ABSTRACT
Introduction
Prior to implementation of Haemophilus influenzae type b (Hib)-conjugate vaccination programs in the 1990s, Hib was the commonest cause of bacterial meningitis in children aged <5 years. While the burden of all Hib disease has significantly decreased in the post-vaccination era, Hib still accounted for >29,000 deaths worldwide in children aged <5 years in 2015.
Areas covered
We reviewed literature data on the most widely used Hib vaccines and vaccination strategies which led to the global prevention and control of Hib disease and aim to highlight important factors for continued disease control and elimination in the future.
Expert commentary
More than 90% of countries worldwide have implemented Hib-conjugate vaccination in their national immunization programs. Vaccines containing Hib polyribosylribitol phosphate (PRP) conjugated with tetanus toxoid (Hib-TT) are the most commonly used. Neisseria meningitidis outer membrane protein complex of PRP (Hib-OMP) is also used. Although the kinetics of the immune response varies with Hib vaccine and schedule used, high control of Hib disease was observed in all settings/scenarios. Further improving global Hib vaccination coverage may result in disease elimination.
Plain language summary
What is the context?
Haemophilus influenzae is causing a variety of diseases, from otitis media and sinusitis to invasive disease (e.g. meningitis and pneumonia).
H. influenzae type b (Hib) was the most common cause of bacterial meningitis in children <5 years of age, and especially among <2-year-olds. Even with appropriate treatment, up to 40% of children with bacterial meningitis can suffer permanent disabilities and up to 5% will die.
The development of vaccines to protect against Hib disease has started in the late 1970s and has culminated with the licensure of 4 Hib conjugate vaccines, of which 2 are currently widely used.
What is new?
In this review, we gathered evidence on the different Hib vaccines and vaccination strategies that have contributed to the global prevention and control of Hib disease.
The review indicates:
the incidence of Hib disease has decreased considerably due to the introduction of Hib vaccines in national immunization programs worldwide. However, Hib disease is not yet completely eradicated.
the vaccines currently used offer protection against Hib over long periods of time.
carriage of the pathogen by healthy individuals seem to be less frequent, but data are still needed to fully evaluate the impact of vaccination.
other H. influenzae types are now more frequent.
Why is this important?
Despite the huge success of Hib vaccination, continuous surveillance is needed to anticipate potential re-emergences and devise the best strategies for prevention and control of disease.
Hib vaccination should be considered in the few countries who have not yet implemented it, to decrease associated morbidity and mortality.
1. Background
Haemophilus influenzae is a gram-negative coccobacillus causing a variety of diseases, from otitis media and sinusitis to invasive diseases, such as meningitis and bacteremic pneumonia. Of the 6 known serotypes (a–f), serotype b (Hib) accounted for 95% of all H. influenzae invasive disease in the pre-vaccine era [Citation1]. At that time Hib was the most common cause of bacterial meningitis in children <5 years of age, with more than 83% of cases occurring in children aged <2 years [Citation2]. Although it has decreased over the last three decades, the burden of Hib disease remains significant. In 2015, among children younger than 5 years, there were an estimated 340,000 cases of severe Hib infection globally, with the majority (76%) presenting as pneumonia, and 29,600 deaths attributed to Hib [Citation3].
Even with appropriate treatment, case-fatality ratios can reach 5%, and up to 40% of children may suffer permanent minor and major sequelae [Citation1]. For Hib meningitis, the risk of at least one major sequelae, including hearing loss, seizures, and intellectual impairment, was estimated to be 9.5% [Citation4].
Increasing antimicrobial resistance has been reported worldwide for Hib strains [Citation1], with multi- [Citation5,Citation6] and even extensively-drug resistant [Citation7] isolates emerging, thus limiting available treatment options. Therefore, to reduce the burden of Hib disease, it is essential to improve prevention strategies, such as the use of routine infant immunization. Vaccine development was based on the discovery that the capsular polysaccharide of the pathogen, polyribosylribitol phosphate (PRP), is the major virulence factor for Hib [Citation1]. Serum anti-PRP antibody concentrations ≥0.15 μg/mL are considered indicative of short-term protection [Citation8]. However, antibody levels were observed to wane rapidly over a few months following either natural exposure or vaccination with polysaccharide vaccines. Therefore, concentrations ≥1 μg/mL after exposure/immunization are correlated with long-term protection against disease [Citation9], by ensuring that levels ≥0.15 μg/mL are maintained throughout periods of risk of invasive Hib disease. Serum anti-PRP levels ≥5 μg/mL have been correlated with protection against Hib nasopharyngeal carriage [Citation10].
Plain PRP polysaccharide vaccines were developed in the 1970s [Citation11,Citation12], but had no demonstrable effect on nasopharyngeal carriage of Hib, and thus did not interrupt transmission of Hib and did not induce herd protection. Polysaccharide vaccines activate B cells via a T-helper cell-independent pathway – which is poorly developed in children <18 months of age – and are characterized by a short-lived antibody response, poor immunogenicity and a lack of induced immunological memory [Citation13]. In the late 1980s, Hib protein-conjugate vaccines were developed, improving the immunogenicity of the PRP polysaccharide. Polysaccharide-protein conjugate vaccines induce a T-cell-dependent response, which develops much earlier in life and can therefore be initiated in infants ≤12 months of age) from the age of 6 weeks [Citation1,Citation13]. Conjugate vaccines provide key benefits based on T-cell-dependent immunogenicity, including induction of immunological memory, reduction of nasopharyngeal carriage leading to a herd effect, and boostability of immune response to subsequent immunization. Four Hib conjugate vaccines were licensed, using different protein carriers for PRP: diphtheria toxoid (Hib-DT), Neisseria meningitidis outer membrane protein complex (Hib-OMP), tetanus toxoid (Hib-TT) and the nontoxic mutant of Corynebacterium diphtheriae toxin (Hib-CRM197). Currently, Hib vaccines are given either as a 2-dose or a 3-dose series in infancy with or without a booster dose between 1–2 years of age, typically as a component of combination vaccines containing tetanus toxoid, diphtheria toxoid and pertussis (acellular [DTaP] or whole-cell [DTwP]) antigens, and may also contain, in addition, hepatitis B and inactivated poliovirus (IPV) components [Citation14].
Following implementation of Hib vaccines in routine infant immunization programs starting in the late 1980s, the incidence of Hib disease has drastically decreased, and a further decline was observed after the 2000s, when use of Hib vaccination was also extended in most low-income countries [Citation1,Citation13]. By the beginning of 2019, 191 countries had introduced Hib vaccination in their national immunization programs (NIPs) and global vaccination coverage with three Hib vaccine doses was estimated at 72% in 2018. However, vaccine coverage varies greatly by region and country (as low as 23% for the Western Pacific Region and up to 87% in the Americas and South East Asia in 2018) [Citation15], and the burden of Hib disease remains considerable in countries with low vaccine uptake. In addition, even in countries with high vaccination coverages, outbreaks continue to occur sporadically [Citation16–18]. Therefore, there is still a need for continuous surveillance and reevaluation of vaccination strategies to ensure progress toward disease eradication.
This review aims to address the different Hib vaccines and vaccination strategies that led to the global prevention and control of Hib disease and to highlight important factors for continued disease containment in the future. A plain-language summary contextualizing the findings and potential clinical research relevance and impact is presented in .
2. Hib in the pre-vaccination era
In the pre-vaccination era, yearly rates of Hib infections reached 40–50 cases/100,000 among children <5 years of age, even in middle- and high-income countries [Citation12]. The most common clinical manifestations of Hib disease were meningitis (52% of cases), pneumonia (12%), epiglottitis (10%), and septicemia (8%) [Citation12], with case-fatality ratios varying between 3% (for epiglottitis) and 28% (meningitis) [Citation12].
Hib meningitis alone reached estimated incidences of 57.9, 67.1 and 31.9/100,000 in children 1–5 months, 6–11 months and 12–23 months of age, respectively, and accounted for 42.4% of all bacterial meningitis cases with known etiology occurring in children <5 years of age. Estimated case-fatality ratios varied by region from as low as 4.1% in the World Health Organization (WHO) European region to 27.6% in the African region [Citation2].
In view of the high burden of disease, most high-income countries had already introduced Hib vaccination before the WHO issued its global recommendation for Hib vaccination in 2006 [Citation19]. With support from the WHO and the Gavi Alliance, several low-income countries had also implemented Hib vaccination, of which the Gambia was the first to introduce a Hib vaccine in its NIP in 1997 [Citation20]. Currently, all Gavi-eligible countries have introduced Hib vaccines [Citation21].
3. Hib in the post-vaccination era
3.1. Reduced global incidence
A rapid impact on the burden of invasive Hib disease was observed in the early 1990s, following the implementation of routine infant immunization in several countries [Citation12], and more than a decade later, disease incidence remains low in countries with high vaccination coverage [Citation1,Citation22]. A significant impact has also been observed on hospitalization rates. For instance, in New Zealand, a decline of 84% in hospitalizations due to Hib disease in <15-year-olds was observed within 2 years of vaccine introduction in 1993 (from 13.53 to 2.19/100,000), and hospitalizations and notification rates continue to decrease over time [Citation23]. In Italy, Hib vaccination was introduced in 1999 and vaccination coverage increased consistently from 83.4% in 2002 to 95% in 2011 [Citation24]. A recent study estimated the impact of Hib vaccination 15 years after its introduction, from 2001 to 2013. Although yearly fluctuations were noted, hospitalization rates due to invasive H. influenzae disease decreased in children 1–4 years (from 2.3 to 0.2/100,000 population) as well as in infants (from 5.4 to 1.6/100,000) [Citation24].
