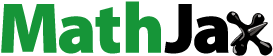
ABSTRACT
Background
V114 (15-valent pneumococcal conjugate vaccine [PCV15]) and a 20-valent PCV (PCV20) are approved for adults (≥18 years) in the United States. We present methodologies to indirectly compare immune responses to V114 versus PCV20.
Research design and methods
Indirect treatment comparison and matching-adjusted indirect comparison (MAIC) were performed to estimate opsonophagocytic activity (OPA) geometric mean titer (GMT) ratios of V114/PCV20 at 30 days post-vaccination with PCV13 as common comparator for 13 serotypes (STs) shared with a 13-valent PCV (PCV13) among pneumococcal vaccine-naïve adults aged ≥60 years. Data from three V114 studies were pooled (V114, N = 2,196; PCV13, N = 843). In the MAIC analysis, data were reweighted, matching participant age and sex in NCT03760146 (PCV20, N = 1,507; PCV13, N = 1,490).
Results
The lower bound of V114/PCV20 OPA GMT ratio for all PCV13 STs is greater than the prespecified 0.5 non-inferiority margin and those for five PCV13 STs (3, 6A, 6B, 18C, and 23F) are greater than the prespecified 1.2 superiority margin. V114 was associated with 77% greater OPA GMT for ST3 versus PCV20.
Conclusion
V114 was non-inferior to PCV20 for all PCV13 STs and statistically superior for five PCV13 STs.
1. Introduction
The morbidity and mortality of noninvasive and invasive pneumococcal disease (PD) are high, particularly in infants and children <5 years of age and older adults [Citation1–5]. The introduction of a pneumococcal conjugate vaccine (PCV) containing seven Streptococcus pneumoniae serotypes (STs) into infant vaccination programs in the 2000s led to a substantial reduction in the incidence of noninvasive and invasive PD caused by these STs, both in those <5 years of age and indirectly in older children and adults not targeted by the vaccination program [Citation6–8]. However, a subsequent increase in the relative incidence of residual disease caused by non-vaccine STs necessitated the development of new PCVs containing additional STs [Citation9]. A 13-valent PCV (PCV13, Prevnar13TM) was introduced in Europe and the United States in 2009/2010, resulting in further reductions in disease burden [Citation10–12]. Two different immunization approaches, 23-valent pneumococcal polysaccharide vaccine (PPSV23) given alone or following PCV13 as a sequential administration, were later recommended for use among older adults (≥65 years of age), immunocompromised individuals, and those who have certain chronic conditions [Citation10,Citation13]. In recent years, the emergence of non-PCV13 STs [Citation1,Citation14], as well as breakthrough disease caused by certain PCV13 STs [Citation7,Citation11,Citation15,Citation16], has highlighted the need for the development of new PCVs that would maintain or improve protection against disease caused by the STs in the licensed PCVs but also extend disease coverage to emerging non-vaccine STs [Citation17].
Two PCVs, V114 (VAXNEUVANCE™, Merck & Co., Inc., Kenilworth, NJ, USA; a 15-valent PCV containing the 13 STs included in the licensed PCV13 [STs 1, 3, 4, 5, 6A, 6B, 7F, 9V, 14, 18C, 19A, 19F, and 23F] and two additional STs [22F and 33F], which are important contributors to residual PD [Citation7,Citation18]) and PCV20 (PREVNAR 20™, Pfizer, New York, NY, USA; a 20-valent PCV containing the 13 STs included in the licensed PCV13 and seven additional STs [8, 10A, 11A, 12F, 15B, 22F, and 33F]), have recently been approved by the United States Food and Drug Administration for use in adults ≥18 years of age [Citation19,Citation20], and are currently in late-stage clinical development in infants and children. As the development timelines for these two vaccines overlap, no head-to-head comparisons are available. However, as both clinical study programs include studies that utilize PCV13 as the active control, it is possible to make indirect treatment comparisons using PCV13 as the common comparator. Indirect treatment comparison is a well-accepted framework in health technology assessment (HTA) to evaluate the comparative efficacy and safety of two interventions when head-to-head data are unavailable [Citation21]. Results on relative immunogenicity, safety, and tolerability could be useful for decision-makers to determine which vaccines to include in national immunization programs [Citation22].
