ABSTRACT
Introduction
As of January 2021, rotavirus vaccination programs have been implemented in 109 countries and their use has resulted in a positive impact on rotavirus-related diarrheal hospitalizations and mortality in children below 5 years of age. Despite these successes, several countries in Africa and Asia where disease burden is high have not yet implemented rotavirus vaccination at all or at a scale sufficient enough to demonstrate impact. This could be, among other reasons, due to poor vaccine coverage and the modest levels of efficacy and effectiveness of the vaccines in these resource-limited settings.
Areas covered
We review various factors related to the human host (malnutrition, maternally derived antibodies and breastfeeding, genetic factors, blood group, and co-administration with oral polio vaccine), rotavirus pathogen (force of infection, strain diversity and coinfections), and the environment (related to the human microbiome) which reflect complex and interconnected processes leading to diminished vaccine performance in resource-limited settings.
Expert opinion
Addressing the limiting factors for vaccine efficacy is needed but likely to take a long time to be resolved. An immediate solution is to increase the immunization coverage to higher values generating an overall effect of adequate proportion of protected population to reduce the prevalence of rotavirus disease.
PLAIN LANGUAGE SUMMARY
What is the context?
Rotavirus is contagious and causes severe diarrhea in children below 5 years of age. It caused 128,500 deaths and 258 million episodes of diarrhea in 2016.
Vaccines protecting children from rotavirus are given orally and have been implemented in 109 countries and used for more than a decade. They considerably decreased the number of hospitalizations and deaths.
Several Asian and African countries experience rotavirus infections in large numbers and lag behind in implementing rotavirus vaccination programs.
In these countries, rotavirus vaccines only prevent a smaller percentage of severe cases. The reasons for this reduction in vaccination impact are not widely known.
What is new?
We reviewed the literature to identify the reasons that could explain the differences in vaccination impact worldwide.
Factors that might influence the impact of vaccination include
Infected children (malnutrition, breastfeeding, blood group, and co-administration with oral polio vaccine);
Circulating virus(es) (force of infection, number of new strains, and coinfections with other pathogens)
Environment: human microbiome (microorganisms at the surface and in the body; that could be altered by diet, method of childbirth, or hygiene).
Why is this important?
In summary, rotavirus vaccination has reduced rotavirus-associated hospitalizations and deaths; however, more research is needed to understand the factors influencing the impact of vaccination in order to optimize them. (see – Graphical PLS).
2. Background
2.1. Rotavirus history and biology
For centuries, acute diarrhea has been one of the leading causes of hospitalization and death [Citation1] and until 1973, one of the major infectious agents among cases of acute diarrhea remained unknown [Citation2]. In 1973, Ruth Bishop and her team identified new ‘virus-like’ particles, from the epithelial cells of duodenal mucosa of children suffering from non-bacterial gastroenteritis and admitted in the Royal Children’s Hospital Melbourne (Australia). Through electron microscopic examination, it was revealed that these ‘’virus-like” particles were distinct from the parvovirus group or Norwalk-like agent but had similar resemblance to orbiviruses. Owing to its ‘wheel-like’ morphology, the causative agent was subsequently named ‘Rotavirus’ (Latin word ‘’rota”, meaning wheel) under the family Reoviridae [Citation2,Citation3].
Rotavirus particles consist of three concentric shells that enclose 11 gene segments of double stranded RNA, which encode six structural proteins (VP1-VP4, VP6, VP7) and five to six non-structural proteins (NSP1-NSP5/6). Rotaviruses are commonly classified according to group and serotype. A total of nine rotavirus serogroups have been described (named A to J, E being currently excluded) based on genetic and antigenic differences in the VP6 protein [Citation4]. Only groups A, B, and C are known to cause disease in humans [Citation5]. Rotavirus group H may be transmissible to humans [6,Citation7]. Group A includes the virus serotypes most frequently associated with disease in humans. Serotypes within group A are defined by epitopes in VP7 (glycoprotein, G types) and VP4 (protease, P types) that induce neutralizing antibodies. At present, at least 36 G serotypes and 51 P genotypes have been characterized [Citation8,Citation9]. Globally, six G types (G1–4, G9, and G12) and three P types (P[Citation4], P[Citation6], and P[Citation8]) are known to be most prevalent [Citation10,Citation11]. Five combinations of these common G and P types have been reported to account for more than 90% of the global circulation of rotavirus strains: P[Citation8]G1, P[Citation4]G2, P[Citation8]G3, P[Citation8]G4, P[Citation8]G9, while P[Citation6]G12 and P[Citation8]G12 are the emerging global strains [Citation8,Citation10,Citation11].
2.2. The global impact of rotavirus infection
Rotavirus has been documented as the most common cause of severe acute diarrhea in children below 5 years of age worldwide. Rotavirus infection is highly contagious as demonstrated by its universal spread. Rotavirus transmission occurs via the fecal-oral route, spreading from person-to-person and through fomites. The pathophysiology of rotavirus diarrhea is multifactorial, resulting from the direct and indirect effects of virus infection, and the indirect effects of host response [Citation5,Citation12]. The incubation period is 1–4 days and the disease lasts from 3 to 9 days. Common clinical features include fever, vomiting, and watery diarrhea that may lead to severe dehydration [Citation13].
From 1986 to 2000, rotavirus infections were responsible for up to half a million deaths with at least 2 million children being hospitalized every year, worldwide [Citation14]. While the vast majority of rotavirus-associated deaths occurred in children in resource-limited countries, particularly in sub-Saharan Africa and in the Indian subcontinent [Citation14,Citation15], deaths due to rotavirus were rarely reported in resource-rich countries. Possible reasons for low mortality could be related to better hygiene and access to timely healthcare resources; however, the morbidity burden is still significant in these settings. Regardless of economic level, rotavirus accounted for an estimated 1,537,000 global hospital admissions due to diarrheal disease among children under 5 years of age in 2016 [Citation16] suggesting that it is a significant global public health burden both in terms of mortality and morbidity.
2.3. Disease management and prevention
There is no specific treatment for rotaviral diarrhea, but as with other forms of gastroenteritis, treatment is based on addressing dehydration through fluid replacement, either orally, through oral rehydration solutions (ORS), or intravenously, depending on the severity of dehydration [Citation17,Citation18]. Like for all cases of gastroenteritis, zinc supplementation is recommended to reduce the duration and severity of diarrhea [Citation19] and breastfeeding encompasses an important aspect of rehydration therapy as well as nutrition for children under 2 years of age [Citation20]. Prevention of infection remains the cornerstone of effective management of rotaviral disease. The provision of clean water, maintaining hygienic practices like handwashing, and promoting exclusive breastfeeding for 6 months is beneficial to reduce the risk of rotavirus infections. Lastly, rotavirus vaccines are considered to have an acceptable safety profile and are effective in preventing rotavirus gastroenteritis in children [Citation17,Citation21,Citation22]. The World Health Organization (WHO) emphasizes on the use of rotavirus vaccination but together with the scaling-up of both prevention (promotion of early and exclusive breastfeeding, handwashing with soap, improved water, and sanitation) and treatment packages (including low-osmolarity ORS and zinc) [Citation17].
3. Licensure and use of rotavirus vaccines
Owing to the existence of a preventable disease burden due to rotavirus diarrhea, vaccine development came into the forefront of research. After decades of various scientific endeavors and engagements, two live, orally administered vaccines: Rotarix (GSK, Belgium), derived from a single common strain of human rotavirus (HRV) and Rotateq (Merck & Co. Inc., West Point, PA, USA), a pentavalent vaccine containing the human antigens G1-G4 and P[Citation8] in mono-reassortant viruses on a bovine rotavirus (WC3 strain) genetic backbone (HBRV), gained licensure and became widely available. Other licensed and locally available vaccines include the oral, live-attenuated Rotavac (Bharat Biotech, India), Rotasiil (Serum Institute of India, India), Rotavin-M1 (Polyvac, Vietnam), and Lanzhou lamb rotavirus vaccine (LLR, Lanzhou Institute of Biological Products, China). In 2006, the WHO’s Strategic Advisory Group of Experts (SAGE) recommended the use of rotavirus vaccines in Europe and Americas and subsequently extended this recommendation in 2009 to all the other regions of the world [Citation23,Citation24]. In its latest position paper, the WHO recommends prioritization of rotavirus vaccination programs in countries with high rotavirus gastroenteritis-associated fatality rates, such as in south and south-eastern Asia and sub-Saharan Africa [Citation17]. The recommendation for global use of rotavirus vaccines by the SAGE group has proven to be useful as it has been estimated to have reduced the magnitude of disease severity, hospitalization, and mortality among children under 5 years of age [Citation16]. As of January 2021, 109 countries have introduced rotavirus vaccines () [Citation25].
