ABSTRACT
Introduction
Streptococcus pneumoniae is the leading cause of invasive bacterial disease, globally. Despite antiretroviral therapy, adults infected with human immunodeficiency virus (HIV) are also at high risk of pneumococcal carriage and disease. Pneumococcal conjugate vaccines (PCVs) provide effective protection against vaccine serotype (VT) carriage and disease in children, and have been introduced worldwide, including most HIV-affected low- and middle-income countries. Unlike high-income countries, the circulation of VT persists in the PCV era in some low-income countries and results in a continued high burden of pneumococcal disease in HIV-infected adults. Moreover, no routine vaccination that directly protects HIV-infected adults in such settings has been implemented.
Areas covered
Nonsystematic review on the pneumococcal burden in HIV-infected adults and vaccine strategies to reduce this burden.
Expert opinion
We propose and discuss the relative merit of changing the infant PCV program to use (1a) a two prime plus booster dose schedule, (1b) a two prime plus booster dose schedule with an additional booster dose at school entry, to directly vaccinate (2a) HIV-infected adults or vaccinating (2b) HIV-infected pregnant women for direct protection, with added indirect protection to the high-risk neonates. We identify key knowledge gaps for such an evaluation and propose strategies to overcome them.
KEYWORDS:
1. Introduction
Streptococcus pneumoniae is a major cause of global childhood mortality [Citation1,Citation2], particularly in <5 year-olds, and also causes a high burden of disease among the elderly and human immunodeficiency virus (HIV) infected adults [Citation3–5]. In the last two decades, infant pneumococcal conjugate vaccine (PCV) programs have substantially reduced the burden of invasive pneumococcal disease (IPD) and mortality in vaccinees [Citation2,Citation6–13]. In contrast to high-income countries though [Citation14,Citation15], routine infant PCV programs in the most low-income sub-Saharan African (sSA) countries have led to a less pronounced herd effect with a continued circulation of vaccine serotypes (VT), especially in the unvaccinated adult population [Citation12,Citation16–20] including those with HIV infection and thus those at high risk for pneumococcal disease [Citation21]. For instance, an over a 3.5-year study in Malawi, post-PCV VT carriage in HIV-infected adults only declined from 15.2%, 95%CI, 10.8–20.9 in survey 1 to 8.9% 95%CI 5.7–13.7 in survey 7 [Citation20]. While the underlying reasons have not been fully established they may include a higher infection pressure as a result of more frequent human contacts and lower vaccine uptake [Citation18].
HIV infection can substantially increase the risk of IPD among otherwise healthy older children or adults on antiretroviral therapy (ART) [Citation5,Citation22–24]. This is in part related to the impairment of both the cell-mediated and humoral arms of the immune system [Citation25,Citation26]. Capsule-specific immunoglobulin G (IgG) antibodies as well as T and B cell-mediated protein-specific responses play a central role in the control of pneumococcal colonization and infection [Citation27–29]. However, HIV affects both T and B cell functions, resulting in the impairment of humoral responses to extracellular pathogens such as pneumococci [Citation30–32] and control of pneumococcus at the mucosal level. ART only partially reconstitutes the immune system of HIV-infected individuals by increasing B and T-lymphocyte numbers and functionality [Citation25,Citation27]. Deficiencies in humoral response due to depleted or persistent defects in memory cell function persist after ART initiation and disproportionately so at the mucosal level [Citation33,Citation34]. Thus, HIV-infected individuals on ART remain with impaired antibody responses to natural pneumococcal infections and vaccination [Citation35,Citation36].
In this article, we present a review of the pneumococcal burden in HIV-infected adults in the presence of mature PCV infant programs, particularly in sub-Saharan Africa (sSA). We highlight four options for vaccine strategies that could be implemented to address this disease burden and discuss the key evidence gaps to enable a solution to be identified.
2. Pneumococcal burden in African HIV-infected adults
Without ART, the risk of IPD in HIV-infected adults is reported to be 30–300 times higher than in HIV-uninfected individuals, and with an about sixfold higher risk of recurrence [Citation25,Citation37–39]. Although ART has reduced the risk of IPD and subsequent mortality [Citation3,Citation40], the risk remains more than 30-fold higher than in HIV-uninfected adults [Citation41] with an incidence of >50 cases per 100,000 person years [Citation3,Citation5,Citation42]. The introduction of infant PCV programs in South Africa and Kenya has led to a further reduction in VT-IPD and pneumococcal pneumonia incidence in HIV-infected adults [Citation9,Citation43,Citation44].
HIV prevalence in African adults varies widely, with Swaziland reporting the highest at 27.2% [Citation45]. In 2017, all sSA countries reported >10% national or subnational adult HIV prevalence [Citation45]. It has been observed that countries with suboptimal PCV herd protection and substantial residual vaccine-serotype circulation, like Malawi and Mozambique, also report a high prevalence of HIV [Citation46]. HIV prevalence amongst children has fallen as a consequence of the effective prevention of vertical transmission of HIV and ART use [Citation47–50]. Only a small proportion of infants are infected with HIV and are already included in the PCV infant programs. HIV prevalence remains high as a consequence of improved survival with ART in adults. Thus, a large proportion of adults at high risk of pneumococcal disease attributable to HIV infection will remain a concern in the years to come in sSA.
3. Pneumococcal vaccines in HIV-infected adults
Since the 1980s, the 23-valent pneumococcal polysaccharide vaccine (PPV23) has been approved for use in HIV-infected adults for direct protection against a wide range of serotypes, in most high-income countries where it is reported to be safe, including in HIV-infected but otherwise healthy adults [Citation51–54]. However, evidence of PPV23’s efficacy in HIV-infected adults is somewhat controversial [Citation53,Citation55–60], with the suggestion of potential hyporesponsiveness in individuals with advanced immunosuppression, and a reported increase, albeit not statistically significant, in the incidence of all-cause pneumonia when given to HIV-infected Ugandan adults, not on ART [Citation61]. Estimates of PPV23 efficacy in HIV-infected adults are highly heterogeneous which may be linked to differences in HIV viral load and ART status at the time of vaccination [Citation25,Citation61–64].