In the United States (US), the overall annual incidence of Hib disease was 0.03 cases/100,000 population between 2009 and 2015, but estimates varied with age group and reached 0.3/100,000 in infants [Citation25]. An increase in non-b H. influenzae-caused infections was observed (3% and 13% annually, for non-typeable H. influenzae [NTHi] and H. influenzae type a [Hia], respectively), while the number of yearly Hib cases remained relatively constant over this period. Moreover, associated case-fatality ratios were considerably lower for Hib (3.9%) than for non-b serotypes (10.8%) or NTHi (16.1%) [Citation25]. In 2017, Hib incidence was 0.02 cases/100,000 in individuals of all ages, lower than the targeted objective for 2020 (0.27/100,000), while the incidence rate among <5-year-olds was 0.19/100,000 [Citation26].
In the European Union (EU) and the European Economic Area (EEA), a constant decline in Hib cases was observed from 1999 to 2017 (). As in the US, this was paralleled by an increase in NTHi invasive infections (from 0.27/100,000 in 1999 to 0.56/100,000 in 2015) and, to a lesser extent, in non-b serotype disease notification rates, especially among infants and adults ≥65 years of age [Citation16,Citation27]. In 2017, a notification rate of 0.8/100,000 was reported, but only 8% of all H. influenzae infections and 3 out of 169 fatal cases were attributable to Hib, all three occurring in infants [Citation16]. The decrease in Hib incidence corresponded to an increase in Hib vaccination coverage [Citation27], with all EU/EEA member states having implemented Hib vaccination in their NIPs by 2010.
Figure 2. Reported invasive Hib cases in EU/EEA (overall and in countries with consistent reporting available), from 1996 to 2017 [Citation28]
![Figure 2. Reported invasive Hib cases in EU/EEA (overall and in countries with consistent reporting available), from 1996 to 2017 [Citation28]](/cms/asset/8e68d745-d258-4c06-a2f1-38cc8016364a/ierv_a_1825948_f0002_oc.jpg)
In Australia, surveillance of Hib has been in place since 1994, 1 year after vaccine introduction in the NIP. A sharp decrease in Hib cases (from >50 to <10 yearly cases) was observed by 1996 and then incidence remained low, with an average annual notification rate of 0.09/100,000 population reported between 2008 and 2011 [Citation29].
In The Gambia, invasive Hib disease virtually disappeared by 2002, at 5 years post-introduction of Hib conjugate vaccine [Citation30]. More recently, a resurgence was observed but annual incidences of Hib meningitis and all invasive Hib disease remained below 5/100,000 in children aged <5 [Citation31,Citation32] and low oropharyngeal carriage rates (0.9%) during 2007–2010 [Citation31]. A peak in Hib meningitis incidence was observed during 2012–2013, followed by a decrease in the subsequent 4 years [Citation33]. Other African countries also reported substantial decreases in Hib disease incidence. In Kenya, a decline from 62.6/100,000 in the pre-vaccine era (2000–2001) to 4.5/100,000 in 2004–2014 was reported in children <5 years of age, along with very low nasopharyngeal carriage rates (0.2%) in the post-vaccine era [Citation34]. A rapid and substantial drop in Hib meningitis cases was also reported in Malawi within 2 years from Hib vaccine introduction in 2002, and a consistent decrease was observed up to 2012 [Citation35].
Among Asian countries, Japan provides a more recent example of the swift impact of Hib vaccination. Hib vaccines were introduced as a voluntary vaccination in 2008 and then as part of the NIP in 2013. A decrease of 93% in the number of H. influenzae cases among children <5 years of age was observed during 2013–2017 as compared with 2008–2012 and no Hib cases have been identified since 2014 [Citation36]. Even in the absence of publicly funded mass vaccination, Singapore reported the near-disappearance of Hib invasive disease in 2010, with a 95% reduction in invasive Hib disease incidence between 1994–2003 and 2004–2010, when national vaccine coverage rose from 22% to >90% [Citation37].
Nevertheless, in regions where Hib vaccination is not implemented or vaccine coverage remains low, the burden of disease is still high, especially in children <5 years of age. Although Hib-associated mortality is believed to have been reduced by 90% from 2000 to 2015, a global incidence rate of 148 cases/100,000 children was estimated in 2015 for invasive Hib disease. The regions with the highest Hib burden were the Western Pacific (317 cases/100,000 children), South East Asia (238/100,000 children) and Africa (75/100,000 children) [Citation3]. Most Hib deaths were clustered in four countries: India, Nigeria, China, and South Sudan [Citation3], all of which had low 3-dose Hib vaccination coverages (≤55% [Citation38]) due to recent or no vaccine introduction in their NIP in 2015.
3.2. Dynamics of H. influenzae epidemiology
Slight increases in the incidence of invasive H. influenzae disease have become apparent since 2012, especially in the EU/EEA and the US, attributed mainly to NTHi and non-b serotypes and this has been more pronounced in individuals >65 years of age [Citation16,Citation27,Citation39]. For instance, in the EU/EEA, non-capsulated strains caused more than 76% of cases of invasive H. influenzae disease in 2017, among all age groups, compared with 8% for Hib [Citation16]. In the same year, in the US, rates were estimated at 1.51 cases/100,000 population for NTHi disease, reaching 14.81/100,000 in ≥85-year-olds, while the incidence of Hib was 0.02/100,000 population for Hib [Citation26]. In addition, increases have been observed in Hia infections in Indigenous communities in the northern regions of Canada and Alaska over the last decades [Citation40] and in invasive disease incidence resulting from H. influenzae serotypes e and f in Europe in the past decade [Citation41]. However, it is unclear if these trends are not in fact related to natural, temporal variations of the pathogen epidemiology. The role of mass Hib vaccination resulting in strain replacement for H. influenzae disease has not been established, as some studies pointed toward a changing epidemiology in the vaccinated population [Citation42,Citation43], while others did not find any evidence for serotype replacement [Citation44,Citation45]. Even if occurring, the risks associated with strain replacement fall far below those associated with withholding routine Hib vaccination. However, this further underlines the importance of ongoing surveillance for H. influenzae disease, in order to fully understand trends in epidemiology and formulate prompt prevention strategies. In this respect, the development of new vaccines against non-b H. influenzae strains should be a priority, especially since associated case-fatality ratios can be higher than for Hib disease [Citation45].
3.3. Current trends in Hib epidemiology and clinical presentation
While Hib infections are largely controlled and prevented by mass vaccination [Citation1], new cases continue to emerge, showing that the disease is not eradicated.
In Argentina, where Hib vaccination was introduced in the NIP in 1998, a possible Hib reemergence was signaled in 2014, when coverage had reached 94% [Citation38] Of note, in the post-vaccine era, an overall reduction in the rates of hospitalized Hib meningitis cases of 89.8% was observed, as compared with the pre-vaccination era [Citation46].
In France, where vaccination coverages ranged between 95% and 97% over the last decade [Citation38], an almost 2-fold increase in Hib cases (from <10 per year) was observed in 2018 compared with previous years. Most of them occurred in children <5 years of age. In addition, 10 vaccine failures have been reported [Citation18].
Eighteen Hib vaccine failures, of which 17 were in healthy children at the time of vaccination, were also reported in Portugal between 2010 and 2018. Vaccine failure was defined as invasive Hib disease occurred ≥2 weeks after one Hib vaccine dose, given after the first birthday, or ≥1 week after ≥2 doses, given at <1 year of age. Half of the vaccine failures were reported in 2017 and 2018, and all occurred in children ≥4 years of age, with one resulting in death [Citation17]. Over the entire period, 94 invasive H. influenzae isolates were reported, of which 29 were Hib. The most common clinical manifestations of the disease were pneumonia, meningitis, epiglottitis, and bacteremia [Citation17].
The potential reemergence of Hib cases in countries with high vaccination coverage is concerning and must be accounted for when formulating future strategies for prevention, control and elimination of Hib.
The clinical presentation of invasive Hib disease has also changed in the post-vaccination era. Following a dramatic decrease in Hib-related meningitis [Citation3] and epiglottitis [Citation47] cases in countries with high vaccination coverage, other presentations of Hib disease have become more common. In Europe, over the last 5 years, bacteremia and pneumonia were the most frequently reported clinical presentations of H. influenzae disease in all ages, including adults; however, meningitis remained the most prominent presentation for Hib cases [Citation16,Citation48]. These changes may also be misleading, as manifestations such as epiglottitis or septic arthritis are less common in the post-vaccination era and therefore, tend to be overlooked. For instance, a recent epiglottitis case in a 2-year-old child was initially misdiagnosed twice before the final diagnosis was reached based on laryngoscopy; Hib was subsequently identified as the causative pathogen [Citation49].
4. Today’s Hib vaccines
The 4 licensed monovalent Hib vaccines contain PRP, conjugated to different carrier proteins (Supplemental Table 1). They also differ in terms of size, activation and functionality of the PRP saccharide and in the method of conjugation and production. All 4 vaccines were shown to be efficacious against invasive Hib disease in clinical trials, with vaccine efficacy varying between 87 and 100% for Hib-DT, 90 and 100% for Hib-CRM, 93 and 95% for Hib-OMP, and 93 and 100% for Hib-TT after at least 2 doses [Citation50,Citation51].
Combination Hib-vaccines were rapidly developed after monovalent vaccines, with most of them containing DTaP or DTwP components. Administration of DTPa/Hib and/or IPV-containing combinations does not impact the immunogenicity and antibody functionality induced by each vaccine component, and protective levels of antibodies against diphtheria, tetanus and Hib are induced after vaccination; moreover, high vaccine effectiveness was shown in several countries using DTaP/Hib combination vaccines [Citation52,Citation53].
The first Hib-containing combination vaccines were licensed in the early 1990s as DTwP combinations and in the mid-1990s as DTaP vaccines. IPV was added to these combinations in 1998, and hexavalent vaccines, also containing hepatitis B antigens appeared in the 2000s. Currently, several Hib-containing combination vaccines (Supplemental Table 1) are used in different countries worldwide. The hexavalent vaccines Infanrix hexa (DTaP-HB-IPV/Hib; a 3-component pertussis vaccine; GSK), Hexacima/Hexyon/Hexaxim (DTaP2-HB-IPV-Hib, a 2-component pertussis vaccine; Sanofi Pasteur) and Vaxelis (DTPa5-HB-IPV-Hib, a 5-component pertussis vaccine; MCM Vaccine Co.) and the pentavalent vaccines Infanrix-IPV/Hib (DTaP-IPV/Hib; GSK) and Pentacel (DTaP/IPV-Hib; Sanofi Pasteur) are approved for use in the EU and/or the US, for primary and booster vaccination. Two meningococcal (against serogroups C and/or Y) Hib combination vaccines, Menitorix (MenC-Hib, GSK) and MenHibrix (MenCY-Hib, GSK) are also available. All these vaccines contain Hib-TT, except DTPa5–HB–IPV–Hib, which contains Hib-OMP.
