ABSTRACT
Introduction
The pneumococcal non-typeable Haemophilus influenzae protein D-conjugate vaccine (PHiD-CV/PCV10) and 13-valent pneumococcal conjugate vaccine (PCV13) protect against vaccine-serotype invasive pneumococcal disease (VT IPD). However, VT IPD can still occur in fully or partially vaccinated children (vaccine failure or breakthrough). We performed a systematic review of vaccine failures and breakthrough IPD with PCV10 and PCV13 in ≤5-year-olds.
Areas covered
We searched Scopus/Medline/EMBASE to retrieve articles/abstracts published between 1/2008-7/2019. We excluded reports only including data from ≥6-year-olds, exclusively assessing PCV7-vaccinated children or children with comorbidities. Twenty-six reports (20 PCV13, 1 PCV10, 5 both), covering studies with various designs in six continents, using different schedules, were included. Collectively, they reported 469 VT IPD cases classified as vaccine failures and 403 as breakthrough. Vaccine failure and breakthrough rates were low: 8.4% and 9.3%, respectively, of all IPD in vaccinated children, consistent with the vaccines’ high effectiveness. The main serotypes associated with vaccine failure/breakthrough were 19A, 3 and 19F for PCV13 and 14, 6B and vaccine-related 19A and 6A for PCV10.
Expert opinion
As we move to vaccines with more serotypes, it is not only important to consider which serotypes are added, but also monitor and address incomplete protection against specific serotypes.
PLAIN LANGUAGE SUMMARY
What is the context?
Pneumococcal conjugate vaccines have been given to children for over 20 years to prevent infections caused by the bacterium Streptococcus pneumoniae (such as pneumonia, meningitis and sepsis).
At least 100 different types of S. pneumoniae, so called serotypes, exist, but a relatively small number causes most disease.
Two current vaccines (Synflorix, GSK and Prevnar 13, Pfizer) protect against 10 to 13 serotypes and have significantly reduced pneumococcal disease caused by these serotypes.
A rise in serotypes not targeted by these vaccines has lessened the vaccines’ expected impact.
As no vaccine is 100% protective, some serotypes targeted by the current vaccines continue to circulate.
What is new?
We performed a systematic literature review to evaluate which serotypes are most often associated with invasive disease occurring after receives all planned pneumococcal vaccine doses (vaccine failure) or after a child receives part of the planned vaccine doses (breakthrough).
We found that vaccine failures and breakthrough disease were uncommon with both vaccines, irrespective of the administered schedule.
A small number of serotypes were responsible for most vaccine failures and breakthrough disease with both vaccines.
What is the impact?
The low rate of vaccine failures and breakthrough disease observed with the current vaccines confirms their high effectiveness in preventing pneumococcal disease.
The primary consideration in developing pneumococcal conjugate vaccines that include more than 13 serotypes will be how additional protection they can provide.
Reduced protection against individual serotypes remains a risk.
The evaluation of current vaccines demonstrates that incomplete protection against specific serotypes should also be addressed.
1. Introduction
It has been over 10 years since higher-valency pneumococcal conjugate vaccines (PCVs) – the pneumococcal non-typeable Haemophilus influenzae protein D-conjugate vaccine (PHiD-CV or PCV10, Synflorix, GSK) and the 13-valent PCV (PCV13, Prevnar 13/Prevenar 13, Pfizer Inc.) – were first introduced [Citation1]. The two vaccines are formulated differently, produced by employing distinct protein carriers and conjugation chemistries, with capsular polysaccharides (CPS) of 10 and 13 of the ~100 circulating serotypes included in their formulations, respectively [Citation1,Citation2Citation3,Citation4]. PCV10 contains CPS of serotypes 1, 4, 5, 6B, 7F, 9V, 14, 18C, 19F and 23F; PCV13 contains CPS of serotypes 3, 6A and 19A in addition to the 10 PCV10 types [Citation1,Citation2]. PCV10 has also demonstrated cross-protection against serotype 19A mediated by cross-reactive 19F antibodies [Citation5–10].
The impact of both of these vaccines on a broad range of pneumococcal diseases in young children has been substantial and has been reported extensively [Citation2,Citation11–15]. Invasive pneumococcal disease (IPD) is the most frequently used measure of PCV impact, and despite the lack of head-to-head comparisons, both PCVs have demonstrated a similar impact on overall IPD (i.e. caused by any pneumococcal serotype) in the pediatric population [Citation1,Citation2,Citation11,Citation13,Citation16]. Importantly, PCV10 and PCV13 have demonstrated strong reductions in vaccine-serotype (VT) IPD [Citation2,Citation11]. Nevertheless, some residual VT disease remains in countries, irrespective of which PCV has been implemented in national infant immunization programs [Citation17,Citation18].
