ABSTRACT
Introduction: Pneumococcal conjugate vaccine (PCV) impact studies have reported substantial reductions in the incidence of invasive pneumococcal disease (IPD) after implementation of childhood PCV programs. Heterogeneity in surveillance systems, local epidemiology and PCV programs hampers comparisons between studies. We aimed to better understand the impact of childhood PCV programs on overall IPD and serotype distribution.
Areas covered: We analyzed the impact of PCV programs on the incidence of overall IPD, and the distribution of vaccine serotypes (VT) and non-vaccine serotypes (NVT) in children <5 years and adults ≥65 years old. We retrieved datasets from observational post-marketing studies and surveillance reports from countries with high-quality surveillance data available for at least 2 years before PCV program initiation and 3 years after higher-valent PCV implementation. We harmonized pre- and post-PCV analysis periods and assessment methods.
Expert commentary: After introduction of pediatric PCV programs, the residual overall IPD burden in children was low and in a narrow range across countries with high vaccination coverage, irrespective of differences in PCV programs and pneumococcal epidemiology. Effects on overall IPD were more variable in the elderly. Whereas IPD was mainly due to VTs before PCV introduction, NVTs are the major contributor today in children and adults.
1. Introduction
Infectious diseases caused by Streptococcus pneumoniae, such as pneumonia, meningitis, and bacteremia, are a major cause of morbidity and mortality in young children and older adults worldwide [Citation1–Citation4]. Pneumococcal conjugate vaccines (PCVs) were introduced in infant immunization programs to reduce the pneumococcal disease burden in young children. Although at least 97 S. pneumoniae serotypes are known [Citation5], a comparatively small number was responsible for the majority of invasive pneumococcal disease (IPD) before PCV introduction [Citation6,Citation7]. PCVs were designed to protect against the most prevalent IPD-causing serotypes [Citation7–Citation9]. The 7-valent PCV (PCV7, Prevnar/Prevenar, Pfizer), which includes capsular polysaccharides from serotypes 4, 6B, 9V, 14, 18C, 19F, and 23F, was first licensed in 2000 and was replaced by two higher valent PCVs starting in 2008–2009. The pneumococcal non-typeable Haemophilus influenzae protein D conjugate vaccine (PHiD-CV, Synflorix, GSK) contains polysaccharides from serotypes 1, 5, and 7F besides the seven PCV7 types [Citation10,Citation11] and has also demonstrated protection against disease due to the vaccine-related serotype 19A [Citation10,Citation12–Citation14]. The 13-valent PCV (PCV13, Prevnar 13/Prevenar 13, Pfizer) contains polysaccharides from serotypes 3, 6A, and 19A along with the 10 included in PHiD-CV [Citation10,Citation15].
The efficacy/effectiveness of PCVs against pneumonia and IPD in infants and young children has been demonstrated in randomized clinical trials [Citation16–Citation21]. Accordingly, significant drops in nasopharyngeal carriage and IPD caused by vaccine serotypes (VTs) have been observed in the pediatric populations of countries that implemented PCVs in their infant immunization programs [Citation10,Citation22,Citation23]. In addition, in countries with high vaccination coverage, VT IPD in older vaccine-ineligible children and adults declined (herd effect), likely due to reduced transmission of VTs [Citation22,Citation24,Citation25]. The effect of PCVs on the overall disease burden has been somewhat attenuated by an increased incidence of IPD caused by non-vaccine serotypes (NVT) after PCV introduction, owing in part to serotype replacement [Citation22,Citation26–Citation28]. Despite the increased incidence of NVT IPD, the impact of PCVs on overall IPD in young children was still substantial [Citation22,Citation29]. However, in unvaccinated older populations, the effect of PCVs on overall IPD has been less consistent and was completely neutralized by NVT disease in the oldest age groups in some settings [Citation22].
Decisions on the choice of higher valent PCV to be included in a country’s immunization program have often been driven by local pneumococcal epidemiology (serotype distribution) in relation to vaccine serotype content [Citation3,Citation9,Citation30]. However, the full impact of PCVs on pneumococcal disease consists of an intricate balance between their direct effects on disease caused by VTs and vaccine-related serotypes (e.g. 6A and 19A for PCV7 and PHiD-CV), serotype replacement, and herd effect [Citation31]. This means that vaccine impact cannot be precisely defined purely based on serotype content [Citation31,Citation32]. Continuously monitoring disease incidence at the population level through reliable surveillance is paramount to understanding the impact of PCV programs and making informed public health decisions about vaccination schedules (e.g. number of primary doses and the need of a booster), the choice of PCV, and vaccination coverage targets.
In this paper, we assessed the impact of PCV programs on overall, VT, and NVT IPD in children <5 years old and adults ≥65 years old based on publicly available data from observational post-marketing studies and national or regional surveillance reports (Supplemental Figure 1). Substantial heterogeneity exists between countries in the quality and methods of their IPD surveillance and in when surveillance was set up relative to PCV introduction [Citation33,Citation34]. For instance, surveillance may cover all cases in a country’s population (passive or active national population-based surveillance) or may involve a limited network of hospitals or reporting sites (sentinel surveillance); it may cover all ages or selected age groups; case reporting may be mandatory or voluntary and performed by laboratories and/or clinicians; IPD case definitions and laboratory methods used to confirm cases are not standardized across countries; and serotyping may or may not be done. This, together with the diversity in medical practice (e.g. indication for blood culture, use of antibiotics), in local epidemiology, in PCV programs, and in analytical methods used when assessing PCV impact, makes it challenging to interpret and hard to compare results obtained in different observational studies. It is not possible to correct for many of these factors retrospectively; hence, they hamper comparisons between PCV impact studies. Therefore, we developed inclusion and exclusion criteria in an attempt to limit the impact of such confounders on our analyses. As such, we aimed to better understand the impact of childhood PCV programs on the overall IPD burden and the relative contribution of VTs and NVTs, in vaccine-eligible and ineligible populations.
2. Methodology
2.1. Search strategy and selection criteria
To assess the impact of infant PCV programs on IPD in young children and older adults, we retrieved datasets from countries or regions fulfilling the following selection criteria: (1) population of 1000,000 or more, because quantitative evaluation of IPD incidence (which is relatively low) necessitates a population large enough to catch a sufficient number of cases; (2) PCV implemented in the infant immunization program [Citation35]; (3) well-established national or regional population-based or sentinel IPD surveillance system in place for at least 2 years before PCV introduction, with data provided for at least 3 years after higher valent PCV introduction; (4) we restricted our searches to high-income economies (as defined by the World Bank as economies with a gross national income per capita of $12,476 or more [Citation36]), as an indirect marker of the reliability of the data in view of the high resources and stability needed for surveillance ().
Figure 1. Flow chart.
N, number of countries; n, number of citation(s); PCV, pneumococcal conjugate vaccine; PCV7, 7-valent PCV; IPD, invasive pneumococcal disease; VT, vaccine serotype; NVT, non-vaccine serotype. aAs defined by the World Bank as countries with a gross national income per capita of $12,476 or more. bData from surveillance reports used; cNo relevant citations found, but data from surveillance reports used.

We used different approaches to gather datasets from countries/regions fulfilling these criteria. First, we used the outcome of a broad systematic search aimed at identifying observational post-marketing studies that evaluated the impact of PCVs on any pneumococcal disease, nasopharyngeal carriage, antibiotic resistance, and serotype distribution. A detailed account of the search terms and methodology used in this systematic search is included in Supplemental Text 1. In brief, English-language articles and abstracts, published between January 2001 and April 2017, were extracted from EMBASE, Scopus, and Searchlight (a Med’meme-powered database specialized in gray literature). Publications reporting results from randomized controlled trials, observational studies that only had data from either before or after PCV introduction and did not allow for evaluation of vaccine impact at the population level, such as cross-sectional, case series studies, and case reports, studies with only immunogenicity, serotype distribution, or preclinical data, modeling or cost-effectiveness studies, and literature reviews were excluded. For the purpose of the current analyses, we further screened the database resulting from this broad systematic search for studies with IPD as an outcome by using the search terms: invasive pneumococcal disease, IPD, meningitis, and bacteremia. The resulting list of publications was further narrowed down to those with data from high-income countries/regions fulfilling the selection criteria stated earlier. The search was supplemented by consulting bibliographies of the obtained publications and of recently published review articles on the topic, and by screening publicly available material presented at recent conferences. As an additional approach to retrieve the most complete and up-to-date datasets, we reviewed publicly available national, regional, and sentinel IPD surveillance reports from the selected countries.