In addition, several other Hib-containing vaccines are currently pre-qualified by the WHO [Citation54]; the Hib protein carrier is TT for all of them (Supplemental Table 2).
4.1. Immune response following Hib-TT and Hib-OMP vaccination
The Hib-conjugate vaccines show different evolution of immune response over time following vaccination, depending on the nature of the carrier protein. Hib-OMP vaccines have been shown to elicit a higher immune response after the first or second dose of the primary series when compared with Hib-TT vaccines [Citation55,Citation56], likely due to the immune-stimulating effect of the meningococcal PorB protein in the OMP, which activate B cells via Toll-like receptor 2 [Citation57]. This observation was the basis for the use of Hib-OMP in certain Indigenous populations (as described in Section 6), where the mounting of an immune response at an early age was key, due to the very high Hib disease incidence rates. However, after completion of a 3-dose series, immune responses were similar for the two vaccines [Citation56]; moreover, some studies evidenced higher immune responses to Hib-TT than Hib-OMP vaccination. In a study comparing Hib-conjugate vaccine administration in infants, mean antibody levels were 3.64 µg/mL for Hib-TT and 1.14 µg/mL for Hib-OMP and 83% and 55% of infants, respectively, had protective antibody levels of 1 µg/mL, 1 month after completing the 3-dose series at 2, 4 and 6 months of age [Citation58].
Immune responses following the administration of a booster dose are demonstrated to be lower after a monovalent Hib-OMP vaccination compared with Hib-TT [Citation13]. In a study comparing Hib-TT with Hib-OMP in which the vaccines were administered at 3, 5 and 12 months of age, significantly higher mean antibodies were observed after the third dose among Hib-TT than Hib-OMP vaccinees (10.21 µg/mL versus 1.90 µg/mL), although 99% of infants had anti-PRP antibody concentrations ≥0.15 µg/mL, regardless of the vaccine received [Citation59]. Similarly, in a head-to-head comparison between the Hib-OMP-containing DTPa5-HB-IPV-Hib and the Hib-TT-containing DTaP-HB-IPV/Hib, higher geometric mean titers (GMTs) were observed in children vaccinated with DTaP-HB-IPV/Hib (21.39 µg/ml) than DTPa5-HB-IPV-Hib (6.79 µg/ml) at 1 month after administration of a 3 + 1 schedule at 2, 3, 4 and 12 months of age. Nevertheless, at the same time point, the percentages of toddlers with anti-PRP antibody levels indicative of short- and long-term protection were similar between the two groups, and pre-booster values indicated higher persistence of immune response among DTPa5-HB-IPV-Hib vaccinees [Citation60]. Similar observations were made for the dynamics of anti-PRP antibody levels following administration of the same combination vaccines according to a 2 + 1 schedule, at 2, 4, and 11–12 months of age. Higher GMTs and percentages of children with anti-PRP antibody concentrations ≥0.15 µg/mL and ≥1 µg/mL were observed after primary vaccination and pre-booster dose for DTPa5-HB-IPV-Hib compared to DTaP-HB-IPV/Hib vaccinees; however, at one-month post-booster dose, GMTs were higher among children receiving DTaP-HB-IPV/Hib and the percentage of toddlers with levels indicative of short- and long-term protection were similar between groups [Citation61]. illustrates the dynamics of anti-PRP antibody levels following administration of DTaP5-HB-IPV-Hib and DTaP-HB-IPV/Hib according to a 2 + 1 schedule [Citation61].
Figure 3. Comparison of dynamics of anti-PRP antibody levels following administration of DTaP5-HB-IPV-Hib and DTaP-HB-IPV/Hib according to a 2 + 1 schedule, at 2, 4 and 11–12 months of age [Citation61]
![Figure 3. Comparison of dynamics of anti-PRP antibody levels following administration of DTaP5-HB-IPV-Hib and DTaP-HB-IPV/Hib according to a 2 + 1 schedule, at 2, 4 and 11–12 months of age [Citation61]](/cms/asset/34a580c6-f826-4fc6-96c4-198c60918f05/ierv_a_1825948_f0003_oc.jpg)
Monovalent Hib-TT vaccination yielded higher persisting antibody levels than Hib-OMP vaccination at 18 months after the completion of a 2 + 1 series, administered at 3, 5, and 12 months of age [Citation59,Citation62]. Persistence of circulating antibodies was also observed for Hib-TT-containing combination vaccines. For instance, among children vaccinated with DTaP2-HB-IPV-Hib (according to a 6–10–14 week schedule plus a booster dose at 15–18 months or a 2-4-6 months primary series and booster dose at 12–24 months), ≥98% and ≥78% of children maintained anti-PRP antibody concentrations ≥0.15 µg/mL and ≥1.0 µg/mL, respectively, at 4.5 years of age, regardless of the vaccination schedule received during the first 2 years of life [Citation63]. Protective antibody levels were also shown to persist until at least 9 years of age in children vaccinated with a 3 + 1 dose schedule of DTPa-HB-IPV/Hib, with ≥98% of children maintaining anti-PRP antibody concentrations ≥0.15 µg/mL [Citation64].
Evidence suggests that antibody quality may also differ with Hib conjugates. In a study comparing antibodies elicited by Hib-CRM, Hib-TT and Hib-OMP vaccination in infants receiving 3 vaccine doses at 2, 4 and 6 months of age, a significantly higher mean avidity was observed for Hib-CRM, followed by Hib-TT and Hib-OMP; antibodies with higher avidity were also ~6.6-fold more potent than low-avidity antibodies in complement-mediated bactericidal activity assays [Citation65]. However, in another study comparing vaccination at 2, 3 and 4 months of age, both Hib-TT and Hib-OMP were found to elicit IgG PRP antibodies with heterogeneous avidity (albeit with high median values), and no correlation between avidity and anti-PRP antibody concentration was established [Citation66].
Despite the mounting of a more rapid and more pronounced immune response for Hib-OMP than for Hib-TT (especially after the first dose), higher antibody levels and better antibody persistence are achieved post-boosting when using Hib-TT. The efficacy of the 2 vaccines is assumed to be similar, although no head-to-head trials have been conducted. Moreover, for both vaccines, a substantial impact on Hib disease incidence and carriage and induction of herd effect has been observed in the populations in which they have been used (see Section 4.3 for Hib-TT and Section 6 for Hib-OMP). In the general population, Hib-TT is currently the most widely used conjugate against Hib [Citation54] and has also had a significant role in reducing disease burden. For instance, in Europe, the successful control of Hib disease was and continues to be driven by vaccines containing the Hib-TT conjugate [Citation27]. It is also important to note that no direct efficacy comparison across Hib-TT vaccines from different manufacturers have been so far performed. Although no significant differences are anticipated, the monitoring of immunogenicity data for each vaccine in clinical trials, as well as real-life settings remains paramount.
4.2. Vaccination schedules
In addition to the number of doses, schedules currently adopted for vaccinations against Hib differ in the age at first vaccination or the intervals between doses. Most countries in the African region implement the Expanded Program on Immunization schedule designed by the WHO (with vaccinations at 6, 10, and 14 weeks of age) or an 8, 12, and 16 weeks of age schedule. A 2, 4, 6-month schedule with a booster in the second year or life (in the US or Canada) or administration of four doses at 2, 3, 4 and 12–14 months of age (3 + 1, in several European countries) is also used, along with the Scandinavian schedule (at 3, 5 and 12 months of age) or a 2, 4 and 11-month schedule (2 + 1, for instance in Austria, France, and Spain) [Citation14]. However, there is a lack of clear evidence to favor any of the schedules.
A meta-analysis comprising 20 randomized clinical trials conducted in 15 countries compared different Hib vaccination schedules (3 + 0, 3 + 1 and 2 + 1) and different intervals between primary doses and primary and booster doses. The study found no difference between the schedules in the degree of protection against Hib disease, in terms of clinical efficacy and immunological response [Citation67]. In another meta-analysis, similar vaccine efficacies were estimated after 3 (82%) and 2 primary doses (79%) of Hib vaccines in infancy [Citation68]. The use of a 2 + 1 schedule which is already implemented and has been shown to be effective in several EU countries [Citation69], may therefore also be considered in countries with low- and middle-income, where the cost of an additional vaccination may be prohibitive.
However, in real-world settings (post-vaccine implementation), a booster dose was found to improve the impact of Hib vaccination. For instance, in the United Kingdom (UK), the accelerated schedule at 2, 3 and 4 months of life was initially used in the NIP, starting in 1992. Long-term persistence studies indicated that 32% of vaccinated children had anti-PRP antibody concentrations <0.15 µg/mL at 72 months of age [Citation70]. The concerns raised by this waning in immunity over a relatively short period of time prompted the implementation of a booster Hib vaccination campaign targeting children aged 6 months to 4 years between May and September 2003 [Citation71]. A booster dose at 12 months of age was introduced in the UK NIP in 2006, using MenC-Hib (both components conjugated to TT) (). In children receiving the 3 + 1 schedule, median anti-PRP antibody concentrations were highest in children 1 year old. A decline in antibody levels was observed with increasing age, but anti-PRP antibody concentrations remained ≥1 µg/mL among children up to 8 years of age in 2013–2014. In total, 89% and 56% of the children had concentrations ≥0.15 and ≥1 µg/mL, respectively [Citation72]. While antibody levels still dropped rapidly after the 12-month booster dose, protection was maintained [Citation72].