Rotavirus-associated mortality has decreased markedly over time in part because of the introduction of the rotavirus vaccine. An estimate from 2016 reported that the introduction and use of rotavirus vaccine reduced mortality in children less than 5 years by 2–6% [Citation26]. In 2016, approximately 28,000 deaths were averted, and approximately 83,200 additional children could have been saved had full vaccine coverage been achieved that year [Citation16] (). In 2017, rotavirus was responsible for 185,390 deaths. Sub-Saharan Africa bears the biggest burden, with over 125,000 deaths annually, followed by South Asia, where more than 30,000 deaths under 5 years of age occur every year due to rotavirus [Citation27]. In addition, rotavirus is still a highly prevalent cause of diarrhea worldwide and is responsible for a substantial non-fatal burden of diarrhea globally, with the majority of this burden being documented in the regions of sub-Saharan Africa and South Asia [Citation28–30]. However, it has become evident that globally available rotavirus vaccines showed varying degrees of efficacy in different regions indicating a clear disparity between regions based on resource availability [Citation31–37] ( ).
Figure 2. Proportion of countries with rotavirus vaccine by WHO region. WHO, World Health Organization. Source: Data as of September 2020 from ViewHub [Citation25]
![Figure 2. Proportion of countries with rotavirus vaccine by WHO region. WHO, World Health Organization. Source: Data as of September 2020 from ViewHub [Citation25]](/cms/asset/be919840-dc76-47aa-a240-286b772ee67d/ierv_a_1951247_f0002_oc.jpg)
Figure 3. Estimate of preventable rotavirus-associated deathsa (2016)
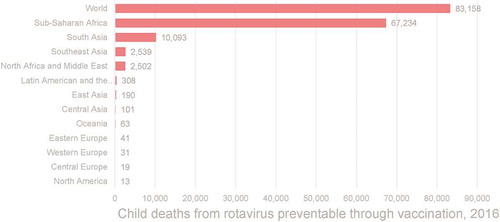
4. Factors impacting vaccine efficacy and effectiveness
The mechanisms and duration of protection against rotavirus infection (natural or vaccine-induced) are yet to be understood completely. This poses a challenge to elucidate the reasons for differences in rotavirus vaccine efficacy and effectiveness between resource-limited and resource-rich regions. Nonetheless, we strive to outline the main factors that could be driving these differences.
Similar to the performance of other oral viral vaccines [Citation38], a lower immunogenicity, efficacy, and effectiveness of both the HRV and HBRV in countries with the lowest income levels has been observed [Citation39–41]. Published literature has highlighted discrepancies in vaccine efficacy between low and high child mortality settings [Citation31–37]. One study estimated that in countries with high child mortality rates, not only is the vaccine efficacy lower (66%) when compared to countries with low child mortality (98%) but also that vaccine efficacy wanes faster in high child mortality countries over time [Citation39]. A recent meta-analysis of rotavirus vaccine performance involving 216,480 participants in low and high child mortality settings, reported that in regions with low child mortality, the use of rotavirus vaccines prevented 84–92% and 82% of severe rotavirus gastroenteritis cases in children under 1 and 2 years of age, respectively. Correspondingly, in regions with high child mortality, the use of rotavirus vaccines prevented only 57–63% and 35–41% of severe rotavirus gastroenteritis cases in children under 1 and 2 years of age, respectively [Citation42]. Similar observations for both rotavirus vaccines are documented when comparing estimates of effectiveness [Citation40,Citation42]. While the exact mechanisms of lower vaccine efficacy and effectiveness in resource-limited countries are unknown, a range of explanations have been mooted. Possible factors include breastfeeding, interference of high maternal antibody titers with vaccine strains, coinfection, nutrient deficiency, and altered intestinal microbiota [Citation8,Citation41]. Identifying whether these factors can explain the reduced vaccine efficacy observed in resource-limited settings, and which factors are most important, can help to prioritize future research aimed at improving vaccine performance [Citation43,Citation44].
In this article, we take the classical triad of host (genetics and commensal microbiota), pathogen (genetic diversity), and environment in epidemiology to discuss the potential factors that influence rotavirus vaccine efficacy and effectiveness against rotavirus diarrhea. Establishing the biological plausibility between host and pathogen and also involving environmental factors like microbiota and reservoir species, are key to understanding the dynamics of infections and developing a well-designed control and prevention strategy. Since HRV and HBRV are the most widely used vaccines for which publications are available, they are the focus of this review.
4.1. Host factors
Heterogeneity in vaccine efficacy and effectiveness estimates across baseline infant characteristics (age at first dose, gender, breastfeeding, and nutritional status) and geographic region have been documented [Citation45]. In this section, we take a closer look at the processes by which host-related factors might affect vaccine immune response and effectiveness.
4.1.1. Malnutrition
Malnutrition has been described as the most common cause of immune deficiency globally [Citation46] and is mainly prevalent in the world’s poorest countries, contributing to nearly half of the deaths among children under 5 years of age [Citation47]. It appears in various forms, including impaired growth (e.g. stunting, kwashiorkor) and deficiencies in specific micronutrients (e.g. zinc, iron, vitamin A and D). Malnutrition is associated with dysfunctions of the mucosal barrier, innate immunity and adaptive immunity, which increase the risk and severity of infections [Citation48]. Due to these immunological deficits, malnutrition is a major factor negatively affecting vaccine efficacy and effectiveness among children in resource-limited regions [Citation49]. A consistent link between malnutrition and oral vaccine performance is yet to be established [Citation50]. While some studies were uncertain about whether malnutrition decreased the efficacy of vaccination, more recently such a correlation was considered likely for rotavirus vaccination. Two components of malnutrition have attracted particular attention for its potential role in rotavirus vaccine performance: zinc deficiency and avitaminoses (i.e. disease caused by a deficiency of one or more vitamins) [Citation47]. Zinc deficiency alters immune functions and is known to be a contributing factor to severe diarrhea [Citation51]. It also plays a key role in the functioning of the adaptive immune system, and its deficiency is associated with depressed T-cell function [Citation52]. While a recent meta-analysis that investigated interventions to improve the performance of the oral viral vaccines (6 trials; with only one rotavirus vaccine trial) suggested that there was no significant effect of zinc supplementation on seroconversion [Citation53], evidence from Bangladesh and Zambia lent support to the use of a strategy involving zinc supplementation together with rotavirus vaccination to reduce the risk of rotavirus diarrhea [Citation54,Citation55]. Considering the effect of zinc on the immune system, it is logical to assume that zinc supplementation could help improve immune response to the vaccine. With regard to avitaminoses, it is well established that adequate levels of vitamins (especially A and D) are vital for the functioning of the immune system [Citation56]. Vitamin A is a key regulator of gut immunity and its supplementation reduces morbidity and mortality from diarrhea. Vitamin D is crucial for the functioning of the immune system since its deficiency has been associated with decreased immune responses to vaccines such as the influenza vaccine [Citation57]. Experimental results in animal models suggest that both vitamin A and D deficiency impacts the immune response to virus challenge or rotavirus vaccination [Citation58–61]. If it translates to human, this could potentially be of concern in impoverished settings as in general the nutritional status of the children from these areas is expected to be poorer compared to that of children from resource-rich countries. This prevailing nutritional status also often affects the vitamin levels (both A and D) of the individuals thus potentially impairing the immune parameter that may lower vaccine effectiveness in this setting. In addition, diarrheal infections in the early months of life of a child, especially recurrent illness, can lead to malnutrition and poor growth in a child living in impoverished settings. This leads to a vicious cycle of diarrhea-malnutrition-diarrhea, lowering the nutritional status and immunity in young infants and can have long-term consequences on the health of the child [Citation62]. Few other studies on other vaccines indicate mixed results regarding the usefulness of vitamin supplementation to improve vaccine performance [Citation53,Citation63] and therefore well-designed studies are required to show confirmatory association that would lend support to the use of vitamin supplementation to improve rotavirus vaccine performance in impoverished settings.
Full-term babies rely on their innate immune response rather than on their adaptive immune response (which usually develops from gestational month 3 onwards and becomes efficient after birth), but these immune defense mechanisms are immature, specifically they lack immune memory from previous exposure to a pathogen [Citation64]. Consequently, babies rely on antibodies they acquire from their mother which are transmitted via the placenta (during pregnancy) and breast milk. While these maternal antibodies provide protection to the child, a potential downside is that maternally derived antibodies can affect vaccine performance. It has been demonstrated that increased levels of transplacental rotavirus-specific immunoglobulin G (IgG) antibody are associated with reduced vaccine seroconversion while withholding breastfeeding does not impact vaccine efficacy in clinical trials, thereby suggesting that transplacental maternal antibodies have an increased effect on vaccine efficacy than antibodies ingested through breastfeeding [Citation65]. This is even more important in resource-limited regions as maternal serum and breast milk antibodies to rotavirus are highly prevalent in a developing country setting [Citation66]. Several studies in children have showed that the mother’s rotavirus-specific IgG levels before their infants received rotavirus vaccination were negatively associated with infants’ seroconversion after rotavirus vaccination [Citation67–69]. A similar trend was observed in New Zealand but lacked significance [Citation70]. In this case, by delaying the first dose of rotavirus vaccination within the prescription window, a combination of low interference from maternal antibodies and maturation of the infant immune function might improve seroconversion [Citation65,Citation71,Citation72]. However, this delay needs to be evaluated versus the need for early protection through vaccination, which is aimed at protection before onset of natural infection. A study from Indonesia evaluated a human neonatal rotavirus vaccine and reported vaccine efficacy against severe rotavirus infections when administered according to the country’s neonatal or infant immunization schedules [Citation73], thus lending support to the argument that vaccination at birth could prevent the interference from maternal antibodies and improve vaccine efficacy [Citation66].