In this article, data from four Phase III studies were used; three comparing V114 with PCV13 in healthy adults ≥50 years of age (analysis restricted to ≥60 years of age) and one comparing PCV20 with PCV13 in healthy adults ≥60 years of age (see ). PNEU-AGE (V114-019; NCT03950622) was the pivotal immunogenicity and safety study of V114 in healthy adults, in which V114 was demonstrated to be non-inferior to PCV13 for all 13 shared STs and superior to PCV13 for unique STs (22F and 33F) and shared ST3 [Citation23]. PNEU-PATH (V114-016; NCT03480763) evaluated the safety, tolerability, and immunogenicity of V114 followed 12 months later by vaccination with PPSV23. Results demonstrated comparable immune responses for all 15 STs in V114 for both V114 and PCV13 groups following PPSV23, and immune responses measured 30 days after receipt of either V114 or PCV13 were also found to be comparable for the 13 shared STs between the two vaccines [Citation24]. PNEU-TRUE (V114-020; NCT03950856) was a lot-to-lot consistency study of three lots of V114 demonstrating that V114 elicits an equivalent immune response across manufacturing lots [Citation25,Citation26]. In addition, the immunogenicity of the three combined lots of V114 was generally comparable to PCV13 for the 13 shared STs [Citation25]. All three studies had randomized, double-blind, active comparator-controlled designs, enrolled healthy adults ≥50 years of age, and had similar inclusion and exclusion criteria. At the time of our analysis, data from the Phase III pivotal clinical study (NCT03760146) evaluating the immunogenicity and safety of PCV20 in vaccine-naïve healthy adults aged ≥18 years compared with PCV13 were available [Citation27]. This study demonstrated non-inferiority for all STs shared with PCV13 in adults ≥60 years of age [Citation27].
Table 1. Characteristics of clinical studies included in the indirect comparison [Citation23–25,Citation32]
All four clinical studies had serotype-specific opsonophagocytic activity (OPA) geometric mean titers (GMTs) measured at approximately 1 month post-vaccination as the primary immunogenicity endpoint. OPA is considered a measure of functional activity of anti-pneumococcal antibodies, as protection against pneumococcal infection is primarily mediated by opsonin-dependent phagocytosis and may be the best in vitro correlate of protection [Citation28,Citation29]. Whether this translates to better protection in clinical practice is yet to be determined. Previous findings have been unable to demonstrate a correlation between immunoglobulin G (IgG) levels (as determined by enzyme-linked immunosorbent assays) and clinical protection [Citation30]. OPA assays carried out at BioA (Richmond, VA, USA) may have differed slightly across the three studies; however, previous studies have demonstrated concordance in OPA performance across assays from different laboratories [Citation31]. Therefore, indirect comparisons of OPA GMTs were feasible. Conversely, because data collection windows, types of adverse events assessed, and the proportion of adverse events reported in the comparator arm differed substantially between the PCV20 and V114 studies, comparability of safety and tolerability outcomes was limited.
By leveraging the individual participant data from three V114 studies and integrating published information regarding the immunogenic response from PCV20, the primary objective was to perform serotype-specific indirect treatment comparisons of the immunogenicity response following receipt of V114 and PCV20, using PCV13 as the common comparator. Serotype-specific comparisons were limited to 13 of the STs shared by PCV13, V114, and PCV20, as immunogenicity of PCV20 among other serotypes has only been evaluated in comparison with individuals who received both PCV13 and PPSV23.
2. Methods
2.1. Data sources
OPA GMT ratios for PCV20 for the 13 shared STs (STs 1, 3, 4, 5, 6A, 6B, 7F, 9V, 14, 18C, 19A, 19F, and 23F) were extracted from the study results published on clinicaltrials.gov for study NCT03760146 (results posted on 29 December 2020) [Citation32] by an internal statistician and were then independently validated by another. OPA GMT ratios were only available for Cohort 1 of the PCV20 study, which enrolled participants ≥60 years of age. In addition to OPA GMT ratios, baseline characteristics and number of participants in each age subgroup and vaccine arm were also extracted. Select V114 studies were pooled due to similarities in study design (Phase III, multicenter, randomized, double-blinded, and active comparator-controlled), study populations (healthy adults who are pneumococcal vaccine-naïve and ≥50 years of age), dose equivalence (single, sterile suspension in pre-filled syringe containing either 0.5 mL V114 or PCV13), and study evaluations (blood samples for immunogenicity assays were drawn at 30 days post-vaccination and evaluated using the microcolony multiplexed opsonophagocytic assay [MOPA] and pneumococcal electrochemiluminescence [PnECL] assay). OPA GMT data for V114 from the Phase III clinical studies previously described were taken from the V114 clinical database [Citation23–25].
2.2. Immunogenicity response
For the V114 studies, serum samples for OPA GMT analysis were drawn at 30 days post-vaccination (range 30–44 days) [Citation23–25], and at 30 days (range 28–42 days) post-vaccination for PCV20 [Citation32] (). The V114 studies used MOPA, whereas the PCV20 study used a single-plex OPA [Citation33]. Concordance in OPA performance from different laboratories has previously been demonstrated [Citation31,Citation34]. Therefore, the OPA GMT ratios of MOPA and single-plex OPAs were considered to be comparable. OPA titers were calculated using linear interpolation of the serum dilution killing 50% of bacteria in the assay [Citation33].