Figure 4. Real-world experience with Hib vaccination. (A) Number of Hib cases in the United Kingdom between 1990 and 2010 [Citation73]: Hib vaccination (according to a 2, 4, 6 month schedule) is implemented from 1992, but a resurgence in cases is observed between 1999 and 2003, prompting the need for booster and catch-up vaccination campaigns, in the context of a high vaccination coverage (≥90%) achieved since 1994 [Citation38]; (B) Invasive Hib cases in Australian children aged <10 years, from 1993 to 2013 (N = 78 for Indigenous children and N = 501 for Non-Indigenous children) [Citation90]: no increase is observed in Hib disease incidence following the change from Hib-OMP to Hib-TT in the national immunization program, in the context of a high vaccination coverage (≥90%) achieved since 2000 [Citation38] Hib, H. influenzae type b; N, number of Hib cases); NIP, national immunization program; OMP, N. meningitidis outer membrane protein complex; TT, tetanus toxoid
![Figure 4. Real-world experience with Hib vaccination. (A) Number of Hib cases in the United Kingdom between 1990 and 2010 [Citation73]: Hib vaccination (according to a 2, 4, 6 month schedule) is implemented from 1992, but a resurgence in cases is observed between 1999 and 2003, prompting the need for booster and catch-up vaccination campaigns, in the context of a high vaccination coverage (≥90%) achieved since 1994 [Citation38]; (B) Invasive Hib cases in Australian children aged <10 years, from 1993 to 2013 (N = 78 for Indigenous children and N = 501 for Non-Indigenous children) [Citation90]: no increase is observed in Hib disease incidence following the change from Hib-OMP to Hib-TT in the national immunization program, in the context of a high vaccination coverage (≥90%) achieved since 2000 [Citation38] Hib, H. influenzae type b; N, number of Hib cases); NIP, national immunization program; OMP, N. meningitidis outer membrane protein complex; TT, tetanus toxoid](/cms/asset/d5d3be74-d0f5-4689-8f17-c57a901f500a/ierv_a_1825948_f0004_oc.jpg)
In France, the recent observed reemergence in Hib cases may potentially be associated with changes in the vaccines and schedule used, although clear evidence is still lacking. A retrospective survey showed that following the switch from a 3 + 1 to a 2 + 1 vaccination schedule in 2013, mean anti-PRP immunoglobulin G (IgG) concentrations peaked at a younger age (6–11 months versus 2 years old) and were lower when compared to those observed in the 3 + 1 schedule era; moreover, antibody levels declined to <1 µg/ml at considerably younger ages (4–5 years versus 20 years) [Citation18]. Nevertheless, new cases have also been reported in Portugal, in the last decade, where a 3 + 1 schedule (at 2, 4, 6 and 18 months of age) was used [Citation74] and in the context of a high vaccination coverage for 3 Hib doses (≥97%) [Citation38].
Results from a modeling study also indicated that, overall, the administration of a booster dose within 1 year after the primary series would lead to a further decrease in the incidence of Hib disease compared to the scenario of only a primary vaccination series, at high vaccine coverages (90% or 100%). While deferring the booster dose to 2 years post-primary vaccination is not expected to impact direct protection, the model predicts that carriage of Hib would be reduced [Citation75].
Therefore, one single Hib vaccination schedule cannot be used with optimal results globally. However, a variety of schedules can be used and there is ample available evidence on their impact on the burden of disease. This, combined with continuous monitoring of Hib disease over time, will allow national authorities to easily update their NIP with the vaccination schedule most suitable to the epidemiological context.
4.3. Real-world experience with current Hib conjugated vaccines
In the UK, in addition to infant immunizations, catch-up campaigns for children up to 2 years of age were launched in parallel in the first year of Hib vaccine introduction. A rapid decline in Hib disease incidence was achieved not only in the targeted population, but also in other age groups [Citation71], in the context of a consistently high vaccination coverage (>91%) [Citation38]. However, a vaccine failure rate of 2.2 cases/100,000 vaccinees was estimated during 1992–1999 [Citation70] and from 1999, an increase in the number of Hib cases was observed on a yearly basis. In 2002, 120 cases were reported in children <5 years of age [Citation76]. This rise in Hib disease incidence was multifactorial and explained by a greater-than-expected decline in the direct protection offered by vaccination in infancy and the catch-up campaign, a greater efficacy in children vaccinated in catch-up campaigns than in infancy, a lower vaccine effectiveness for children receiving a DTaP rather than a DTwP-Hib vaccine in 2000–2001, and concomitant administration of the CRM-conjugated MenC vaccine [Citation76]. The latter was attributed to bystander interference induced by the CRM carrier protein in the DTaP component and in the CRM-conjugated MenC vaccine, altering the immune response to the Hib component conjugated to TT [Citation77,Citation78]. In 2004, the combination vaccine was changed to one containing a different aP component and this, together with the implementation of the booster dose in the NIP, led to a clear reduction in Hib cases in the following years [Citation71], with annual Hib disease incidence being maintained under 0.1/100,000 during 2012–2016 [Citation72].
The UK experience () underlined the need for a booster dose as part of Hib vaccination strategies. In contrast, in low-income countries like The Gambia [Citation33] or Kenya, where high vaccination coverage (~90%) was achieved, a booster dose does not seem to be needed. In Kenya, a vaccine effectiveness of 93% against invasive Hib disease was reported for the 3-dose series over a period of 15 years from Hib conjugate vaccine implementation [Citation34].
Today, control of Hib disease has been achieved in countries implementing mass vaccination. Combination vaccines, including hexavalent ones, have also shown high vaccine effectiveness against Hib. This is illustrated by the case of DTaP-HB-IPV/Hib, which is currently used extensively in Europe, with continued documented effectiveness against Hib [Citation27].
Adverse events following immunization are uncommon, and Hib vaccines are considered one of the safest available vaccines [Citation1]. Beyond the acceptable safety profile for Hib monovalent and combination vaccines demonstrated in the clinical development programs, after almost 3 decades of post-marketing surveillance, safety data continues to be reassuring [Citation27,Citation79].
5. Nasopharyngeal carriage control – a stepping stone toward Hib disease eradication
Nasopharyngeal Hib carriage rates among children are estimated at 3–5%, although values up to 50% are reported in crowded situations [Citation80], such as children attending day-care centers [Citation81]. Hib mass vaccination also led to a considerable decrease in carriage rates in vaccinated versus unvaccinated children [Citation32,Citation34,Citation82,Citation83] and delayed initial acquisition of Hib following exposure. Consequently, Hib incidence decreased in unvaccinated populations, indicative of a herd protection effect which is now well-established in different geographical regions and socio-economic settings [Citation1,Citation81,Citation84].
Anti-PRP concentration ≥5.0 µg/ml has previously been correlated with protection against Hib carriage, indicating also that higher antibody levels are needed for protection against colonization/carriage than against Hib disease [Citation10]. Modeling studies have shown a higher impact on Hib carriage reduction if the booster dose is administered at 2 years versus 1 year post-primary vaccination [Citation75]. However, a head-to-head comparison of the impact on nasopharyngeal carriage provided by different Hib immunization schedules has not been yet performed [Citation67]. However, available data so far show an impact on Hib carriage regardless of the different settings and vaccination schedule used (for instance in the UK and African countries) [Citation31,Citation32,Citation34,Citation85].
While reduced colonization rates provide indirect protection and minimize the risk of outbreaks, carriage is also temporally associated with the acquisition of natural immunity [Citation86], which accounts for increased resistance to severe Hib infections at older ages. This suggests a complex and yet to be fully elucidated impact of carriage on the Hib epidemiology in the overall population. While Hib carriage continues to remain low in age groups targeted for vaccination [Citation72,Citation87,Citation88], sporadic cases of Hib still occur, underlying the importance of sustained high vaccination coverage across all ages.
6. Hib infections in high-risk populations
Prevention of Hib through vaccination should take into account special populations with a higher risk for developing the disease, such as preterm infants, and/or immunocompromised individuals, as well as certain ethnic groups.
For instance, in various Indigenous populations from industrialized countries (Alaskan Eskimos, Apache Indians and Navajo Indians in the US, Keewatin Natives in Canada, and Aboriginals in Australia), Hib incidence rates run several-fold higher than in similar age groups of the general population. In the pre-vaccination era, the incidence of Hib meningitis in these special-risk groups was estimated at 418.1/100,000 among children <5 years of age, compared to 22.8/100,000 in children the same age in the remaining population [Citation2].
In native Alaskan children, the Hib-CRM vaccine was initially used for mass vaccination in 1991, but it was soon changed with Hib-OMP vaccination, due to the latter’s ability to induce protective antibody levels after the first dose [Citation55] and the lower immunogenicity observed for Hib-CRM (please see Section 4.1). This led to a significant decrease in yearly Hib disease rates among <5-year-old children, from 309.4/100,000 in the pre-vaccination era to 18.3/100,000 in 1992–1995 [Citation89]. Other changes in the vaccination schedules were made over time. For instance, in 1997, the vaccination schedule was modified to Hib-OMP for the first dose and Hib-CRM for the subsequent ones. Starting with 2001, only the Hib-OMP vaccine is used in the native Alaskan population as a stand-alone or combination vaccine [Citation89] and led to further decrease in Hib incidence. Even in the post-vaccination era, the incidence of H. influenzae disease among American Indian and Alaska Natives children <5 years remains higher than in other populations (15.19 versus 2.62/100,000 during 2009–2015). Although most cases are caused by Hia, Hib incidence is also several fold higher, with values estimated at 2.05/100,000 for the children in high-risk group compared with 0.00–0.10/100,000 among the general population [Citation25].