PCVs are more immunogenic than PPV23 in HIV-infected adults [Citation23,Citation65] and are highly efficacious in preventing VT-disease in HIV-uninfected children and adults [Citation6,Citation66]. Two formulations, a 10- and a 13-valent product, are currently in use worldwide. They have comparable effectiveness and have been licensed based on their noninferiority to a previous 7-valent formulation (PCV7) [Citation67]. While the efficacy of PCVs against VT-disease in HIV-infected children is somewhat inferior to that of HIV-uninfected children (51% vs 77%) [Citation6], the efficacy against carriage is similar irrespective of HIV status [Citation68]. PCV7 has been shown to be immunogenic [Citation69] and 74% efficacious against VT-IPD in HIV-infected adults, with the highest efficacy within the first 12 months of vaccination even in those with CD4+ count <200 cells/mm3 and with unsuppressed HIV viral load at vaccination [Citation65,Citation70]. Further evidence on PCV13 efficacy of 75% against VT-IPD and 72.8% against VT community-acquired pneumonia in older adults (aged ≥65 years) have been reported in the Netherlands and the United States, respectively [Citation66,Citation71,Citation72].
In many high-income countries PCV is recommended for use as priming of HIV-infected adults followed by a PPV23 booster [Citation52,Citation73,Citation74], which acts only against IPD. This is despite the limited PCV serotype disease incidence in these settings as a result of effective herd effects from infant PCV programs [Citation75]. However, no such pneumococcal immunization program for HIV-infected adults exists in low-income African countries [Citation76], where the highest disease burden exists [Citation2]. Implementation barriers include the high costs of PCV [Citation77] and a limited amount of evidence on effective and cost-effective vaccine strategies to address the high pneumococcal disease burden among HIV-infected adults in Africa.
4. Optimal vaccination strategies
We propose two potential approaches for reducing the disproportionate burden of pneumococcal disease in HIV-infected African adults: through (1) expanded indirect protection or (2) introduction of direct protection. For expanded protection, options include either (1a) changing the three-doses infant PCV schedule to a two prime plus boost schedule with potentially longer-lasting protection and greater herd protection against IPD in HIV-infected adults or (1b) using a three dose prime-boost strategy but with a fourth dose given as an additional booster at school entry to further enhance the duration of protection and thereby limit onward transmission. Direct protection could be achieved by (2a) vaccinating all HIV-infected adults to confer direct protection, or (2b) vaccinating HIV-infected pregnant women which has the additional benefit of protecting the young infant via the transplacental transfer of antibody and reduced maternal exposure .
Figure 1. Schematic of potential pneumococcal vaccine strategies against invasive pneumococcal disease (IPD) in HIV-infected adults through indirect (1) and direct (2) approaches. Change the infant PCV schedule from 3p+0 to 2p+1 to enhance herd immunity against IPD in HIV-infected adults through a single booster dose (1a), change the infant PCV schedule from 3p+0 to 2p+1(+1) to enhance herd immunity against IPD in HIV-infected adults through double booster doses (1b), vaccinate HIV-infected adults for direct protection (2a), vaccinate HIV-infected pregnant women for direct protection, with some indirect protection for their neonates (2b). Option 1a will not require any additional vaccine doses as it simply rearranges the timing of the three-dose schedule. Option 1b will need additional vaccine doses equivalent to the number of 5 -year-old children in a country per year (e.g. about 300,000 doses per annum for Malawi). Option 2a will need one additional dose of PCV and one of PPV for each HIV-infected adult (e.g. assuming revaccination of the 970,000 HIV-infected adults in Malawi (http://aidsinfo.unaids.org/) every 10 years would use about 200,000 doses per annum, although the rate of new HIV infections is lower than that). The last option 2b will need one additional dose of PCV and one of PPV for each HIV-infected pregnant women (e.g. 90,000 doses per annum for the about 45,000 HIV-infected pregnant women in Malawi each year). Options 1a and 1b are likely to have large impact because of their potential to elicit herd immunity, however, to date it is not well established that herd immunity would indeed be enhanced through a booster dose schedule (several trials are under way to assert this). Option 1b offers less uncertainty due to extra dose included. Option 2a only provides direct protection against vaccine serotypes to HIV-infected adults while 2b will see only a small subset of that vaccinated. There is limited uncertainty for the impact of the latter two strategies as PCV’s efficacy is relatively well established in the two groups
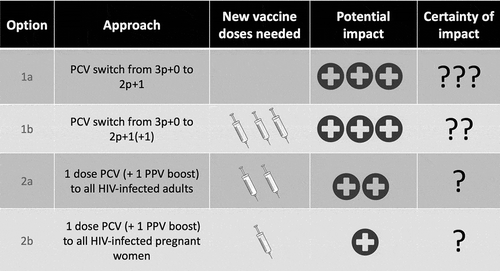
4.1. (1a) Switch from 3p+0 to 2p+1 PCV schedule to potentially increase herd protection
The World Health Organization currently recommends a three dose schedule administered either as three doses in early infancy (3p+0) or as two infant priming doses followed by a booster typically at 9–12 months (2p+1) [Citation67]. In contrast to high-income countries, the vast majority of Africa opted for a 3p+0 schedule based on prioritization of direct protection against the high disease burden in early childhood, alignment with other routine immunizations, and concerns about potentially low booster dose coverage in a 2p+1 schedule. A notable exception is South Africa where the 2p+1 PCV schedule was introduced in 2009 with a booster dose at 9 months to align with measles vaccine dose 1 [Citation8,Citation9,Citation78].
In theory, a third dose given as a booster to 9–12 month olds elicits high levels of an antibody that extend protection against VT-carriage acquisition into early or preschool age and hence further interrupt transmission in one of the key age groups for pneumococcal spread [Citation79,Citation80]. While trials to test this hypothesis is ongoing [Citation81,Citation82], there is currently no clear evidence to confirm that a 2p+1 schedule would induce superior herd protection in those African countries that have been using a 3p+0 with unsatisfactory indirect effects [Citation67].