A recent review summarized evidence showing that both antibody and non-antibody components of breast milk may have an effect on the infectivity of rotavirus strains and therefore may partially contribute to blocking the immune response of infants. Indeed, a general association of higher levels of rotavirus-specific immunoglobulin A (IgA) in breast milk with failed seroconversion has been reported [Citation65]. But clinical trials that withheld or delayed breastfeeding at the time of vaccination have also reported that there was no significant effect on seroconversion of infants [Citation20,Citation74]. A recent meta-analysis assessing the effectiveness of withholding breastfeeding to improve oral viral vaccine performance (of the six trials included in the analysis, three trials assessed rotavirus vaccination) also found no evidence of any effect on seroconversion [Citation53]. Other studies did not show significant differences in rotavirus vaccine efficacy, which depended on rotavirus-specific antibodies passively transferred through breast milk [Citation45,Citation75–77].
It remains unclear whether rotavirus-specific IgG, IgA or neutralizing antibodies, in breast milk or serum IgG exert the greatest inhibitory effect on vaccine performance. Although rotavirus-protein specific IgG has been shown to be highly efficient in neutralizing the rotavirus in vivo and may affect vaccine efficacy [Citation78], further research is required to understand the role anti-rotavirus antibodies have on the efficacy and effectiveness of rotavirus vaccines in resource-limited settings [Citation66].
4.1.2. Blood group antigens
There is a growing body of evidence to suggest that the vaccine efficacy and effectiveness of oral rotavirus vaccine is associated with histo-blood group antigen (HBGA)-secretor and salivary ABO blood group antigen status of young infants. Rotaviruses, specifically the distal portions of rotavirus VP4 spike protein (VP8*), attach themselves to HBGAs [Citation79–81]. These HBGAs are synthesized by glycosyltransferases encoded by ABO, Lewis (FUT3/4), and secretor (FUT2) gene families. Recent investigations have suggested that the sialic acid–independent human rotaviruses recognize certain HBGAs in a P genotype–dependent manner. Epidemiologic associations have been reported between particular HBGA types and disease (or lack of disease) from particular human rotavirus P genotypes. Specifically, differences in HBGA expression are known to influence the susceptibility of the infant to infection by different rotavirus strains [Citation82]. These differences may also be responsible for some of the discrepancy in the level of protection detected for rotavirus vaccines in different settings [Citation83]. The secretor and Lewis-positive genotypes were independently associated with increased risk of rotavirus infections in Burkina Faso [Citation82] and France [Citation79] whereas the non-secretors (those having mutations in FUT2 or FUT3 leading to a total absence or a severe decrease of fucosylated antigens in mucous membranes and secretions) are less susceptible to rotavirus diarrhea [Citation79,Citation84]. During vaccination, non-secretors may not have a productive infection with the live attenuated virus to stimulate an immune response, which possibly translates into a lower vaccine efficacy. However, the distribution of HBGA genotypes is largely different in distinct ethnic groups. For instance, there are very few ‘non-secretors’ among Southeast and East Asians [Citation85,Citation86] which is why rotavirus vaccine efficacy might be affected in these regions [Citation82,Citation87]. Recently, null HBGA phenotypes have demonstrated complete resistance to rotavirus serotype P[Citation8], the sole P-antigen of human rotavirus origin contained in current rotavirus vaccines [Citation50,Citation79]. In resource-limited regions, such as sub-Saharan Africa, there is a greater prevalence of both non-P[Citation8] serotypes and null HBGA phenotypes, compared to resource-rich regions. In these regions, individuals with null HBGA phenotypes may be at increased risk of rotavirus vaccine failure [Citation50].
4.1.3. Impact of the oral polio vaccine (OPV) on rotavirus vaccine performance
Both polio and rotavirus vaccines are recommended to be given orally at the same period and as a part of the routine immunization program in early infancy. It has been speculated that the use of OPV in resource-limited countries (as opposed to the inactivated poliovirus vaccine only schedules generally adopted in resource-rich countries) contributes to the geographic discrepancies observed in rotavirus vaccine performance [Citation50]. Incidentally, studies indicate interference of the OPV resulting in the induction of lower titers of antibody to the rotavirus vaccine [Citation88,Citation89]. In another study among infants in Bangladesh who received the HRV at 6 and 10 weeks of age, concomitant OPV administration during at least one of these doses was associated with a reduction in rotavirus seroconversion rate (47%) compared with staggered vaccine administration (≥1 day apart) at both doses (63%) [Citation88]. This interference is observed to be greater after the first dose of OPV, probably due to the fact that the first dose is associated with the highest levels of intestinal replication of vaccine polio virus strains, and this interference is overcome with the administration of subsequent rotavirus vaccine doses [Citation89]. A recent analysis provides evidence that children given rotavirus vaccination alone or separated from OPV were more likely to seroconvert against rotavirus compared with children concomitantly given rotavirus and OPV vaccination [Citation53].
4.2. Pathogen factors
It is suspected that intrinsic epidemiological factors related to the pathogen-affecting populations living in resource-limited settings might be driving differences in rotavirus vaccine performance. In this section, we closely examine the role that force of infection, strain diversity, and the presence of coinfections play in determining the effectiveness of rotavirus vaccination programs in resource-limited settings.
4.2.1. Force of infection
Force of infection is defined as the rate at which an individual transitions from being susceptible to being infectious [Citation90,Citation91]. There are disparities in the force of infection of rotavirus infection. For example, one study showed that the incidence of any and severe rotavirus gastroenteritis is higher in infants from resource-limited countries or regions in the first year of life than their counterparts from resource-rich regions [Citation92]. This could be due to several factors related to children being relatively more exposed to an adverse environment such as poor sanitation and hygiene, and water accessibility in resource-limited regions as compared to their counterparts in resource-rich regions. Moreover, a very small inoculum is usually sufficient to cause active infection and therefore these children in resource-limited regions are more prone to suffer from the diarrheal disease in the early months of their life [Citation93], if they are not protected by vaccination. Early and multiple rotavirus exposure can lead to generation of an active immune response similar to maternal antibodies and potentially interfere with vaccine efficacy [Citation94]. Considering early onset of diarrheal disease among infants in impoverished settings, the existing rotavirus vaccination schedules could be a factor for reduced vaccine efficacy and effectiveness, driven by the fact that when vaccine efficacy or effectiveness is measured, infants in the control group have already been exposed to rotavirus and have developed natural immunity [Citation92].
A high force of infection coupled with low vaccination coverage as has been documented in resource-limited regions is expected to influence the vaccine effectiveness of rotavirus vaccination programs as achieving high levels of vaccine effectiveness relies on indirect protection. For example, one study in rural Bangladesh attempted to measure indirect protection with the HRV but found none with the authors noting that larger scale sustained vaccination might be required in such a high force of infection setting to obtain measurable indirect effects [Citation95]. Even within resource-limited settings, notable differences in the force of infection were observed. For example between infants from Vellore (India) and Karonga (Malawi), children in Vellore appearing to be infected earlier in their first year of life than children in Karonga [Citation96]. Identifying heterogeneities in infant rotavirus transmission patterns and patterns of incidence is important to fine-tune existing vaccination schedules to make them more relevant for impoverished settings, for example, number of vaccine doses can be increased [Citation43], the use of a neonatal dose may be considered [Citation73], or vaccination time could be scheduled earlier than what is recommended at present [Citation97].
4.2.2. Strain diversity
Oral rotavirus vaccines confer homotypic and heterotypic protection against a wide variety of rotavirus genotypes [Citation98]. Protection against rotavirus infection is mediated primarily by neutralizing antibodies that target epitopes on VP4, VP7, or both, which prevents adsorption, uncoating and penetration of the virus into enterocytes, while VP6-specific antibodies prevent intracellular viral replication during transcytosis across enterocytes. In resource-limited countries, the annual circulation of new rotavirus strains is more common [Citation32,Citation99–102]. Epidemiological studies indicate that rotavirus diversity changes over the years within and between countries by point mutations (genetic drift) leading to antigenic changes, reassortment within and between animal strains or between animal and human strains, infections of other species as well as genetic rearrangements [Citation103]. Indeed, the African Region has the highest proportion (35%) of new rotavirus strains causing diarrhea. In this region, rotavirus vaccination can provide protection against a broad spectrum of strains, but emerging strains might not be fully neutralized by antibodies raised against vaccine strains, thus leading to the lower levels of efficacy [Citation32]. A study in India also found a high proportion of rare rotavirus strains in circulation [Citation101]. Similarly, there is a high proportion of unusual P/G combinations, suggesting viral reassortment; increased zoonotic rotavirus transmission; the emergence and spread of serotype G9; and a high prevalence of the P[Citation6] VP4 genotype in Africa [Citation102]. Molecular epidemiological and bioinformatics studies indicate that rotavirus genotypes appear to be more diverse and dynamic following mass vaccination programs [Citation44]. But even in low coverage areas like in Indonesia, occurrence of disease due to new variants has been observed [Citation104]. Nonetheless, the positive impact of rotavirus vaccination programs, where rotavirus strain diversity is high confirms that strain replacement, while a potential challenge to the use of current rotavirus vaccines, may not occur, but needs sustained monitoring [Citation44].