Publications obtained from the systematic search and public IPD surveillance reports were independently screened by two reviewers against the following inclusion criteria: (1) IPD incidence or number of cases reported for at least 2 years before any PCV introduction and at least 3 years after higher valent PCV introduction, and, where relevant, in the PCV7 period; (2) IPD incidence and/or case counts stratified by age (<5 years old and/or ≥65 years old); (3) IPD incidence and/or case counts segregated by pneumococcal serotype or grouped serotypes (VT and NVT). For comparability, VT IPD was defined as IPD caused by serotypes included in any of the three PCVs, i.e. serotypes 1, 3, 4, 5, 6A, 6B, 7F, 9V, 14, 18C, 19A, 19F, and 23F, regardless of which vaccine was used. NVT IPD was defined as IPD caused by any other serotype. Datasets from which either IPD incidences or case counts were not directly reported and could not be calculated or estimated from the available information were excluded. For countries where population denominators were not reported in the publications or reports, national statistics databases were used to obtain the required information to derive incidences from case counts or vice versa. When multiple sources (publications/reports) were available for one country, we used the one with the most recent and complete dataset. In some cases, data from different sources were combined.
2.2. Data extraction and analysis
Data extraction from the selected publications and reports was performed in parallel by two authors. Pre-PCV, PCV7, and higher valent PCV analysis periods were those defined by the authors for datasets from published articles. For datasets from surveillance reports, analysis periods were defined as follows: (1) the pre-PCV era contained all years with data available; (2) the PCV7 era contained the last 2 years of PCV7 immunization program; (3) the higher valent PCV era included the two most recent years with available data.
Incidences and absolute case counts for overall IPD, VT IPD, NVT IPD, and IPD caused by serotypes 3, 6A, and 19A in each age group (<5 years old and ≥65 years old) in the period before PCV introduction, in the PCV7 period (where applicable), and in the period after higher valent PCV introduction were either extracted directly from the publications/reports or calculated based on available information (e.g. case counts derived from incidences and population denominators or vice versa).
Age groups were chosen as those with the highest IPD burden. As we selected countries with at least 3 years of higher valent PCV use in the national/regional infant immunization schedule, most children under the age of 5 would have been eligible for vaccination and would represent the vaccine target group. Analysis of adults 65 years and older would provide an estimate of herd effect.
For each of the IPD end points (overall IPD, VT IPD, and NVT IPD), we calculated and plotted the average incidence and average number of cases over the different years during each period (pre-PCV, PCV7, and higher valent PCV), per age group and country. Showing both incidences and case counts on the same graphs allowed us to compare the disease burden between countries and analysis periods, while simultaneously providing a measure of the sizes of the individual datasets. Based on the average incidences we obtained, we also calculated the proportions of overall IPD that were due to VT, NVT, or the individual serotypes 3, 6A, and 19A, to assess the relative importance of these serotype groups or individual serotypes to the overall IPD burden in each era.
Due to the low number of datasets included and high variability between studies, it was not possible to perform a meta-analysis. We therefore provide a descriptive analysis of the included studies.
3. Results
3.1. Characteristics of selected countries/regions
Of 45 high-income countries with a population of 1000,000 or more, 29 had a PCV implemented in their infant immunization program and IPD surveillance systems from which comparable data could be retrieved (). For 11 of these countries, we could retrieve datasets that fulfilled our inclusion criteria (). For 3 of these 11 countries, the available data did not allow us to perform our analyses in adults ≥65 years old; for one country, the necessary data were not available for children <5 years of age (). The reasons for exclusion by country are summarized in Supplemental Table 1.
Table 1. Characteristics of the included datasets.
Characteristics of the datasets included in our analyses are shown in . The total duration of the PCV program, calculated from the date of first PCV introduction to the last year with available data included in our analyses, varied across countries from 4 years (Israel) to 15 years (United States). All countries except one (Finland) started universal mass vaccination with PCV7. The time between introduction of PCV7 and the switch to higher valent PCVs varied from 1 year (Israel) to 10 years (United States). Most countries/regions used a prime/boost schedule with either two or three primary doses. Australia had a three-dose priming schedule without booster (except in indigenous populations for whom a booster is scheduled) [Citation41,Citation71]. Nine countries had catchup campaigns with PCV7, three with higher valent PCVs. All countries had 23-valent pneumococcal polysaccharide vaccine (PPV23) available for older adults.
The majority of included countries had nationwide population-based surveillance with mandatory reporting of IPD cases (). For the United States and the Netherlands, data from sentinel surveillance were used [Citation59,Citation72]. For comparison, characteristics of the surveillance systems and vaccination programs from the 18 high-income countries excluded from our analyses are presented in Supplemental Table 1.
3.2. Changes in IPD in children <5 years old
3.2.1. Changes in overall, VT, and NVT IPD
In the countries eligible for our analysis, the overall reported IPD incidence in children <5 years old in the pre-PCV era was highly variable, ranging from 17.1 to 94.7 cases/100,000 child-years, with the highest incidence in North America and lowest incidences in European countries ( and Supplemental Table 2). After PCV introduction, regardless of the size of the dataset, the pre-PCV incidence, schedule, duration of the PCV program, or PCV used, the incidence was lower in all countries and the range became narrower, with 9.6–33.0 and 6.9–18.8 cases/100,000 child-years in the PCV7 and higher valent PCV periods, respectively () and Supplemental Table 2). On average, most of the absolute rate reductions in overall IPD were observed during the PCV7 era in countries where this vaccine was introduced, due to reductions in IPD caused by VTs, while during the higher valent PCV era, an additional smaller absolute reduction in overall IPD was observed. In Finland, which introduced PHiD-CV without prior PCV7 use, the remaining overall IPD burden in the higher valent PCV era seemed to be similar to that seen in countries with sequential PCV7 and higher valent PCV use.
Figure 2. Invasive pneumococcal disease distribution across analyzed countries or regions by PCV era for children <5 years old.
PCV, pneumococcal conjugate vaccine; Overall IPD, invasive pneumococcal disease due to any pneumococcal serotype; VT IPD, IPD caused by serotypes included in any of the three PCVs, i.e. serotypes 1, 3, 4, 5, 6A, 6B, 7F, 9V, 14, 18C, 19A, 19F, and 23F; NVT IPD, IPD caused by any other serotype; PCV7, 7-valent PCV; HV-PCV, higher-valent PCV (PHiD-CV, PCV13). Notes: A bubble represents one country. The size of the bubble indicates the number of cases. The center location of the bubble indicates the incidence of the country for the respective analysis period. Rectangles illustrate the mean incidence across analyzed countries (center of rectangle) ± 1 standard deviation (height of rectangle, calculated based on IPD incidences without considering the absolute numbers of cases).
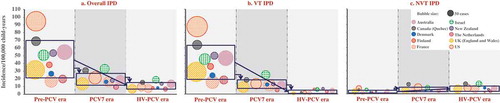
The incidence of VT IPD ranged from 15.4 to 87.4 cases/100,000 child-years across analyzed countries in the pre-PCV era, accounting for 85–94% of the overall disease burden (), , and Supplemental Table 2). Sharp drops in VT IPD incidence were observed after the implementation of PCV7. The residual VT IPD burden of 5.5–24.9 cases/100,000 child-years further declined in the higher valent PCV era to 0.8–7.1 cases/100,000 child-years () and Supplemental Table 2). An increase was observed in NVT IPD in most settings, with 1.6–7.2 cases/100,000 person-years in the pre-PCV period, 3.8–12.4 cases/100,000 person-years in the PCV7 era, and 2.2–12.9 cases/100,000 person-years after higher valent PCV use. After introduction of higher valent PCVs, NVT IPD accounted for 26–90% of the remaining IPD burden (), , and Supplemental Table 2).
Table 2. Proportion of overall IPD attributed to vaccine serotypes, non-vaccine serotypes, and serotypes 3, 6A, and 19A separately by PCV era in children <5 years old.
3.2.2. Changes in IPD caused by serotypes 3, 6A, and 19A
Some serotypes included in higher valent PCVs still remain a cause of disease in children and adults. Therefore, we attempted to analyze these individual serotypes, in particular 3 and 19A. Disease caused by serotype 6A has been largely eliminated following PCV introduction. Furthermore, in most of the countries, serotypes 6A and 6C were not discriminated in the pre-PCV era, precluding us from analyzing the impact of PCVs on 6A. However, in recent years, countries have been reporting 6A separately from 6C; hence, we were able to report on the incidence of 6A IPD in the higher valent PCV era ( and Supplemental Table 2). Two of the 10 countries included in our analyses of children <5 years old (Denmark and the United States) did not have data available for serotypes 3, 6A, and 19A. In the analyzed datasets, the reported incidence of 19A IPD ranged between 1.2 and 5.1 cases/100,000 child-years in the pre-PCV era, between 1.8 and 11.1 cases/100,000 child-years (i.e. 15–45% of the overall IPD burden) in the PCV7 period, and between 0.2 and 3.5 cases/100,000 child-years (i.e. 2–42% of the overall IPD burden) after higher valent PCV introduction (, , and Supplemental Table 2). The incidence of serotype 3 IPD ranged between 0.3 and 0.7 cases/100,000 child-years in the pre-PCV era, between 0.3 and 1.6 cases/100,000 child-years in the PCV7 era, and between 0.1 and 1.8 cases/100,000 child-years (i.e. 1–13% of the overall IPD burden) after higher valent PCV introduction (, , and Supplemental Table 2). Serotype 6A IPD has nearly been eliminated in children <5 years old after higher valent PCV implementation in all countries included in the analysis. 6A cases were only detected in the three countries with the shortest PCV program duration (Israel, Finland, and New Zealand; 0.2–0.6 cases/100,000 child-years) ( and Supplemental Table 2).