For now, a conclusive comparison of the differential impact of the two vaccine schedules across countries is hindered by the strong correlation between vaccine schedule and pneumococcal transmission intensity. Most high-income countries have been using 2p+1, and most African countries, typically with higher overall carriage prevalence, have been using 3p+0. Exceptions to this include South Africa introducing PCV in a 2p+1 schedule and Australia using a 3p+0 (but switching to a 2p+1 as a result of breakthrough disease in the PCV13 era) [Citation83,Citation84]. South Africa has experienced substantial reductions in VT-carriage and IPD in unvaccinated populations including HIV-infected adults [Citation44]. Other low-income African countries like Malawi [Citation16,Citation20], Mozambique [Citation19], and the Gambia [Citation85] have substantially less evidence of herd immunity from their mature PCV infant programs with overall residual VT-carriage prevalence of 13.9%, 19.7%, and 11.4%, respectively [Citation46], at a minimum third dose vaccine coverage rate of ≥81% [Citation20,Citation86,Citation87].
4.2. (1b) Switch from 3p+0 to 2p+1(+1) PCV schedule to increase herd protection
A switch from 3p+0 to a 2p+1 schedule would not have major cost implications. Also, if indeed with the addition of a booster dose herd effect can be enhanced, the impact of such schedule change extends beyond just HIV-infected adults and will benefit other unvaccinated individuals as well. However, herd immunity is the result of a complex interplay of factors including bacterial physiology, booster dose coverage, the average age of pneumococcal carriage, duration of vaccine protection against the carriage, and social mixing patterns [Citation88–90]. Additionally, the high average age of carriage, intense social mixing, and the waning of PCV protection against carriage (estimated half-life is 4–6 years) may imply that, in some settings, older children are a key source of pneumococcal transmission and are not fully protected in a 2p+1 schedule [Citation91,Citation92]. A school entry PCV booster dose may be necessary to interrupt transmission and increase herd protection [Citation91].
4.3. (2a) Vaccinating HIV-infected adults
PCV is more immunogenic than PPV23 [Citation23,Citation57,Citation65,Citation69] and efficacious in preventing recurrent episodes of VT-IPD in HIV-infected adults [Citation65]. As per existing recommendations in many high-income countries [Citation51,Citation52], a single dose of PCV followed by a PPV23 booster for expanded serotype coverage could be given to HIV-infected adults in low-income countries to prevent VT-IPD [Citation73,Citation74]. As PCV is efficacious in vaccinees with low CD4+ cell count [Citation65], it could be given immediately at HIV diagnosis to increase PCV coverage [Citation93]. Since most sSA countries have considerably high ART coverage between 65% and 85% [Citation94], adoption of this strategy will require a carefully considered integration of the proposed HIV-infected adult pneumococcal vaccine program into the mature ART programs to achieve a similar high coverage in the midst of competing health priorities [Citation49,Citation50,Citation95,Citation96]. In order to optimize local resources, PCV/PPV23 could concurrently be given with ART to HIV-infected adults [Citation97].
PCV-mediated protection against IPD in HIV-infected adults has been reported to wane rapidly beyond 12 months, especially in those with low CD4+ cell count [Citation65]. Revaccination with PCV or PPV is feasible [Citation98], but of unproven clinical value. Choosing a PPV booster repeated may seem more rational as it is a cheaper vaccine and has higher serotype coverage, but also carries theoretical risks of generating hyporesponsiveness. This approach may also have additional indirect effect benefits if a PCV + PPV strategy could be shown to reduce carriage in this population. HIV-infected adults typically have higher pneumococcal carriage prevalence than HIV-uninfected adults [Citation99,Citation100], and may be part of a reservoir for residual VT circulation in high HIV prevalence sSA settings. Whilst there is no substantial evidence to suggest they sustain transmission, they consequently represent a disproportionate health burden [Citation101–103].
4.4. (2b) Vaccinating HIV-infected pregnant women
Where there are insufficient resources for targeting all HIV-infected adults, HIV-infected pregnant women could be prioritized for PCV protection. Their vaccination would come with the added benefit of indirect protection of their infant and hence would be particularly relevant in settings where the benefits of herd immunity from PCV pediatric programs are limited or neonatal acquisition of VT pneumococcal carriage commonly occurs before the infant can be directly protected by vaccination [Citation46]. Transmission of pneumococci from HIV-infected mothers to their children has been reported [Citation101,Citation102], and this is also likely to be seen in infants not yet eligible for PCV vaccination; e.g. >40% of the infants in the Gambia [Citation104,Citation105], and Kenya [Citation106,Citation107] were reported to acquire pneumococci by the age of 4 weeks. Although HIV infection is reported to reduce the efficiency of maternal antibody transfer to the infant [Citation108], maternal vaccination may still be useful since the (reduced) transfer ratio is applied to a higher vaccine-induced anti-pneumococcal capsular IgG in pregnancy. Thus, vaccination may directly protect the mother and fetus against pneumococcal carriage and disease [Citation105,Citation107,Citation109], and interrupt VT transmission between mother and neonate, thereby providing cocooning immunity during neonatal life.
Maternal PCV vaccination has been shown to be safe. No, serious adverse pregnancy-associated outcomes has been reported from clinical trials where the average gestation at vaccination was between 27 and 38 weeks [Citation110]. However, data on the IPD burden in neonates and mothers, as well as the benefits of maternal immunization from low-income countries are limited. A Cochrane systematic review highlighted that there is insufficient evidence to assess whether pneumococcal vaccination during pregnancy could reduce infant infections [Citation110].
National antenatal programs in most low-income countries are well established, with substantial service coverage [Citation48]. Vaccine doses along with other services could be given to HIV-infected pregnant women who attend the antenatal clinics or otherwise ART clinics.
5. Expert opinion
PCV is widely used in global infant immunization programs and has been recommended for use in HIV-infected adults in high-income countries along with a PPV23 booster dose. In parts of Africa, there is a combination of substantial residual VT circulation among adults despite mature infant PCV programs, still relatively high adult HIV prevalence, and persistent high risk of IPD in HIV-infected adults. An adapted vaccination strategy could reduce the risk of IPD in HIV-infected adults. Here, we have presented a number of options through direct and indirect vaccine protection to enable the identification of an effective way forward.