There is some evidence suggesting that the emergence of new strains leads to a decrease in rotavirus vaccine effectiveness as was observed during rotavirus outbreaks in 2009 and 2010 in the Northern Territories of Australia. Reasons for this low vaccine effectiveness could be attributable to several factors including the emergence of rotavirus antigenic variants, which provide the first evidence of rotavirus vaccine escape mutants [Citation105]. However, a few studies show that the circulation of new strains, which are in a constant cyclical fluctuation with none predominating, has no impact on overall reduction of disease and virus transmission [Citation106,Citation107]. The role of strain diversity on vaccine efficacy needs to be studied further though the impact is limited [Citation98].
4.2.3. Coinfections
Burden of diarrheal disease due to coinfections with one or more enteric or extra-intestinal pathogens, even in apparently healthy infants in resource-limited settings, is common [Citation108], and recent studies have looked at associations between their presence and impaired immune response to rotavirus vaccines [Citation109–111]. Coinfections can affect the efficacy and effectiveness of rotavirus vaccination driven by their role in increasing the severity of diarrhea [Citation109,Citation111,Citation112]. Some studies indicate that rotavirus coinfections with bacterial enteropathogens were associated with a longer duration of the diarrheal episodes. While the accentuation of rotavirus disease severity in the presence of co-pathogens has been described previously [Citation109,Citation112], some have failed to show such an effect [Citation113]. Accordingly, rotavirus vaccine effectiveness in India increased by 11.3% when adjusted for coinfections [Citation109]. In a study conducted in Bangladesh, concurrent enterovirus infections were correlated with poor IgA seroconversion to HRV [Citation111]. While this may not directly affect vaccine effectiveness, the increased severity of diarrhea because of the other pathogens may lead to the conclusion that rotavirus is the cause of diarrhea despite the rotavirus vaccination history. In other instances, coinfections of rotaviruses with enteric pathogens such as Giardia, E. coli and Shigella can act synergistically, with the pathogenicity of the individual microbe being enhanced [Citation114,Citation115]. Children infected with C. difficile had severe clinical symptoms when co-infected with rotaviruses [Citation112]. Notwithstanding the synergistic effect, further research is required to ascertain whether coinfections with other pathogens directly affect rotavirus vaccine effectiveness. In terms of the extent to which coinfections in rotavirus efficacy trials are affecting these estimates, one study found that rotavirus vaccine efficacy against severe rotavirus gastroenteritis increased from 49.3% to 60.6% in the absence of coinfections [Citation109]. The underlying mechanism leading to impaired vaccine efficacy can only be speculated upon, based on the phenomenon of interference via the induction of nonspecific innate immunity. In particular, interferons induced by viral or bacterial pathogens may inhibit vaccine virus replication and immunogenicity through diverse mechanisms such as direct antiviral activity, innate immune cell activation, or adaptive immune response regulation. Other relevant immunomodulatory effects may include suppression of Th1 cytokine production by intestinal helminth, the promotion of mucosal tolerance through regulatory T cells induction or the functional reprogramming of innate immune cells conferring protection against reinfection (trained immunity) [Citation50].
4.3. Environmental factors
The microbes that colonize the gut and other body surfaces (microbiome) have changed with their host species to maintain a symbiotic relationship [Citation116]. The microbiome as a whole or its individual members play important roles in the development and functionality of the immune system [Citation117]. The microbiome starts developing during birth and matures by 2 years of age. Until the microbiome matures, its composition is continuously changing and is known to be affected by the environment [Citation118,Citation119]. The environmental influences of early life that affect the microbiome include diet, method of childbirth (vaginal versus cesarean section), and hygiene (clean versus unsanitary environment) [Citation120–123]. A combination of gut microbiome alterations due to environmental influences is known as intestinal or enteric dysbiosis. The latter is often accompanied by environmental enteropathy representing structural and functional disorder of the small intestine that is frequently seen in children from resource-limited countries [Citation124].
Alterations in gut microbiota composition compromises the immunogenicity of enteric live attenuated vaccines and is driven by an inefficient vaccine virus strain replication in the intestinal tract which happens in direct interaction with the intestinal microbiota [Citation125,Citation126]. Importantly, immunogenicity is associated with the ‘take’ following vaccination, which is often assessed by the magnitude of replication in the gut assessed by viral loads in the stool. Evidence from studies is starting to emerge associating the relative abundance of particular members of the gut bacteria with oral rotavirus vaccine immunogenicity. Some enhance while some others inhibit the immunogenicity of rotavirus vaccination [Citation127] through mechanisms influencing gut innate and adaptive immune responses to rotavirus vaccination, and interference by damage to the intestinal epithelium through environmental enteropathy [Citation128,Citation129].
From studies conducted in resource-limited settings, a significant correlation between the composition of the infant gut microbiome and response to rotavirus vaccination was found in Ghana [Citation130]. In 2017 and 2018, Vanessa Harris and colleagues showed in two studies that the composition of the gut microbiome of infants in both Pakistan and Ghana affected their responses to the rotavirus vaccine [Citation130,Citation131]. Certain gut bacteria have demonstrated enhanced adaptive response on vaccination, like a probiotic [Citation132]. Meanwhile, in other studies, the response to rotavirus vaccination is decreased in children presenting with biomarkers of environmental enteropathy [Citation133,Citation134]. Few studies now suggest that altering the bacterial intestinal microbiome with antibiotics can affect a vaccine’s response to natural rotavirus infection [Citation132,Citation135,Citation136]. In this case, it would be debatable in infants <6 months of age and may lead to dysbiosis or increased bacterial resistance [Citation132]. It could be hypothesized that a dysbiotic microbiome alters the replication of the vaccine virus strain, but it was not associated with changes in rotavirus-specific IgA levels, suggesting that it could have resulted from direct rotavirus–microbiota interactions. At the microbiotic level, and independently of the type of antibiotics used, the vaccine immunogenicity was associated with an increase in Enterobacteriaceae (belonging to the Proteobacteria group) and a decrease in Prevotellaceae (belonging to the Bacteroidetes group). According to the authors, virions could be stabilized by bacterial lipopolysaccharides (LPS), which would promote their reproduction and thus a stronger immune reaction. The bacterial LPS is a potent immunostimulant, and variation in the LPS composition among bacterial species significantly affects their immune stimulatory properties. More generally, antibiotics could act by reducing the number of bacteria promoting immune tolerance or increasing the proportion of bacteria promoting intestinal mucosal immunity [Citation136]. However, studies are limited to come to a definite conclusion yet.
Intestinal homeostasis can also affect vaccine effectiveness. Immune cells in the gut–associated lymphoid tissue (GALT) respond to invading microbiota by creating dendritic cell-associated-mediated T-cell and an antibody response. This triggers a cycle of inflammation; the influx of proinflammatory cells, decrease in absorption potential, and shift in the immune population impact host responses to oral antigenic challenges. Microbial translocation leads to an increase in dendritic cells with microbiota antigens, which may diminish the immunogenicity of a vaccine antigen because the immune system is already engaged in the prevention of a systemic microbial breach of the intestine [Citation137].
Enteric dysbiosis is a naturally occurring phenomenon and studies in resource-limited settings have reported that alteration of the gut microbiota or the use of antibiotics which clears the gut microbiota influences the immunogenicity of rotavirus and other vaccines. On the other hand, such phenomenon are not clearly established in resource-rich settings leading to the hypothesis that both host (overcrowding, poor water and hygiene conditions, access to balanced diet, etc.) and pathogen (co-existence with other pathogen and microbiota) environmental factors play an important role in disease severity in rotavirus diarrhea and thus impact vaccine effectiveness.