In Australia, Indigenous Aboriginal and Torres Strait Islander children had higher Hib disease incidence rates and earlier onset than non-Indigenous children. Mass vaccination with Hib-OMP was implemented in 1993 in Indigenous children, leading to declines in Hib incidence from the pre-vaccination era. In the early post-vaccination period (1993–1996), disease incidence was 18.1/100,000, further decreasing to 6.2/100,000 in the late (1996–2009) Hib-OMP period. Hib-OMP was also used in non-Indigenous Australians between 2000 and 2005 but was replaced completely by Hib-TT by 2009. Following introduction of Hib-TT, an incidence of 4.7/100,000 was estimated in 2009–2013 [Citation90]. In the post-vaccination era, up to 2013, no significant differences were observed in the number of yearly Hib cases following the switch from Hib-OMP to Hib-TT vaccines (), in both Indigenous and non-Indigenous children <10 years [Citation90]. The Australian experience indicated that transition to Hib-TT is feasible in other vulnerable populations from North America, where Hib-OMP continues to be recommended.
Hib carriage rates also continue to be higher among Indigenous children than among similar age groups in the general population, even in the post-vaccine era, when an important reduction was noted. Among native populations in the US, considerably lower carriage rates were observed after Hib-TT (but not Hib-OMP) vaccination, but remained higher than in the general population [Citation91]. In Australia, at 10 years after the introduction of the Hib vaccine, carriage rates of 3.4% were estimated in Indigenous children, compared to 0.2% in children from the general populations attending childcare centers [Citation92].
The disparities in the incidence of Hib disease between Indigenous populations and non-Indigenous children have been attributed mainly to differences in living conditions (household overcrowding, poor access to washing and laundry facilities, etc) which lead to higher Hib carriage and transmission rates, even among vaccinated children. Despite high vaccination coverage and significant vaccination impact, Indigenous populations remain at higher risk of H. influenzae disease, including Hib disease [Citation93].
Other populations at higher risk of Hib disease include individuals with early component complement deficiencies, immunoglobulin deficiency, anatomic or functional asplenia, or HIV infection; recipients of hematopoietic stem cell transplant; and recipients of chemotherapy or radiation therapy for malignant neoplasms [Citation94]. In the US, Hib vaccination is recommended for all those in high-risk groups as a booster dose [Citation95]. Hib vaccination induces protective antibody levels in asplenic children, adolescents and adults [Citation96,Citation97], in immunocompromised children [Citation98] and patients with multiple myeloma [Citation99]. Following administration of ≥2 doses of Hib conjugate vaccines, vaccine effectiveness estimates of 37.9% in infants and 54.7% in children <2 years of age were previously reported in HIV-infected children, compared with 96.5% and 90.8% in age-matched HIV negative children and an overall lower immune response was observed in HIV positive individuals [Citation100]. In preterm infants, responses to Hib vaccination also tend to be lower than in those born at normal gestational age [Citation101,Citation102], although this difference seems to fade with increasing age [Citation103,Citation104], and several Hib-containing combination vaccines have been shown to be immunogenic and well-tolerated in prematurely born infants [Citation105]. Despite the expected lower immunogenicity and effectiveness of Hib vaccines in these special groups, their vaccination should remain a priority in view of their higher risk of Hib incidence compared with the general population.
7. Conclusion
After decades of global vaccine use, impressive control of Hib invasive disease has been achieved through mass vaccination. However, the disease is not yet eradicated. High vaccination coverage has been achieved in most parts of the world and the number of cases remains low, although there are still a few countries where Hib vaccination has not been implemented in the NIP. The burden of disease and associated mortality in these countries is very high [Citation3], and introduction of mass vaccination should be considered.
8. Expert opinion
Worldwide implementation of mass Hib vaccination is one of the greatest success stories but it has yet to achieve its final goal: eliminating invasive Hib disease. At present, 175 countries are implementing mass Hib vaccination [Citation14]. An important step is introduction of routine immunizations against Hib in the rest of the world, although the right vaccination strategy should be carefully considered and adapted to the current H. influenzae epidemiology in each region. For instance, in China, which has a large population, Hib vaccines are only available in the private market, leading to relatively low vaccine coverage (~55% for the complete vaccination schedule) [Citation106]. In countries where vaccination is already in the NIP, several factors can potentially contribute to global Hib eradication: an improved herd effect and control of carriage by the use of effective vaccines and schedules, tailored to the specificities of each country and ethnic groups; continued surveillance (extended to all H. influenzae strains) and improvement in vaccination coverage by raising awareness on the disease among both healthcare practitioners and the general population (). When implemented globally, these measures will bring us within reach of disease elimination and open prospects for eradication of Hib. Current efforts are impressive, such as the proposed Defeating meningitis by 2030 plan by the Meningitis Research Foundation in collaboration with the WHO [Citation107], calling for action in the prevention, control and surveillance of causative pathogens, including Hib, and underlining the need for improvements in diagnosis, treatment and support for those impacted by the disease [Citation108].
Figure 5. Hib disease: improved control leading toward elimination
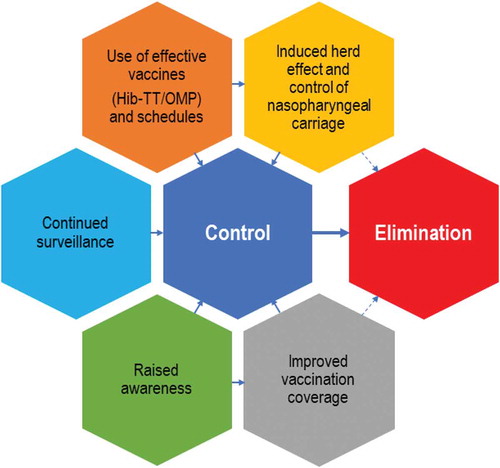
The use of 2 + 1 and 3 + 1 schedules for Hib vaccination is justified by current effectiveness data, along with programmatic fit in the infant immunization programs. However, close monitoring is needed to ensure that herd protection is sustained, in order to maintain current levels of disease control and to reduce Hib risk to young infants whose immunization is not completed. However, reemerging cases of Hib disease even in infants, despite highly effective vaccination, are concerning. These might indirectly be due to the lack of a booster dose at older age, which would otherwise afford greater herd protection; however, evidence on this point remains unclear. For instance, in a study in Sweden assessing the prevalence of IgG antibodies against Hib in the general population, median anti-PRP antibody concentrations were similar at 5 and 15 years post-introduction of mass vaccination, while the proportion above the cutoff for protection decreased in individuals 2–19 years old and increased in adults [Citation109]. The decreasing trend observed in the younger age groups may be explained by a lack of natural boosting, as exposure to the pathogen continues to decrease with the decline in Hib incidence and carriage. These observations indicating a variation over time in protective antibody levels in Sweden are probably applicable to other countries and warrant close monitoring. However, so far, there is no conclusive evidence that a booster dose at older ages is needed.
Maintaining low Hib carriage rates among all age groups brings us one step closer toward interruption of transmission, as nasopharyngeal carriage is currently the most important source of Hib infection. Despite an obvious reduction in Hib carriage rates induced by Hib vaccination, more evidence of indirect effects and their duration in groups not targeted for vaccination is needed. While indirect protection afforded by herd effect has been documented in several countries [Citation30,Citation110,Citation111,Citation112,Citation113], there are not sufficient data to fully understand its role in maintaining the high level of disease control achieved so far, especially in view of the difference in immunogenicity profiles of Hib-TT and Hib-OMP vaccines and the increasing use of the 2 + 1 schedules.
Sporadic cases which may lead to outbreaks are still documented, emphasizing the need for on-going epidemiological and microbiological surveillance of invasive H. influenzae infection cases, including typing of strains to identify Hib. It is also vitally important to maintain high levels of vaccination coverage, and to use effective vaccines and schedules against Hib carriage and Hib disease. To raise awareness, and ultimately increase vaccination uptake, healthcare providers should advocate Hib vaccination to parents, including by educating them on the severity of the disease and potential long-term sequalae. However, new generation physicians may have low awareness of Hib infections, due to the reduced number of clinical cases in the post-vaccine era. Therefore, periodic education programs should be considered by health authorities. Raising awareness becomes more and more important, as in recent decades, vaccine hesitancy has led to an overall decrease in vaccination coverage rates [Citation114,Citation115], underlying localized increases of otherwise controlled childhood diseases such as measles or poliomyelitis, which is on the brink of eradication [Citation116,Citation117].
Continuous surveillance remains paramount in order to inform future vaccination strategies, including those targeting non-b serotypes and NTHi, which have become more important as Hib disease incidence has declined.
In the future Hib vaccination could benefit from several approaches. First, the use of larger vaccine combinations would reduce the number of injections and thus improve acceptability by the public. Hexavalent combinations are currently approved for use, but any future higher-valent formulations should be carefully assessed to reduce the risk of altered immune response (as previously documented for Hib and DTaP components) [Citation77] and of a potential increase in the incidence of adverse events following vaccination. Second, improved or alternative methods of administering vaccines should be considered. Recent studies have shown that a potent immune response can be induced by intradermal vaccination, and that dose-sparing can be achieved [Citation118]. To date, no Hib vaccine has been evaluated for intradermal administration, although the advantages and potential downsides of this delivery method have been discussed [Citation119].
Following the success in controlling Hib-caused disease, future efforts should also concentrate on the prevention of all types of H. influenzae disease. To date, there are no licensed vaccines against NTHi or Hia, which have become more relevant in the context of decreased Hib incidence. An increase in NTHi invasive infections has been documented among the elderly, a population at high risk of acquiring the disease and developing complications due to immune-senescence and underlying comorbidities. There is little prospect of a NTHi vaccine because of the marked heterogeneity of NTHi strains. The development of a vaccine against Hia is of particular concern for Indigenous communities in the northern regions of Canada and Alaska, for which an emergence of Hia infections has been observed over the last decades [Citation40]. A capsular polysaccharide-based glycoconjugate vaccine has shown promising results, but its development is still in very early stages [Citation120].
With Hib vaccination being implemented in >90% of countries worldwide, efforts to eliminate Hib disease should focus on improving vaccination coverages, with a special focus on reducing vaccine hesitancy. Continuous surveillance of Hib incidence and carriage is paramount for the prediction and timely prevention of future reemergence of the disease.