We define the optimal vaccination strategy as one that maximizes the reduction in pneumococcal disease burden in HIV-infected adults in Africa. There are of course other factors as well that may determine whether a theoretically optimal strategy is indeed programmatically feasible. Important evidence gaps exist to enable evaluation of the optimal pneumococcal vaccine strategy. It is uncertain if a 2p+1 dosing schedule will work to achieve better herd protection than a 3p+0 in settings where it has not yet been implemented, or whether an additional booster at school entry 2p+1 (+1) may be needed if protection against carriage wanes considerably in early childhood. Two cluster randomized trials in Malawi and Vietnam, in which 3p+0 and 2p+1 schedules are being compared head to head in each trial, can be used to evaluate their impact on pneumococcal carriage [Citation81,Citation82], and could provide crucial information on their relative merits for providing herd protection.
While PCV7 vaccine efficacy against IPD in HIV-infected adults has been previously estimated at 74% [Citation65], efficacy against VT-carriage is unknown. Moreover, the duration of protection against both carriage and disease in HIV-infected adults is unknown, with some evidence for the latter pointing to a rapid decline after 12 months of vaccination [Citation65]. Data on the interaction between ART (which may improve efficacy) and PCV shows no major impact of ART [Citation25], but since PPV23 effectiveness/efficacy against IPD is contentious [Citation60], dosing intervals to optimize efficacy and protection remains uncertain. More importantly, it is uncertain whether, in the era of routine PCV use in infants, HIV-infected adults substantially contribute to the residual VT transmission because of their elevated rates of carriage. Despite the importance of social interactions for pneumococcal transmission [Citation111,Citation112], only a few social mixing pattern studies have been conducted in low-income countries [Citation112–116]. Moreover, data on whether HIV-infected adults have differential social mixing behavior compared to HIV-uninfected adults are not available. Thus, limiting our ability to precisely quantify transmission dynamics in HIV-infected adults.
Uncertainties around the benefits of maternal immunization with PCV also need to be addressed. The efficacy of maternal vaccination in protecting the neonate from carriage acquisition and the duration of vaccine-induced protection in the newborn need to be established [Citation105,Citation110]. Also, there are concerns that maternal vaccination could interfere with the benefits of infant priming doses by inhibiting the antibody responses particularly when high residual concentration of maternal placentally transferred antigen-specific antibodies are present at the time of infant immunization [Citation117,Citation118].
Article highlights
Circulation of VTs persists in the PCV era in some low-income countries and results in a continued high and potentially vaccine-preventable burden of pneumococcal disease in HIV-infected adults.
Routine pneumococcal vaccination programs for HIV-infected adults are not implemented in low-income countries.
Mitigation of the VT-disease burden in HIV-infected adults in low-income countries may be achieved either by added direct protection or increased indirect protection from the infant program (via increased coverage or a change in vaccine schedule).
For added direct protection both PCV and PPV are licensed and used in high-income countries. PCV is more immunogenic but also substantially more expensive and has inferior serotype coverage.
For added indirect protection a change in infant immunization schedule to stipulate longer-lasting protection may largely mitigate the risk for vaccine-preventable pneumococcal disease in the HIV-infected.
Both strategies will need a formal evaluation of their likely effectiveness and cost-effectiveness.
Author Contributions
DT, NF, and SF conceived the idea for the manuscript. DT wrote the first manuscript draft with support from AP, OJ, KEG, NF, and SF. All authors contributed to, and approved the final draft. All authors declare that they meet the ICMJE criteria for authorship.
Declaration of interest
D Thindwa, J Ojal, K E Gallagher, N French, and S Flasche are supported by the National Institute for Health Research (NIHR) Global Health Research Unit on Mucosal Pathogens using UK aid from the UK Government. The views expressed in this publication are those of the author(s) and not necessarily those of the NIHR or the Department of Health and Social Care. A Pinsent was supported by a grant from the Bill and Melinda Gates Foundation (OPP1139859). S Flasche is also supported by a Sir Henry Dale Fellowship jointly funded by the Wellcome Trust and the Royal Society (Grant number 208,812/Z/17/Z). The authors have no other relevant affiliations or financial involvement with any organization or entity with a financial interest in or financial conflict with the subject matter or materials discussed in the manuscript apart from those disclosed.
Reviewer Disclosures
Peer reviewers on this manuscript have no relevant financial or other relationships to disclose.
Acknowledgments
We would like to thank Robert S Heyderman of the University College London, and John W Edmund of the London School of Hygiene and Tropical Medicine, in London, United Kingdom, for insightful discussions at the initial stage of this work.
Additional information
Funding
References
- O’Brien KL, Wolfson LJ, Watt JP, et al. Burden of disease caused by Streptococcus pneumoniae in children younger than 5 years: global estimates. Lancet. 2009;374:893–902.
- Wahl B, O’Brien KL, Greenbaum A, et al. Burden of Streptococcus pneumoniae and Haemophilus influenzae type b disease in children in the era of conjugate vaccines: global, regional, and national estimates for 2000–15. Lancet Glob Health. 2018;6:e744–57.
- van Aalst M, Lötsch F, Spijker R, et al. Incidence of invasive pneumococcal disease in immunocompromised patients: a systematic review and meta-analysis. Travel Med Infect Dis. 2018;24:89–100.
- Corcoran M, Vickers I, Mereckiene J, et al. The epidemiology of invasive pneumococcal disease in older adults in the post-PCV era. Has there been a herd effect? Epidemiol Infect. 2017;145:2390–2399.
- Meiring S, Cohen C, Quan V, et al. HIV infection and the epidemiology of invasive pneumococcal disease (IPD) in South African adults and older children prior to the introduction of a pneumococcal conjugate vaccine (PCV). Plos One. 2016;11:e0149104.
- Vardanjani HM, Borna H, Ahmadi A. Effectiveness of pneumococcal conjugate vaccination against invasive pneumococcal disease among children with and those without HIV infection: a systematic review and meta-analysis. BMC Infect Dis. 2019;19:685.
- Cohen C, von Mollendorf C, de Gouveia L, et al. Effectiveness of 7-valent pneumococcal conjugate vaccine against invasive pneumococcal disease in HIV-infected and -uninfected children in South Africa: a matched case-control study. Clin Infect Dis. 2014;59:808–818.
