transformed cells.
The development of prophylactic vaccines against complex pathogens requiring a multifaceted immune response for the prevention of infection has, until recently, been unsuccessful using the available vaccine technologies. Potential new technologies include the use of viral vectors to deliver antigen and novel vaccine administration routes (such as intranasal administration). However, the most advanced solution is in the field of vaccine adjuvants.
Adjuvants are combined with antigen to enhance the immunogenicity of the vaccine with the aim of conferring long-term protection. The first adjuvant used in human vaccines was aluminum salt, which has been included in vaccines since the 1920s to increase the magnitude of the antibody response [Citation1]. Because of their potential to modulate and enhance immune responses, adjuvants are enabling the development of vaccines for populations and pathogens for which traditional vaccines have been ineffective [Citation2,Citation3].
The immune response to pathogen/antigen occurs in two broad phases: the initial nonspecific innate response followed by the specific adaptive response (). Because adjuvants interact with the innate immune response, mimicking certain aspects of the natural response to pathogens, they can be used to enhance the desired adaptive response, thereby maximizing vaccine efficacy. Knowledge of the mode of action of an adjuvant allows a better understanding of the role of the adjuvant in the observed immunogenicity, reactogenicity, and safety profile of the vaccine [Citation4].
Figure 1. Current model of innate control of adaptive immunity. Adapted from Ishii et al. Curr Pharm Des 2006;12: 4135–42 © Bentham Science Publishers. Reproduced with permission. Immune responses to infection occur over a timescale of minutes to years (upper graph). Innate immune responses act minutes after infection, begin to clear the pathogen, and lasts for several days. While the adaptive immune responses are mount during the following weeks to generate a long lasting immunological memory. Minutes after a pathogen or vaccine enters the body, antigens are detected by the immune system (lower graph). Antigen-presenting cells take up, process and present these antigens to T cells. This first signal (1) is antigen-specific. It is provided through the T cell receptor which interacts with peptide-MHC molecules on the membrane of antigen presenting cells (APC). When additional signals (2) (pathogen defence triggers or adjuvants) are also recognized by pattern recognition receptors, pro-inflammatory cytokine production and up-regulation of co-stimulatory molecules occur (3). APCs activated by signals (1 + 2 (3)) promote naïve T cells to initiate adaptive immune responses, including T cell help for B cells and development of immune memory. Vaccine adjuvants can mimic immune defence triggers bore by pathogens (2) and contribute to elicit the activation of T-cells by antigen-presenting cells. Activation of T cells without defence triggers and co-stimulatory molecules/cytokines (ie. only signal (1)) may lead to tolerance/ignorance.
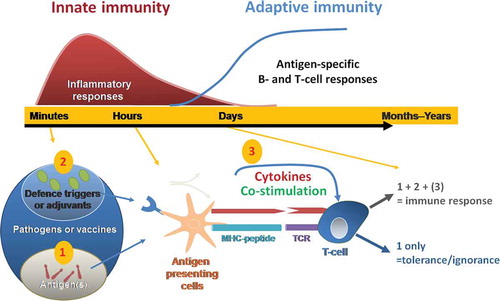
The Adjuvant System AS01 began being developed more than 20 years ago and is one of several proprietary Adjuvant Systems. AS01 is a liposome-based adjuvant that contains two immunostimulants: MPL and QS-21 (licensed by GSK from Antigenics Inc., a wholly owned subsidiary of Agenus Inc., a Delaware, USA corporation)[Citation5]. MPL (3-O-desacyl–monophosphoryl lipid A) is the detoxified derivative of lipopolysaccharide from Salmonella minnesota and stimulates activation of innate immunity via Toll-like receptor 4 (TLR4) [Citation6]. MPL directly activates antigen-presenting cells (APCs) expressing TLR4, stimulating the production of cytokines and co-stimulatory molecules [Citation7]. In mice, it is reported that MPL favors the induction of antigen-specific T cells producing interferon (IFN)-gamma and antibody isotype switching toward IgG2a/c antibodies [Citation8]. QS-21 is a saponin molecule extracted from the bark of the South American tree Quillaja saponaria Molina, fraction 21. QS-21 promotes antigen-specific antibody responses but also stimulates cytotoxic CD8+ T cells, most notably in nonhuman species [Citation9,Citation10]. In contrast to MPL, the signaling pathways triggered by QS-21 are not fully understood. QS-21 is a water-soluble triterpene glycoside with amphiphilic character known for its hemolytic activity. This hemolytic activity can be eliminated through formulation in liposomes in the presence of cholesterol, as has been done in AS01 [Citation11,Citation12]. QS-21 has been recently shown to activate specific innate pathways in monocytes (i.e. the ASC-NLRP3 inflammasome, a multi-protein complex leading to the release of IL-1β/IL-18 [Citation13]) but the role of this pathway in vivo remains to be determined.
Here, we review the current knowledge on the mode of action of AS01 and how this helps in understanding the immunogenicity profiles of candidate vaccines for human use. We specifically consider the role of AS01 in enhancing specific immune responses for selected candidate vaccines targeting malaria and herpes zoster.
2. Plasmodium and varicella-zoster virus: two pathogens with common challenges for vaccines
Malaria is estimated to cause 584,000 deaths in 2013, of which the majority occurred in children living in sub-Saharan Africa [Citation14]. Attempts to develop a malaria vaccine have been hindered by the complex life cycle of the Plasmodium parasite, much of which occurs intracellularly, by its large physical size, and by antigenic diversity among surface structures [Citation15]. Antibodies alone are not sufficient to clear malaria parasites at any stage in its life cycle, but effective T-cell responses are capable of identifying and killing malaria-infected hepatocytes [Citation16]. Because malaria deaths mainly occur at a young age, a successful malaria vaccine must be able to induce both humoral and T-cell responses in unprimed young children.