Article highlights
Invasive Hib disease incidence declined dramatically after implementation of routine Hib vaccination
Conjugate vaccines (Hib-TT, Hib-OMP) are currently used in national immunization programs, generally as combination vaccines
Despite reduced incidence and low carriage rates, cases of invasive Hib disease continue to occur sporadically and need to be addressed
While >90% of countries are currently implementing Hib vaccination, coverage needs to be improved by raising awareness of Hib disease and reducing vaccine hesitancy
Prevention strategies for all H. influenzae invasive infections need to be developed, as other strains continue to cause disease
Author contributions
All authors performed literature searches, contributed in the interpretation of the data and participated in the development and the review of the manuscript. All authors approved the final submitted version
Author disclosures
M Slack has received personal fees from GlaxoSmithKline, Pfizer, AstraZeneca and Sanofi Pasteur as a speaker at international meetings and as a member of advisory boards, and has undertaken contract work for Pfizer. S Esposito reports personal fees from the GlaxoSmithKline group of companies. H Haas has nothing to disclose. L Harrington is employee of the GlaxoSmithKline group of companies. A Mihalyi, M Nissen and P Mukherjee are employees of and hold shares in the GlaxoSmithKline group of companies. Publication support was utilized in the production of this manuscript: P M Petrar (Modis c/o GlaxoSmithKline) provided medical writing support and F Danhier (Modis c/o GlaxoSmithKline) provided coordination of the manuscript development and editorial support, which was funded by GlaxoSmithKline Biologicals SA. The authors have no other relevant affiliations or financial involvement with any organization or entity with a financial interest in or financial conflict with the subject matter or materials discussed in the manuscript apart from those disclosed.
Declaration of interest
M Slack has received personal fees from GSK, Pfizer, AstraZeneca and Sanofi Pasteur as a speaker at international meetings and as a member of advisory boards, and has undertaken contract work for Pfizer. S Esposito reports personal fees from the GSK group of companies. H Haas has nothing to disclose. L Harrington is employee of the GSK group of companies. A Mihalyi, M Nissen and P Mukherjee are employees of and hold shares in the GSK group of companies. Publication support was utilized in the production of this manuscript: P M Petrar (Modis c/o GSK) provided medical writing support and F Danhier (Modis c/o GSK) provided coordination of the manuscript development and editorial support, which was funded by GlaxoSmithKline Biologicals SA. The authors have no other relevant affiliations or financial involvement with any organization or entity with a financial interest in or financial conflict with the subject matter or materials discussed in the manuscript apart from those disclosed.
Reviewer disclosures
Peer reviewers on this manuscript have no relevant financial or other relationships to disclose.
Trademark statement
Infanrix hexa, Menitorix, MenHibrix and Infanrix-IPV/Hib are trademarks of the GSK group of companies. Hexacima/Hexyon/Hexaxim and Pentacel are trademarks of Sanofi Pasteur. Vaxelis is trademark of MCM Vaccine Co.
Supplemental Material
Download Zip (32.9 KB)Acknowledgements
Authors thank the Modis platform for editorial assistance and manuscript coordination, on behalf of GSK. Petronela M. Petrar provided medical writing support and Fabienne Danhier coordinated the manuscript development and editorial support.
Supplementary material
Supplemental data for this article can be accessed here.
Additional information
Funding
References
- World Health Organization. Haemophilus influenzae type b (Hib) vaccination position paper - July 2013. Wkly Epidemiol Rec. 2013;88(39):413–426.
- World Health Organization. Haemophilus influenzae type b (Hib) meningitis in the pre-vaccine era: a global review of incidence, age distributions, and case-fatality rates. 2002. [ cited 2019 Nov 18]. Available from: http://apps.who.int/iris/bitstream/handle/10665/67572/WHO_V-B_02.18_eng.pdf.
- Wahl B, O’Brien KL, Greenbaum A, et al., Burden of Streptococcus pneumoniae and Haemophilus influenzae type b disease in children in the era of conjugate vaccines: global, regional, and national estimates for 2000-15. Lancet Glob Health. 6(7): e744–e757. 2018. .
- Edmond K, Clark A, Korczak VS, et al. Global and regional risk of disabling sequelae from bacterial meningitis: A systematic review and meta-analysis. Lancet Infect Dis. 2010;10(5):317–328. .
- Tristram S, Jacobs MR, Appelbaum PC. Antimicrobial resistance in Haemophilus influenzae. Clin Microbiol Rev. 2007;20(2):368–389.
- Van Eldere J, Slack MP, Ladhani S, et al. Non-typeable Haemophilus influenzae, an under-recognised pathogen. Lancet Infect Dis. 2014;14(12):1281–1292. .
- Su PY, Huang AH, Lai CH, et al. Extensively drug-resistant Haemophilus influenzae - emergence, epidemiology, risk factors, and regimen. BMC Microbiol. 2020;20(1):102.
- Kayhty H, Peltola H, Karanko V, et al. The protective level of serum antibodies to the capsular polysaccharide of Haemophilus influenzae type b. J Infect Dis. 1983;147(6):1100.
- Anderson P. The protective level of serum antibodies to the capsular polysaccharide of Haemophilus influenzae type b. J Infect Dis. 1984;149(6):1034–1035.
- Fernandez J, Levine OS, Sanchez J, et al. Prevention of Haemophilus influenzae type b colonization by vaccination: correlation with serum anti-capsular IgG concentration. J Infect Dis. 2000;182(5):1553–1556.
- Zarei AE, Almehdar HA, Redwan EM. Hib vaccines: past, present, and future perspectives. J Immunol Res. 2016;2016:7203587.
- Peltola H. Worldwide Haemophilus influenzae type b disease at the beginning of the 21st century: global analysis of the disease burden 25 years after the use of the polysaccharide vaccine and a decade after the advent of conjugates. Clin Microbiol Rev. 2000;13(2):302–317.
- World Health Organization. Immunological basis for immunization: haemophilus influenzae type b vaccines. 2007. [ cited 2019 Nov 18]. Available from: http://apps.who.int/iris/handle/10665/43799.
- World Health Organization. WHO vaccine-preventable diseases: monitoring system. 2019 global summary. 2019. [ cited 2020 Jan 17]. Available from: http://apps.who.int/immunization_monitoring/globalsummary/schedules.
- World Health Organization. Immunization coverage. Key facts. 2019. [ cited 2019 Jul 20]. Available from: http://www.who.int/news-room/fact-sheets/detail/immunization-coverage.
- European Centre for Disease Prevention and Control. ECDC. Annual epidemiological report for 2017. In: Haemophilus influenzae. Stockholm: ECDC; 2019.
- Bajanca-Lavado MP, Bettencourt C, Cunha F, et al. Haemophilus influenzae serotype b vaccine failure in Portugal: A new threat? OC- EMGM2019-13331. 15th EMGM Congress, 27-30 May 2019; Lisbon, Portugal.
- Hong E, Terrade A, Denizon M, et al. Recent evolution of the epidemiology of Haemophilus influenzae type b and seroprevalence in France. OC EMGM2019-13190. 15th EMGM Congress, 27-30 May 2019; Lisbon, Portugal.
- WHO position paper on Haemophilus influenzae type b conjugate vaccines. Wkly Epidemiol Rec. WHO; 2006;81(47):445–452.
- Progress introducing Haemophilus influenzae type b vaccine in low-income countries, 2004-2008. Wkly Epidemiol Rec. 2008;83(7):61–67.
- Sambala EZ, Wiyeh AB, Ngcobo N, et al. New vaccine introductions in Africa before and during the decade of vaccines - Are we making progress? Vaccine. 2019;37(25):3290–3295.
- Nanduri SA, Sutherland AR, Gordon LK, et al. Haemophilus influenzae type b vaccines. In: Plotkin’s Vaccines. Elsevier; 2018. p. 301–318. e310.
- Walls T, Cho V, Martin N, et al., Vaccine impact on long-term trends in invasive bacterial disease in New Zealand children. Pediatr Infect Dis J. 37(10): 1041–1047. 2018. .
- Martinelli D, Azzari C, Bonanni P, et al. Impact of Haemophilus influenzae type b conjugate vaccination on hospitalization for invasive disease in children fifteen years after its introduction in Italy. Vaccine. 2017;35(46):6297–6301. .
- Soeters HM, Blain A, Pondo T, et al., Current epidemiology and trends in invasive Haemophilus influenzae disease-United States, 2009-2015. Clin Infect Dis. 67(6): 881–889. 2018. .
- Centers for Disease Control and Prevention. Active Bacterial Core Surveillance Report. Emerging Infections Program Network. In: Haemophilus influenza. 2017. 2019 Aug 1 cited]. Available from http://www.cdc.gov/abcs/reports-findings/survreports/hib17.html
- Wang S, Tafalla M, Hanssens L, et al. A review of Haemophilus influenzae disease in Europe from 2000-2014: challenges, successes and the contribution of hexavalent combination vaccines. Expert Rev Vaccines. 2017;16(11):1095–1105. .
- European Centre for Disease Prevention and Control. Surveillance Atlas of Infectious Diseases. Invasive Haemophilus influenzae disease. Serotype b reported cases. 2019. [ cited 2020 Jan 17]. Available from: http://atlas.ecdc.europa.eu/public/index.aspx.
- Dey A, Knox S, Wang H, et al. Summary of national surveillance data on vaccine preventable diseases in Australia, 2008-2011. Commun Dis Intell Q Rep. 2016;40(Suppl):S1–70.
- Adegbola RA, Secka O, Lahai G, et al. Elimination of Haemophilus influenzae type b (Hib) disease from The Gambia after the introduction of routine immunisation with a Hib conjugate vaccine: a prospective study. Lancet. 2005;366(9480):144–150. .
- Howie SR, Oluwalana C, Secka O, et al. The effectiveness of conjugate Haemophilus influenzae type B vaccine in The Gambia 14 years after introduction. Clin Infect Dis. 2013;57(11):1527–1534. .
- Oluwalana C, Howie SR, Secka O, et al. Incidence of Haemophilus influenzae type b disease in The Gambia 14 years after introduction of routine Haemophilus influenzae type b conjugate vaccine immunization. J Pediatr. 2013;163(1 Suppl):S4–7. .