- Cohen C, von Mollendorf C, de Gouveia L, et al. Effectiveness of the 13-valent pneumococcal conjugate vaccine against invasive pneumococcal disease in South African children:a case-control study. Lancet Glob Health. 2017;5:e359–69.
- von Gottberg A, de Gouveia L, Tempia S, et al. Effects of vaccination on invasive pneumococcal disease in South Africa. N Engl J Med. 2014;371:1889–1899.
- Klugman KP, Madhi SA, Huebner RE, et al. Trial of a 9-valent pneumococcal conjugate vaccine in children with and those without HIV infection. N Engl J Med. 2003;349:1341–1348.
- Whitney CG, Farley MM, Hadler J, et al. Decline in invasive pneumococcal disease after the introduction of protein–polysaccharide conjugate vaccine. N Engl J Med. 2003;348:1737–1746.
- Hammitt LL, Etyang AO, Morpeth SC, et al. Effect of ten-valent pneumococcal conjugate vaccine on invasive pneumococcal disease and nasopharyngeal carriage in Kenya: a longitudinal surveillance study. Lancet. 2019;393:2146–2154.
- Ngocho JS, Magoma B, Olomi GA, et al. Effectiveness of pneumococcal conjugate vaccines against invasive pneumococcal disease among children under five years of age in Africa: a systematic review. Plos One. 2019;14:e0212295.
- Loughlin AM, Hsu K, Silverio AL, et al. Direct and indirect effects of PCV13 on nasopharyngeal carriage of PCV13 unique pneumococcal serotypes in massachusetts’ children. Pediatr Infect Dis J. 2014;33:504.
- Southern J, Andrews N, Sandu P, et al. Pneumococcal carriage in children and their household contacts six years after introduction of the 13-valent pneumococcal conjugate vaccine in England. Plos One. 2018;13:e0195799.
- Heinsbroek E, Tafatatha T, Phiri A, et al. Pneumococcal carriage in households in Karonga District, Malawi, before and after introduction of 13-valent pneumococcal conjugate vaccination. Vaccine. 2018;36:7369–7376.
- Ojal J, Flasche S, Hammitt LL, et al. Sustained reduction in vaccine-type invasive pneumococcal disease despite waning effects of a catch-up campaign in Kilifi, Kenya: a mathematical model based on pre-vaccination data. Vaccine. 2017;35:4561–4568.
- Lourenço J, Obolski U, Swarthout TD, et al. Determinants of high residual post-PCV13 pneumococcal vaccine-type carriage in Blantyre, Malawi: a modelling study. BMC Med. 2019;17. DOI:10.1186/s12916-019-1450-2..
- Sigaúque B, Moiane B, Massora S, et al. Early declines in vaccine type pneumococcal carriage in children less than 5 years old after introduction of 10-valent pneumococcal conjugate vaccine in mozambique. Pediatr Infect Dis J. 2018;37:1054.
- Swarthout TD, Fronterre C, Lourenço J, et al. *High residual carriage of vaccine-serotype Streptococcus pneumoniae after introduction of pneumococcal conjugate vaccine in Malawi. Nat Commun. 2020;11:2222.
- Aston SJ, Ho A, Jary H, et al. Etiology and risk factors for mortality in an adult community-acquired Pneumonia Cohort in Malawi. Am J Respir Crit Care Med. 2019;200:359–369.
- Feikin DR, Jagero G, Aura B, et al. High rate of pneumococcal bacteremia in a prospective cohort of older children and adults in an area of high HIV prevalence in rural western Kenya. BMC Infect Dis. 2010;10:186.
- Cordonnier C, Averbuch D, Maury S, et al. Pneumococcal immunization in immunocompromised hosts: where do we stand? Expert Rev Vaccines. 2014;13:59–74.
- Madhi SA, Nunes MC. The potential impact of pneumococcal conjugate vaccine in Africa: considerations and early lessons learned from the South African experience. Hum Vaccin Immunother. 2016;12:314–325.
- Nunes MC, Madhi SA. Safety, immunogenicity and efficacy of pneumococcal conjugate vaccine in HIV-infected individuals. Hum Vaccin Immunother. 2012;8:161–173.
- Glennie SJ, Sepako E, Mzinza D, et al. Impaired CD4 T cell memory response to Streptococcus pneumoniae precedes CD4 T cell depletion in HIV-infected Malawian adults. PLoS ONE. 2011;6:e25610.
- Zhang L, Li Z, Wan Z, et al. Humoral immune responses to Streptococcus pneumoniae in the setting of HIV-1 infection. Vaccine. 2015;33:4430–4436.
- Kerr AR, Paterson GK, Riboldi-Tunnicliffe A, et al. Innate immune defense against pneumococcal pneumonia requires pulmonary complement component C3. Infect Immun. 2005;73:4245–4252.
- Jochems SP, Weiser JN, Malley R, et al. The immunological mechanisms that control pneumococcal carriage. PLoS Pathog. 2017;13:e1006665.
- Iwajomo OH, Finn A, Moons P, et al. Deteriorating pneumococcal-specific B-cell memory in minimally symptomatic African children with HIV infection. J Infect Dis. 2011;204:534–543.
- Picard C, Puel A, Bustamante J, et al. Primary immunodeficiencies associated with pneumococcal disease. Curr Opin Allergy Clin Immunol. 2003;3:451–459.
- Iwajomo OH, Finn A, Ogunniyi AD, et al. Impairment of pneumococcal antigen specific isotype-switched igg memory B-cell immunity in HIV infected Malawian adults. Plos One. 2013;8:e78592.
- Eley B. Immunization in patients with HIV infection. Drugs. 2008;68:1473–1481.
- Glennie SJ, Banda D, Gould K, et al. Defective pneumococcal-specific Th1 responses in HIV-infected adults precedes a loss of control of pneumococcal colonization. Clin Infect Dis. 2013;56:291–299.
- De Milito A, Mörch C, Sönnerborg A, et al. Loss of memory (CD27) B lymphocytes in HIV-1 infection. AIDS. 2001;15:957.