A number of studies have explored the occurrence of VT IPD in children immunized with PCVs [Citation19]. Oligbu and colleagues previously reported the results of a systematic review of the literature published between January 2000 and April 2016 on PCV vaccine failures (i.e. VT IPD after a complete vaccination schedule) in children ≤5 years old [Citation19]. They concluded that 19F and 6B were the major serotypes associated with PCV vaccine failure. While providing an extensive review of the published data, Oligbu et al. mostly covered vaccine failures in children immunized with the 7-valent PCV (PCV7, Prevnar/Prevenar, Pfizer Inc., which contains CPS of serotypes 4, 6B, 9V, 14, 18C, 19F and 23F), with two out of twenty included citations reporting vaccine failures for PCV10 and four for PCV13 [Citation19]. Consequently, the review provides limited information on vaccine failures associated with serotypes included in higher-valency PCVs. To address this gap in knowledge, we conducted a systematic review of PCV vaccine failures for VT IPD in children 5 years of age and younger, focused on PCV10 and PCV13, with studies of PCV7 being specifically excluded from the analysis. Additionally, while the systematic review by Oligbu et al. assessed vaccine failures (in fully vaccinated children), the current review also evaluated PCV breakthrough cases in partially vaccinated children to determine the effect of the number of primary doses on protection against VT IPD.
A plain language summary is provided in .
2. Methods
2.1. Search strategy and eligibility criteria
Our review is registered on the international prospective register of systematic reviews (PROSPERO, registration number: CRD42019139248), where the protocol can be accessed. We followed the Preferred Reporting Items for Systematic reviews and Meta-Analyses (PRISMA) statement [Citation20]. Our review includes published journal articles and conference abstracts describing vaccine failures and breakthrough cases of VT IPD in children ≤5 years of age following complete or partial vaccination with PCV10, PCV13 or both since their licensure. We searched Scopus, Medline (via PubMed) and EMBASE databases using the search string ‘(pneumococcal AND vaccine AND failure) AND (“2008/01/01”[Date – Publication]: “3000”[Date – Publication])’ to retrieve articles and abstracts published in English from 1 January 2008. The last search was performed on 19 July 2019. We excluded reports from animal studies, studies confined to adults or only including data from children ≥6 years of age, studies exclusively assessing PCV7-vaccinated children and studies on immunocompromised children or children with comorbidities that increase the risk of IPD. We did not impose any other restrictions on the type of study included in the review for the initial analysis, but we performed a sub-analysis restricted to case-control studies. If two or more publications were based on the same or overlapping datasets, we only kept the most recent one to avoid duplication of cases.
Our final search strategy differed slightly from the one outlined in the protocol. To expand sample size, we included children aged 5 years in addition to those younger than 5 as initially planned, and we also included a small number of vaccine failures and breakthrough cases in older children from studies where the vast majority of the dataset reported cases aged 5 years or under. We included a small number of articles published in languages other than English if the abstract was available in English and contained most of the necessary information.
2.2. Record selection and data collection
After removal of duplicates, identified search results were independently, manually reviewed for eligibility by three contributors (BAM, JNG and NL) based on title and abstract. Those deemed likely to contain relevant data were independently reviewed by the three contributors as full-text articles. At this step, if a screened article referred to another article with potentially relevant data, this was also screened for eligibility. Following selection of final articles, the three contributors met to compare results and resolve any discrepancies in article selection. In the event of no agreement, the primary author (BAM) made the final selection.
The primary author did the final data extraction from each publication, which included: publication details, country of origin of cases, vaccination schedule, total number of IPD cases in PCV10/PCV13-vaccinated children (or an approximation thereof), number of cases of VT IPD in fully vaccinated children (i.e. vaccine failures), number of cases of VT IPD in partially vaccinated children (i.e. breakthrough cases), vaccine and number of doses received for each case, serotype details for each case, study design, study period, surveillance type and population details for the surveillance system (if available). All extracted data were verified by an independent reviewer and discrepancies were discussed and corrected where needed.