The candidate malaria vaccine contains RTS,S, a recombinant portion of the circumsporozoite protein of Plasmodium falciparum fused to hepatitis B surface antigen, i.e. administered with AS01. The vaccine aims to interrupt the preerythrocytic stage of the malaria life cycle. The vaccine needs to be able to induce both humoral immunity to prevent parasites from invading the liver and cell-mediated immunity facilitating the identification and destruction of newly infected hepatocytes.
By contrast, herpes zoster or shingles infrequently results in death but is associated with substantial morbidity and long-term sequelae in older adults age 50 years and over, and in the immunocompromised [Citation17,Citation18]. Herpes zoster occurs following reactivation of latent virus in dorsal root ganglia after varicella-zoster virus (VZV) infection; and primary VZV infection typically happens during childhood. Patients with herpes zoster frequently have circulating anti-VZV antibodies. A decline in cell-mediated immunity has been associated with VZV reactivation, and thus CMI is considered to be a key component ensuring suppression of latent virus.
The incidence and severity of herpes zoster increases as cell-mediated immunity declines [Citation19,Citation20]. A successful vaccine to prevent herpes zoster not only needs to boost preexisting cellular immunity induced by previous varicella infection or immunization, but also needs to be effective in both older individuals with impaired T-cell mechanisms due to immune senescence [Citation21,Citation22], and in specific high-risk groups with immunosuppression (caused by disease such as cancer or HIV, or by immunosuppressive treatment, e.g. in transplant recipients or patients with autoimmune diseases). The candidate herpes-zoster vaccine (HZ/su) currently in Phase-3 evaluation contains the VZV glycoprotein E subunit (gE) and AS01 [Citation23]. gE is abundantly expressed on the surface of varicella-zoster virions and by infected cells, and appears to be a major target for VZV-specific CD4+ T-cell responses [Citation24]. HZ/su was also designed to address the burden of herpes zoster in the immunocompromised population [Citation25,Citation26], where live-attenuated vaccine is contraindicated [Citation27].
While differing markedly in structure, life cycle, pathogenicity, and epidemiology, vaccines targeting malaria and herpes zoster face a common challenge: namely the requirement to induce strong cell-mediated responses in individuals with suboptimal (immature, senescent, or suppressed) immune function. The next section aims at describing the rationale for including AS01 in both vaccines to enhance both humoral and cell-mediated immune responses, starting with a summary of what is known about its mode of action.
3. The mode of action of AS01
The key role of innate immunity in the mode of action of adjuvants has been well described in recent reviews [Citation28,Citation29]. Several recently developed adjuvants target specific receptors of the innate response, such as TLRs, whereas for other adjuvants such as emulsions and aluminum salts, the exact molecular pathways responsible for the adjuvant effect in vivo are still unclear.
Adjuvants have been described to stimulate the production of cytokines by resident innate cells, including stromal cells. The cytokine release leads to the rapid recruitment of neutrophils, monocytes, and dendritic cells (DCs) that can all take up antigen [Citation7,Citation30–Citation33]. Some adjuvants also increase antigen uptake by these cells. The cells most critical to integrating and translating innate signals into antigen-specific effectors in the lymph node are APCs, in particular DCs, as shown by depletion experiments in mice [Citation34,Citation35]. Therefore, the specificity of adjuvants is likely to rely on the modulation of the innate response by each adjuvant, in particular, in differences in the quality and extent of activated APC subset.
As shown for other adjuvants, AS01 also interacts with the immune system at the level of the innate-immune response. Initial studies in mice confirm that the magnitude of the downstream adaptive humoral and cell-mediated immune response is higher when malaria and herpes-zoster antigens are co-injected with AS01 than when these antigens are administered alone [Citation36–Citation38]. In these experiments, AS01 performed better than other adjuvants. This indicates that the effects of AS01 on the innate response is particularly effective and successfully translates into improved antigen-specific responses.
A series of recently reported experiments conducted in mice describe the magnitude, quality, and kinetics of the innate-immune response induced by AS01 [Citation34]. Mice were used because numerous tools exist for studying the immune response at a cellular level in this animal model. The antigens used in the mouse-model experiments were ovalbumin (as a model antigen) and gE. The experiments described in Didierlaurent et al. aimed at (1) visualizing the localization of adjuvant and antigen molecules after intramuscular injection; (2) evaluating the duration and the profile of the local innate-immune response (site of injection and draining lymph node); and (3) analyzing the recruitment and activation of APCs, and their role in modulating the adaptive-immune response.
3.1. What happens after injection into muscle?
Fluorescently labeled QS-21 and gE were used to monitor the vaccine’s distribution after intramuscular injection. The data indicate that AS01 and gE are not retained in the muscle, excluding a mechanism of local depot as described for aluminum salts. Although QS-21 and antigen were visible in the muscle interstitium 30 min after injection, they were rapidly cleared and almost undetectable within 24 h ().