2.3. Analysis population
The populations included from V114 and PCV20 studies were considered comparable for the purpose of this analysis. Included in the analysis were three studies comparing V114 with PCV13 in healthy pneumococcal vaccine-naïve adults ≥50 years of age (analysis populations were restricted to ≥60 years of age; age ranges enrolled in each study are listed in ) and one comparing PCV20 with PCV13 in healthy pneumococcal vaccine-naïve adults ≥60 years of age (see ). OPA GMT data for V114 were taken from the per-protocol population and had similar blood sample collection times at approximately 30 days [Citation23–25]. For PCV20, the immunogenicity analysis population included participants who had at least one valid OPA titer for any of the 13 shared STs approximately 30 days after the first vaccination and no major protocol deviations [Citation32] ().
2.4. Estimation of OPA GMT ratio
The GMT ratios were estimated by calculating differences in geometric means from baseline and their 95% confidence intervals (CIs) on the natural log scale, then exponentiating the results to calculate GMT ratios. In the V114 studies, the constrained longitudinal data analysis (cLDA) method was used [Citation23–25], while, in the PCV20 study, GMT ratios were based on the t-distribution [Citation32]. As both methodologies produce the same relative measure of vaccination effect against PCV13, the two OPA GMT ratios were considered sufficient for comparison; under the assumption that any linear systematic differences between the GMT measurements would cancel out when the unitless GMT ratio is constructed.
2.5. Indirect treatment comparisons
The network of evidence for the indirect treatment comparisons is shown in . The comparisons were carried out using the Bucher method as an unadjusted comparison [Citation35,Citation36], and using the matching-adjusted indirect comparison (MAIC) method to adjust for the case-mix of participant baseline characteristics between V114 and PCV20 studies [Citation37], augmented by the Bucher method, as described below.
Figure 1. Indirect treatment comparison network.
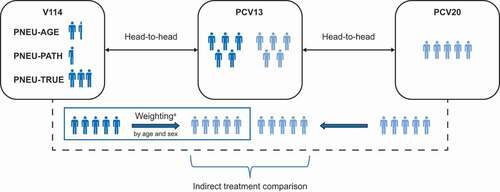
In the unadjusted comparisons using the Bucher method [Citation35,Citation36], the relative immunogenicity response (RIR) of OPA GMTs of V114 versus PCV20 anchored through PCV13 is,
where represents a specific ST and
represents the immunogenicity response of
for ST
in terms of change in 30 day OPA GMT. Assuming normal distribution on the log scale and due to PCV20 data availability, the OPA GMT ratios
obtained from the cLDA model (described in Section 2.4) and
(as reported on clinicaltrials.gov) were transformed to natural logarithm and then the differences in the log GMT ratios between V114 and PCV20 were calculated. The standard error for the difference in the log GMT ratios was calculated using the standard variance formula for independent additive normal distributions (square root of the sum of the variances) and 95% CIs of
were calculated using the following formula:
where is the top 2.5th quantile of the inverse cumulative standard normal distribution, and
is the standard error. One-sided p-values for non-inferiority of vaccination were based on the pre-specified margin and the Z-statistic.
Serotype-specific comparisons were limited to 13 of the STs shared by PCV13, V114, and PCV20. The two remaining shared STs between V114 and PCV20 (22F and 33F) have only been reported for PCV20 in comparison with individuals who received both PCV13 and PPSV23, rendering the use of PCV13 as the common comparator for 22F and 33F, infeasible. Therefore, these data were excluded from the analysis. Similarly, immune responses to additional STs in PCV20 (8, 10A, 11A, 12F, and 15B) but not present in V114 were excluded. For each of the shared STs, OPA GMT ratios between participants who received V114 and participants who received PCV13, and between participants who received PCV20 and participants who received PCV13, were compared. Non-inferiority margin was defined as a lower bound of the 95% CI of >0.50 (corresponding to a < 2.0-fold decrease in OPA GMT in the V114 group versus the PCV20 group). This statistical criterion is consistent with that used for previous pneumococcal vaccine studies [Citation38,Citation39]. Superiority analyses are not reported here; however, should this methodology be adapted to assess superiority, a margin of 1.2 would reflect a meaningful difference beyond a level that could be related to assay variability.
2.6. Matching-adjusted indirect comparisons
For the MAIC analysis, individuals () from V114 studies were reweighted with weight (
) from a logistic regression model
to match the mean age and sex proportion of the participants reported in the PCV20 study vaccinated population [Citation36,Citation37,Citation40]. The method of moments was used to estimate the beta values in the absence of individual-level data from the PCV20 study. Age and sex were identified as effect modifiers, as well as prognostic factors, based on previous studies and established impact on immune responses to vaccination [Citation38,Citation41,Citation42]. Presence of comorbidities was also considered as an effect modifier but was excluded from the MAIC analysis due to insufficient data published on comorbidities for the PCV20 study [Citation27,Citation32].