2.3. Risk of bias assessment
As the majority of studies included in this review were non-randomized, observational studies, including cohort studies and case-control studies, the risk of bias in the studies was assessed using a modified version of the ROBINS-I (Risk Of Bias In Non-randomized Studies – of Interventions) tool [Citation21]. We selected the three most likely and most relevant confounding domains appropriate for this review for risk of bias assessment: bias in selection of participants into the study, bias in classification of interventions and bias due to missing data. These criteria were scored independently by two contributors (JNG and NL) and results pooled and discussed for agreement. In the event of no agreement, the primary author (BAM) decided on the final score. The overall risk of bias judgment was summarized across these three domains and reflected the highest level of bias determined for any individual domain.
2.4. Data analysis
Data were collated and analyzed to determine the number and proportion of vaccine failures and breakthrough VT IPD cases, in fully or partially vaccinated children ≤5 years, respectively, according to the number of vaccine doses they received. Cases in immunocompromised children or in children with comorbidities that increase the risk of IPD were excluded from the analyses where possible, as these provide a substantial source of variability in terms of susceptibility to infection.
Vaccine failures were classified as VT IPD cases in fully vaccinated children according to the country’s schedule in which they were reported, either through primary/booster infant schedules (2+1, 3+0, 3+1) or through catch-up schedules (1, 2 or 3 doses according to age). Vaccine breakthrough cases were all VT IPD cases that received ≥1 dose of PCV, minus vaccine failure cases reported in the same study. For some studies, vaccine failure could not be distinguished from breakthrough (for instance, if cases vaccinated with ≥1 dose were reported without further details on the exact number of doses). These cases were not included in either category but were counted in the total of VT IPD cases having received ≥1 PCV dose.
For PCV10 failure or breakthrough, VT IPD was defined as IPD caused by any of the 10 serotypes included in PCV10 and serotypes 6A and 19A when available (6A and 19A were included in some studies because of cross-reactivity with 6B and 19F antibodies [Citation10]). VT IPD for PCV13 failure or breakthrough was defined as IPD caused by any of the 13 serotypes included in PCV13 and 6C when available (6C was included in some studies because of cross-reactivity with 6A antibodies [Citation22]). When no information was available on the specific VT in the vaccine failure or breakthrough case, cases were only included in the assessment of the total number of vaccine failures or breakthrough cases.
We noted that different studies stratified cases using different criteria, and some studies only reported VT IPD cases grouped as receipt of ≥1 dose of PCV. While this grouping contains children who only received 1 dose, it is mixed with children receiving 2 or more doses. Similar caveats apply to studies reporting IPD cases in children receiving ≥2 doses. For clarity, we present the number/proportion of cases for each VT, reported by the specific number of doses received or by grouped doses (≥1 or ≥2), as available in the different studies or derived from the available information. We also present vaccine failures for each serotype by schedule received (2+1, 3+0 or 3+1).
Vaccine failure and breakthrough IPD rates (across studies or subsets of studies, e.g. for different vaccination schedules) were calculated by dividing the number of vaccine failures or breakthrough cases by the total number of IPD cases in PCV-vaccinated children for studies where the latter was available or could be derived or approximated from the available information.
We initially set out to compare vaccine failures and breakthrough disease with PCV10 and PCV13. However, to avoid bias due to the large imbalance in the number of publications retrieved for the two PCVs, we analyzed data for each PCV separately in addition to combining all cases for both vaccines, but we did not perform a formal comparison between PCVs.
All analyses were descriptive. No formal statistical testing was performed because of the differences between the type and extent of information available from the different studies. No assessment of certainty in the body of evidence was performed.
A sub-analysis restricted to case-control studies was conducted to provide a more robust dataset where the vaccination status was known for the entire cohorts.
3. Results
3.1. Study selection and characteristics
Our literature search initially identified 1,121 unique publications (). After screening for eligibility based on titles and abstracts, 104 publications were selected for full-text review, with the remaining excluded for not meeting one or more eligibility criteria or including non-relevant data. An additional four articles were identified among references cited in the screened publications and were included in the full-text review. A total of 26 publications provided relevant data [Citation6,Citation9,Citation23–46]. Reasons for exclusion are summarized in . One of the remaining abstracts was replaced by the full-length publication at the time of data extraction as this had meanwhile become available [Citation40,Citation47]. This resulted in a total of 25 peer-reviewed articles and 1 conference abstract being included in the final analysis () [Citation6,Citation9,Citation23–39,Citation41–47].
Figure 2. Selection of publications in the systematic review. AOM, acute otitis media; IPD, invasive pneumococcal disease; n, number of records; PCV(9/13), (9/13-valent) pneumococcal conjugate vaccine; PCV10, pneumococcal non-typeable Haemophilus influenzae protein D-conjugate vaccine.