Figure 2. Immune responses following vaccination with AS01-adjuvanted vaccine at the injection site (muscle). The immune response to an AS01-adjuvanted vaccine injected into a muscle involves multiple cellular interactions. The adjuvant effect requires the spatial and temporal co-localization of AS01 and antigen. The two immune-stimulatory components of AS01, MPL and QS-21, act synergistically together to stimulate the release of immune mediators by innate cells allowing an enhanced neutrophil and monocyte recruitment at the injection site. The AS01-adjuvanted vaccine induces an early and transient activation of the innate immune response. Cellular and cytokine responses peak at day 1 and are fully resolved by day 7.
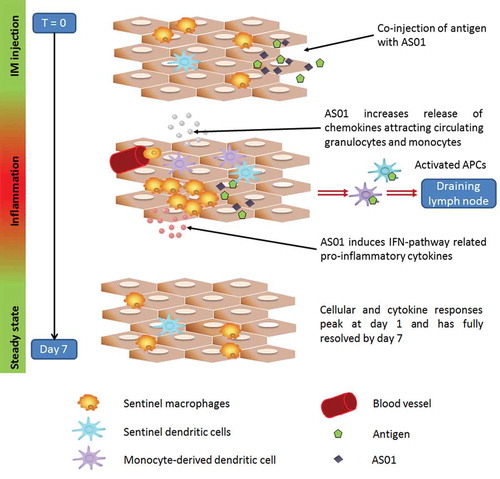
Cytokines were markedly higher in mice that received AS01 and gE compared with mice that had received gE alone. Cytokine levels began to increase in muscle within 3 h after injection, suggesting early production by resident cells, including stromal cells, peaking at day 1 before returning more gradually to baseline by day 7 ().
Figure 3. Concentrations of cytokines in muscle and draining lymph node after injection with AS01 and gE antigen (data from [Citation34]). In mice, AS01 induced rapid and transient cytokine production at the injection site in muscle and in the draining lymph node compared with antigen alone (gE/AS01 versus gE/no adjuvant). Graphs show geometric mean of cytokine concentrations (IL6, CXCL1, CCL2 and CXCL9 (ng/ml)) from tissue homogenates at 3, 6, 24, 48 and 168 hours (7 days) after injection.
![Figure 3. Concentrations of cytokines in muscle and draining lymph node after injection with AS01 and gE antigen (data from [Citation34]). In mice, AS01 induced rapid and transient cytokine production at the injection site in muscle and in the draining lymph node compared with antigen alone (gE/AS01 versus gE/no adjuvant). Graphs show geometric mean of cytokine concentrations (IL6, CXCL1, CCL2 and CXCL9 (ng/ml)) from tissue homogenates at 3, 6, 24, 48 and 168 hours (7 days) after injection.](/cms/asset/74790df4-ea84-4ffa-85cd-6921e598692d/ierv_a_1213632_f0003_oc.jpg)
Concomitant to the cytokine response, neutrophils and monocytes were rapidly recruited in the muscles in greater numbers when gE was coadministered with AS01 compared with gE alone, with levels returning to baseline at day 7.
3.2. What happens in the draining lymph node?
Fluorescently labeled QS-21 and gE were detectable in draining lymph nodes as early as 30 min after injection indicating rapid drainage from the injection site. QS-21 and gE were found at different areas within the lymph node and displayed different kinetics, suggesting that those two components were not physically associated after the injection.
The pattern of cytokine release and cellular recruitment in the draining lymph node mirrored that observed in muscle (). In addition to neutrophils and monocytes, there was also a rapid and substantial influx of DC and T-cell populations in the lymph node. Interestingly, in both muscle and lymph node, chemokines related to the IFN-pathway were significantly increased upon AS01 injection. Those chemokines, such as CXCL9 and CXCL-10, are known to recruit lymphocytes via CXCR3 interaction and have been shown to be associated with efficient induction of cellular responses [Citation39].
3.3. How does AS01 affect APCs?
AS01 enhanced the number of DCs and monocytes in the draining lymph node and significantly enhanced their surface levels of T-cell stimulatory molecules (CD86 and CD40). This effect was transient, peaked at day 1 and returns to baseline by day 7. Using fluorescently labeled gE, it was observed that monocytes and neutrophils were the main cells carrying the antigen, followed by DCs. Using an ex vivo antigen-presentation assay, activated DCs, isolated from the lymph node of mice vaccinated with antigen and AS01, were found to be the cells responsible for the efficient priming of CD4+ T cells (). Depletion of those cells in vaccinated mice completely abrogated the effect of AS01 on the specific response to coadministered antigens. A more detailed analysis of the different cell subsets revealed that the profile of the activated APCs in the draining lymph node was broad, including DCs resident in the lymph node, DCs that migrated from the injected muscle, and monocyte-derived DCs. Regarding the latter, AS01 appeared to enhance the differentiation of monocytes into DCs, thus leading to a more heterogeneous DC population and eventually to a more efficient antigen presentation to T cells. Unlike other adjuvants such as emulsions or aluminum salts, the effect of AS01 on APCs does not appear to involve an increase in antigen uptake [Citation7,Citation33].
Figure 4. Immune responses following vaccination with AS01-adjuvanted vaccine in the draining lymph node. Some early immunological events occurring at the injection site contribute to the immune response in the draining lymph node. Those include (i) the rapid drainage of some antigen and adjuvant within 30 minutes after vaccination and (ii) hours later, the migration, among other innate cells, of dendritic cells recruited from the injection site. While migrating to the draining lymph node, those dendritic cells breakdown/ present antigens and mature into efficient antigen presenting cells. In the draining lymph node, AS01 and antigen may activate resident dendritic cells. Those latter forms, with dendritic cells coming from the injection site form, a broad population of highly activated dendritic cells efficient at activating T cells. Activated T cells differentiate into effector populations and at a later stage into memory cells, meant to persist in our body to provide long term protection. Cytokines secreted by effector CD4 positive T cells stimulate antigen-specific B cells to divide into antibody-secreting cells and memory B cells. Ultimately, those memory and effector T or B cells, as well as antibodies, will leave the lymph node and enter the bloodstream.