The serotype-specific OPA GMT ratios for the reweighted V114 versus reweighted PCV13 data were estimated using the cLDA method [Citation43]. The log-transformed OPA GMTs were regressed, by ST, against vaccination group (V114 and PCV13), time (baseline and day 30), interaction of time-by-vaccination group, age stratum (60–64, 65–74, and ≥75 years), and age stratum-by-time interaction as covariates. This allowed for different baseline means per stratum, while ensuring that the baseline mean within each age stratum was the same for each vaccination group. An unstructured covariance matrix was used to model the correlation among repeated measurements, and the Kenward–Roger adjustment was used with restricted maximum likelihood.
Using the weighted serotype-specific log OPA GMT ratios for V114 versus PCV13 from the cLDA model, the Bucher method [Citation35] was applied to estimate the serotype-specific OPA GMT ratios for V114 versus PCV20. The impact of weighting the V114 study data was assessed by computing the effective sample size as the square of the summed weights divided by the sum of the squared weights. The maximum effective sample size occurred when all participants had equal weight.
3. Results
3.1. Participants
A summary of the characteristics of the V114 studies and Cohort 1 of the PCV20 study is provided in . Participants were generally comparable between V114 and PCV20 studies by sex and age. The PCV20 study included 2,997 participants. In total, 3,039 participants from the per-protocol populations of the V114 studies were ≥60 years of age. After matching, the effective sample size for V114 was 1,710, indicating that the effect of weighting was equivalent to almost half the overall sample size. The mean age in the PCV20 and matching-adjusted V114 studies was 64.6 years, and 59.3% and 59.2%, respectively, were female ().
Table 2. Baseline characteristics for matched variables
3.2. Immunogenicity
Unadjusted and MAIC OPA GMT ratios for V114 versus PCV20 are shown in . Results were consistent across the two analyses. However, owing to the impact of weighting, the MAIC analysis would provide an alternative approach to estimating relative vaccine immunogenicity responses. The lower bound of the two-sided 95% CI was >0.50 for all 13 shared STs in the unadjusted and MAIC analyses, suggesting non-inferiority of V114 compared with PCV20 in adults ≥60 years of age for the 13 shared STs under investigation, ranging from 0.91 (95% CI: 0.76–1.10 and 0.77–1.07) for STs 4 and 14 to 1.93 (95% CI: 1.62–2.30) for ST6B. (, ). Under a non-inferiority hypothesis, the OPA GMT ratio for ST3 of V114 versus PCV20 was 1.77 (95% CI: 1.55–2.02). The lower bound of 1.55 is compared with the 0.50 non-inferiority margin, suggesting that V114 is non-inferior to PCV20, and, additionally, associated with a 77% greater adjusted mean OPA GMT. For seven STs in V114 (STs 3, 6A, 6B, 18C, 19A, 19F, and 23F), as compared with PCV20, the lower bound for the CI for the OPA GMT ratio was numerically greater than PCV20 (i.e. >1.0). There were no STs in which the upper bound of the CI was numerically lower than PCV20 (i.e. <1.0; ). Under a superiority hypothesis, the lower bound would be compared with the proposed 1.2 superiority margin and interpreted in a similar way. Five STs: 3, 6A, 6B, 18C, and 23F would meet this criterion for V114 compared with PCV20.
Figure 2. MAIC analysis of GMT ratio for V114 versus PCV20.
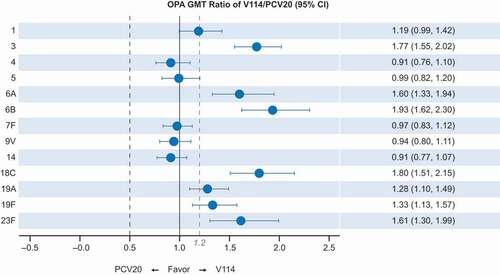
Table 3. Unadjusted and matching-adjusted indirect comparison (MAIC) analyses of OPA GMT ratio for V114 versus PCV20
4. Discussion
This paper presents a comparative analysis of two pneumococcal vaccines (V114 and PCV20) that provide important evidence to support HTAs and inform public health decision-making. The unadjusted and MAIC indirect treatment analyses demonstrated non-inferiority of V114 compared with PCV20 for all 13 STs in common with PCV13, as assessed in adults ≥60 years of age. Five STs (STs 3, 6A, 6B, 18C, and 23F) met a 1.2 superiority margin, although the clinical significance of these findings is yet to be demonstrated in a real-world setting. V114 was associated with a 77% greater adjusted mean OPA GMT for ST3 versus PCV20 with a 95% CI from 55% to 102% higher, which is consistent with numerically higher immune responses for ST3 observed in several V114 Phase III studies in adults [Citation23,Citation24]. In addition, V114 also elicited higher antibody titers than PCV20 for six additional shared STs, which are associated with high disease burden [Citation44]. These findings are consistent with previous studies showing lower immunogenicity for some shared STs in PCVs containing additional STs compared with PCVs with fewer STs [Citation45,Citation46]. We were unable to assess the comparative immune responses to 22F and 33F, which are STs covered by both V114 and PCV20, but not PCV13. Similarly, we were unable to assess immune responses to additional STs in PCV20 (8, 10A, 11A, 12F, and 15B) not present in V114 [Citation44].