Figure 3. Serotype-specific invasive pneumococcal disease incidence and case counts in the higher-valent PCV era in children <5 years old.
PCV, pneumococcal conjugate vaccine; Overall IPD, invasive pneumococcal disease due to any pneumococcal serotype; NVT IPD, IPD caused by any serotype other than those included in PCVs; 3, 6A, 19A IPD, IPD caused by serotypes 3, 6A and 19A, respectively. aData not available for Denmark and US. bFor New Zealand, 6A and 6C combined data.
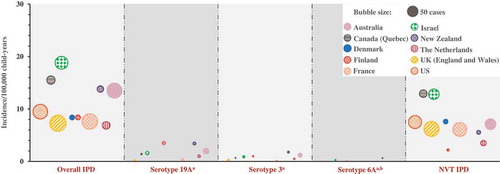
3.3. Changes in IPD in adults ≥65 years old
3.3.1. Changes in overall, VT, and NVT IPD
The reported incidence of overall IPD in adults ≥65 years old also varied greatly between countries in the pre-PCV era, ranging from 27.7 to 75.3 cases/100,000 person-years. This variability was reduced in the higher valent PCV era, where the overall IPD incidence extended from 20.0 to 50.5 cases/100,000 person-years () and Supplemental Table 3). The magnitude of reductions in the incidence of overall IPD between the pre-PCV and higher valent PCV eras, when observed, varied widely () and Supplemental Table 3).
Figure 4. Invasive pneumococcal disease distribution across analyzed countries or regions by PCV era in adults ≥65 years old.
PCV, pneumococcal conjugate vaccine; Overall IPD, invasive pneumococcal disease due to any pneumococcal serotype; VT IPD, IPD caused by serotypes included in any of the three PCVs, i.e. serotypes 1, 3, 4, 5, 6A, 6B, 7F, 9V, 14, 18C, 19A, 19F, and 23F; NVT IPD, overall IPD caused by any other serotype; PCV7, 7-valent PCV; HV-PCV, higher-valent PCV (PHiD-CV, PCV13). Notes: A bubble represents one country. The size of the bubble indicates the number of cases. The center location of the bubble indicates the incidence of the country for the respective analysis period. Rectangles illustrate the mean incidence across analyzed countries (center of rectangle) ± 1 standard deviation (height of rectangle, calculated based on IPD incidences without considering the absolute numbers of cases).
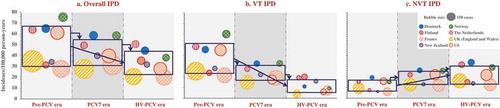
Before PCV introduction, VT IPD accounted for 70–82% of the overall IPD burden (). The incidence of VT IPD was consistently lower in the higher valent PCV era (4.3–20.0 cases/100,000 person-years) than in the pre-PCV and PCV7 eras (21.5–60.1 and 14.9–32.6 cases/100,000 person-years, respectively) () and Supplemental Table 3).
Table 3. Proportion of overall IPD attributed to vaccine serotypes, non-vaccine serotypes, and serotypes 3, 6A, and 19A separately by PCV era in adults ≥65 years old.
The proportion of NVT IPD in the pre-PCV era was higher in the older adult population (18–30%) compared to that in young children (6–10%). NVT IPD increased from the pre-PCV (6.2–19.4 cases/100,000 person-years) to the PCV7 period (12.0–26.1 cases/100,000 person-years) in all countries analyzed that used PCV7. In these countries, further increases were reported in the higher valent PCV era albeit negligible for France, New Zealand, and the United States (range across all analyzed countries: 12.7–33.5 cases/100,000 person-years) () and Supplemental Table 3). After 5–15 years of PCV use, NVTs have become the major contributors to the IPD burden in older adults in most countries included in our analyses, with 43–79% of IPD being caused by NVTs ().
3.3.2. Changes in IPD caused by serotypes 3, 6A, and 19A
Three of the eight countries included in our analyses of adults ≥65 years old (Denmark, Norway, and the United States) did not have data available for the three serotypes included exclusively in PCV13 (3, 6A, and 19A). In adults ≥65 years old, the reported incidence of 19A IPD in the five countries with available data ranged from 1.2 to 2.8 cases/100,000 person-years in the pre-PCV era, from 3.1 to 4.7 cases/100,000 person-years in the PCV7 era, and from 1.1 to 5.5 cases/100,000 person-years in the higher valent PCV era, thereby contributing to 5–14% of the overall IPD burden in the latter period (, , and Supplemental Table 3). The incidence of IPD due to serotype 3 was 2.2–3.7 cases/100,000 person-years in the pre-PCV era and 1.6–6.3 cases/100,000 person-years after 5–11 years of infant PCV immunization, corresponding to 6–18% of the overall IPD burden. As in children, 6A was an infrequent cause of IPD in adults ≥65 years old (0.1–2.0 cases/100,000 person-years; 0–6% of overall IPD) in the higher valent PCV period, with the highest incidence observed in countries with the shortest PCV program duration (Finland and New Zealand) (, , and Supplemental Table 3).
Figure 5. Serotype-specific invasive pneumococcal disease incidence and case counts in the higher-valent PCV era in adults ≥65 years old.
PCV, pneumococcal conjugate vaccine; Overall IPD, invasive pneumococcal disease due to any pneumococcal serotype; NVT IPD, IPD caused by any serotype other than those included in PCVs; 3, 6A, 19A IPD, IPD caused by serotypes 3, 6A and 19A, respectively.; HV-PCV, higher-valent PCV (PHiD-CV, PCV13). aData not available for Denmark, Norway and US. bFor New Zealand, 6A and 6C combined data. Note: Serotypes 19A, 3 and 6A are included in PCV13 and not in PHiD-CV.

4. Discussion
To date, 139 countries have introduced a PCV in their infant immunization program [Citation35]. The effect of PCV programs on the IPD burden has been evaluated in numerous post-marketing observational studies which have shown different degrees of impact, in the vaccinated as well as unvaccinated population. Despite the available information from individual studies, only a few populations worldwide can be used for accurate quantitative evaluation of vaccine impact, since, besides a universal pediatric immunization program, also high vaccination coverage and a high-quality population-based surveillance system are needed for this purpose. In an attempt to eliminate some of the sources of study-to-study heterogeneity in observed PCV impact, we carried out a multi-country analysis focusing on countries with surveillance data available for at least 2 years before a universal PCV program was initiated and 3 years after higher valent PCV implementation, and we harmonized the pre- and post-PCV analysis periods and assessments. By plotting both incidences and case counts on the same graphs, we were able to evaluate changes in the IPD burden while having a measure of the sizes of the individual datasets. We evaluated absolute IPD rates before and after implementation of PCV programs as a measure of success of PCV programs, rather than the more commonly used approach of calculating proportional reductions or incidence rate ratios, because the residual absolute disease burden represents the closest estimate of the overall public health impact of PCV programs.
Our analyses show that, regardless of differences in PCV programs (such as previous PCV7 use, choice of higher valent PCV or immunization schedules), and despite epidemiological differences (such as pre-PCV incidence or serotype distribution), the incidence of overall IPD was strongly reduced and ended up in a consistently low and narrow range across countries in children <5 years old after implementation of childhood PCV immunization programs. Effects on overall IPD were more variable in adults ≥65 years old. A shift was observed in the main cause of disease from VTs to NVTs, in both children and older adults.
The overall impact of higher valent PCVs on IPD reported here is similar to what was shown in a study covering nine surveillance sites from seven countries in Europe [Citation29] and in a recent systematic review in young children in high- and low-income countries [Citation73], which showed that the incidence of IPD in children <5 years old substantially decreased after higher valent PCV introduction, due to a decline in VT IPD, and that the incidence of NVT IPD increased. The observed increase in the burden of NVT IPD is suggestive of serotype replacement [Citation22], and warrants careful monitoring. Similar to our findings in adults ≥65 years old, a systematic review on the indirect effect of PCVs showed variable reductions in overall IPD in older adults across countries [Citation37]. The observed decreases in VT IPD in older adults indicate that higher valent PCVs are effective in inducing herd protection in the unvaccinated population, the duration of which is yet not clear.