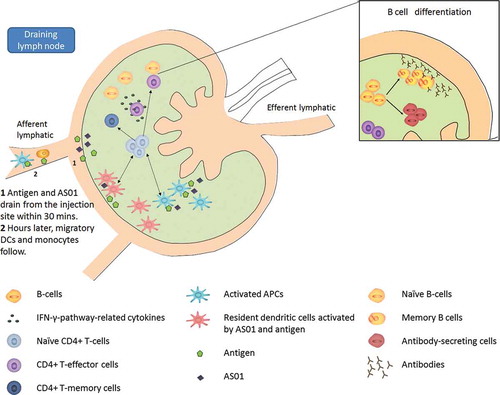
3.4. Co-localization of AS01 and antigen is necessary for adjuvant benefit
In order to investigate the period during which AS01 retains its adjuvant properties, AS01 was first injected followed by gE after different time intervals and at the same site. When gE was administered 24 and 72 h after AS01, the T-cell responses and antibody response, respectively, were no different than when gE antigen was administered alone. Similarly, when AS01 and gE were administered at the same time but in contralateral limbs, gE-specific responses were no different to gE administered alone. Therefore, the effect of AS01 on the antibody and T-cell responses to the vaccine antigen is spatially and temporally constrained, in line with the local and transient effect of AS01 on innate immunity.
3.5. MPL and QS-21 contribute synergistically to AS01 adjuvant effect
Combining MPL and QS-21 components in AS01 is required to achieve the highest antigen-specific adaptive response [Citation37]. QS-21 contributes the most to the antibody response, and the addition of MPL greatly enhances this response. Interestingly, the combination of both was essential to drive gE-specific polyfunctional CD4+ T-cell response, i.e. CD4+ T cells expressing several cytokines such as IL-2, TNF-α, and IFNγ. The molecular and cellular details underlying the observed synergy between MPL and QS-21 are being further investigated. Preliminary evaluation suggests a key role for early production of IFNγ by innate cells in the draining lymph node (unpublished).
4. AS01 mode of action: application to human vaccines
AS01 was selected in a number of human vaccine candidates because of its association with enhanced humoral and cellular immune responses [Citation5,Citation37,Citation40]. As observed with other adjuvants [Citation7,Citation28], AS01 induces a local and transient activation of the innate-immune system. However, there are specific features of the AS01-triggered innate response that distinguish AS01 from other adjuvants: (1) no depot effect observed; (2) a robust increase of a broad population of activated APCs, including classical DCs as well as monocyte-derived DCs; (3) a synergy between MPL and QS-21 leading to higher antigen-specific response. In addition, AS01 directs the response toward a predominantly IFN-driven pathway which may reinforce the cellular immune response and promote antibody isotype switching. Importantly, cytokine and innate-immune cell responses are largely resolved by day 7, highlighting the transient nature of the AS01-enhanced innate-response at both sites, muscle () and draining lymph node. Detailed characterization of the local innate response to AS01/Ag in mouse model facilitates the interpretation of the high level of adaptive responses detected in human recipients of an AS01-containing vaccine, either a malaria or herpes-zoster candidate. The common pattern to emerge from the clinical experience with AS01-adjuvanted vaccines is that AS01 promotes both antigen-specific antibodies and CD4+ T cells, which can be detected in the peripheral blood. On the other hand, antigen-specific CD8+ T-cell frequencies in the peripheral blood are low and generally not induced by AS01-adjuvanted subunit antigens, in contrast to what is seen in preclinical models.
4.1. Rationale for the selection of AS01 as adjuvant in two candidate vaccines
4.1.1. RTS,S/AS01 malaria vaccine candidate
The first malaria vaccine that used an Adjuvant System was an RTS,S/AS02 candidate formulation, an adjuvant system containing MPL combined with QS-21 in an oil-in-water emulsion [Citation11]. The choice of adjuvant was reconsidered following evidence in larger studies conducted in adult volunteers, and subsequently in children, of improved CD4+ T-cell responses and improved efficacy when AS01 was used instead of AS02 [Citation41–Citation45]. In a large Phase-3 study, efficacy against any malaria due to P. falciparum over 12 months of follow-up after dose 3 was 31.3% in infants 6–12 weeks of age with coadministration of EPI (Expanded Program on Immunization) vaccines and 55.8% in children 5–17 months of age at the time of the first dose [Citation46]. Efficacy waned over time, but a fourth vaccine dose enhanced and extended protection [Citation47]. Given the magnitude of the malaria burden, even the partial prevention of malaria has the potential to provide substantial public health benefits, especially in areas where children can have several disease episodes each year [Citation48].
To date, more than 10,000 children have received the RTS,S/AS01 candidate vaccine [Citation49–Citation51]. RTS,S/AS01 has an acceptable clinical safety profile. In a Phase-3 study, systemic symptoms, including fever, drowsiness, and irritability, were reported more frequently in children who received RTS,S/AS01 than controls who received licensed rabies or meningococcal serogroup C conjugate vaccine [Citation49,Citation52]. Febrile seizures within 7 days of immunization with RTS,S/AS01 occurred in 0.1% of children (rate in 5–17 month olds 1.04/100,000, 95% confidence interval [CI] 0.62–1.64) [Citation49]. In pooled analysis of safety of Phase-2 studies, children who received RTS,S/AS01 had fewer serious adverse events after vaccination than children who received control vaccines [Citation53].