The analysis was limited to immunogenicity outcomes and, as such, was neither designed to assess efficacy nor safety of either vaccine. Nevertheless, because antibody responses lie on the causal pathway leading to protection, immunogenicity represents an acceptable surrogate endpoint for protection by pneumococcal vaccines, as a definitive correlate of protection has yet to be determined [Citation30]. In addition, the clinical significance of the immunogenicity data and numerical differences reported here and how they relate to potential differences in protection are yet to be determined in clinical practice. These results may serve as an intermediate bridging analysis to support further analyses, such as health economic assessments or estimates of impact on invasive PD based on immunogenicity responses [Citation47]. Direct head-to-head comparisons of efficacy would be needed to confirm results from this analysis. The latter may be of particular value in the short term, as true effectiveness data for these vaccines will not be available for several years and efficacy studies are not planned.
There are several limitations to our analyses. No comparative analyses on safety could be performed. Owing to disparities between the PCV20 and V114 studies in the types of adverse events being collected, differing time windows, and the subjectivity of safety reporting, comparison of safety outcomes would be biased and misleading. However, the safety and tolerability profiles of both vaccines were similar to thaose of PCV13 in clinical studies, and no safety concerns were identified [Citation23–25,Citation27].
Although different OPA measurements were used in the two studies, previous studies have demonstrated that OPA assays performed in different laboratories with long-standing experience in this assay yielded acceptable agreement in key assay characteristics, such as precision, accuracy, and concordant results among laboratories [Citation31,Citation34]. Although there were some variations in numerical GMTs within the three V114 studies, overall, the findings were generally consistent across the studies. In addition, the method presented here used the ratio from each individual assay, which would, in effect, cancel out any assay-specific multiplicative differences. The ratio is also dependent on the performance of the comparator vaccine and OPA GMT ratio does not effectively reflect the overall vaccine performance. If the difference was not linear (for example, if the relationship between the assay recordings were measurement-dependent), bias related to comparability of assay measurements could have been introduced. In addition, some OPA GMT measurements in the V114 studies were below the lower limit of quantification or above the upper limit of quantification. This limitation in assay sensitivity would likely also be present for PCV20; however, these data were not available in the public domain and, as such, could not be considered in our analyses. Race or geography may affect immune responses to vaccines; studies from Asia, Africa, and Latin America have shown greater antibody responses to PCVs compared with studies from Europe and North America [Citation48]. The majority of the V114 study sites were in the United States. V114-016 had 15 US and four Asia sites out of 22 and ~60% of participants were White, V114-019 had 14 US and seven Asia sites out of 30, and ~70% of participants were White, and V114-020 had 30 US and no Asia sites out of 55, and ~90% of participants were White. The PCV20 study was conducted in the United States and Sweden and Cohort 1 used in our analysis had an ~86% White population.
Subgroup analyses for different baseline factors could not be performed because only overall population data from the PCV20 study were available. In addition, as the PCV20 study only assessed immunogenicity in adults ≥60 years of age, the vaccines could not be compared in adults 50–59 years of age, despite the inclusion of this age group in the V114 studies. As both vaccines are now licensed for use in adults ≥18 years of age in the United States, comparative data in younger adults would add to a broader comparison. Furthermore, the effect of weighting led to a large reduction in effective sample size compared with the per-protocol population, which contributed to an increase in the variance estimates. Despite some differences in GMT measurements across the V114 studies and the PCV20 study that could not fully be eliminated, the overall conclusions remained consistent across unadjusted and MAIC analyses. Additional baseline participant characteristics, such as presence of comorbidities, would have provided more accurate matching for the MAIC analysis, but these data were not published for the PCV20 study. Therefore, our analyses assumed that comorbidities in the participant populations in the V114 and PCV20 studies were similar, which could not be validated with the present data available to us. The MAIC results representing population-averaged effects are relevant to decision-making for national immunization programs in the absence of head-to-head studies. However, only the immune response to PCV13 STs can be quantified using this approach. In combination with the prevalence of STs in different geographic regions, additional analyses can inform and potentially affect specific decision-making processes.
The MAIC method assumes that there are no unknown confounders or treatment effect modifiers and prognostic factors not included in the proposed analyses. This assumption is not testable. Furthermore, multiplicity control was not applied in these analyses. However, as comparisons are made individually for each of the 13 shared STs, this approach controls the one-sided type-I error rate at 0.025.