Our analyses underscore the previously observed large variation in IPD incidence between countries before introduction of PCVs [Citation22,Citation37,Citation74]. In young children, the highest incidence was observed in the United States and was two to five times higher than in most other countries analyzed. In adults ≥65 years old, the incidence was approximately twice as high in Norway, Denmark, the Netherlands, and the United States than in the other countries analyzed (United Kingdom, New Zealand, Finland, and France). Three- to eightfold higher IPD rates were previously noted in children younger than 6 years in the United States compared to western European countries [Citation74]. Similarly, in a pooled analysis of multiple surveillance sites, the pre-PCV IPD incidence varied greatly across sites, ranging from 4.7 to 280.3 cases/100,000 person-years in children younger than 5 years and from 0.6 to 101.7 cases/100,000 person-years in adults 18 years and older (with the highest incidence in Australian indigenous populations) [Citation22]. Likewise, a recent systematic review on the indirect impact of PCVs on IPD showed that pre-PCV IPD rates in ≥65-year olds ranged from 16 to 80 cases/100,000 person-years in studies that used isolation of S. pneumoniae from sterile body fluids as IPD case definition [Citation37]. The observed diversity in IPD incidence before PCV introduction could be ascribed to real differences in disease incidence between settings due to e.g. differences in the prevalence of IPD risk factors and comorbidities, age distribution, social mixing patterns, or attendance to day care [Citation75]. Alternatively, this variability could be caused by under-reporting of IPD in some countries. As the diagnosis of IPD often relies on blood culture, differences in blood culture practices can have an impact on reported IPD rates [Citation74]. In the United States, blood culture was recommended for all children aged 3–36 months with a fever of at least 39.0°C and white-blood-cell counts of at least 15,000/µL [Citation76]. Therefore, a large proportion of pediatric IPD isolates in studies in the United States come from outpatients, in contrast to Europe where most blood isolates in the pre-PCV era were obtained from hospitalized children [Citation74,Citation75]. In European countries, reported IPD rates in young children may thus be skewed toward more severe manifestations of IPD, such as meningitis, and may under-report mild disease [Citation74,Citation75]. Other differences in clinical practice (such as antimicrobial prescription guidelines), or heterogeneity in reporting requirements or case identification within the individual countries’ surveillance systems may also impact reported IPD rates [Citation34,Citation75,Citation77].
Despite the large disparities in pre-PCV IPD incidence between settings, post-PCV overall IPD rates ended up in a narrow range in young children across the datasets we analyzed. In older adults, post-PCV IPD rates also varied less than those pre-PCV, but the range was wider than what was observed in children. This could in part be explained by the higher heterogeneity of this population (e.g. age distribution, prevalence of IPD risk factors, and comorbidities leading to an increased risk of infection by serotypes with less disease potential [NVTs]). Another explanation may be that the higher remaining IPD burden in this nontarget population may allow for factors related to local clinical practice and surveillance systems to influence the absolute rate of IPD. All countries included in our analysis had PPV23 available for older adults. Little information is available on PPV23 uptake in most countries which makes it hard to assess if PPV23 may have contributed to the observed varying reductions in IPD rates, especially given the overlap between serotypes included in PCVs and those included in PPV23.
Our analyses highlight that whereas before PCV implementation IPD was mostly dominated by VTs, NVTs have become a major contributor to the overall IPD burden in the higher valent PCV era in most countries. This is in line with previous reports. In a systematic review that estimated the global and regional distributions of serotypes causing IPD in children <5 years old, VTs accounted for 74–88% of IPD in the pre-PCV era globally [Citation6]. A study that analyzed IPD isolates from 50 countries worldwide showed that 61% of IPD in adults ≥65 years old was caused by VTs prior to higher valent PCV introduction compared to 50% in the higher valent PCV era [Citation78]. In a recent review that examined the relative importance of NVTs after higher valent PCV implementation, 40–86% of all IPD in <5-year olds and 57–84% of all IPD in adults ≥65 years old were caused by NVTs in PCV13 and PHiD-CV using countries. An important difference is that the authors of this review considered serotype 3 as a NVT [Citation79]. Similarly, another recent systematic review and meta-analysis that assessed serotype distribution in childhood IPD in the post-PCV era showed that in countries that had introduced higher valent PCVs, NVTs contributed to 42.2% (95% confidence interval [CI]: 36.1–49.5%) of childhood IPD [Citation80]. However, large regional differences were observed; in European countries, the post-higher-valent PCV proportion of NVT IPD was 71.9% (95% CI: 63.1–82.0%) compared to 57.8% (95% CI: 41.6–80.4%) in North America and 28.5% (95% CI: 23.4–34.7%) in Latin America [Citation80]. Most of the data from Latin American countries included in this meta-analysis represented the transition year or first years after higher valent PCV introduction in contrast to the European datasets which also represented later years. This may in part explain these regional differences.
A clear benefit of higher valent PCVs compared to PCV7 was apparent in the datasets we analyzed, as supported by the additional decreases in overall IPD observed in the higher valent PCV era compared to the PCV7 era in young children as well as in older adults. The number of datasets included in our analyses is too small to make comparisons between the two higher valent PCVs currently in use; only three countries in our adult datasets and only four countries in our children datasets used PHiD-CV, of which one had no prior PCV7 use and two switched to PCV13. Moreover, numerous other factors differ between PCV13 and PHiD-CV using countries, including the total duration of the PCV program (5–10 years for PHiD-CV or PHiD-CV + PCV13 using countries; 4–15 years for PCV13 using countries), immunization schedules (2 + 1, 3 + 1, 3 + 0), the implementation of a catchup campaign or not, the type of surveillance, the pre-PCV IPD incidence, and serotype distribution. With these caveats in mind, we did note that in young children, regardless of higher valent PCV used, the overall IPD rates were in a narrow range in the higher valent PCV era, suggesting that there was no dominant effect of the choice of vaccine. Similarly, in adults 65 years and older, no clear pattern could be discerned in overall IPD rates in the higher valent PCV era in terms of which higher valent PCV was used. We cannot exclude that a differential effect of these two vaccines exists but that this may have been overruled by other, more impactful, differences between countries or may become apparent upon analysis of a higher number of datasets. A recent review on the impact and effectiveness of PHiD-CV and PCV13 in children younger than 5 years of age in Latin American countries showed no evidence of one higher valent PCV being superior over the other in terms of impact on hospitalizations for pneumonia, IPD, or meningitis [Citation81]. A population-based cohort study in Sweden directly compared the impact of PCV13 and PHiD-CV on IPD and found no difference between the vaccines in their effect on overall IPD [Citation82]. Sweden represents a unique opportunity to compare the two higher valent vaccines in equivalent populations as the different counties can independently choose which vaccine they use. A recent report with a global assessment of PCVs also concluded that both vaccines have shown a similar disease impact following routine use [Citation10]. PCV13 and PHiD-CV differ not only in the number of serotypes for which capsular polysaccharides are included as antigens but also in carrier proteins and conjugation methods. PCV13 includes serotype 3, 6A, and 19A capsular polysaccharides, whereas PHiD-CV does not. However, vaccine effectiveness of PHiD-CV has been observed against 19A IPD [Citation12–Citation14] and 6A IPD [Citation83], possibly due to cross-reactivity by functional antibodies induced by the VTs 19F and 6B. Indeed, PHiD-CV induced functional (opsonophagocytic) antibodies to 19A and 6A after primary and booster vaccination [Citation84–Citation90]. In comparative studies between PHiD-CV and PCV7 (for which limited or no protection against 19A disease has been observed) [Citation91], 19A opsonophagocytic activity responses were substantially higher after PHiD-CV than after PCV7 vaccination [Citation84–Citation90]. It has been hypothesized that this may be due to the different conjugation methods used for 19F in the two vaccines [Citation92]. PHiD-CV has no impact on serotype 3 disease while effectiveness of PCV13 against serotype 3 disease has been observed in some but not other studies [Citation93–Citation96]. Serotype-specific information was available for eight children and five adult datasets. In all of these, 6A IPD was very rare after higher valent PCV introduction. Serotype 19A accounted for 2–42% and 5–14% of IPD in the higher valent PCV era in children <5 years old and adults ≥65 years old, respectively. Serotype 3 contributed to 1–13% of overall IPD in children <5 years old and 6–18% in adults ≥65 years old. In line with these observations, a recent systematic review and meta-analysis of post-PCV serotype distribution showed that serotypes 19A and 3 accounted for 14.2% (95% CI: 11.1–18.3%) and 5.3% (95% CI: 4.2–6.7%), respectively, of childhood IPD cases globally [Citation80]. Strong regional variations were seen in these proportions. In another review that assessed serotype distribution in IPD after introduction of higher valent PCVs, serotypes 19A and 3 were also shown to remain a prominent cause of IPD in all age groups in most PHiD-CV and PCV13 using countries [Citation79]. Interestingly, in Finland, the only country included in our analysis which introduced a higher valent PCV (PHiD-CV) without prior PCV7 program, the incidence of 19A IPD increased first in the elderly population. Increases in 19A disease have been reported for several countries using either of the three PCVs (e.g. also recently in the United Kingdom after PCV13 use) [Citation97,Citation98]. Previous publications have shown that factors other than PCV7 introduction may have contributed to the reported rises in 19A disease in the PCV7 era, including antibiotic pressure causing the spread of resistant clones or secular trends, as supported by increases in 19A incidence observed in countries before PCV7 introduction [Citation99].