5. Conclusion
The widespread use of rotavirus vaccines since 2008 has established their value in reducing rotavirus-associated deaths and hospitalizations, regardless of setting [Citation16,Citation21,Citation22,Citation138]. The benefits of rotavirus immunization, particularly in resource-limited countries with high diarrhea burden, are indisputable [Citation44,Citation92]. Despite this positive outcome, many countries have not introduced rotavirus vaccines. In low resource countries, it is often not possible to demonstrate the potential impact of vaccination due to a range of host, pathogen and environmental factors, and to suboptimal vaccine coverage. While the evidence shows that several factors specific to children living in impoverished settings with high exposure to rotavirus infection tend to influence the performance of rotavirus vaccination programs, the evidence is debatable. Moreover, the mechanisms presented in this review do not occur in isolation and could have a combination effect on reducing vaccine efficacy and effectiveness. In this context, singular associations of specific host, pathogen and environmental factors with vaccine efficacy and effectiveness would benefit from a dedicated systematic review and meta-analysis. It is clear though that rotavirus vaccination programs will need adapting for resource-limited countries for instance, early vaccination with currently available rotavirus vaccines or with neonatal strains, which is expected to have a positive impact on vaccine efficacy [Citation42,Citation73]. In addition, the development of novel vaccine formulations such as plasmid only based reverse genetic systems to generate attenuated recombinant viruses could be considered, and interventions to improve the immune response to existing vaccines, or administration of probiotics and micronutrients, or the judicial use of antibiotics could all be carefully considered during vaccine administration based on the setting [Citation44,Citation53,Citation92,Citation139]. While there is no confirmatory evidence to substantiate the use of any of these strategies, none of these strategies have been tried in combination before in any settings. In this context, robust evidence is needed to substantiate the use of any of the strategies aiming to improve vaccine performance. These strategies may also need to reflect the diversity of mechanisms that affect the generation of immune response in order to achieve vaccine effectiveness. Although the discussions so far are promising in vaccine effectiveness enhancement, in the current state of the knowledge, no convincing solution is readily available. Additional research into nutritional factors that could prove beneficial may take years to yield acceptable results. Meanwhile, an immediate solution to overcome the modest levels of vaccine efficacy and effectiveness is to increase the immunization coverage to higher values where generating an overall effect of adequate proportion of protected population to break rotavirus transmission.
6. Expert opinion
Disease epidemiology follows a classical interaction between the host, pathogen, and the environment. The progression of the disease as well as its containment depends on how these factors are addressed both independently and through an all-inclusive approach. The vicious cycle of malnutrition and diarrheal infection that begins at birth, leading to vitamin, nutrition complement, and immune deficiency should be taken into consideration. The debate continues over what precedes the other and how and when the cycle can be broken. The interference of maternal antibodies during the early stage of infancy may pose a hindrance to vaccination response. The inherent or ethnic specificity of a given region also cannot be ignored, nor the nature of oral vaccines administered simultaneously in early childhood. Competition for cell entry or receptor binding, or (after entry) for cellular factors required for transcription, replication, and assembly are naturally occurring physiological phenomenon that cannot be altered by any simple approach.
The available vaccines provide immunity through mimicking a natural infection. Interestingly, in resource-limited settings, most children are infected by rotavirus at an early age leading to different degrees of disease (mild, moderate, or severe). Thus, identifying a completely naïve control to compare with a rotavirus diarrhea case and estimation of vaccine efficacy in a resource-limited setting always poses a challenge.
The force of transmission of the virus, given a particular threshold, influences the severity of disease. Increasing vaccination coverage could decrease both the number of susceptible individuals in a targeted age-group and the severity of the disease. Available vaccines protect against various genotypes, but the strains circulating in resource-limited settings are diverse. A high proportion of unusual P/G combination can be observed in such settings, possibly due to viral reassortment leading to the emergence of new wild-type viruses. Consequently, studies have failed to concretely establish that vaccine introduction may lead to strain diversity. Despite this knowledge, the low efficacy of vaccines against those unusual strains cannot be ruled out. Coinfections with other enteric pathogens is common in resource-limited settings. This may reduce or interfere with the immune response to rotavirus vaccine, and the vaccine failure being established as the cause for severe diarrhea, when, in fact, they are being caused by other enteric co-pathogens.
The enteric dysbiosis due to the environment within which both the host and pathogen reside plays an important role in disease progression. Overcrowding, access to clean water, sanitation, and hygiene inversely affect the immune system of the host indirectly. The gut environment is influenced by the existence of microbiota and the use of antibiotics, which affect the proliferation of some microorganisms in the gut. Thus, if a balance in the environments of the host and pathogen cannot be maintained, the resulting severity of the disease and the failure of its containment may be attributed only to rotavirus vaccine effectiveness.
Rotavirus remains the leading cause of diarrhea in young children, especially in resource-limited settings, despite improvements in disease management (rehydration and zinc supplementation) and prevention through vaccination. Access to safe drinking water, development of sanitation and improvement of overall nutritional status in a population has drastically changed the scenario in resource-rich settings. While all these are expected equally in resource-limited settings, other socio-political compelling reasons will deter those to happen for a foreseeable future. While rotavirus vaccines can reduce the severity of the disease, in resource-limited settings other factors must also be taken into consideration (as described in this article). Unfortunately, vaccine efficacy and effectiveness are potentially low in such geographical locations. Despite this fact, high vaccine coverage may substantially support bringing down the annual incidence of rotavirus diarrhea in the affected population, while simultaneously addressing other host, pathogen and environmental factors (). There is published evidence that shows that altering the bacterial intestinal microbiome with antibiotics can affect a vaccine’s immunogenicity; thus, a strategy for the judicial use of antibiotics may be developed to increase immunogenicity and improve the microbiota in the gut, but this strategy in children below 6 months of age is debatable. Further research into vaccine efficacy and effectiveness in developing countries that addresses specific host, pathogen, and environmental factors could support country-level decision-making for rotavirus vaccine introduction in resource-limited settings.
ARTICLE HIGHLIGHTS
Despite the availability and widespread use of rotavirus vaccines, rotavirus disease burden remains high in countries in sub-Saharan Africa and regions of Asia. In these resource-limited regions, among the main barriers to achieving the full potential of rotavirus vaccination is the reduced efficacy and effectiveness of rotavirus vaccines.
Factors related to the human host (malnutrition, maternally derived antibodies, genetic factors, blood group, and co-administration with oral polio vaccine), rotavirus pathogen (force of infection, strain diversity and coinfections) and the environment (related to the human microbiome) may lead to diminished vaccine efficacy in resource-limited settings.
Rotavirus vaccination programs should consider the interaction between the host, pathogen, and environmental factors described in this review to be successfully adapted in resource-limited countries to improve impact.
While efforts are being made to improve the various factors for optimizing the effect of rotavirus vaccines, achieving higher immunization coverage rates could be an immediate solution to providing the required herd immunity to curtail the burden of disease.
Declaration of interest
All authors are employed by the GSK group of companies and hold shares in the GSK group of companies. The authors declare no other financial and non-financial relationships and activities.
Author contributions
All authors contributed to the conception and design of the manuscript. MOCO, PP, PB, and SB contributed to the literature search and interpretation of the data. All authors revised the article critically for important intellectual content and provided final approval of the submitted version.
Reviewer disclosures
Peer reviewers on this manuscript have no relevant financial or other relationships to disclose.
Trademarks
Rotarix is a trademark owned by or licensed to the GSK group of companies. Rotateq is a trademark owned by or licensed to Merck & Co. Inc. Rotavac is a trademark owned by or licensed to Bharat Biotech International. Rotasiil is a trademark owned by or licensed to Serum Institute of India Ltd. Rotavin-M1 is a trademark owned by or licensed to Polyvac, Vietnam. Lanzhou lamb rotavirus vaccine is a trademark owned by or licensed to Lanzhou Institute of Biological Products, China.
Acknowledgments
The authors thank the Business & Decision Life Sciences platform for editorial assistance and manuscript coordination, on behalf of GSK. Amandine Radziejwoski coordinated the publications development and editorial support. Amrita Ostawal (Arete Communication UG, on behalf of GSK) provided medical writing support.
Additional information
Funding
References
- Lim ML, Wallace MR. Infectious diarrhea in history. Infect Dis Clin North Am. 2004;18(2):261–274.
- Bishop RF, Davidson GP, Holmes IH, et al. Virus particles in epithelial cells of duodenal mucosa from children with acute non-bacterial gastroenteritis. Lancet. 1973;2(7841):1281–1283.
- Flewett TH, Bryden AS, Davies H, et al. Relation between viruses from acute gastroenteritis of children and newborn calves. Lancet. 1974;2(7872):61–63.
- International Committee on Taxonomy of Viruses. 2021. https://talk.ictvonline.org/taxonomy/. [Accessed: 2021 04 21].
- Lundgren O, Svensson L. Pathogenesis of rotavirus diarrhea. Microbes Infect. 2001;3(13):1145–1156.
- Suzuki T, Inoue D. Full genome-based genotyping system for rotavirus H and detection of potential gene recombination in nonstructural protein 3 between porcine rotavirus H and rotavirus C. J Gen Virol. 2018;99(12):1582–1589.
- Flores PS, Costa FB, Amorim AR, et al. Rotavirus A, C, and H in Brazilian pigs: potential for zoonotic transmission of RVA. J Vet Diagn Invest. 2021;33(1):129–135.
- Sadiq A, Bostan N, Yinda KC, et al. Rotavirus: genetics, pathogenesis and vaccine advances. Rev Med Virol. 2018;28(6):e2003.
- Rotavirus classification working group. List of Accepted Genotypes. https://rega.kuleuven.be/cev/viralmetagenomics/virus-classification/rcwg. [ Accessed: 26.03.2021].
- Yen C, Cortese MM. 216 - Rotaviruses. Principles and Practice of Pediatric Infectious Diseases. (Fourth. Long SS, editor. Content Repository Only!. Edinburgh ; New York : Elsevier Churchill Livingstone:2012. p. 1094–7.e3.