5. Conclusions
MAIC represents an important tool to HTA agencies and other decision-makers in the absence of head-to-head studies that could facilitate early comparative assessments of competing vaccines. The indirect treatment comparisons presented here provide the most current evidence on the immunogenicity of V114 compared with PCV20. The immunogenicity results for the 13 shared PCV13 STs in the unadjusted and MAIC analyses support non-inferiority of V114 compared with PCV20 in adults ≥60 years of age. Under a superiority hypothesis, V114 was associated with a greater immune response for five STs (shared by all three vaccines, PCV13, V114, and PCV20) versus PCV20. Maintenance of protection against PD caused by PCV STs remains important, due to the high prevalence of these STs. Overall, these analyses suggest that V114 may offer better protection against the 13 shared STs when compared with PCV20 in adults ≥60 years of age.
Author contributions
L Abderhalden: analysis and interpretation of data, preparation of the manuscript; review of the manuscript. S Mt-Isa, L Musey, T Weiss: Indirect treatment comparison study conception, authoring of the study protocol and analysis and interpretation of data, preparation of the manuscript; review of the manuscript.
Acknowledgments
The authors would like to thank Yirong Cao for contribution towards designing and writing of the statistical programs for these analyses. Medical writing support, including assisting authors with the development of the initial draft and incorporation of comments, was provided by Rachel Wright, PhD, of Scion, London, and Salini Mohanty, DrPH, MPH, of Merck Sharp & Dohme Corp., a subsidiary of Merck & Co., Inc., Kenilworth, NJ, USA, and editorial support was provided by Ian Norton, PhD, of Scion, London, according to Good Publication Practice guidelines.
Disclosure statement
S Mt-Isa, L Abderhalden, L Musey, and T Weiss are employees of Merck Sharp & Dohme Corp., a subsidiary of Merck & Co., Inc., Kenilworth, NJ, USA and may own stock and/or stock options in Merck & Co., Inc., Kenilworth, NJ, USA. The authors have no other relevant affiliations or financial involvement with any organization or entity with a financial interest in or financial conflict with the subject matter or materials discussed in the manuscript apart from those disclosed.
Additional information
Funding
References
- European Centre for Disease Prevention and Control. Invasive pneumococcal disease. Annual epidemiological report for 2018. 2018 [cited 2020 Sept 14]. Available from: https://www.ecdc.europa.eu/sites/default/files/documents/AER_for_2018_IPD.pdf
- Centers for Disease Control and Prevention. Surveillance and reporting 2017 [2019 Apr 3]. Available from: https://www.cdc.gov/pneumococcal/surveillance.html
- Drijkoningen JJ, Rohde GG. Pneumococcal infection in adults: burden of disease. Clin Microbiol Infect. 2014;20(Suppl 5):45–51.
- Suaya JA, Gessner BD, Fung S, et al. Acute otitis media, antimicrobial prescriptions, and medical expenses among children in the United States during 2011–2016. Vaccine 2018;36(49):7479–7486.
- Wahl B, O’Brien KL, Greenbaum A, et al. Burden of Streptococcus pneumoniae and Haemophilus influenzae type b disease in children in the era of conjugate vaccines: global, regional, and national estimates for 2000–15. The Lancet Global Health. 2018;6(7):e744–e757.
- Myint TT, Madhava H, Balmer P, et al. The impact of 7-valent pneumococcal conjugate vaccine on invasive pneumococcal disease: a literature review. Adv Ther. 2013;30(2):127–151.
- Balsells E, Guillot L, Nair H, et al. Serotype distribution of Streptococcus pneumoniae causing invasive disease in children in the post-PCV era: a systematic review and meta-analysis. PLoS One. 2017;12(5):e0177113.
- Pichichero ME, Casey JR. Emergence of a multiresistant serotype 19A pneumococcal strain not included in the 7-valent conjugate vaccine as an otopathogen in children. JAMA 2007;298(15):1772–1778.
- Feikin DR, Kagucia EW, Loo JD, et al. Serotype-specific changes in invasive pneumococcal disease after pneumococcal conjugate vaccine introduction: a pooled analysis of multiple surveillance sites. PLOS Med. 2013;10(9):e1001517.
- Matanock A, Lee G, Gierke R, et al. Use of 13-valent pneumococcal conjugate vaccine and 23-valent pneumococcal polysaccharide vaccine among adults aged ≥65 years: updated recommendations of the advisory committee on immunization practices. MMWR Morb Mortal Wkly Rep. 2019;68(46):1069–1075.
- Pichichero M, Kaur R, Scott DA, et al. Effectiveness of 13-valent pneumococcal conjugate vaccination for protection against acute otitis media caused by Streptococcus pneumoniae in healthy young children: a prospective observational study. Lancet Child Adolesc Health. 2018;2(8):561–568.