Close monitoring will be required to evaluate if with increasing years of higher valent PCV use, the 19A IPD burden will further decrease. Protection against serotype 3 disease may require a different approach because of the increasing rates of serotype 3 disease and the inconsistent pattern of protection provided by PCV13.
This review has several limitations. First, our literature search was restricted to articles published in English, and our search terms and criteria may not have been sufficient to identify all relevant articles. However, we supplemented our literature search with publicly available surveillance reports and by screening bibliographies of the obtained articles and recent literature reviews.
Second, as we relied on data from published articles and public surveillance reports, our analyses were subject to factors that confound observational studies. A reliable estimate of the impact of a vaccination program by comparing pre- and postvaccination populations requires that all factors, other than the intervention, remain constant over time in the population. However, in many settings, this assumption does not hold in the context of PCV impact studies [Citation77,Citation100]. The baseline risk of disease may change over time due to vaccine-independent secular trends in serotype-specific IPD incidence. In addition, surveillance methods may differ and become more sensitive upon introduction of a vaccine in the national immunization schedule (e.g. voluntary vs. mandatory reporting, changes in case definitions). Similarly, laboratory methods and medical practices (e.g. antibiotic use, blood culture practice, vaccination against other diseases) may evolve with time. Finally, population characteristics such as age distribution, frequency of underlying IPD risk factors and comorbidities, and social mixing patterns may change over time. All of these factors may influence the observed IPD incidence and thereby confound the assessment of vaccine impact [Citation34,Citation77,Citation100]. The same factors can also contribute to setting-to-setting variability. We tried to limit the impact of some of these confounders by focusing our analyses on countries or regions with a well-established surveillance system in place before PCV implementation.
The analytical methods used in observational studies can also influence observed vaccine impact and cause setting-to-setting variation [Citation75,Citation100,Citation101]. In particular, the choice of pre- and postvaccination periods is an important variable. Where possible, we harmonized analysis periods across datasets by using as many pre-PCV years as available (to even out year-to-year fluctuations in IPD rates) and by using the last 2 years of PCV7 or higher valent PCV use as post-PCV periods, from countries with at least 3 years of post-higher-valent PCV data available. We thereby avoided using the transition year and first year(s) after PCV introduction during which vaccine impact, and in particular herd effect, is usually sub-optimal and strongly depends on the speed of vaccine uptake [Citation22]. In the countries included in our analyses, vaccination coverage was around 90% or higher in the higher valent PCV periods we used and was therefore likely not a factor contributing to study-to-study variation. Due to the heterogeneity between datasets, we could not perform a meta-analysis, and all our analyses were descriptive.
In our analyses, we used case counts or incidences as available in the published articles and surveillance reports, and in some instances, we had to estimate these from graphs or calculate them based on serotype distribution. We did not apply any corrections for possible preexisting trends. In some of the datasets, this was, however, done by the authors of the original publications or reports.
A third limitation is the small number of datasets included in our analyses, due to the stringent inclusion criteria we applied to select high-quality data. Because of the resources needed and constraints in infrastructure, low- and middle-income countries may have major hurdles to develop and maintain high-quality disease surveillance [Citation33,Citation102,Citation103]. Therefore, our work was conducted on data from high-income countries and may not be directly applicable to low or middle-income countries, which generally have the highest IPD burden. Nevertheless, a few studies in middle- and low-income countries that have established surveillance programs with standardized methods, such as South Africa and the Gambia, have shown similar results. Among HIV-uninfected children younger than 2 years of age in South Africa, the overall IPD incidence decreased from 28.6 cases/100,000 population in the pre-PCV era to 14.5 cases/100,000 population in the year after PCV13 introduction, driven by a sharp drop in VT IPD and in part counteracted by an increase in NVT IPD [Citation104]. A population-based surveillance study in the Gambia showed a decline in overall IPD incidence from 253 to 113 cases per 100,000 population in children 2–23 months of age between the pre-PCV baseline and 2–3 years after routine PCV13 use, again with increases in NVT IPD observed over this period [Citation105]. A recently published systematic review on the impact of higher valent PCVs on pediatric IPD and pneumonia in high and non-high income countries confirmed that the same conclusions applied to both sets of countries; the observed decline in overall pneumococcal disease is driven by the disappearance of most VTs and attenuated in part by increases in NVTs [Citation73].
In this review, we focused solely on IPD, which can be regarded as another limitation of our work. To understand the full overall health benefit of PCV programs, it is important to also evaluate their impact on pneumonia, as this is the most common clinical syndrome caused by S. pneumoniae, particularly in young children and older adults. Analysis of the impact of PCV programs on pneumonia would require a different approach since different case definitions are used and etiology is not always established across studies.
5. Expert commentary
PCVs have been a remarkable public health success. Introduction of the vaccines in the pediatric schedules in high-income countries has had a major impact on pediatric IPD. Furthermore, additional benefits from herd protection were reported in unvaccinated populations, although to a lesser and more variable extent than the direct effect. The World Health Organization recommends that PCVs should be included in routine infant immunization programs globally, especially in countries with high childhood mortality [Citation3]. Reports are indicating a similar impact of PCVs on IPD in these countries as compared to high-income countries. Particular to pneumococcal epidemiology is the rapid replacement by NVTs. This remains a main concern and the long-term effects of PCVs are yet unknown. Continuing development of pneumococcal vaccines that can cover emerging NVTs might be needed. In high-income countries, after at least 3 years of higher valent PCV use in their universal infant immunization programs, the incidence of overall IPD in children younger than 5 years old was low and in a narrow range across countries irrespective of PCV programs and epidemiology of pneumococcal disease. VTs have dropped dramatically in young children. Whereas VTs were the major cause of disease before vaccine introduction, NVTs have become a major cause of the residual IPD burden in most countries analyzed. Similarly, in adults ≥65 years old, NVTs have become a leading cause of IPD in the higher valent PCV era.
The reported increases in NVT IPD warrant careful monitoring, and factors influencing the increase in NVTs need to be thoroughly investigated. Therefore, efforts should be made to improve national surveillance systems and reduce the currently existing heterogeneity between countries that confounds interpretation of PCV impact data and hampers comparisons between countries. Continuing surveillance in children and other age groups, including the elderly, founded on rigorous and standardized methods is required to determine the long-term effects of higher valent PCV programs in terms of direct impact, herd effect, and replacement disease.
There are indications to suggest that the current higher valent PCVs have achieved the purpose for which they were developed. Large reductions of IPD have been recorded after their introduction, irrespective of vaccine used. But there are also signs of increased NVT disease especially in older adults, which may require a different approach. The long-term impact of PCVs may depend on the emergence of a steady state balance between reducing VTs and increasing NVTs, which will ultimately depend on the disease-causing potential of replacement NVTs. It is yet unclear how long it will take to reach this point of equilibrium, and for how long it can be maintained. What constitutes a steady state may also differ by region given the diversity in NVTs with potential implications for the long-term use of current vaccines and the design of future formulations. Current PCVs have removed most of the more virulent serotypes from the nasopharynx leaving a niche for colonization by serotypes that are less virulent. Moreover, the spectrum and impact of other microorganisms within the nasopharyngeal microbiome remain to be further elucidated. Continuing availability of postvaccination surveillance data will be required to elucidate this further. While access to PCVs has increased due to laudable efforts of GAVI, the Vaccine Alliance, and their partners, uptake and coverage of these life-saving vaccines are still relatively low in middle-income settings, especially in countries in Asia. Widespread introduction of the PCVs should be encouraged in order to attain their full benefits.
6. Five-year view
Our analyses suggest that further decreases in the overall IPD burden in young children are unlikely to occur with the currently available pneumococcal vaccines in countries with a high vaccine uptake and mature PCV program. The question remains if further benefits of higher valent PCVs can be expected in older adults. Our results also indicate that adding more serotypes to novel PCVs may only have a temporary beneficial effect and that alternative strategies such as protein-based vaccines that provide serotype-independent protection remain important to pursue. It is however possible that a threshold may be reached such that the remaining serotypes are significantly less virulent and therefore of importance only in highly vulnerable populations. Development of reliable surveillance systems to record the incidence and serotype distribution of IPD is of crucial importance for evaluating the long-term impact of PCV programs and developing new vaccines. A summary of the manuscript intended to highlight the importance of the findings for health-care providers has been included in the supplementary material (Supplemental Figure 1).
Key issues
Pneumococcal infections are a major cause of morbidity and mortality in young children and older adults worldwide.
The incidence of overall IPD was strongly reduced and ended up in a consistently low and narrow range across countries in children <5 years old after implementation of childhood PCV programs.
Effects on overall IPD were more variable in adults ≥65 years old.
The main cause of IPD has shifted from vaccine serotypes to non-vaccine serotypes in both young children and older adults after implementation of pediatric PCV programs.