- Zaman SM, Howie SR, Ochoge M, et al. Impact of routine vaccination against Haemophilus influenzae type b in The Gambia: 20 years after its introduction. J Glob Health. 2020;10(1):010416. .
- Hammitt LL, Crane RJ, Karani A, et al. Effect of Haemophilus influenzae type b vaccination without a booster dose on invasive H influenzae type b disease, nasopharyngeal carriage, and population immunity in Kilifi, Kenya: a 15-year regional surveillance study. Lancet Glob Health. 2016;4(3):e185–194. .
- Wall EC, Everett DB, Mukaka M, et al. Bacterial meningitis in Malawian adults, adolescents, and children during the era of antiretroviral scale-up and Haemophilus influenzae type b vaccination, 2000-2012. Clin Infect Dis. 2014;58(10):e137–145.
- Suga S, Ishiwada N, Sasaki Y, et al., A nationwide population-based surveillance of invasive Haemophilus influenzae diseases in children after the introduction of the Haemophilus influenzae type b vaccine in Japan. Vaccine. 36(38): 5678–5684. 2018. .
- Thoon KC, Tee NW, Chew L, et al. Near disappearance of childhood invasive Haemophilus influenzae type b disease in Singapore. Vaccine. 2014;32(44):5862–5865.
- World Health Organization. Reported estimates of Hib3 coverage. Last updated 10 December 2019. [ cited 2020 Jan 14]. Available from: http://apps.who.int/immunization_monitoring/globalsummary/timeseries/tscoveragehib3.html.
- Blain A, MacNeil J, Wang X, et al. Invasive Haemophilus influenzae disease in adults ≥65 years, United States, 2011. Open Forum Infect Dis. 2014;1(2):ofu044.
- Cox AD, Barreto L, Ulanova M, et al. Developing a vaccine for Haemophilus influenzae serotype a: proceedings of a workshop. Can Commun Dis Rep. 2017;43(5):89–95.
- Whittaker R, Economopoulou A, Dias JG, et al. Epidemiology of invasive Haemophilus influenzae disease, Europe, 2007-2014. Emerg Infect Dis. 2017;23(3):396–404.
- Adam HJ, Richardson SE, Jamieson FB, et al. Changing epidemiology of invasive Haemophilus influenzae in Ontario, Canada: evidence for herd effects and strain replacement due to Hib vaccination. Vaccine. 2010;28(24):4073–4078.
- Ribeiro GS, Reis JN, Cordeiro SM, et al. Prevention of Haemophilus influenzae type b (Hib) meningitis and emergence of serotype replacement with type a strains after introduction of Hib immunization in Brazil. J Infect Dis. 2003;187(1):109–116.
- Ladhani S, Ramsay ME, Chandra M, et al. No evidence for Haemophilus influenzae serotype replacement in Europe after introduction of the Hib conjugate vaccine. Lancet Infect Dis. 2008;8(5):275–276.
- Ladhani S, Slack MP, Heath PT, et al. Invasive Haemophilus influenzae disease, Europe, 1996-2006. Emerg Infect Dis. 2010;16(3):455–463.
- Gentile A, Martinez AC, Juarez MDV, et al. Haemophilus influenzae type B meningitis: is there a re-emergence? 24 years of experience in a children’s hospital. Arch Argent Pediatr. 2017;115(3):227–233.
- Shah RK, Roberson DW, Jones DT. Epiglottitis in the Hemophilus influenzae type b vaccine era: changing trends. Laryngoscope. 2004;114(3):557–560.
- European Centre for Disease Prevention and Control. ECDC. Annual epidemiological report for 2016. In: Haemophilus influenzae. Stockholm: ECDC; 2018.
- Garcia S, Ciriaci C, Montes G, et al. [Epiglottitis due to Haemophilus influenzae type b in the vaccination era: pediatric clinical case]. Arch Argent Pediatr. 2019;117(4):e403–e405.
- Heath PT. Haemophilus influenzae type b conjugate vaccines: A review of efficacy data. Pediatr Infect Dis J. 1998;17(9 Suppl):S117–122.
- Mulholland EK, Adegbola RA, Gambian T. Haemophilus influenzae type b vaccine trial: what does it tell us about the burden of Haemophilus influenzae type b disease? Pediatr Infect Dis J. 1998;17(9 Suppl):S123–125.
- Eskola J, Ward J, Dagan R, et al. Combined vaccination of Haemophilus influenzae type b conjugate and diphtheria-tetanus-pertussis containing acellular pertussis. Lancet. 1999;354(9195):2063–2068. .
- Kalies H, Verstraeten T, Grote V, et al. Four and one-half-year follow-up of the effectiveness of diphtheria-tetanus toxoids-acellular pertussis/Haemophilus influenzae type b and diphtheria-tetanus toxoids-acellular pertussis-inactivated poliovirus/H. influenzae type b combination vaccines in Germany. Pediatr Infect Dis J. 2004;23(10):944–950.
- World Health Organization. WHO prequalified vaccines (last updated June 2020). [ cited 2020 Jun 12]. Available from: https://extranet.who.int/gavi/PQ_Web/Default.aspx?nav=2.
- Bulkow LR, Wainwright RB, Letson GW, et al. Comparative immunogenicity of four Haemophilus influenzae type b conjugate vaccines in Alaska Native infants. Pediatr Infect Dis J. 1993;12(6):484–492.
- Granoff DM, Anderson EL, Osterholm MT, et al. Differences in the immunogenicity of three Haemophilus influenzae type b conjugate vaccines in infants. J Pediatr. 1992;121(2):187–194.
- Wetzler LM. Innate immune function of the neisserial porins and the relationship to vaccine adjuvant activity. Future Microbiol. 2010;5(5):749–758.
- Decker MD, Edwards KM, Bradley R, et al. Comparative trial in infants of four conjugate Haemophilus influenzae type b vaccines. J Pediatr. 1992;120(2 Pt 1):184–189.
- Carlsson RM, Claesson BA, Iwarson S, et al. Antibodies against Haemophilus influenzae type b and tetanus in infants after subcutaneous vaccination with PRP-T/diphtheria, or PRP-OMP/diphtheria-tetanus vaccines. Pediatr Infect Dis J. 1994;13(1):27–33.
- Vesikari T, Becker T, Vertruyen AF, et al. A phase III randomized, double-blind, clinical trial of an investigational hexavalent vaccine given at two, three, four and twelve months. Pediatr Infect Dis J. 2017;36(2):209–215.
- Silfverdal SA, Icardi G, Vesikari T, et al. A phase III randomized, double-blind, clinical trial of an investigational hexavalent vaccine given at 2, 4, and 11-12 months. Vaccine. 2016;34(33):3810–3816.
- Carlsson RM, Claesson B A, Lagergard T, et al. Serum antibodies against Haemophilus influenzae type b and tetanus at 2.5 years of age: A follow-up of 2 different regimens of infant vaccination. Scand J Infect. 1996;28(5):519–523.
- Madhi SA, Lopez P, Zambrano B, et al. Antibody persistence in pre-school children after hexavalent vaccine infant primary and booster administration. Hum Vaccin Immunother. 2019;15(3):658–668.
- Zinke M, Disselhoff J, Gartner B, et al. Immunological persistence in 4-6 and 7-9 year olds previously vaccinated in infancy with hexavalent DTPa-HBV-IPV/Hib. Hum Vaccin. 2010;6(2):189–193.
- Schlesinger Y, Granoff DM. Avidity and bactericidal activity of antibody elicited by different Haemophilus influenzae type b conjugate vaccines. The vaccine study group. JAMA. 1992;267(11):1489–1494.
- Agbarakwe AE, Griffiths H, Begg N, et al. Avidity of specific IgG antibodies elicited by immunisation against Haemophilus influenzae type b. J Clin Pathol. 1995;48(3):206–209.
- Low N, Redmond SM, Rutjes AW, et al. Comparing Haemophilus influenzae type b conjugate vaccine schedules: a systematic review and meta-analysis of vaccine trials. Pediatr Infect Dis J. 2013;32(11):1245–1256.
- Thumburu KK, Singh M, Das RR, et al. Two or three primary dose regime for Haemophilus influenzae type b conjugate vaccine: meta-analysis of randomized controlled trials. Ther Adv Vaccines. 2015;3(2):31–40.
- Watt J, Chen S, Santosham M Haemophilus influenzae type b conjugate vaccine: review of observational data on long term vaccine impact to inform recommendations for vaccine schedules, Geneva, World Health Organization. 2012. [ cited 2020 Feb 25]. Available from: http://www.who.int/immunization/sage/meetings/2012/november/5_Review_observational_data_long_term_vaccine_impact_recommendations_vaccine_schedules_Watt_J_et_al_2012.pdf.
- Heath PT, Booy R, Azzopardi HJ, et al. Antibody concentration and clinical protection after Hib conjugate vaccination in the United Kingdom. JAMA. 2000;284(18):2334–2340.
- Ladhani S, Slack MP, Heys M, et al. Fall in Haemophilus influenzae serotype b (Hib) disease following implementation of a booster campaign. Arch Dis Child. 2008;93(8):665–669.
- Collins S, Litt D, Almond R, et al. Haemophilus influenzae type b (Hib) seroprevalence and current epidemiology in England and Wales. J Infect. 2018;76(4):335–341.
- Public Health England. Laboratory reports of Haemophilus influenzae type b infection by age group and quarter: england, 1990-2014. In: Haemophilus influenzae: epidemiological data. 2015. 2020 Jan 17 cited]. Available from http://assets.publishing.service.gov.uk/government/uploads/system/uploads/attachment_data/file/407091/Laboratory_reports_of_Haemophilus_influenzae_type_b_infection_by_age_group_and_quarter_England_1990_-_2014.pdf
- European Centre for Disease Prevention and Control. Haemophilus Influenzae type B infection: recommended vaccinations. [ cited 2020 Jul 29]. Available from: http://vaccine-schedule.ecdc.europa.eu/.