- Savulescu C, Krizova P, Lepoutre A, et al. Effect of high-valency pneumococcal conjugate vaccines on invasive pneumococcal disease in children in SpIDnet countries: an observational multicentre study. Lancet Respir Med. 2017;5(8):648–656.
- Bonnave C, Mertens D, Peetermans W, et al. Adult vaccination for pneumococcal disease: a comparison of the national guidelines in Europe. Eur J Clin Microbiol Infect Dis. 2019;38(4):785–791.
- Varghese J, Chochua S, Tran T, et al. Multistate population and whole genome sequence-based strain surveillance of invasive pneumococci recovered in the USA during 2017. Clin Microbiol Infect. 2020;26(4):e511–512.
- Ladhani SN, Collins S, Djennad A, et al. Rapid increase in non-vaccine serotypes causing invasive pneumococcal disease in England and Wales, 2000–17: a prospective national observational cohort study. Lancet Infect Dis. 2018;18(4):441–451.
- Pick H, Daniel P, Rodrigo C, et al. Pneumococcal serotype trends, surveillance and risk factors in UK adult pneumonia, 2013–18. Thorax. 2020;75(1):38–49.
- Klugman KP, Rodgers GL. Time for a third-generation pneumococcal conjugate vaccine. Lancet Infect Dis. 2020;21(1):14–16.
- Moore MR, Link-Gelles R, Schaffner W, et al. Effect of use of 13-valent pneumococcal conjugate vaccine in children on invasive pneumococcal disease in children and adults in the USA: analysis of multisite, population-based surveillance. Lancet Infect Dis. 2015;15(3):301–309.
- Food and Drug Administration. VAXNEUVANCE™ (pneumococcal 15-valent conjugate vaccine) prescribing information 2021. 2021 [cited 2021 Jul 21]. Available from: https://www.fda.gov/media/150819/download
- Food and Drug Administration. PREVNAR 20 (pneumococcal 20-valent conjugate vaccine) prescribing information 2021. 2021 [cited 2021 Jul 26]. Available from: https://www.fda.gov/media/149987/download
- Es-Skali IJ, and Spoors J. Analysis of indirect treatment comparisons in national health technology assessments and requirements for industry submissions. J Comp Eff Res. 2018;7(4):397–409.• Reference of interest as it highlights relevance and value of indirect treatment comparisons when head-to-head data are not available.
- Hausdorff WP, Hoet B, Adegbola RA. Predicting the impact of new pneumococcal conjugate vaccines: serotype composition is not enough. Expert Rev Vaccines. 2015;14(3):413–428.
- Platt HL, Cardona JF, and Haranaka M, et al. A phase 3 trial of safety, tolerability, and immunogenicity of V114, 15-valent pneumococcal conjugate vaccine, compared with 13-valent pneumococcal conjugate vaccine in adults 50 years of age and older (PNEU-AGE). Vaccine. 2021;In press doi:https://doi.org/10.1016/j.vaccine.2021.08.049. Sep 7;S0264-410X(21)01097-5. • Reference of interest as it describes results for a study included in the indirect comparison.
- Song J-Y, Chang C-J, and Andrews C, et al. Safety, tolerability, and immunogenicity of V114, a 15-valent pneumococcal conjugate vaccine, followed by sequential PPSV23 vaccination in healthy adults aged ≥50 years: a randomized phase III trial (PNEU-PATH). Vaccine. 2021;39(43) 6422–6436 .
- Merck Sharp & Dohme Corp., a subsidiary of Merck & Co., Inc., Kenilworth, NJ, USA. Lot-to-lot consistency of V114 in healthy adults (V114-020) (PNEU-TRUE) 2021.2021 [cited 2021 Jul 26]. Available from: https://clinicaltrials.gov/ct2/show/NCT03950856.• Reference of interest as it describes results for a study included in the indirect comparison
- Simon JK. A phase III, randomised, double-blind, active comparator-controlled, lot-to-lot consistency study to evaluate the safety and immunogenicity of V114 in healthy adults ≥50 years of age (PNEU-TRUE). Presented at: 31st European Congress of Clinical Microbiology and Infectious Diseases (ECCMID); 2021 July 9–12.• Reference of interest as it describes results for a study included in the indirect comparison.
- Essink B, Sabharwal C, and Xu X, et al. Phase 3 pivotal evaluation of 20-valent pneumococcal conjugate vaccine (PCV20) safety, tolerability, and immunologic noninferiority in participants 18 years and older. Presented at: IDWeek (Infectious Diseases Society of America); 2020 October 21–25.• Reference of interest as it describes results for a study included in the indirect comparison.
- Vakevainen M, Jansen W, Saeland E, et al. Are the opsonophagocytic activities of antibodies in infant sera measured by different pneumococcal phagocytosis assays comparable? Clin Diagn Lab Immunol. 2001;8(2):363–369.