Continuing surveillance in children and other age groups, including the elderly, is required to determine the long-term effects of higher-valent PCV program.
Declaration of interest
All authors are employed by the GSK group of companies. P Izurieta, R Adegbola, C Clarke and B Hoet hold shares in the GSK group of companies. The authors have no other relevant affiliations or financial involvement with any organization or entity with a financial interest in or financial conflict with the subject matter or materials discussed in the manuscript apart from those disclosed. A reviewer on this manuscript has been on advisory boards for PCV for Pfizer, GSK and Merck.
Trademarks
Synflorix is a trademark of the GSK group of companies. Prevnar/Prevenar and Prevnar 13/Prevenar 13 are trademarks of Wyeth/Pfizer Inc.
Supplemental Material
Download MS Word (285.3 KB)Acknowledgements
The authors would like to thank Lamine Soumahoro, Marcus Nordgren, Muriel Carcano (GSK) for help with the data collection and analysis and Ekaterina Ivanova, Natalie Denef and Ioana Cristina Ilea (XPE Pharma & Science c/o GSK) for manuscript writing and coordination.
Supplemental data
Supplemental data for this article can be accessed here.
Additional information
Funding
References
- O’Brien KL, Wolfson LJ, Watt JP, et al. Burden of disease caused by Streptococcus pneumoniae in children younger than 5 years: global estimates. Lancet. 2009;374:893–902.
- Russell F, Sanderson C, Temple B, et al. Global review of the distribution of pneumococcal disease by age and region. World Health Organization; 2011. [Cited 2017 Jul 26] Available from: http://www.who.int/immunization/sage/6_Russel_review_age_specific_epidemiology_PCV_schedules_session_nov11.pdf
- WHO. Pneumococcal vaccines WHO position paper–2012. Wkly Epidemiol Rec. 2012;87:129–144. It is an evidence based document which provides guidance on the use of PCVs worldwide.
- Drijkoningen JJ, Rohde GG. Pneumococcal infection in adults: burden of disease. Clin Microbiol Infect. 2014;20:45–51.
- Geno KA, Gilbert GL, Song JY, et al. Pneumococcal capsules and their types: past, present, and future. Clin Microbiol Rev. 2015;28:871–899.
- Johnson HL, Deloria-Knoll M, Levine OS, et al. Systematic evaluation of serotypes causing invasive pneumococcal disease among children under five: the pneumococcal global serotype project. PLoS Med. 2010;7.
- Hausdorff WP, Bryant J, Paradiso PR, et al. Which pneumococcal serogroups cause the most invasive disease: implications for conjugate vaccine formulation and use, part I. Clin Infect Dis. 2000;30:100–121.
- Hausdorff WP, Bryant J, Kloek C, et al. The contribution of specific pneumococcal serogroups to different disease manifestations: implications for conjugate vaccine formulation and use, part II. Clin Infect Dis. 2000;30:122–140.
- Cherian T. WHO expert consultation on serotype composition of pneumococcal conjugate vaccines for use in resource-poor developing countries, 26–27 October 2006, Geneva. Vaccine. 2007;25:6557–6564.
- Cohen O, Knoll M, O’Brien K, et al. Pneumococcal conjugate vaccine (PCV) product assessment. 2017. [Cited 2017 Jul 26]. Available from: https://www.jhsph.edu/research/centers-and-institutes/ivac/resources/pcv-product-assessment-april-25-2017.pdf
- Prymula R, Schuerman L. 10-valent pneumococcal nontypeable Haemophilus influenzae PD conjugate vaccine: synflorix. Expert Rev Vaccines. 2009;8:1479–1500.
- Deceuninck G, De Serres G, Boulianne N, et al. Effectiveness of three pneumococcal conjugate vaccines to prevent invasive pneumococcal disease in Quebec, Canada. Vaccine. 2015;33:2684–2689.
- Domingues CMAS, Verani JR, Montenegro Renoiner EI, et al. Effectiveness of ten-valent pneumococcal conjugate vaccine against invasive pneumococcal disease in Brazil: a matched case-control study. Lancet Respir Med. 2014;2:464–471.
- Verani JR, Domingues CM, de Moraes JC, et al. Indirect cohort analysis of 10-valent pneumococcal conjugate vaccine effectiveness against vaccine-type and vaccine-related invasive pneumococcal disease. Vaccine. 2015;33:6145–6148.
- Grijalva CG, Pelton SI. A second-generation pneumococcal conjugate vaccine for prevention of pneumococcal diseases in children. Curr Opin Pediatr. 2011;23:98–104.
- Palmu AA, Jokinen J, Borys D, et al. Effectiveness of the ten-valent pneumococcal Haemophilus influenzae protein D conjugate vaccine (PHiD-CV10) against invasive pneumococcal disease: a cluster randomised trial. Lancet. 2013;381:214–222.
- Tregnaghi MW, Saez-Llorens X, Lopez P, et al. Efficacy of pneumococcal nontypable Haemophilus influenzae protein D conjugate vaccine (PHiD-CV) in young Latin American children: a double-blind randomized controlled trial. PLoS Med. 2014;11:e1001657.
- Black S, Shinefield H, Fireman B, et al. Efficacy, safety and immunogenicity of heptavalent pneumococcal conjugate vaccine in children. Northern California Kaiser Permanente Vaccine Study Center Group. Pediatr Infect Dis J. 2000;19:187–195.
- O’Brien KL, Moulton LH, Reid R, et al. Efficacy and safety of seven-valent conjugate pneumococcal vaccine in American Indian children: group randomised trial. Lancet. 2003;362:355–361.
- Klugman KP, Madhi SA, Huebner RE, et al. A trial of a 9-valent pneumococcal conjugate vaccine in children with and those without HIV infection. N Engl J Med. 2003;349:1341–1348.
- Cutts FT, Zaman SM, Enwere G, et al. Efficacy of nine-valent pneumococcal conjugate vaccine against pneumonia and invasive pneumococcal disease in The Gambia: randomised, double-blind, placebo-controlled trial. Lancet. 2005;365:1139–1146.
- Feikin DR, Kagucia EW, Loo JD, et al. Serotype-specific changes in invasive pneumococcal disease after pneumococcal conjugate vaccine introduction: a pooled analysis of multiple surveillance sites. PLoS Med. 2013;10:e1001517.
- Conklin L, Loo JD, Kirk J, et al. Systematic review of the effect of pneumococcal conjugate vaccine dosing schedules on vaccine-type invasive pneumococcal disease among young children. Pediatr Infect Dis J. 2014;33:S109–18.
- Davis SM, Deloria-Knoll M, Kassa HT, et al. Impact of pneumococcal conjugate vaccines on nasopharyngeal carriage and invasive disease among unvaccinated people: review of evidence on indirect effects. Vaccine. 2013;32:133–145.
- Loo JD, Conklin L, Fleming-Dutra KE, et al. Systematic review of the indirect effect of pneumococcal conjugate vaccine dosing schedules on pneumococcal disease and colonization. Pediatr Infect Dis J. 2014;33:S161–71.
- Weinberger DM, Malley R, Lipsitch M. Serotype replacement in disease after pneumococcal vaccination. Lancet. 2011;378:1962–1973.
- Galanis I, Lindstrand A, Darenberg J, et al. Effects of PCV7 and PCV13 on invasive pneumococcal disease and carriage in Stockholm, Sweden. Eur Respir J. 2016;47:1208–1218.
- Pilishvili T, Lexau C, Farley MM, et al. Sustained reductions in invasive pneumococcal disease in the era of conjugate vaccine. J Infect Dis. 2010;201:32–41.
- Savulescu C, Krizova P, Lepoutre A, et al. Effect of high-valency pneumococcal conjugate vaccines on invasive pneumococcal disease in children in SpIDnet countries: an observational multicentre study. Lancet Respir Med. 2017;5:648–656.
- GAVI’s PneumoADIP. Pneumococcal regional serotype distribution for pneumococcal AMC TPP: codebook to assess whether a pneumococcal vaccine meets the pneumococcal AMC target product profile for regional vaccine serotype coverage. 2008. [Cited 2017 Jul 26]. Available from: http://www.vaccineamc.org/files/TPP_codebook.pdf
- Hausdorff WP, Hoet B, Adegbola RA. Predicting the impact of new pneumococcal conjugate vaccines: serotype composition is not enough. Expert Rev Vaccines. 2015;14:413–428.
- Izurieta P, Clarke C, Mungall B, et al. Is there a correlation between the serotype content of different pneumococcal conjugate vaccines and their clinical impact? 8th Asian Congress of Pediatric Infectious Diseases; 2016 Nov 7–10; Bangkok, Thailand
- Murray J, Agocs M, Serhan F, et al. Global invasive bacterial vaccine-preventable diseases surveillance–2008–2014. MMWR Morb Mortal Wkly Rep. 2014;63:1159–1162.