- Matthijnssens J, Heylen E, Zeller M, et al. Phylodynamic analyses of rotavirus genotypes G9 and G12 underscore their potential for swift global spread. Mol Biol Evol. 2010;27(10):2431–2436.
- Ramig RF. Pathogenesis of Intestinal and Systemic Rotavirus Infection. J Virol. 2004;78(19):10213–10220.
- Korsman SNJ, Van Zyl GU, Nutt L, et al. Gastrointestinal illness. In: Korsman SNJ, Van Zyl GU, Nutt L, et al.editors. Virology. Edinburgh: Churchill Livingstone; 2012. p. 106–107.
- Parashar UD, Hummelman EG, Bresee JS, et al. Global illness and deaths caused by rotavirus disease in children. Emerg Infect Dis. 2003;9(5):565–572.
- Parashar UD, Gibson CJ, Bresee JS, et al. Rotavirus and severe childhood diarrhea. Emerg Infect Dis. 2006;12(2):304–306.
- Troeger C, Khalil IA, Rao PC, et al. Rotavirus Vaccination and the Global Burden of Rotavirus Diarrhea Among Children Younger Than 5 Years. JAMA Pediatr. 2018;172(10): 958–965.
- World Health Organization. Rotavirus vaccines WHO position paper: January 2013 - Recommendations. Vaccine. 2013;31(52):6170–6171.
- Hartling L, Bellemare S, Wiebe N, et al. Oral versus intravenous rehydration for treating dehydration due to gastroenteritis in children. Cochrane Database Syst Rev. 2006;2006(3):CD004390.
- Lamberti LM, Walker CL, Chan KY, et al. Oral zinc supplementation for the treatment of acute diarrhea in children: a systematic review and meta-analysis. Nutrients. 2013;5(11):4715–4740.
- Groome MJ, Moon SS, Velasquez D, et al. Effect of breastfeeding on immunogenicity of oral live-attenuated human rotavirus vaccine: a randomized trial in HIV-uninfected infants in Soweto, South Africa. Bull World Health Organ. 2014;92(4):238–245.
- Burke RM, Tate JE, Kirkwood CD, et al. Current and new rotavirus vaccines. Curr Opin Infect Dis. 2019;32(5):435–444.
- Burnett E, Parashar UD, Tate JE. Real-world effectiveness of rotavirus vaccines, 2006-19: a literature review and meta-analysis. Lancet Glob Health. 2020;8(9):e1195–e1202.
- World Health Organization. Meeting of the immunization Strategic Advisory Group of Experts, April 2009 – conclusions and recommendations. Wkly Epidemiol Rec. 2009;84(23):213-236.
- World Health Organization. Meeting of the immunization Strategic Advisory Group of Experts, November 2006 – conclusions and recommendations. Wkly Epidemiol Rec. 2007;1-2(82):1–16.
- International Vaccine Access Center (IVAC). VIEW-hub Global Vaccine Introduction Report. 2020. https://www.jhsph.edu/ivac/. [Accessed: 2020 12 16].
- Troeger C, Blacker BF, Khalil IA, et al. Estimates of the global, regional, and national morbidity, mortality, and aetiologies of diarrhoea in 195 countries: a systematic analysis for the Global Burden of Disease Study 2016. Lancet Infect Dis. 2018;18(11):1211–1228.
- Thirteenth International Rotavirus Symposium. Expanding Rotavirus Vaccine Impact: Improving Coverage and Equity [Internet]. 2018. https://www.sabin.org/sites/sabin.org/files/resources/rota2018report_final.pdf. [Accessed: 2021 03 27].
- Troeger C, Forouzanfar M, Rao PC, et al. Estimates of global, regional, and national morbidity, mortality, and aetiologies of diarrhoeal diseases: a systematic analysis for the Global Burden of Disease Study 2015. Lancet Infect Dis. 2017;17(9):909–948.
- Tate JE, Burton AH, Boschi-Pinto C, et al. Global, regional, and national estimates of rotavirus mortality in children <5 years of age, 2000-2013. Clin Infect Dis. 2016;62(Suppl 2):S96–s105.
- Buchy P, Chen J, Zhang X-H, et al. A review of rotavirus vaccine use in Asia and the Pacific regions: challenges and future prospects. Expert Rev Vaccines. 2021.
- Armah GE, Sow SO, Breiman RF, et al. Efficacy of pentavalent rotavirus vaccine against severe rotavirus gastroenteritis in infants in developing countries in sub-Saharan Africa: a randomised, double-blind, placebo-controlled trial. Lancet. 2010;376(9741):606–614.
- Madhi S, Cunliffe N, Steele D, et al. Effect of human rotavirus vaccine on severe diarrhea in African infants. New Engl J Med. 2010;362(4):289–298.
- Zaman K, Dang DA, Victor JC, et al. Efficacy of pentavalent rotavirus vaccine against severe rotavirus gastroenteritis in infants in developing countries in Asia: a randomised, double-blind, placebo-controlled trial. Lancet. 2010;376(9741):615–623.
- Leshem E, Moritz RE, Curns AT, et al. Rotavirus vaccines and health care utilization for diarrhea in the United States (2007–2011). Pediatrics. 2014;134(1):15–23.
- Rha B, Tate JE, Payne DC, et al. Effectiveness and impact of rotavirus vaccines in the United States - 2006-2012. Expert Rev Vaccines. 2014;13(3):365–376.
- Vesikari T, Karvonen A, Prymula R, et al. Efficacy of human rotavirus vaccine against rotavirus gastroenteritis during the first 2 years of life in European infants: randomised, double-blind controlled study. Lancet. 2007;370(9601):1757–1763.
- Vesikari T, Matson DO, Dennehy P, et al. Safety and efficacy of a pentavalent human-bovine (WC3) reassortant rotavirus vaccine. N Engl J Med. 2006;354(1):23–33.
- Levine MM. Immunogenicity and efficacy of oral vaccines in developing countries: lessons from a live cholera vaccine. BMC Biol. 2010;8(1):129.
- Clark A, van Zandvoort K, Flasche S, et al. Efficacy of live oral rotavirus vaccines by duration of follow-up: a meta-regression of randomised controlled trials. Lancet Infect Dis. 2019;19(7): 717–727.
- Lamberti LM, Ashraf S, Walker CL, et al. A Systematic Review of the Effect of Rotavirus Vaccination on Diarrhea Outcomes Among Children Younger Than 5 Years. Pediatr Infect Dis J. 2016;35(9):992–998.
- Desselberger U. Differences of Rotavirus Vaccine Effectiveness by Country: likely Causes and Contributing Factors. Pathogens. 2017;6(4):65.
- Soares-Weiser K, Bergman H, Henschke N, et al. Vaccines for preventing rotavirus diarrhoea: vaccines in use. Cochrane Database Syst Rev. 2019;3(3):Cd008521.
- Lopman BA, Pitzer VE, Sarkar R, et al. Understanding reduced rotavirus vaccine efficacy in low socio-economic settings. PLOS ONE. 2012;7(8):e41720.
- Steele AD, Victor JC, Carey ME, et al. Experiences with rotavirus vaccines: can we improve rotavirus vaccine impact in developing countries? Hum Vaccin Immunother. 2019;15(6): 1215–1227.
- Gruber JF, Hille DA, Liu GF, et al. Heterogeneity of rotavirus vaccine efficacy among infants in developing countries. Pediatr Infect Dis J. 2017;36(1):72–78.
- Katona P, Katona-Apte J. The Interaction between Nutrition and Infection. Clin Inf Dis. 2008;46(10):1582–1588.
- Black RE, Victora CG, Walker SP, et al. Maternal and child undernutrition and overweight in low-income and middle-income countries. Lancet. 2013;382(9890):427–451.
- Prendergast AJ. Malnutrition and vaccination in developing countries. Philos Trans R Soc Lond B Biol Sci. 2015;370(1671):20140141.
- Hoest C, Seidman JC, Pan W, et al. Evaluating associations between vaccine response and malnutrition, gut function, and enteric infections in the MAL-ED cohort study: methods and challenges. Clin Infect Dis. 2014;59(Suppl4):S273–9.
- Parker EP, Ramani S, Lopman BA, et al. Causes of impaired oral vaccine efficacy in developing countries. Future Microbiol. 2018;13(1):97–118.
- Young GP, Mortimer EK, Gopalsamy GL, et al. Zinc deficiency in children with environmental enteropathy-development of new strategies: report from an expert workshop. Am J Clin Nutr. 2014;100(4):1198–1207.
- Fraker PJ, King LE. Reprogramming of the immune system during zinc deficiency. Annu Rev Nutr. 2004;24:277–298.
- Church JA, Parker EP, Kirkpatrick BD, et al. Interventions to improve oral vaccine performance: a systematic review and meta-analysis. Lancet Infect Dis. 2019;19(2): 203–214.
- Bosomprah S, Beach LB, Beres LK, et al. Findings from a comprehensive diarrhoea prevention and treatment programme in Lusaka, Zambia. BMC Public Health. 2016;16:475.