- Song JY, Moseley MA, Burton RL, et al. Pneumococcal vaccine and opsonic pneumococcal antibody. J Infect Chemother. 2013;19(3):412–425.
- World Health Organization (WHO). Correlates of vaccine-induced protection: methods and implications 2013 [2021 Aug 25]. Available from: https://apps.who.int/iris/bitstream/handle/10665/84288/WHO_IVB_13.01_eng.pdf
- Rose CE, Romero-Steiner S, Burton RL, et al. Multilaboratory comparison of streptococcus pneumoniae opsonophagocytic killing assays and their level of agreement for the determination of functional antibody activity in human reference sera. Clin Vaccine Immunol. 2011;18(1):135–142.
- Clinicaltrials.gov. Trial to evaluate the safety and immunogenicity of a 20-valent pneumococcal conjugate vaccine in pneumococcal vaccine-naïve adults 2020 [cited 2021 Jul 26]. Available from: https://www.clinicaltrials.gov/ct2/show/NCT03760146.
- Nolan KM, Bonhomme ME, Schier CJ, et al. Optimization and validation of a microcolony multiplexed opsonophagocytic killing assay for 15 pneumococcal serotypes. Bioanalysis 2020;12(14):1003–1020.
- Burton RL, Antonello J, Cooper D, et al. Assignment of opsonic values to pneumococcal reference serum 007sp for use in opsonophagocytic assays for 13 serotypes. Clin vaccin immunol. 2017;24(2). DOI:https://doi.org/10.1128/CVI.00457-16.
- Bucher HC, Guyatt GH, Griffith LE, et al. The results of direct and indirect treatment comparisons in meta-analysis of randomized controlled trials. J Clin Epidemiol. 1997;50(6):683–691.
- Signorovitch JE, Sikirica V, Erder MH, et al. Matching-adjusted indirect comparisons: a new tool for timely comparative effectiveness research. Value Health. 2012;15(6):940–947.
- Phillippo DM, Ades AE, Dias S, et al. Methods for population-adjusted indirect comparisons in health technology appraisal. Med Decis Making. 2018;38(2):200–211.
- Jackson LA, Gurtman A, van Cleeff M, et al. Immunogenicity and safety of a 13-valent pneumococcal conjugate vaccine compared to a 23-valent pneumococcal polysaccharide vaccine in pneumococcal vaccine-naive adults. Vaccine 2013;31(35):3577–3584.
- Greenberg RN, Gurtman A, Frenck RW, et al. Sequential administration of 13-valent pneumococcal conjugate vaccine and 23-valent pneumococcal polysaccharide vaccine in pneumococcal vaccine-naive adults 60-64 years of age. Vaccine. 2014;32(20):2364–2374.
- Signorovitch JE, Wu EQ, Yu AP, et al. Comparative effectiveness without head-to-head trials: a method for matching-adjusted indirect comparisons applied to psoriasis treatment with Adalimumab or etanercept. Pharmacoeconomics 2010;28(10):935–945.
- Bryant KA, Frenck R, Gurtman A, et al. Immunogenicity and safety of a 13-valent pneumococcal conjugate vaccine in adults 18–49 years of age, naive to 23-valent pneumococcal polysaccharide vaccine. Vaccine 2015;33(43):5854–5860.
- Fink AL, Klein SL. Sex and gender impact immune responses to vaccines among the elderly. Physiology (Bethesda). 2015;30:408–416.
- Liang K-Y, Zeger SL. Longitudinal data analysis of continuous and discrete responses for pre-post designs. Sankhyā Indian J Stat. 2000;62:134–148.
- Cui YA, Patel H, O’Neil WM, et al. Pneumococcal serotype distribution: a snapshot of recent data in pediatric and adult populations around the world. Hum Vaccin Immunother. 2017;13(6):1–13.
- Grant LR, O’Brien SE, Burbidge P, et al. Comparative immunogenicity of 7 and 13-valent pneumococcal conjugate vaccines and the development of functional antibodies to cross-reactive serotypes. PLoS One. 2013;8(9):e74906.
- Dagan R, Patterson S, Juergens C, et al. Comparative immunogenicity and efficacy of 13-valent and 7-valent pneumococcal conjugate vaccines in reducing nasopharyngeal colonization; a randomized double-blind trial. Clin Infect Dis. 2013;57(7):952–962.
- Stoecker C. Economic assessment of PCV15 & PCV202021. 2021 Jul 27. Available from: https://www.cdc.gov/vaccines/acip/meetings/downloads/slides-2021-06/03-Pneumococcal-Stoecker-508.pdf
- Park DE, Johnson TS, Nonyane BA, et al. The differential impact of coadministered vaccines, geographic region, vaccine product and other covariates on pneumococcal conjugate vaccine immunogenicity. Pediatr Infect Dis J. 2014;33(Suppl 2):S130–139.