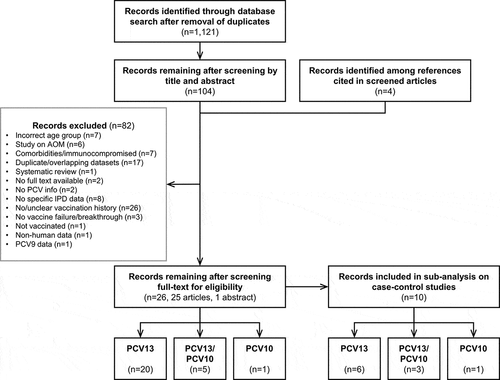
summarizes the main characteristics of the studies described in these 26 publications. Additional information is provided in Supplementary Table 1. Sixteen publications covered studies in different countries in Europe, three in Africa, three in North America, two in Australia, one in Asia and one in South America. Schedules used in these countries included 2 primary doses with a booster (n = 13) and 3 primary doses with (n = 10) or without (n = 3) booster. The assessment periods of the different studies varied, starting as early as 2009 and up to maximum 2018 (). Twenty publications reported on vaccine failures or breakthrough disease after PCV13 vaccination, one after PCV10 vaccination and five included data on both PCV13 and PCV10 (including mixed schedules) (). Of note, in a small number of studies, vaccine failures or breakthrough cases were reported after receipt of one or more doses of PCV7 and one or more doses of the higher-valency PCVs.
Table 1. Characteristics of included studies
Ten articles were included in our sub-analysis restricted to case-control studies, of which six evaluated PCV13, one PCV10 and three both vaccines ( and ).
3.2. Vaccine failure and breakthrough, overall analysis
Collectively, the 26 included studies reported 1,058 VT IPD cases in children who had received ≥1 dose of PCV13 and/or PCV10. Of these, 469 could be classified as vaccine failures (i.e. were fully vaccinated according to the country’s schedule) and 403 as breakthrough IPD cases (i.e. did not receive the country’s complete schedule) (). As the majority of studies took place in settings where PCV13 was used, the overall analysis presented in this section mostly reflects vaccine failures and breakthrough cases after PCV13. See section 3.4 for a limited vaccine-specific analysis.
Table 2. Breakthrough IPD cases and vaccine failures identified in the included studies
Thirteen studies enabled the calculation of the overall vaccine failure rate. Across these studies, there were 429 vaccine failures out of a total of 5,114 IPD cases in vaccinated children, resulting in a failure rate of 8.4%. Ten studies enabled the calculation of the overall breakthrough IPD rate, which was 9.3% (361/3,888 across studies). Rates varied substantially according to study and vaccination schedule. Average vaccine failure rates were 19.0% (267/1,403) across two studies assessing a 3+0 schedule (both in Australia [Citation28,Citation36]), 4.2% (130/3,097) across six studies assessing a 2+1 schedule (in France [Citation23], United Kingdom [Citation25], Switzerland [Citation27], South Africa [Citation29], Canada [Citation6] and Morocco [Citation31]) and 5.2% (32/614) across five studies evaluating a 3+1 schedule (in Portugal [Citation24], Spain [Citation32,Citation34,Citation37] and Germany [Citation46]). Differences in study settings, populations and follow-up periods may have, however, influenced these results.
Of the 469 VT IPD cases that could be classified as vaccine failures after PCV13 or PCV10, 463 had serotype information available. Among these cases, serotypes 3 and 19A were most frequently reported as vaccine failures (185/463, 40.0% and 166/463, 35.9%, respectively) followed by serotype 19F (66/463, 14.3%) (). All 403 VT IPD cases that could be classified as breakthrough disease after ≥1 dose of PCV13 or PCV10 had serotype information available. Among these breakthrough cases, the most commonly reported serotype was 19A (180/403, 44.7%), followed by serotype 3 (80/403, 19.9%) and 19F (34/403, 8.4%) (). Similar proportions were observed for the 206 breakthrough cases reported after ≥2 vaccine doses ().
Figure 3. Vaccine failures and breakthrough IPD cases after PCV13 or PCV10, reported for each serotype in the included studies, shown as proportions of the total vaccine failures or breakthrough cases across serotypes. Panel a shows vaccine failures, and breakthrough cases after receipt of ≥1 dose or ≥2 doses of PCV13 or PCV10. Panel b shows breakthrough cases after receipt of exactly 1, 2 or 3 doses of PCV13 or PCV10. IPD, invasive pneumococcal disease; N, total number of reported vaccine failures or breakthrough cases after the indicated number of doses, including only cases with serotype information available; PCV13, 13-valent pneumococcal conjugate vaccine; PCV10, pneumococcal non-typeable Haemophilus influenzae protein D-conjugate vaccine.