- Madhi SA, Kuwanda L, Cutland C, et al. Quantitative and Qualitative Antibody Response to Pneumococcal Conjugate Vaccine Among African Human Immunodeficiency Virus-Infected and Uninfected Children. Pediatr Infect Dis J. 2005;24:410.
- Jones N, Huebner R, Khoosal M, et al. The impact of HIV on Streptococcus pneumoniae bacteraemia in a South African population. AIDS. 1998;12:2177.
- Madhi SA, Madhi A, Petersen K, et al. Impact of human immunodeficiency virus type 1 infection on the epidemiology and outcome of bacterial Meningitis in South African children. Inter J Infect Dis. 2001;5:119–125.
- McEllistrem MC, Mendelsohn AB, Pass MA, et al. Recurrent invasive pneumococcal disease in individuals with human immunodeficiency virus infection. J Infect Dis. 2002;185:1364–1368.
- Everett DB, Mukaka M, Denis B, et al. Ten years of surveillance for invasive streptococcus pneumoniae during the era of antiretroviral scale-up and cotrimoxazole prophylaxis in Malawi. Plos One. 2011;6:e17765.
- Heffernan RT, Barrett NL, Gallagher KM, et al. Declining incidence of invasive streptococcus pneumoniae infections among persons with AIDS in an era of highly active antiretroviral therapy, 1995—2000. J Infect Dis. 2005;191:2038–2045.
- Bar-Zeev N, Mtunthama N, Gordon SB, et al. Minimum incidence of adult invasive pneumococcal disease in Blantyre, Malawi an Urban African setting: a hospital based prospective cohort study. Plos One. 2015;10:e0128738.
- Bigogo GM, Audi A, Auko J, et al. Indirect effects of 10-valent pneumococcal conjugate vaccine against adult pneumococcal pneumonia in rural Western Kenya. Clin Infect Dis. 2019;69:2177–2184.
- Nzenze SA, Madhi SA, Shiri T, et al. Imputing the direct and indirect effectiveness of childhood pneumococcal conjugate vaccine against invasive pneumococcal disease by surveying temporal changes in nasopharyngeal pneumococcal colonization. Am J Epidemiol. 2017;186:435–444.
- Dwyer-Lindgren L, Cork MA, Sligar A, et al. *Mapping HIV prevalence in sub-Saharan Africa between 2000 and 2017. Nature. 2019;570:189.
- Klugman KP, Rodgers GL. *Population versus individual protection by pneumococcal conjugate vaccination. Lancet. 2019;393:2102–2104.
- Kuhn L, Goga AE. Moving towards elimination: findings from the South Africa prevention of mother to child transmission evaluation (SAPMTCTE). BMC Infect Dis. 2019;19:782.
- van Lettow M, Landes M, van Oosterhout J, et al. Prevention of mother-to-child transmission of HIV: a cross-sectional study in Malawi. Bull World Health Organ. 2018;96:256–265.
- Harries AD, Ford N, Jahn A, et al. Act local, think global: how the Malawi experience of scaling up antiretroviral treatment has informed global policy. BMC Public Health. 2016;16:938.
- Jahn A, Harries AD, Schouten EJ, et al. Scaling-up antiretroviral therapy in Malawi. Bull World Health Organ. 2016;94:772–776.
- Mirsaeidi M, Schraufnagel DE. Pneumococcal vaccines: understanding centers for disease control and prevention recommendations. Ann ATS. 2014;11:980–985.
- Bonnave C, Mertens D, Peetermans W, et al. *Adult vaccination for pneumococcal disease: a comparison of the national guidelines in Europe. Eur J Clin Microbiol Infect Dis. 2019;38:785–791.
- Ho Y-L, Brandão AP, de Cunto Brandileone MC, et al. Immunogenicity and safety of pneumococcal conjugate polysaccharide and free polysaccharide vaccines alone or combined in HIV-infected adults in Brazil. Vaccine. 2013;31:4047–4053.
- Lombardi F, Belmonti S, Fabbiani M, et al. Immunogenicity and safety of the 13-valent pneumococcal conjugate vaccine versus the 23-valent polysaccharide vaccine in unvaccinated HIV-infected adults: a pilot, prospective controlled study. Plos One. 2016;11:e0156523.
- Lu C-L, Hung -C-C, Chuang Y-C, et al. Serologic response to primary vaccination with 7-valent pneumococcal conjugate vaccine is better than with 23-valent pneumococcal polysaccharide vaccine in HIV-infected patients in the era of combination antiretroviral therapy. Hum Vaccin Immunother. 2013;9:398–404.
- Lesprit P, Pédrono G, Molina J-M, et al. Immunological efficacy of a prime-boost pneumococcal vaccination in HIV-infected adults. AIDS. 2007;21:2425.
- Feikin DR, Elie CM, Goetz MB, et al. Randomized trial of the quantitative and functional antibody responses to a 7-valent pneumococcal conjugate vaccine and/or 23-valent polysaccharide vaccine among HIV-infected adults. Vaccine. 2001;20:545–553.
- Søgaard OS, Lohse N, Harboe ZB, et al. Improving the immunogenicity of pneumococcal conjugate vaccine in HIV-infected adults with a toll-like receptor 9 agonist adjuvant: a randomized, controlled trial. Clin Infect Dis. 2010;51:42–50.
- Peñaranda M, Payeras A, Cambra A, et al. Group the MPS. Conjugate and polysaccharide pneumococcal vaccines do not improve initial response of the polysaccharide vaccine in HIV-infected adults. AIDS. 2010;24:1226.
- Pedersen RH, Lohse N, Østergaard L, et al. The effectiveness of pneumococcal polysaccharide vaccination in HIV-infected adults: a systematic review. HIV Med. 2011;12:323–333.
- French N, Nakiyingi J, Carpenter LM, et al. 23–valent pneumococcal polysaccharide vaccine in HIV-1-infected Ugandan adults: double-blind, randomised and placebo controlled trial. Lancet. 2000;355:2106–2111.
- López‐Palomo C, Martín‐Zamorano M, Benítez E, et al. Pneumonia in HIV-infected patients in the HAART era: incidence, risk, and impact of the pneumococcal vaccination. J Med Virol. 2004;72:517–524.