- Charania NA, Moghadas SM. Modelling the effects of booster dose vaccination schedules and recommendations for public health immunization programs: the case of Haemophilus influenzae serotype b. BMC Public Health. 2017;17(1):705.
- Ladhani SN. Two decades of experience with the Haemophilus influenzae serotype b conjugate vaccine in the United Kingdom. Clin Ther. 2012;34(2):385–399. .
- Borrow R, Dagan R, Zepp F, et al. Glycoconjugate vaccines and immune interactions, and implications for vaccination schedules. Expert Rev Vaccines. 2011;10(11):1621–1631.
- Voysey M, Sadarangani M, Clutterbuck E, et al. The impact of administration of conjugate vaccines containing cross reacting material on Haemophilus influenzae type b antibody responses in infants: a systematic review and meta-analysis of randomised controlled trials. Vaccine. 2016;34(34):3986–3992.
- Moro PL, Jankosky C, Menschik D, et al. Adverse events following Haemophilus influenzae type b vaccines in the vaccine adverse event reporting system, 1990-2013. J Pediatr. 2015;166(4):992–997.
- Granoff DM, Daum RS. Spread of Haemophilus influenzae type b: recent epidemiologic and therapeutic considerations. J Pediatr. 1980;97(5):854–860.
- Morris SK, Moss WJ, Halsey N. Haemophilus influenzae type b conjugate vaccine use and effectiveness. Lancet Infect Dis. 2008;8(7):435–443.
- de Carvalho CX, Kipnis A, Thörn L, et al. Carriage of Haemophilus influenzae among Brazilian children attending day care centers in the era of widespread Hib vaccination. Vaccine. 2011;29(7):1438–1442. .
- McVernon J, Howard AJ, Slack MP, et al. Long-term impact of vaccination on Haemophilus influenzae type b (Hib) carriage in the United Kingdom. Epidemiol Infect. 2004;132(4):765–767.
- DeAntonio R, Amador S, Bunge EM, et al. Vaccination herd effect experience in Latin America: a systematic literature review. Hum Vaccin Immunother. 2019;15(1):49–71.
- McVernon J, Trotter CL, Slack MP, et al. Trends in Haemophilus influenzae type b infections in adults in England and Wales: surveillance study. BMJ. 2004;329(7467):655–658.
- Michaels RH, Norden CW. Pharyngeal colonization with Haemophilus influenzae type b: a longitudinal study of families with a child with meningitis or epiglottitis due to H. influenzae type b. J Infect Dis. 1977;136(2):222–228.
- Giufre M, Daprai L, Cardines R, et al. Carriage of Haemophilus influenzae in the oropharynx of young children and molecular epidemiology of the isolates after fifteen years of H. influenzae type b vaccination in Italy. Vaccine. 2015;33(46):6227–6234.
- Zanella RC, Brandileone MC, Andrade AL, et al. Evaluation of Haemophilus influenzae type b carrier status among children 10 years after the introduction of Hib vaccine in Brazil. Mem Inst Oswaldo Cruz. 2015;110(6):755–759. .
- Singleton R, Hammitt L, Hennessy T, et al. The Alaska Haemophilus influenzae type b experience: lessons in controlling a vaccine-preventable disease. Pediatrics. 2006;118(2):e421–429.
- Menzies RI, Bremner KM, Wang H, et al. Long-term trends in invasive Haemophilus influenzae type b disease among indigenous Australian children following use of PRP-OMP and PRP-T vaccines. Pediatr Infect Dis J. 2015;34(6):621–626.
- Tsang RS, Bruce MG, Lem M, et al., A review of invasive Haemophilus influenzae disease in the Indigenous populations of North America. Epidemiol Infect. 142(7): 1344–1354. 2014. .
- Jacups SP, Morris PS, Leach AJ. Haemophilus influenzae type b carriage in Indigenous children and children attending childcare centers in the Northern Territory, Australia, spanning pre- and post-vaccine eras. Vaccine. 2011;29(16):3083–3088.
- Cleland G, Leung C, Wan Sai Cheong J, et al. Paediatric invasive Haemophilus influenzae in Queensland, Australia, 2002-2011: young Indigenous children remain at highest risk. J Paediatr Child Health. 2018;54(1):36–41.
- Diseases AAoPCoI. Red book: report of the Committee on Infectious Diseases. Elk Grove Village, IL: American Academy of Pediatrics; 2006.
- Briere EC, Rubin L, Moro PL, et al. Prevention and control of Haemophilus influenzae type b disease: recommendations of the advisory committee on immunization practices (ACIP). MMWR Recomm Rep. 2014;63(RR–01):1–14.
- Kristensen K. Antibody response to a Haemophilus influenzae type b polysaccharide tetanus toxoid conjugate vaccine in splenectomized children and adolescents. Scand J Infect Dis. 1992;24(5):629–632.
- Meerveld-Eggink A, de Weerdt O, van Velzen-blad H, et al. Response to conjugate pneumococcal and Haemophilus influenzae type b vaccines in asplenic patients. Vaccine. 2011;29(4):675–680.
- Feldman S, Gigliotti F, Shenep JL, et al. Risk of Haemophilus influenzae type b disease in children with cancer and response of immunocompromised leukemic children to a conjugate vaccine. J Infect Dis. 1990;161(5):926–931. .
- Robertson JD, Nagesh K, Jowitt SN, et al. Immunogenicity of vaccination against influenza, Streptococcus pneumoniae and Haemophilus influenzae type b in patients with multiple myeloma. Br J Cancer. 2000;82(7):1261–1265. .
- Mangtani P, Mulholland K, Madhi SA, et al. Haemophilus influenzae type b disease in HIV-infected children: a review of the disease epidemiology and effectiveness of Hib conjugate vaccines. Vaccine. 2010;28(7):1677–1683. .
- Baxter D. Vaccine responsiveness in premature infants. Hum Vaccin. 2010;6(6):506–511.
- Heath PT, Booy R, McVernon J, et al. Hib vaccination in infants born prematurely. Arch Dis Child. 2003;88(3):206–210. .
- Bednarek A, Bartkowiak-Emeryk M, Klepacz R, et al. Persistence of vaccine-induced immunity in preschool children: effect of gestational age. Med Sci Monit. 2018;24:5110–5117.
- Omenaca F, Garcia-Sicilia J, Boceta R, et al. Antibody persistence and booster vaccination during the second and fifth years of life in a cohort of children who were born prematurely. Pediatr Infect Dis J. 2007;26(9):824–829. 10.4161/hv.6.2.10117.
- Omenaca F, Vazquez L, Garcia-Corbeira P, et al. Immunization of preterm infants with GSK’s hexavalent combined diphtheria-tetanus-acellular pertussis-hepatitis B-inactivated poliovirus-Haemophilus influenzae type b conjugate vaccine: A review of safety and immunogenicity. Vaccine. 2018;36(7):986–996.
- Yang Y, Yang Y, Scherpbier RW, et al. Coverage of Haemophilus influenzae type b conjugate vaccine for children in mainland China: systematic review and meta-analysis. Pediatr Infect Dis J. 2019;38(3):248–252. .
- Meningitis Research Foundation (MRF). Global plan to defeat meningitis gets go ahead to progress to World Health Assembly. 18 February 2020. [ cited 2020 Jun 12]. Available from: https://www.meningitis.org/meningitis-2030-plan-agreed-by-who.
- The Lancet. A new roadmap for meningitis. Lancet. 2020;395(10232):1230.
- Hallander HO, Lepp T, Ljungman M, et al. Do we need a booster of Hib vaccine after primary vaccination? A study on anti-Hib seroprevalence in Sweden 5 and 15 years after the introduction of universal Hib vaccination related to notifications of invasive disease. APMIS. 2010;118(11):878–887.
- Barbour ML, Mayon-White RT, Coles C, et al. The impact of conjugate vaccine on carriage of Haemophilus influenzae type b. J Infect Dis. 1995;171(1):93–98.
- Forleo-Neto E, de Oliveira CF, Maluf EM, et al. Decreased point prevalence of Haemophilus influenzae type b (Hib) oropharyngeal colonization by mass immunization of Brazilian children less than 5 years old with Hib polyribosylribitol phosphate polysaccharide-tetanus toxoid conjugate vaccine in combination with diphtheria-tetanus toxoids-pertussis vaccine. J Infect Dis. 1999;180(4):1153–1158.
- Murphy TV, Pastor P, Medley F, et al. Decreased Haemophilus colonization in children vaccinated with Haemophilus influenzae type b conjugate vaccine. J Pediatr. 1993;122(4):517–523.
- Rushdy A, Ramsay M, Heath PT, et al. Infant Hib vaccination and herd immunity. J Pediatr. 1999;134(2):253–254.
- Gilkey MB, McRee AL, Magnus BE, et al. Vaccination confidence and parental refusal/delay of early childhood vaccines. PLoS One. 2016;11(7):e0159087.
- McClure CC, Cataldi JR, O’Leary ST. Vaccine hesitancy: where we are and where we are going. Clin Ther. 2017;39(8):1550–1562.
- World Health Organization. New measles surveillance data from WHO- Provisional data based on monthly reports to WHO (Geneva) as of August 2019. [ cited 2019 Jul 29]. Available from: http://www.who.int/immunization/newsroom/new-measles-data-august-2019/en/.
- Ali M, Ahmad N, Khan H, et al. Polio vaccination controversy in Pakistan. Lancet. 2019;394(10202):915–916.
- Saitoh A, Aizawa Y. Intradermal vaccination for infants and children. Hum Vaccin Immunother. 2016;12(9):2447–2455.
- Zehrung D, Jarrahian C, Wales A. Intradermal delivery for vaccine dose sparing: overview of current issues. Vaccine. 2013;31(34):3392–3395.
- Cox AD, Williams D, Cairns C, et al., Investigating the candidacy of a capsular polysaccharide-based glycoconjugate as a vaccine to combat Haemophilus influenzae type a disease: A solution for an unmet public health need. Vaccine. 35(45): 6129–6136. 2017. .