- Colgate ER, Haque R, Dickson DM, et al. Delayed dosing of oral rotavirus vaccine demonstrates decreased risk of rotavirus gastroenteritis associated with serum zinc: a randomized controlled trial. Clin Infect Dis. 2016;63(5):634–641.
- Mora JR, Iwata M, von Andrian UH. Vitamin effects on the immune system: vitamins A and D take centre stage. Nat Rev Immunol. 2008;8(9):685–698.
- Surman SL, Penkert RR, Jones BG, et al. Vitamin supplementation at the time of immunization with a cold-adapted influenza virus vaccine corrects poor mucosal antibody responses in mice deficient for vitamins A and D. Clin Vaccine Immunol. 2016;23(3):219–227.
- Chattha KS, Kandasamy S, Vlasova AN, et al. Vitamin A deficiency impairs adaptive B and T cell responses to a prototype monovalent attenuated human rotavirus vaccine and virulent human rotavirus challenge in a gnotobiotic piglet model. PLOS ONE. 2013;8(12):e82966.
- Zhao Y, Yu B, Mao X, et al. Dietary vitamin D supplementation attenuates immune responses of pigs challenged with rotavirus potentially through the retinoic acid-inducible gene I signalling pathway. Br J Nutr. 2014;112(3):381–389.
- Kandasamy S, Chattha KS, Vlasova AN, et al. Prenatal vitamin A deficiency impairs adaptive immune responses to pentavalent rotavirus vaccine (RotaTeq®) in a neonatal gnotobiotic pig model. Vaccine. 2014;32(7):816–824.
- Vlasova AN, Chattha KS, Kandasamy S, et al. Prenatally acquired vitamin A deficiency alters innate immune responses to human rotavirus in a gnotobiotic pig model. J Immunol. 2013;190(9):4742–4753.
- Guerrant RL, DeBoer MD, Moore SR, et al. The impoverished gut--a triple burden of diarrhoea, stunting and chronic disease. Nat Rev Gastroenterol Hepatol. 2013;10(4):220–229.
- Jensen KJ, Ndure J, Plebanski M, et al. Heterologous and sex differential effects of administering vitamin A supplementation with vaccines. Trans R Soc Trop Med Hyg. 2015;109(1):36–45.
- Durandy A. Ontogeny of the immune system. Transfus Med Hemother. 2003;30(5):222–227.
- Mwila K, Chilengi R, Simuyandi M, et al. Contribution of maternal immunity to decreased rotavirus vaccine performance in low- and middle-income countries. Clin Vaccine Immunol. 2017;24(1):e00405–16.
- Chan J, Nirwati H, Triasih R, et al. Maternal antibodies to rotavirus: could they interfere with live rotavirus vaccines in developing countries? Vaccine. 2011;29(6):1242–1247.
- Becker-Dreps S, Vilchez S, Velasquez D, et al. Rotavirus-specific IgG antibodies from mothers’ serum may inhibit infant immune responses to the pentavalent rotavirus vaccine. Pediatr Infect Dis J. 2015;34(1):115–116.
- Appaiahgari MB, Glass R, Singh S, et al. Transplacental rotavirus IgG interferes with immune response to live oral rotavirus vaccine ORV-116E in Indian infants. Vaccine. 2014;32(6):651–656.
- Moon SS, Groome MJ, Velasquez DE, et al. Prevaccination rotavirus Serum IgG and IgA are associated with lower immunogenicity of live, oral human rotavirus vaccine in South African infants. Clin Infect Dis. 2016;62(2):157–165.
- Chen MY, Kirkwood CD, Bines J, et al. Rotavirus specific maternal antibodies and immune response to RV3-BB neonatal rotavirus vaccine in New Zealand. Hum Vaccin Immunother. 2017;13(5):1126–1135.
- Gruber JF, Gruber LM, Weber RP, et al. Rotavirus vaccine schedules and vaccine response among infants in low- and middle-income countries: a systematic review. Open Forum Infect Dis. 2017;4(2):ofx066.
- Siegrist C-A, Aspinall R. B-cell responses to vaccination at the extremes of age. Nat Rev Immunol. 2009;9(3):185–194.
- Bines JE, At Thobari J, Satria CD, et al. Human Neonatal Rotavirus Vaccine (RV3-BB) to Target Rotavirus from Birth. New Engl J Med. 2018;378(8):719–730.
- Rongsen-Chandola T, Strand TA, Goyal N, et al. Effect of withholding breastfeeding on the immune response to a live oral rotavirus vaccine in North Indian infants. Vaccine. 2014;32(Suppl 1):A134–9.
- Goveia MG, DiNubile MJ, Dallas MJ, et al. Efficacy of pentavalent human-bovine (WC3) reassortant rotavirus vaccine based on breastfeeding frequency. Pediatr Infect Dis J. 2008;27(7):656–658.
- Vesikari T, Prymula R, Schuster V, et al. Efficacy and immunogenicity of live-attenuated human rotavirus vaccine in breast-fed and formula-fed European infants. Pediatr Infect Dis J. 2012;31(5):509–513.
- Rennels MB, Wasserman SS, Glass RI, et al. Comparison of immunogenicity and efficacy of rhesus rotavirus reassortant vaccines in breastfed and nonbreastfed children. US Rotavirus Vaccine Efficacy Group. Pediatrics. 1995;96(6):1132–1136.
- Caddy SL, Vaysburd M, Wing M, et al. Intracellular neutralisation of rotavirus by VP6-specific IgG. PLoS Pathog. 2020;16(8):e1008732.
- Imbert-Marcille BM, Barbé L, Dupé M, et al. A FUT2 gene common polymorphism determines resistance to rotavirus A of the P[8] genotype. J Infect Dis. 2014;209(8):1227–1230.
- Hu L, Crawford SE, Czako R, et al. Cell attachment protein VP8* of a human rotavirus specifically interacts with A-type histo-blood group antigen. Nature. 2012;485(7397):256–259.
- Ramani S, Hu L, Venkataram Prasad BV, et al. Diversity in rotavirus-host glycan interactions: a “sweet” spectrum. Cell Mol Gastroenterol Hepatol. 2016;2(3):263–273.
- Nordgren J, Sharma S, Bucardo F, et al. Both Lewis and secretor status mediate susceptibility to rotavirus infections in a rotavirus genotype-dependent manner. Clin Infect Dis. 2014;59(11):1567–1573.
- Kazi AM, Cortese MM, Yu Y, et al. Secretor and salivary ABO blood group antigen status predict rotavirus vaccine take in infants. J Infect Dis. 2017;215(5):786–789.
- Trang NV, Vu HT, Le NT, et al. Association between Norovirus and rotavirus infection and histo-blood group antigen types in Vietnamese children. J Clin Microbiol. 2014;52(5):1366–1374.
- Chang JG, Yang TY, Liu TC, et al. Molecular analysis of secretor type alpha(1,2)-fucosyltransferase gene mutations in the Chinese and Thai populations. Transfusion. 1999;39(9):1013–1017.
- Yu LC, Chu CC, Chan YS, et al. Polymorphism and distribution of the Secretor alpha(1,2)-fucosyltransferase gene in various Taiwanese populations. Transfusion. 2001;41(10):1279–1284.
- Kambhampati A, Payne DC, Costantini V, et al. Host genetic susceptibility to enteric viruses: a systematic review and metaanalysis. Clin Infect Dis. 2016;62(1):11–18.
- Emperador DM, Velasquez DE, Estivariz CF, et al. Interference of monovalent, bivalent, and trivalent oral poliovirus vaccines on monovalent rotavirus vaccine immunogenicity in rural Bangladesh. Clin Infect Dis. 2016;62(2):150–156.
- Patel M, Steele AD, Parashar UD. Influence of oral polio vaccines on performance of the monovalent and pentavalent rotavirus vaccines. Vaccine. 2012;30(Suppl 1):A30–5.
- Hens N, Aerts M, Faes C, et al. Seventy-five years of estimating the force of infection from current status data. Epidemiol Infect. 2010;138(6):802–812.
- Muench H. Derivation of Rates from Summation Data by the Catalytic Curve. J Am Stat Assoc. 1934;29(185):25–38.
- Steele AD, Madhi SA, Cunliffe NA, et al. Incidence of rotavirus gastroenteritis by age in African, Asian and European children: relevance for timing of rotavirus vaccination. Hum Vaccin Immunother. 2016;12(9):2406–2412.
- Payment P, Morin É. Minimal infective dose of the OSU strain of porcine rotavirus. Arch Virol. 1990;112(3):277–282.
- Velázquez FR, Matson DO, Calva JJ, et al. Rotavirus infection in infants as protection against subsequent infections. N Engl J Med. 1996;335(14):1022–1028.
- Zaman K, Sack DA, Neuzil KM, et al. Effectiveness of a live oral human rotavirus vaccine after programmatic introduction in Bangladesh: a cluster-randomized trial. PLoS Med. 2017;14(4):e1002282.