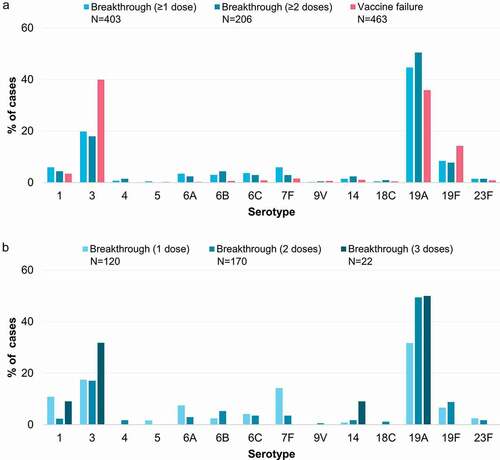
To determine the effect of each subsequent dose, we used the available data stratified by the number of doses received (which was not available for all studies). This revealed the same overall pattern after 1 or 2 doses, with 19A accounting for the highest proportion of breakthrough cases, followed by serotype 3, and all other VT IPD breakthrough cases occurring less frequently (). Very few cases were reported as vaccine breakthrough after 3 doses (n = 22, ) because this was considered as a vaccine failure in many studies (e.g. those assessing a 3+0 or 2+1 schedule). Interestingly, stratifying by the number of doses indicated two patterns of breakthrough cases according to serotype. Serotypes 1, 6A and 7F occurred relatively more commonly after 1 dose of PCV, compared to after 2 doses (). In contrast, the relative proportions of serotypes 3, 6B, 14, 19A and 19F were at least as high, if not higher, after 2 doses of PCV than after 1, although the absolute number of cases for many serotypes was small ().
shows vaccine failures by serotype according to PCV schedule. The patterns were similar for 2+1 and 3+0 schedules, with a higher proportion of failures for serotypes 3, 19A and 19F than other serotypes, although the number of studies included varied substantially by schedule, limiting the robustness of these comparisons. For serotype 3, vaccine failures were observed relatively more frequently (vs other serotypes) with 3+1 schedules compared to 2+1 schedules, while the opposite was seen for serotype 19A. However, only a small number of cases contributed to the analysis of vaccine failures after a 3+1 schedule (n = 17).
Figure 4. Vaccine failures after receipt of a 2+1, 3+0 or 3+1 PCV13 or PCV10 schedule reported for each serotype in the included studies, shown as proportions of the total vaccine failures across serotypes. 2+1, schedule consisting of 2 primary doses and a booster; 3+0, schedule consisting of 3 primary doses; 3+1, schedule consisting of 3 primary doses and a booster; N, total number of reported vaccine failures after the indicated schedule, including only cases with serotype information available; PCV13, 13-valent pneumococcal conjugate vaccine; PCV10, pneumococcal non-typeable Haemophilus influenzae protein D-conjugate vaccine.
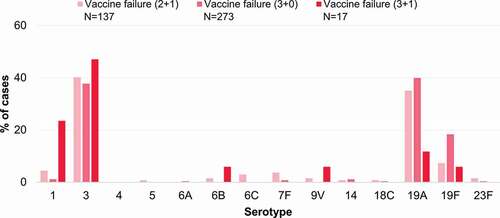
3.3. Vaccine failure and breakthrough, sub-analysis on case-control studies
Restricting the analysis to case-control studies, for which vaccination history was known for all cases, reveals a very similar pattern for serotype frequency in vaccine failure or breakthrough, with serotypes 19A and 3 being the most common, followed by serotype 19F (). Serotype 3 was more frequently isolated than 19A in vaccine failures, while the opposite was observed for vaccine breakthrough.
Figure 5. Vaccine failures or breakthrough IPD cases after PCV13 or PCV10 reported for each serotype in the included case-control studies. Panel a shows vaccine failure or breakthrough cases per serotype (as explained for panels b to d) as a proportion of all failure or breakthrough cases across case-control studies; N values indicate the latter. Panel b shows the number of vaccine-serotype IPD cases per study and serotype in children who received ≥1 dose of PCV13 or PCV10 (vaccine failures and breakthrough). Panel c shows the number of vaccine failures per study and serotype. Panel d shows the number of breakthrough cases per study and serotype. *Vaccine failure data were only available for children 7–24 months of age who received a 2-dose catch-up schedule. IPD, invasive pneumococcal disease; PCV13, 13-valent pneumococcal conjugate vaccine; PCV10, pneumococcal non-typeable Haemophilus influenzae protein D-conjugate vaccine.