- Watera C, Nakiyingi J, Miiro G, et al. 23-Valent pneumococcal polysaccharide vaccine in HIV-infected Ugandan adults: 6-year follow-up of a clinical trial cohort. AIDS. 2004;18:1210–1213.
- Dworkin MS, Ward JW, Hanson DL, et al. Pneumococcal disease among human immunodeficiency virus-infected persons: incidence, risk factors, and impact of vaccination. Clin Infect Dis. 2001;32:794–800.
- French N, Gordon SB, Mwalukomo T, et al. *A trial of a 7-valent pneumococcal conjugate vaccine in HIV-infected adults. N Engl J Med. 2010;362:812–822.
- Bonten MJM, Huijts SM, Bolkenbaas M, et al. Polysaccharide conjugate vaccine against pneumococcal pneumonia in adults. N Engl J Med. 2015;372:1114–1125.
- WHO. Pneumococcus vaccines position paper. World Health Organisation, Switzerland; 2019.
- Madhi SA, Moreira M, Koen A, et al. Impact of HIV status and vaccination schedule on bacterial nasopharyngeal carriage following infant immunisation with the pneumococcal non-typeable Haemophilus influenzae protein D conjugate vaccine in South Africa. Vaccine. 2020. DOI:10.1016/j.vaccine.2020.01.062..
- Miiro G, Kayhty H, Watera C, et al. Conjugate pneumococcal vaccine in HIV-infected ugandans and the effect of past receipt of polysaccharide vaccine. J Infect Dis. 2005;192:1801–1805.
- Ibarz-Pavon AB, French N. No changes on viral load and CD4+ T-cell counts following immunization with 7-valent pneumococcal conjugate vaccine among HIV-infected adults in Malawi. Vaccine. 2018;36:2504–2506.
- McLaughlin JM, Swerdlow DL, Isturiz RE, et al. Decision-making for PCV in adults. Hum Vaccin Immunother. 2019;15:584–593.
- McLaughlin JM, Jiang Q, Isturiz RE, et al. Effectiveness of 13-valent pneumococcal conjugate vaccine against hospitalization for community-acquired pneumonia in older US adults: a test-negative design. Clin Infect Dis. 2018;67:1498–1506.
- Lee K-Y, Tsai M-S, Kuo K-C, et al. Pneumococcal vaccination among HIV-infected adult patients in the era of combination antiretroviral therapy. Hum Vaccin Immunother. 2014;10:3700–3710.
- Licciardi PV, Tan EL, Li P, et al. Pneumococcal vaccination for HIV-infected individuals in Singapore. Proc Singapore Healthcare. 2019;28:55–60.
- Choi YH, Andrews N, Miller E. Estimated impact of revising the 13-valent pneumococcal conjugate vaccine schedule from 2+1 to 1+1 in England and Wales: A modelling study. PLoS Med. 2019;16:e1002845.
- VACFA. Immunization schedules - Africa Vaccines for Africa 2018. [cited 2020 Aug 27].http://www.vacfa.uct.ac.za/immunization-schedules-africa
- Gavi, The vaccine alliance. Pneumococcal vaccine support 2017. [cited 2019 Aug 1]. https://www.gavi.org/support/nvs/pneumococcal/
- Ntshoe GM, McAnerney JM, Archer BN, et al. Measles outbreak in south africa: epidemiology of laboratory-confirmed measles cases and assessment of intervention, 2009–2011. Plos One. 2013;8:e55682.
- Weinberger DM, Pitzer VE, Regev-Yochay G, et al. Association between the decline in pneumococcal disease in unimmunized adults and vaccine-derived protection against colonization in toddlers and preschool-aged children. Am J Epidemiol. 2019;188:160–168.
- Le Polain De Waroux O, Flasche S, Prieto-Merino D, et al. The efficacy and duration of protection of pneumococcal conjugate vaccines against nasopharyngeal carriage: a meta-regression model. Pediatr Infect Dis J. 2015;34:858.
- An Evaluation of PCV13 Vaccine Schedules, Comparing Impact of 2+1 vs 3+0 on Pneumococcal Carriage in Blantyre, Malawi. 2019. [cited 2019 Nov 29]. https://clinicaltrials.gov/ct2/show/NCT04078997
- Evaluation of PCV Schedules in a Naive Population in Vietnam n.d. [cited 2019 Dec 16]. https://clinicaltrials.gov/ct2/show/NCT02961231
- Blyth CC, Jayasinghe S, Andrews RM. A rationale for change: an increase in invasive pneumococcal disease in fully vaccinated children. Clin Infect Dis. n.d.. DOI:10.1093/cid/ciz493.
- Jayasinghe S, Menzies R, Chiu C, et al. Long-term impact of a “3 + 0” schedule for 7- and 13-valent pneumococcal conjugate vaccines on invasive pneumococcal disease in Australia, 2002–2014. Clin Infect Dis. 2017;64:175–183.
- Usuf E, Bottomley C, Bojang E, et al. Persistence of nasopharyngeal pneumococcal vaccine serotypes and increase of nonvaccine serotypes among vaccinated infants and their mothers 5 years after introduction of pneumococcal conjugate vaccine 13 in the Gambia. Clin Infect Dis. 2019;68:1512–1521.
- Mackenzie GA, Hill PC, Jeffries DJ, et al. Effect of the introduction of pneumococcal conjugate vaccination on invasive pneumococcal disease in The Gambia: a population-based surveillance study. Lancet Infect Dis. 2016;16:703–711.
- WHO. Pneumococcal conjugate 3rd dose (PCV3) immunization coverage. WHO 2019. [cited 2019 Dec 16]. http://www.who.int/gho/immunization/pneumococcal/en/
- Keeling MJ, Rohani P. Modeling Infectious Diseases in Humans and Animals. Princeton, New Jersey: Princeton University Press; 2011.
- Vynnycky E, White R. An introduction to infectious disease modelling. Oxford, New York: Oxford University Press; 2010.
- Anderson RM, May RM. Infectious diseases of humans: dynamics and control. Oxford, New York: Oxford University Press; 1992.