- Hanquet G, Perrocheau A, Kissling E, et al. Surveillance of invasive pneumococcal disease in 30 EU countries: towards a European system? Vaccine. 2010;28:3920–3928.
- VIEW-hub [Internet]. PCV: Current vaccine intro status; [cited 2017 Jul 26]. Available from: http://view-hub.org/viz/
- The World Bank [Internet]. GNI per capita ranking, Atlas method and PPP based; 2016 [cited 2017 Jul 26]. Available from: http://data.worldbank.org/data-catalog/GNI-per-capita-Atlas-and-PPP-table
- Tsaban G, Ben-Shimol S. Indirect (herd) protection, following pneumococcal conjugated vaccines introduction: a systematic review of the literature. Vaccine. 2017;35:2882–2891.
- Castiglia P. Recommendations for pneumococcal immunization outside routine childhood immunization programs in Western Europe. Adv Ther. 2014;31:1011–1044.
- Ministry of Health. Immunisation Handbook 2014. New Zealand Government. Wellington: Ministry of Health; 2014. [Cited 2017 Jul 26]. Available from: http://www.moh.govt.nz/notebook/nbbooks.nsf/0/5B75258BCFDED7CACC257CF4007CE5B8/$file/immunisation-handbook-may14-v2.pdf
- NNDSS. Invasive pneumococcal disease surveillance, IPD quarterly reports. Australian Government, Department of Health; 2016. [Cited 2017 Jul 26]. Available from: http://www.health.gov.au/internet/main/publishing.nsf/Content/2016+issues-1
- The Australian immunisation handbook. 10th ed. Australian Government. Department of Health; 2017. [Cited 2017 Jul 26]. Available from: http://www.health.gov.au/internet/immunise/publishing.nsf/Content/Handbook10-home~handbook10part4~handbook10-4-13#4-13-4
- De Wals P, Lefebvre B, Markowski F, et al. Impact of 2+1 pneumococcal conjugate vaccine program in the province of Quebec, Canada. Vaccine. 2014;32:1501–1506.
- INSPQ. Programme de surveillance du pneumocoque rapport 2014. Institut national de santé publique du Québec; 2016. [Cited 2017 Jul 26]. Available from: https://www.inspq.qc.ca/publications/2081
- Harboe ZB, Espenhain L, Dalby T, et al. Effects of pneumococcal conjugate vaccination eight years after its implementation in Denmark. Nordic Vaccine Meeting; 2016 Apr 28–29; Reykjavik, Iceland
- Statistics Denmark [Internet]. StatBank Denmark: Population and elections 2000–2015; [cited 2017 Apr 26]. Available from: http://www.statbank.dk/10021
- THL, 2004–2015 [Internet]. [Cited 2017 Jul 26]. Available from: https://www.thl.fi/en/web/thlfi-en/research-and-expertwork/projects-and-programmes/monitoring-the-population-effectiveness-of-pneumococcal-conjugate-vaccination-in-the-finnish-national-vaccination-programme/incidence-of-invasive-pneumococcal-disease-in-finland
- Lepoutre A, Varon E, Georges S, et al. Impact of the pneumococcal conjugate vaccines on invasive pneumococcal disease in France, 2001–2012. Vaccine. 2015;33:359–366.
- Varon E, Claire J, Laurent G. Rapport d’activité 2013: epidémiologie 2012. Centre National de Référence des Pneumocoques; 2013. [Cited 2017 Jul 26]. Available from: http://cnr-pneumo.com/docs/rapports/CNRP2013.pdf
- Varon E, Janoir C, Gutmann L. Rapport d’activité 2014: epidémiologie 2013. Centre National de Référence des Pneumocoques; 2014. [Cited 2017 Jul 26]. Available from: http://cnr-pneumo.com/docs/rapports/CNRP2014.pdf
- Ben-Shimol S, Greenberg D, Givon-Lavi N, et al. Early impact of sequential introduction of 7-valent and 13-valent pneumococcal conjugate vaccine on IPD in Israeli children <5 years: an active prospective nationwide surveillance. Vaccine. 2014;32:3452–3459.
- ESR. Invasive pneumococcal disease in New Zealand, 2013. Institute of Environmental Science and Research Ltd (ESR); 2014. [Cited 2017 Jul 26]. Available from: https://surv.esr.cri.nz/PDF_surveillance/IPD/2013/2013AnnualIPDRpt.pdf
- ESR. Invasive pneumococcal disease in New Zealand, 2014. Institute of Environmental Science and Research Ltd (ESR); 2016. [Cited 2017 Jul 26]. Available from: https://surv.esr.cri.nz/PDF_surveillance/IPD/2014/2014IPDAnnualReport.pdf
- Ali Borman, Helen Heffernan. Invasive pneumococcal disease quarterly report October–December 2015. Institute of Environmental Science and Research Ltd (ESR); 2016. [Cited 2017 Jul 26]. Available from: https://surv.esr.cri.nz/PDF_surveillance/IPD/2015/2015Q4_IPDReport.pdf
- Bergsaker M Impact of childhood pneumococcal vaccination in Norway. Nordic Vaccine Meeting; 2016 Apr 28–29; Reykjavik, Iceland
- Statistics Norway [Internet]. Population: 2004–2015; [cited 2017 Apr 26]. Available from: http://www.ssb.no/en
- Norwegian Institute of Public Health [Internet]. Folkehelseinstituttet SYSVAK; 2014 [cited 2017 Jul 26]. Available from: https://www.fhi.no/globalassets/migrering/dokumenter/pdf/fylker-2-aringer-2012.pdf
- Norwegian Institute of Public Health [Internet]. Folkehelseinstituttet SYSVAK; 2015 [cited 2017 Jul 26]. Available from: https://www.fhi.no/globalassets/2_ar_til_publisering.pdf
- Steens A, Bergsaker MA, Aaberge IS, et al. Prompt effect of replacing the 7-valent pneumococcal conjugate vaccine with the 13-valent vaccine on the epidemiology of invasive pneumococcal disease in Norway. Vaccine. 2013;31:6232–6238.
- Knol MJ, Sanders EAM, De Melker HE, et al. Incidence of IPD in the Netherlands up to five years after introduction of PCV10. Poster session presented at: burden of pneumococcal disease: global epidemiology post-PCV. 10th International Symposium on Pneumococci & Pneumococcal Diseases; 2016 Jun 26–30; Glasgow, Scotland
- Statistics Netherlands. Population 2000–2015 [Internet]. [Cited 2017 Jul 26]. Available from: http://statline.cbs.nl/Statweb/?LA=en
- Waight PA, Andrews NJ, Ladhani SN, et al. Effect of the 13-valent pneumococcal conjugate vaccine on invasive pneumococcal disease in England and Wales 4 years after its introduction: an observational cohort study. Lancet Infect Dis. 2015;15:535–543.
- Miller E, Andrews NJ, Waight PA, et al. Herd immunity and serotype replacement 4 years after seven-valent pneumococcal conjugate vaccination in England and Wales: an observational cohort study. Lancet Infect Dis. 2011;11:760–768.
- Active Bacterial Core Surveillance. Emerging infections program network, Streptococcus pneumoniae. Centers for Disease Control and Prevention; 1998. [Cited 2017 Jul 26]. Available from: https://www.cdc.gov/abcs/reports-findings/survreports/spneu98.html
- Active Bacterial Core Surveillance. Emerging infections program network, Streptococcus pneumoniae. Centers for Disease Control and Prevention; 1999. [Cited 2017 Jul 26]. Available from: https://www.cdc.gov/abcs/reports-findings/survreports/spneu99.html
- Active Bacterial Core Surveillance. Emerging infections program network, Streptococcus pneumoniae. Centers for Disease Control and Prevention; 2008. [Cited 2017 Jul 26]. Available from: https://www.cdc.gov/abcs/reports-findings/survreports/spneu08.html
- Active Bacterial Core Surveillance. Emerging infections program network, Streptococcus pneumoniae. Centers for Disease Control and Prevention; 2009. [Cited 2017 Jul 26]. Available from: http://www.cdc.gov/abcs/reports-findings/survreports/spneu09.html
- Active Bacterial Core Surveillance. Emerging infections program network, Streptococcus pneumoniae. Centers for Disease Control and Prevention; 2013. [Cited 2017 Jul 26]. Available from: http://www.cdc.gov/abcs/reports-findings/survreports/spneu13.html
- Active Bacterial Core Surveillance. Emerging infections program network, Streptococcus pneumoniae. Centers for Disease Control and Prevention; 2014. [Cited 2017 Jul 26]. Available from: http://www.cdc.gov/abcs/reports-findings/survreports/spneu14.html
- Gierke R, McGee L, Beall B, Pilishivili T. Manual for the Surveillance of Vaccine-Preventable Diseases, Chapter 11: Pneumococcal. Centre for Disease Control and Prevention; 2012. [Cited 2017 Jul 26]. Available from: https://www.cdc.gov/vaccines/pubs/surv-manual/chpt11-pneumo.html
- Moore MR, Link-Gelles R, Schaffner W, et al. Effect of use of 13-valent pneumococcal conjugate vaccine in children on invasive pneumococcal disease in children and adults in the USA: analysis of multisite, population-based surveillance. Lancet Infect Dis. 2015;15:301–309.