In the Phase-3 study, meningitis (any etiology) was reported more frequently in RTS,S/AS01 recipients than in controls for up to 18 months after vaccination [Citation46]. Meningitis was reported as a serious adverse event in 16/5949 children who received RTS,S/AS01 and 1/2974 children in the control group [Citation46]. No pathogen was identified in 11 of the cases. The cases were not temporally related to vaccination and no biologically plausible explanation is available to link these cases to vaccination. The European Medicine Agency considered that the imbalance is likely to be a chance finding, also because of the low incidence (one single case) in the control group [Citation54].
4.1.2. HZ/su herpes-zoster vaccine candidate
The humoral and cellular response to recombinant gE antigen administered alone, with aluminum salt, with AS02, or with AS01 as adjuvant was investigated in mice that had been primed with the live-attenuated varicella (chicken pox) vaccine. The mice were primed to model the VZV-specific immune status of individuals who could develop herpes zoster [Citation37]. Although all of the vaccines induced anti-gE antibodies and specific CD4+ T-cell responses, the AS01-adjuvanted vaccine induced higher numbers of IFN-γ-producing CD4+ T cells than the other adjuvants, including AS02 [Citation37]. In another study, AS01 induced better T-cell responses than other Adjuvant Systems i.e. AS04 (containing MPL and aluminum) or AS03 (vitamin E and squalene in an oil-in-water emulsion) [Citation38]. AS01 was therefore selected as adjuvant for the candidate herpes-zoster vaccine. A Phase 1/2 trial in adults subsequently demonstrated antigen-specific humoral and CD4 T-cell responses induced by the herpes-zoster-AS01 vaccine that were significantly higher than those induced by unadjuvanted varicella vaccine [Citation23], confirming the suitability of the choice of adjuvant. In a Phase-3 efficacy trial, which included 7700 adult recipients of the vaccine and demonstrated 97.2% (95% CI 93.7–99.0) efficacy in preventing herpes zoster in adults ≥50 years of age [Citation55], the vaccine had a clinically acceptable safety profile. More Hz/su recipients (17%) than placebo recipients (3.2%) reported symptoms of grade 3 intensity (prevented normal daily activities) within 7 days after vaccination. Injection-site reactions and myalgia were the most frequently reported grade 3 symptoms in Hz/su recipients. These events were transient, with a median duration of 1–3 days. Importantly, a similar proportion of HZ/su and placebo recipients reported serious adverse events within 31 days postvaccination (1.1% and 1.3%, respectively) [Citation55]. A parallel trial of a similar size in adults over 70 years of age is ongoing, as are studies in immunocompromised populations.
5. Expert commentary
AS01 is a novel adjuvant combination designed to enhance antigen-specific humoral and cellular responses. This is supported by investigations of its mode of action which showed that the specific activation of the innate response by AS01 induces a broad population of activated APCs and that AS01’s activity to enhance adaptive responses is dependent on synergistic activities of QS-21 and MPL. AS01 is included in the RTS,S/AS01 malaria vaccine which recently received a positive opinion from the European Medicines Agency [Citation56], as well as other vaccines in development including HZ/su and a vaccine against tuberculosis [Citation57]. These vaccines have strikingly different aims: RTS,S/AS01 targets a parasite that causes a substantial global burden in terms of morbidity and mortality, while HZ/su addresses the challenge of VZV latency and reactivation in older age groups or in conditions of immunosuppression. Studies to date confirm the benefits of AS01 in improving the magnitude of the immune response compared with the administration of antigen alone, or with other adjuvants depending on antigens. The available evidence with the RTS,S malaria and zoster candidate vaccines indicates that these AS01-adjuvanted vaccines have a clinically acceptable safety profile, and a positive-benefit–risk ratio, supported by a recent meta-analysis of clinical trials of newly adjuvanted vaccines including AS01 in children, which did not identify any safety concerns [Citation58]. Adjuvants such as AS01 have good prospects for disease control through vaccination, such as in populations with challenging immune statuses and against diseases caused by complex pathogens.
6. Five-year view
The field of adjuvant technology is growing exponentially. Aluminum salt was the only adjuvant used in human vaccines for more than 70 years and continues to be used. The last 20 years has seen six new adjuvants included in licensed vaccines, the first positive opinion for an AS01-containing vaccine, and many other different adjuvants that are under investigation at various stages of development [Citation59]. The next 5 years will see new vaccines containing novel adjuvants, such as the CpG-adjuvanted candidate hepatitis B vaccine, designed to be used against pathogens for which aluminum salt as adjuvant proved insufficient. These new adjuvanted vaccines will aim to provide improved protection to vulnerable populations such as the elderly and immunocompromised. There is little evidence that adjuvants, including AS01, can reliably induce cytotoxic lymphocytes in humans when coadministered with recombinant antigens. This remains an unmet challenge, and the next 5 years is likely to see advances in this area, such as the use of liver vectors to facilitate the induction of cytotoxic responses, in combination or not with adjuvanted approaches. As new products move from clinical trials into widespread use, assessment of their population impact will be possible and comprehensive evaluation of the safety of vaccines containing new adjuvants will continue.