- Bennett A, Nagelkerke N, Heinsbroek E, et al. Estimating the incidence of rotavirus infection in children from India and Malawi from serial anti-rotavirus IgA titres. PLOS ONE. 2017;12(12):e0190256.
- Cherian T, Wang S, Mantel C. Rotavirus vaccines in developing countries: the potential impact, implementation challenges, and remaining questions. Vaccine. 2012;30(Suppl 1):A3–6.
- Vojtek I, Buchy P, Doherty TM, et al. Would immunization be the same without cross-reactivity? Vaccine. 2019;37(4):539–549.
- Kang G, Desai R, Arora R, et al. Diversity of circulating rotavirus strains in children hospitalized with diarrhea in India, 2005–2009. Vaccine. 2013;31(27):2879–2883.
- Santos N, Hoshino Y. Global distribution of rotavirus serotypes/genotypes and its implication for the development and implementation of an effective rotavirus vaccine. Rev Med Virol. 2005;15(1):29–56.
- Gupta S, Chaudhary S, Bubber P, et al. Epidemiology and genetic diversity of group A rotavirus in acute diarrhea patients in pre-vaccination era in Himachal Pradesh, India. Vaccine. 2019;37(36):5350–5356.
- Todd S, Page NA, Steele AD, et al. Rotavirus strain types circulating in Africa: review of studies published during 1997–2006. J Infect Dis. 2010;202(Suppl):S34–S42.
- Kirkwood CD. Genetic and antigenic diversity of human rotaviruses: potential impact on vaccination programs. J Infect Dis. 2010;202(Suppl):S43–8.
- Athiyyah AF, Utsumi T, Wahyuni RM, et al. Molecular epidemiology and clinical features of rotavirus infection among pediatric patients in East Java, Indonesia during 2015-2018: dynamic changes in rotavirus genotypes from equine-Like G3 to typical human G1/G3. Front Microbiol. 2019;10:940.
- Donato CM, Cowley D, Snelling TL, et al. Characterization of a G1P[8] rotavirus causing an outbreak of gastroenteritis in the Northern Territory, Australia, in the vaccine era. Emerg Microbes Infect. 2014;3(7):e47.
- Hull JJ, Teel EN, Kerin TK, et al. United States rotavirus strain surveillance from 2005 to 2008: genotype prevalence before and after vaccine introduction. Pediatr Infect Dis J. 2011;30(1 Suppl):S42–7.
- Pitzer VE, Bilcke J, Heylen E, et al. Did large-scale vaccination drive changes in the circulating rotavirus population in Belgium? Sci Rep. 2015;5(18585). DOI:https://doi.org/10.1038/srep18585
- Kotloff KL, Nataro JP, Blackwelder WC, et al. Burden and aetiology of diarrhoeal disease in infants and young children in developing countries (the Global Enteric Multicenter Study, GEMS): a prospective, case-control study. Lancet. 2013;382(9888):209–222.
- Praharaj I, Platts-Mills JA, Taneja S, et al. Diarrheal etiology and impact of coinfections on rotavirus vaccine efficacy estimates in a clinical trial of a monovalent human-bovine (116E) oral rotavirus vaccine, Rotavac, India. Clin Infect Dis. 2019;69(2):243–250.
- Mokomane M, Tate JE, Steenhoff AP, et al. Evaluation of the influence of gastrointestinal coinfections on rotavirus vaccine effectiveness in Botswana. Pediatr Infect Dis J. 2018;37(3):e58–e62.
- Taniuchi M, Platts-Mills JA, Begum S, et al. Impact of enterovirus and other enteric pathogens on oral polio and rotavirus vaccine performance in Bangladeshi infants. Vaccine. 2016;34(27):3068–3075.
- Valentini D, Vittucci AC, Grandin A, et al. Coinfection in acute gastroenteritis predicts a more severe clinical course in children. Eur J Clin Microbiol Infect Dis. 2013;32(7):909–915.
- Moyo SJ, Kommedal Ø, Blomberg B, et al. Comprehensive analysis of prevalence, epidemiologic characteristics, and clinical characteristics of monoinfection and coinfection in diarrheal diseases in children in Tanzania. Am J Epidemiol. 2017;186(9):1074–1083.
- Bhavnani D, Goldstick JE, Cevallos W, et al. Synergistic effects between rotavirus and coinfecting pathogens on diarrheal disease: evidence from a community-based study in northwestern Ecuador. Am J Epidemiol. 2012;176(5):387–395.
- Vasco G, Trueba G, Atherton R, et al. Identifying etiological agents causing diarrhea in low income Ecuadorian communities. Am J Trop Med Hyg. 2014;91(3):563–569.
- Brown EM, Sadarangani M, Finlay BB. The role of the immune system in governing host-microbe interactions in the intestine. Nat Immunol. 2013;14(7):660–667.
- Jamieson AM. Influence of the microbiome on response to vaccination. Hum Vaccin Immunother. 2015;11(9):2329–2331.
- Subramanian S, Huq S, Yatsunenko T, et al. Persistent gut microbiota immaturity in malnourished Bangladeshi children. Nature. 2014;510(7505):417–421.
- Yatsunenko T, Rey FE, Manary MJ, et al. Human gut microbiome viewed across age and geography. Nature. 2012;486(7402):222–227.
- Guaraldi F, Salvatori G. Effect of breast and formula feeding on gut microbiota shaping in newborns. Front Cell Infect Microbiol. 2012;2(94). DOI:https://doi.org/10.3389/fcimb.2012.00094
- Jost T, Lacroix C, Braegger CP, et al. New insights in gut microbiota establishment in healthy breast fed neonates. PLOS ONE. 2012;7(8):e44595.
- Penders J, Thijs C, Vink C, et al. Factors influencing the composition of the intestinal microbiota in early infancy. Pediatrics. 2006;118(2):511–521.
- Tasnim N, Abulizi N, Pither J, et al. Linking the gut microbial ecosystem with the environment: does gut health depend on where we live? Front Microbiol. 2017;8(1935). DOI:https://doi.org/10.3389/fmicb.2017.01935
- Tickell KD, Atlas HE, Walson JL. Environmental enteric dysfunction: a review of potential mechanisms, consequences and management strategies. BMC Med. 2019;17(1):181.
- Vlasova AN, Takanashi S, Miyazaki A, et al. How the gut microbiome regulates host immune responses to viral vaccines. Curr Opin Virol. 2019;37:16–25.
- Iturriza-Gómara M, Cunliffe NA. The Gut Microbiome as Possible Key to Understanding and Improving Rotavirus Vaccine Performance in High–Disease Burden Settings. J Infect Dis. 2017;215(1):8–10.
- Magwira CA, Taylor MB. Composition of gut microbiota and its influence on the immunogenicity of oral rotavirus vaccines. Vaccine. 2018;36(24):3427–3433.
- Korpe PS, Petri WA Jr. Environmental enteropathy: critical implications of a poorly understood condition. Trends Mol Med. 2012;18(6):328–336.
- Lin A, Bik EM, Costello EK, et al. Distinct distal gut microbiome diversity and composition in healthy children from Bangladesh and the United States. PLOS ONE. 2013;8(1):e53838.
- Harris VC, Armah G, Fuentes S, et al. Significant correlation between the infant gut microbiome and rotavirus vaccine response in rural Ghana. J Infect Dis. 2017;215(1):34–41.
- Harris V, Ali A, Fuentes S, et al. Rotavirus vaccine response correlates with the infant gut microbiota composition in Pakistan. Gut Microbes. 2018;9(2):93–101.
- Desselberger U. The Mammalian intestinal microbiome: composition, interaction with the immune system, significance for vaccine efficacy, and potential for disease therapy. Pathogens. 2018;7(3):3.
- Becker-Dreps S, Vilchez S, Bucardo F, et al. The association between fecal biomarkers of environmental enteropathy and rotavirus vaccine response in Nicaraguan infants. Pediatr Infect Dis J. 2017;36(4):412–416.
- Naylor C, Lu M, Haque R, et al. Environmental enteropathy, oral vaccine failure and growth faltering in infants in Bangladesh. EBioMedicine. 2015;2(11):1759–1766.
- Uchiyama R, Chassaing B, Zhang B, et al. Antibiotic treatment suppresses rotavirus infection and enhances specific humoral immunity. J Infect Dis. 2014;210(2):171–182.
- Harris VC, Haak BW, Handley SA, et al. Effect of antibiotic-mediated microbiome modulation on rotavirus vaccine immunogenicity: a human, randomized-control proof-of-concept trial. Cell Host Microbe. 2018;24(2):197–207.
- Valdez Y, Brown EM, Finlay BB. Influence of the microbiota on vaccine effectiveness. Trends Immunol. 2014;35(11):526–537.
- Aliabadi N, Antoni S, Mwenda JM, et al. Global impact of rotavirus vaccine introduction on rotavirus hospitalisations among children under 5 years of age, 2008-16: findings from the Global Rotavirus Surveillance Network. Lancet Glob Health. 2019;7(7):e893–e903.
- Desselberger U. Potential of plasmid only based reverse genetics of rotavirus for the development of next-generation vaccines. Curr Opin Virol. 2020;44:1–6.