- Jayasinghe S, Menzies R, Chiu C, et al. Long-term impact of a “3 + 0” schedule for 7- and 13-valent pneumococcal conjugate vaccines on invasive pneumococcal disease in Australia, 2002–2014. Clin Infect Dis. 2017;64:175–183.
- Centre for Disease Control and Prevention [Internet]. Active Bacterial Core Surveillance: Surveilance reports 1998–2016; [cited 2017 Jul 27]. Available from: https://www.cdc.gov/abcs/reports-findings/surv-reports.html
- Cohen R, Cohen JF, Chalumeau M, et al. Impact of pneumococcal conjugate vaccines for children in high- and non-high-income countries. Expert Rev Vaccines. 2017;16:625–640.
- Hausdorff WP, Siber G, Paradiso PR. Geographical differences in invasive pneumococcal disease rates and serotype frequency in young children. Lancet. 2001;357:950–952.
- Vergison A, Hanquet G. Challenges in estimating the impact of pneumococcal conjugate vaccines through surveillance. Pediatr Infect Dis J. 2012;31:400–403.
- Baraff LJ, Bass JW, Fleisher GR, et al. Practice guideline for the management of infants and children 0 to 36 months of age with fever without source. Agency for health care policy and research. Ann Emerg Med. 1993;22:1198–1210.
- O’Brien KL. PCV13 impact evaluations: the obvious and the unpredicted. Pediatr Infect Dis J. 2013;32:264–265.
- Kazmierczak K, Hackel M, Henry LI et al. Distribution of Streptococcus pneumoniae serotypes among global populations. 27th European Congress of Clinical Microbiology and Infectious Diseases; 2017 Apr 22–25; Vienna, Austria
- Hausdorff WP, Hanage WP. Interim results of an ecological experiment - Conjugate vaccination against the pneumococcus and serotype replacement. Hum Vaccin Immunother. 2016;12:358–374.
- Balsells E, Guillot L, Nair H, et al. Serotype distribution of Streptococcus pneumoniae causing invasive disease in children in the post-PCV era: A systematic review and meta-analysis. PLoS One. 2017;12:e0177113.
- De Oliveira LH, Camacho LAB, Coutinho ESF, et al. Impact and effectiveness of 10 and 13-valent pneumococcal conjugate vaccines on hospitalization and mortality in children aged less than 5 years in Latin American countries: a systematic review. PLoS One. 2016;11:e0166736.
- Naucler P, Galanis I, Morfeldt E, et al. Comparison of the impact of PCV10 or PCV13 on invasive pneumococcal disease in equivalent populations. Clin Infect Dis. 2017.
- Rinta-Kokko H, Toropainen M, Palmu AA et al. Impact of 10-valent pneumococcal conjugate vaccine (PCV10) among vaccine-eligible children in Finland, 2010–2015. 10th International Symposium on Pneumococci & Pneumococcal Diseases; 2016 Jun 26–30; Glasgow, Scotland.
- Vesikari T, Wysocki J, Chevallier B, et al. Immunogenicity of the 10-valent pneumococcal non-typeable Haemophilus influenzae protein D conjugate vaccine (PHiD-CV) compared to the licensed 7vCRM vaccine. Pediatr Infect Dis J. 2009;28:S66–76.
- Wysocki J, Tejedor JC, Grunert D, et al. Immunogenicity of the 10-valent pneumococcal non-typeable Haemophilus influenzae protein D conjugate vaccine (PHiD-CV) when coadministered with different neisseria meningitidis serogroup C conjugate vaccines. Pediatr Infect Dis J. 2009;28:S77–88.
- Bermal N, Szenborn L, Chrobot A, et al. The 10-valent pneumococcal non-typeable Haemophilus influenzae protein D conjugate vaccine (PHiD-CV) coadministered with DTPw-HBV/Hib and poliovirus vaccines: assessment of immunogenicity. Pediatr Infect Dis J. 2009;28:S89–96.
- Bermal N, Szenborn L, Edison A, et al. Safety and immunogenicity of a booster dose of the 10-valent pneumococcal nontypeable Haemophilus influenzae protein D conjugate vaccine coadministered with DTPw-HBV/Hib and poliovirus vaccines. Pediatr Infect Dis J. 2011;30:69–72.
- Kim CH, Kim JS, Cha SH, et al. Response to primary and booster vaccination with 10-valent pneumococcal nontypeable Haemophilus influenzae protein D conjugate vaccine in Korean infants. Pediatr Infect Dis J. 2011;30:e235–43.
- van den Bergh MR, Spijkerman J, Francois N, et al. Immunogenicity, safety, and reactogenicity of the 10-valent pneumococcal nontypeable Haemophilus influenzae protein D conjugate vaccine and DTPa-IPV-Hib when coadministered as a 3-dose primary vaccination schedule in The Netherlands: a randomized controlled trial. Pediatr Infect Dis J. 2011;30:e170–8.
- van den Bergh MR, Spijkerman J, Francois N, et al. Immunogenicity, safety and reactogenicity of a booster dose of the 10-valent pneumococcal nontypeable H. influenzae protein D conjugate vaccine coadministered with DTPa-IPV-Hib in Dutch children: a randomized controlled trial. Pediatr Infect Dis J. 2016;35:e206–19.
- Hausdorff WP, Hoet B, Schuerman L. Do pneumococcal conjugate vaccines provide any cross-protection against serotype 19A? BMC Pediatr. 2010;10:4.
- Poolman J, Frasch C, Nurkka A, et al. Impact of the conjugation method on the immunogenicity of Streptococcus pneumoniae serotype 19F polysaccharide in conjugate vaccines. Clin Vccine Immunol. 2011;18:327–336.
- Moore MR, Link-Gelles R, Schaffner W, et al. Effectiveness of 13-valent pneumococcal conjugate vaccine for prevention of invasive pneumococcal disease in children in the USA: a matched case-control study. Lancet Respir Med. 2016;4:399–406.
- Weinberger R, Van Der Linden M, Imöhl M, et al. Vaccine effectiveness of PCV13 in a 3 + 1 vaccination schedule. Vaccine. 2016;34:2062–2065.
- van der Linden M, Falkenhorst G, Perniciaro S, et al. Effectiveness of Pneumococcal Conjugate Vaccines (PCV7 and PCV13) against invasive pneumococcal disease among children under two years of age in Germany. PLoS One. 2016;11:e0161257.
- Andrews NJ, Waight PA, Burbidge P, et al. Serotype-specific effectiveness and correlates of protection for the 13-valent pneumococcal conjugate vaccine: a postlicensure indirect cohort study. Lancet Infect Dis. 2014;14:839–846.
- Collins S, Sheppard C, Litt D et al. Trends in invasive pneumococcal disease over time: England and Wales 2000/01 to 2014/15. Poster session presented at: plenary session: burden of pneumococcal disease. 10th International Symposium on Pneumococci & Pneumococcal Diseases; 2016 Jun 26–30; Glasgow, Scotland
- Corcoran M, Vickers F, Fitzgerald M et al. The persistence of serotype 19A – despite the introduction of PCV13 vaccine. Poster session presented at: bacterial genomics: vaccine driven serotype/genotype changes. 10th International Symposium on Pneumococci & Pneumococcal Diseases; 2016 Jun 26–30; Glasgow, Scotland
- Van Effelterre T, Moore MR, Fierens F, et al. A dynamic model of pneumococcal infection in the United States: implications for prevention through vaccination. Vaccine. 2010;28:3650–3660.
- Hanquet G, Valenciano M, Simondon F, et al. Vaccine effects and impact of vaccination programmes in post-licensure studies. Vaccine. 2013;31:5634–5642.
- Ruckinger S, Van Der Linden M, Reinert RR, et al. Reduction in the incidence of invasive pneumococcal disease after general vaccination with 7-valent pneumococcal conjugate vaccine in Germany. Vaccine. 2009;27:4136–4141.
- Petti CA, Polage CR, Quinn TC, et al. Laboratory medicine in Africa: a barrier to effective health care. Clin Infect Dis. 2006;42:377–382.
- Global health. Challenges in improving infectious disease surveillance systems. United States General Accounting Office; 2001. [Cited 2017 Jul 26]. Available from: http://www.gao.gov/new.items/d01722.pdf
- Von Gottberg A, De Gouveia L, Tempia S, et al. Effects of vaccination on invasive pneumococcal disease in South Africa. N Engl J Med. 2014;371:1889–1899.
- Mackenzie GA, Hill PC, Jeffries DJ, et al. Effect of the introduction of pneumococcal conjugate vaccination on invasive pneumococcal disease in the Gambia: a population-based surveillance study. Lancet Infect Dis. 2016;16:703–711.