Key issues
Because of their potential to modulate immune responses, novel adjuvants are enabling the development of vaccines for populations and pathogens for which traditional vaccines have been ineffective or have not addressed all the medical needs.
An understanding of the mode of action of an adjuvant can inform on its role in the immunogenicity and reactogenicity profile of a vaccine.
AS01 is a liposome-based adjuvant that contains two immunostimulants (MPL and QS-21). A series of experiments in mice determined that AS01 induces a rapid and transient innate immune response at injected site and the draining lymph node.
AS01 induces an innate-immune response that leads to the activation of a broad range of APCs that are efficient at presenting antigen to activate T cells leading to modulation and enhancement of protective immune response. AS01 activity depends on the synergistic contributions of MPL and QS-21.
Because of its impact on cellular and humoral response, AS01 has been selected for a number of human vaccine candidates
To date more than 10,000 children and 30,000 adults have received AS01-containing vaccines. Comprehensive evaluation of efficacy and safety of AS01-adjuvanted vaccines is ongoing.
Declaration of interest
A Didierlaurent, B Laupèze, A Di Pasquale, N Hergli and C Collignon are employees of the GSK group of companies. N Garçon is a former employee of the GSK group of companies and declared patent ownership of adjuvant systems AS01, 02, 03, 04, 15. A Didierlaurent, A Di Pasquale and N Garçon declared stock ownership in the GSK group of companies. Writing assistance was provided by Joanne Wolter (Independent medical writer on behalf of GSK Vaccines) and editorial and coordination assistance was provided by Regis Azizieh (XPE Pharma & Science on behalf of GSK Vaccines). The authors have no other relevant affiliations or financial involvement with any organization or entity with a financial interest in or financial conflict with the subject matter or materials discussed in the manuscript apart from those disclosed.
Acknowledgments
The authors wish to thank scientists from GSK and collaborators involved in the development and assessment of AS01.
Additional information
Funding
Notes on contributors
Arnaud M. Didierlaurent
All authors participated to the development and the review of the manuscript and approved the final submitted version. Corresponding author had final responsibility to submit for publication. Drafts were developed by a professional publication writer according to the recommendations, documentation, and outline provided by the lead author.
Béatrice Laupèze
All authors participated to the development and the review of the manuscript and approved the final submitted version. Corresponding author had final responsibility to submit for publication. Drafts were developed by a professional publication writer according to the recommendations, documentation, and outline provided by the lead author.
Alberta Di Pasquale
All authors participated to the development and the review of the manuscript and approved the final submitted version. Corresponding author had final responsibility to submit for publication. Drafts were developed by a professional publication writer according to the recommendations, documentation, and outline provided by the lead author.
Nadia Hergli
All authors participated to the development and the review of the manuscript and approved the final submitted version. Corresponding author had final responsibility to submit for publication. Drafts were developed by a professional publication writer according to the recommendations, documentation, and outline provided by the lead author.
Catherine Collignon
All authors participated to the development and the review of the manuscript and approved the final submitted version. Corresponding author had final responsibility to submit for publication. Drafts were developed by a professional publication writer according to the recommendations, documentation, and outline provided by the lead author.
Nathalie Garçon
All authors participated to the development and the review of the manuscript and approved the final submitted version. Corresponding author had final responsibility to submit for publication. Drafts were developed by a professional publication writer according to the recommendations, documentation, and outline provided by the lead author.
References
- Marrack P, McKee AS, Munks MW. Towards an understanding of the adjuvant action of aluminium. Nat Rev Immunol. 2009;9:287–293.
- Wilson-Welder JH, Torres MP, Kipper MJ, et al. Vaccine adjuvants: current challenges and future approaches. J Pharm Sci. 2009;98:1278–1316.
- Reed SG, Orr MT, Fox CB. Key roles of adjuvants in modern vaccines. Nat Med. 2013;19:1597–1608.
- Awate S, Babiuk LA, Mutwiri G. Mechanisms of action of adjuvants. Front Immunol. 2013;4:114.
- Garçon N, Van Mechelen M. Recent clinical experience with vaccines using MPL- and QS-21-containing adjuvant systems. Expert Rev Vaccines. 2011;10;471–486.
- Baldridge JR, McGowan P, Evans JT, et al. Taking a toll on human disease: toll-like receptor 4 agonists as vaccine adjuvants and monotherapeutic agents. Expert Opin Biol Ther. 2004;4:1129–1138.
- Didierlaurent AM, Morel S, Lockman L, et al. AS04, an aluminum salt- and TLR4 agonist-based adjuvant system, induces a transient localized innate immune response leading to enhanced adaptive immunity. J Immunol Baltim Md. 1950 2009;183:6186–6197.
- Casella CR, Mitchell TC. Putting endotoxin to work for us: monophosphoryl lipid A as a safe and effective vaccine adjuvant. Cell Mol Life Sci CMLS. 2008;65:3231–3240.
- Newman MJ, Wu JY, Gardner BH, et al. Induction of cross-reactive cytotoxic T-lymphocyte responses specific for HIV-1 gp120 using saponin adjuvant (QS-21) supplemented subunit vaccine formulations. Vaccine. 1997;15:1001–1007.
- Kashala O, Amador R, Valero MV, et al. Safety, tolerability and immunogenicity of new formulations of the Plasmodium falciparum malaria peptide vaccine SPf66 combined with the immunological adjuvant QS-21. Vaccine. 2002;20:2263–2277.