- Flasche S, Lipsitch M, Ojal J, et al. *Estimating the contribution of different age strata to vaccine serotype pneumococcal transmission in the pre vaccine era:a modelling study. BMC Med. 2020;18. DOI:10.1186/s12916-020-01601-1.
- Wyllie AL, Warren JL, Regev-Yochay G, et al. Serotype patterns of pneumococcal disease in adults are correlated with carriage patterns in older children. MedRxiv. 2019;2019:12.18.19015180.
- Crum-Cianflone NF, Wallace MR. Vaccination in HIV-infected adults. AIDS Patient Care STDS. 2014;28:397–410.
- Frank TD, Carter A, Jahagirdar D, et al. Global, regional, and national incidence, prevalence, and mortality of HIV, 1980–2017, and forecasts to 2030, for 195 countries and territories: a systematic analysis for the Global Burden of Diseases, Injuries, and Risk Factors Study 2017. Lancet HIV. 2019;6:e831–59.
- Meyer-Rath G, Johnson LF, Pillay Y, et al. Changing the South African national antiretroviral therapy guidelines: the role of cost modelling. PLoS One. 2017;12. DOI:10.1371/journal.pone.0186557..
- Cawley C, McRobie E, Oti S, et al. Identifying gaps in HIV policy and practice along the HIV care continuum: evidence from a national policy review and health facility surveys in urban and rural Kenya. Health Policy Plan. 2017;32:1316–1326.
- Wallace A, Dietz V, Cairns KL. Integration of immunization services with other health interventions in the developing world: what works and why? Systematic literature review. Trop Med Int Health. 2009;14:11–19.
- Crum-Cianflone NF, Hullsiek KH, Roediger M, et al. A randomized clinical trial comparing revaccination with pneumococcal conjugate vaccine to polysaccharide vaccine among HIV-infected adults. J Infect Dis. 2010;202:1114–1125.
- Nunes MC, von Gottberg A, de Gouveia L, et al. The impact of antiretroviral treatment on the burden of invasive pneumococcal disease in South African children: a time series analysis. AIDS. 2011;25:453.
- Heinsbroek E, Tafatatha T, Phiri A, et al. Persisting high prevalence of pneumococcal carriage among HIV-infected adults receiving antiretroviral therapy in Malawi. Aids. 2015;29:1837–1844.
- Heinsbroek E, Tafatatha T, Chisambo C, et al. Pneumococcal acquisition among infants exposed to HIV in rural Malawi: a longitudinal household study. Am J Epidemiol. 2016;183:70–78.
- Nunes MC, Shiri T, van Niekerk N, et al. Acquisition of streptococcus pneumoniae in pneumococcal conjugate vaccine-naïve South African children and their mothers. Pediatr Infect Dis J. 2013;32:e192.
- Shiri T, Auranen K, Nunes MC, et al. Dynamics of pneumococcal transmission in vaccine-naïve children and their HIV-infected or HIV-uninfected mothers during the first 2 years of life. Am J Epidemiol. 2013;178:1629–1637.
- Hill PC, Cheung YB, Akisanya A, et al. Nasopharyngeal carriage of streptococcus pneumoniae in gambian infants: a longitudinal study. Clin Infect Dis. 2008;46:807–814.
- Clarke E, Kampmann B, Goldblatt D. Maternal and neonatal pneumococcal vaccination - where are we now? Expert Rev Vaccines. 2016;15:1305–1317.
- Tigoi CC, Gatakaa H, Karani A, et al. Rates of acquisition of pneumococcal colonization and transmission probabilities, by serotype, among newborn infants in Kilifi District, Kenya. Clin Infect Dis. 2012;55:180–188.
- Ojal J, Goldblatt D, Tigoi C, et al. Effect of maternally derived anti-protein and anticapsular IgG antibodies on the rate of acquisition of nasopharyngeal carriage of pneumococcus in newborns. Clin Infect Dis. 2018;66:121–130.
- Abu-Raya B, Smolen KK, Willems F, et al. Transfer of maternal antimicrobial immunity to HIV-exposed uninfected newborns. Front Immunol. 2016;7. DOI:10.3389/fimmu.2016.00338.
- Gupta A, Mathad JS, Yang W-T, et al. Maternal pneumococcal capsular IgG antibodies and transplacental transfer are low in South Asian HIV-infected mother-infant pairs. Vaccine. 2014;32:1466–1472.
- Chaithongwongwatthana S, Yamasmit W, Limpongsanurak S, et al. Pneumococcal vaccination during pregnancy for preventing infant infection. Cochrane Database Syst Rev. 2015. DOI:10.1002/14651858.CD004903.pub4.
- Heesterbeek H, Anderson RM, Andreasen V, et al. Modeling infectious disease dynamics in the complex landscape of global health. Science. 2015;347:aaa4339.
- le Polain de Waroux O, Flasche S, Kucharski AJ, et al. Identifying human encounters that shape the transmission of Streptococcus pneumoniae and other acute respiratory infections. Epidemics. 2018;25:72–79.
- le Polain de Waroux O, Cohuet S, Ndazima D, et al. Characteristics of human encounters and social mixing patterns relevant to infectious diseases spread by close contact: a survey in Southwest Uganda. BMC Infect Dis. 2018;18:172.
- Kiti MC, Kinyanjui TM, Koech DC, et al. Quantifying age-related rates of social contact using diaries in a rural coastal population of Kenya. Plos One. 2014;9:e104786.
- Melegaro A, Fava ED, Poletti P, et al. Social contact structures and time use patterns in the manicaland province of zimbabwe. Plos One. 2017;12:e0170459.
- Johnstone-Robertson SP, Mark D, Morrow C, et al. Social mixing patterns within a South African township community: implications for respiratory disease transmission and control. Am J Epidemiol. 2011;174:1246–1255.
- Voysey M, Kelly DF, Fanshawe TR, et al. The influence of maternally derived antibody and infant age at vaccination on infant vaccine responses. JAMA Pediatr. 2017;171:637–646.
- Jones C, Pollock L, Barnett SM, et al. The relationship between concentration of specific antibody at birth and subsequent response to primary immunization. Vaccine. 2014;32:996–1002.