- Garçon N, Chomez P, Van Mechelen M. GlaxoSmithKline adjuvant systems in vaccines: concepts, achievements and perspectives. Expert Rev Vaccines. 2007;6:723–739.
- Beck Z, Matyas GR, Alving CR. Detection of liposomal cholesterol and monophosphoryl lipid A by QS-21 saponin and Limulus polyphemus amebocyte lysate. Biochim Biophys Acta. 2015;1848:775–780.
- Marty-Roix R, Vladimer GI, Pouliot K, et al. Identification of QS-21 as an Inflammasome-activating molecular component of saponin adjuvants. J Biol Chem. 2016;291:1123–1136.
- World Health Organization. World Malaria Report. 2014. [cited 2015 Jul 31]. Available from: http://www.who.int/malaria/publications/world_malaria_report_2014/en/
- Ferreira MU, Da Silva Nunes M, Wunderlich G. Antigenic diversity and immune evasion by malaria parasites. Clin Diagn Lab Immunol. 2004;11:987–995.
- Morrot A, Rodrigues MM. Tissue signatures influence the activation of intrahepatic CD8(+) T cells against malaria sporozoites. Front Microbiol. 2014;5:440.
- Kawai K, Gebremeskel BG, Acosta CJ. Systematic review of incidence and complications of herpes zoster: towards a global perspective. BMJ Open. 2014;4:e004833.
- Johnson RW, Whitton TL. Management of herpes zoster (shingles) and postherpetic neuralgia. Expert Opin Pharmacother. 2004;5:551–559.
- Levin MJ. Immune senescence and vaccines to prevent herpes zoster in older persons. Curr Opin Immunol. 2012;24:494–500.
- Arvin AM. Humoral and cellular immunity to varicella-zoster virus: an overview. J Infect Dis. 2008;197:S58–S60.
- Burke BL, Steele RW, Beard OW, et al. Immune responses to varicella-zoster in the aged. Arch Intern Med. 1982;142:291–293.
- Kovaiou RD, Herndler-Brandstetter D, Grubeck-Loebenstein B. Age-related changes in immunity: implications for vaccination in the elderly. Expert Rev Mol Med. 2007;9:1–17.
- Leroux-Roels I, Leroux-Roels G, Clement F, et al. A phase 1/2 clinical trial evaluating safety and immunogenicity of a varicella zoster glycoprotein e subunit vaccine candidate in young and older adults. J Infect Dis. 2012;206:1280–1290.
- Arvin AM, Kinney-Thomas E, Shriver K, et al. Immunity to varicella-zoster viral glycoproteins, gp I (gp 90/58) and gp III (gp 118), and to a nonglycosylated protein, p 170. J Immunol Baltim Md. 1950 1986;137:1346–1351.
- Berkowitz EM, Moyle G, Stellbrink H-J, et al. Safety and immunogenicity of an adjuvanted herpes zoster subunit candidate vaccine in HIV-infected adults: a phase 1/2a randomized, placebo-controlled study. J Infect Dis. 2015;211:1279–1287.
- Stadtmauer EA, Sullivan KM, Marty FM, et al. A phase 1/2 study of an adjuvanted varicella-zoster virus subunit vaccine in autologous hematopoietic cell transplant recipients. Blood. 2014;124:2921–2929.
- European Medicines Agency - Human medicines - Zostavax. 2016. [cited 2016 Mar 22]. Available from: http://www.ema.europa.eu/ema/index.jsp?curl=pages/medicines/human/medicines/000674/human_med_001185.jsp&mid=WC0b01ac058001d125
- Coffman RL, Sher A, Seder RA. Vaccine adjuvants: putting innate immunity to work. Immunity. 2010;33;492–503.
- Duthie MS, Windish HP, Fox CB, et al. Use of defined TLR ligands as adjuvants within human vaccines. Immunol Rev. 2011;239:178–196.
- O’Hagan DT, Ott GS, De Gregorio E, et al. The mechanism of action of MF59 - an innately attractive adjuvant formulation. Vaccine. 2012;30:4341–4348.
- Pearse MJ, Drane D. ISCOMATRIX adjuvant: a potent inducer of humoral and cellular immune responses. Vaccine. 2004;22:2391–2395.
- Di Pasquale A, Preiss S, Tavares Da Silva F, et al. Vaccine adjuvants: from 1920 to 2015 and beyond. Vaccines. 2015;3;320–343.
- Morel S, Didierlaurent A, Bourguignon P, et al. Adjuvant system AS03 containing α-tocopherol modulates innate immune response and leads to improved adaptive immunity. Vaccine. 2011;29:2461–2473.
- Didierlaurent AM, Collignon C, Bourguignon P, et al. Enhancement of adaptive immunity by the human vaccine adjuvant AS01 depends on activated dendritic cells. J Immunol. 2014;193:1920–1930.
- Wilson NS, Yang B, Morelli AB, et al. ISCOMATRIX vaccines mediate CD8+ T-cell cross-priming by a MyD88-dependent signaling pathway. Immunol Cell Biol. 2012;90:540–552.
- Mettens P, Dubois PM, Demoitié M-A, et al. Improved T cell responses to Plasmodium falciparum circumsporozoite protein in mice and monkeys induced by a novel formulation of RTS,S vaccine antigen. Vaccine. 2008;26:1072–1082.
- Dendouga N, Fochesato M, Lockman L, et al. Cell-mediated immune responses to a varicella-zoster virus glycoprotein E vaccine using both a TLR agonist and QS21 in mice. Vaccine. 2012;30;3126–3135.
- Fochesato M, Dendouga N, Boxus M. Comparative preclinical evaluation of AS01 versus other adjuvant systems in a candidate herpes zoster glycoprotein E subunit vaccine. Hum Vaccines Immunother. 2016 Mar 2;1–4. [Epub ahead of print]
- Groom JR, Richmond J, Murooka TT, et al. CXCR3 chemokine receptor-ligand interactions in the lymph node optimize CD4+ T helper 1 cell differentiation. Immunity. 2012;37:1091–1103.
- Vandepapelière P, Horsmans Y, Moris P, et al. Vaccine adjuvant systems containing monophosphoryl lipid A and QS21 induce strong and persistent humoral and T cell responses against hepatitis B surface antigen in healthy adult volunteers. Vaccine. 2008;26:1375–1386.
- Olotu A, Moris P, Mwacharo J, et al. Circumsporozoite-specific T cell responses in children vaccinated with RTS,S/AS01E and protection against P falciparum clinical malaria. PloS One. 2011;6:e25786.
- Ansong D, Asante KP, Vekemans J, et al. T cell responses to the RTS,S/AS01(E) and RTS,S/AS02(D) malaria candidate vaccines administered according to different schedules to Ghanaian children. PloS One. 2011;6:e18891.
- Owusu-Agyei S, Ansong D, Asante K, et al. Randomized controlled trial of RTS,S/AS02D and RTS,S/AS01E malaria candidate vaccines given according to different schedules in Ghanaian children. PloS One. 2009;4(10):e7302.
- Kester KE, Cummings JF, Ofori-Anyinam O, et al. Randomized, double-blind, phase 2a trial of falciparum malaria vaccines RTS,S/AS01B and RTS,S/AS02A in malaria-naive adults: safety, efficacy, and immunologic associates of protection. J Infect Dis. 2009;200:337–346.
- Leroux-Roels G, Leroux-Roels I, Clement F, et al. Evaluation of the immune response to RTS,S/AS01 and RTS,S/AS02 adjuvanted vaccines: randomized, double-blind study in malaria-naïve adults. Hum Vaccines Immunother. 2014;10:2211–2219.
- RTS,S Clinical Trials Partnership. Efficacy and safety of the RTS,S/AS01 malaria vaccine during 18 months after vaccination: a phase 3 randomized, controlled trial in children and young infants at 11 African sites. PLoS Med. 2014;11:e1001685.
- RTS,S Clinical Trials Partnership. Efficacy and safety of RTS,S/AS01 malaria vaccine with or without a booster dose in infants and children in Africa: final results of a phase 3, individually randomised, controlled trial. Lancet. 2015;386:31–45.
- Penny MA, Verity R, Bever CA, et al. Public health impact and cost-effectiveness of the RTS,S/AS01 malaria vaccine: a systematic comparison of predictions from four mathematical models. Lancet. 2016;387:367–375.
- RTS,S Clinical Trials Partnership. First results of phase 3 trial of RTS,S/AS01 malaria vaccine in African children. N Engl J Med. 2011;365:1863–1875.
- Lusingu J, Olotu A, Leach A, et al. Safety of the malaria vaccine candidate, RTS,S/AS01E in 5 to 17 month old Kenyan and Tanzanian children. PloS One. 2010;5:e14090.
- Asante KP, Abdulla S, Agnandji S, et al. Safety and efficacy of the RTS,S/AS01E candidate malaria vaccine given with expanded-programme-on-immunisation vaccines: 19 month follow-up of a randomised, open-label, phase 2 trial. Lancet Infect Dis. 2011;11:741–749.
- RTS,S Clinical Trials Partnership. A phase 3 trial of RTS,S/AS01 malaria vaccine in African infants. N Engl J Med. 2012;367:2284–2295.
- Vekemans J, Guerra Y, Lievens M, et al. Pooled analysis of safety data from pediatric Phase II RTS,S/AS malaria candidate vaccine trials. Hum Vaccin. 2011;7:1309–1316.
- European Medicines Agency. Mosquirix H-W-2300. European public assessment report [cited 2016 Mar 22]. Available from: http://www.ema.europa.eu/ema/index.jsp?curl=pages/medicines/document_listing/document_listing_000395.jsp&mid=
- Lal H, Cunningham AL, Godeaux O, et al. Efficacy of an adjuvanted herpes zoster subunit vaccine in older adults. N Engl J Med. 2015;372:2087–2096.
- European Medicines Agency - News and Events - First malaria vaccine receives positive scientific opinion from EMA. [cited 2016 Mar 22]. Available from: http://www.ema.europa.eu/ema/index.jsp?curl=pages/news_and_events/news/2015/07/news_detail_002376.jsp&mid=WC0b01ac058004d5c1
- Penn-Nicholson A, Geldenhuys H, Burny W, et al. Safety and immunogenicity of candidate vaccine M72/AS01E in adolescents in a TB endemic setting. Vaccine. 2015;33:4025–4034.
- Stassijns J, Bollaerts K, Baay M, et al. A systematic review and meta-analysis on the safety of newly adjuvanted vaccines among children. Vaccine. 2016;34;714–722.
- Garçon N, Leroux-Roels G, Cheng W. Vaccine adjuvants. In: Garçon N, Stern PL, Cunningham AL, et al., editors. Understanding modern vaccines: perspectives in vaccinology. Elsevier; 2011. p. 89–113.