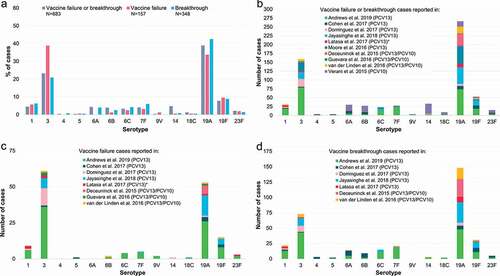
3.4. Vaccine failure and breakthrough, comparison between PCV13 and PCV10
While the absolute number of vaccine failures or breakthrough cases occurring with PCV13 or PCV10 cannot be compared directly due to the significant difference in the number of studies identified for each vaccine and the population sizes in the different studies, we assessed the distribution of serotypes most commonly associated with each PCV to evaluate if there were specific weaknesses apparent with either vaccine ().
Figure 6. Percentage of the total number of vaccine failures or breakthrough IPD cases after PCV13 or PCV10 associated with each serotype. ≥1 dose refers to all vaccine-serotype IPD cases after receipt of ≥1 dose of either PCV13 or PCV10, including those that could not be classified as either vaccine failure or breakthrough (i.e. with no further information on the exact number of doses received, which was the case for two PCV13 studies: Asner et al. [Citation27], Moore et al. [Citation41], and one PCV10 study accounting for a high proportion of all cases: Verani et al. [Citation9]). IPD, invasive pneumococcal disease; N, total number of reported vaccine failures, breakthrough cases or vaccine-serotype IPD cases after receipt of ≥1 dose of either PCV13 or PCV10, including only cases with serotype information available and excluding cases where children had received a mixed PCV13/PCV10 schedule; PCV13, 13-valent pneumococcal conjugate vaccine; PCV10, pneumococcal non-typeable Haemophilus influenzae protein D-conjugate vaccine.
![Figure 6. Percentage of the total number of vaccine failures or breakthrough IPD cases after PCV13 or PCV10 associated with each serotype. ≥1 dose refers to all vaccine-serotype IPD cases after receipt of ≥1 dose of either PCV13 or PCV10, including those that could not be classified as either vaccine failure or breakthrough (i.e. with no further information on the exact number of doses received, which was the case for two PCV13 studies: Asner et al. [Citation27], Moore et al. [Citation41], and one PCV10 study accounting for a high proportion of all cases: Verani et al. [Citation9]). IPD, invasive pneumococcal disease; N, total number of reported vaccine failures, breakthrough cases or vaccine-serotype IPD cases after receipt of ≥1 dose of either PCV13 or PCV10, including only cases with serotype information available and excluding cases where children had received a mixed PCV13/PCV10 schedule; PCV13, 13-valent pneumococcal conjugate vaccine; PCV10, pneumococcal non-typeable Haemophilus influenzae protein D-conjugate vaccine.](/cms/asset/da4ff09f-88da-497b-8ba1-36cf2a992582/ierv_a_2012455_f0006_oc.jpg)
The most frequently reported vaccine failures and breakthrough cases with PCV13 occurred with serotype 19A (40.2% of all 902 VT IPD after ≥1 dose, 35.3% of 450 failures, 42.2% of 370 breakthroughs), serotype 3 (32.3%, 41.1%, 21.6%) and, to a lesser extent, serotype 19F (10.8%, 14.4%, 9.2%). Vaccine failures and breakthrough cases with PCV10 were most commonly associated with serotypes 19A (31.9% of all 135 VT IPD after ≥1 dose, 40.0% of 10 failures, 72.7% of 33 breakthroughs), 14 (23.7%, 10.0%, 9.1%), 6B (14.1%, 10.0%, 6.1%) and 6A (12.6%, 0.0%, 3.0%) ().
3.5. Risk of bias assessment
The abstract provided too little information to adequately perform a risk of bias assessment. For the remaining studies, four were considered to have a moderate risk of bias, all for the same reason (bias in classification of interventions), while the remaining studies were considered to have a low overall risk of bias (Supplementary Table 2).
4. Discussion
This systematic review of vaccine failures and breakthrough IPD in children in the higher-valency PCV era showed that vaccine failure and breakthrough rates were low (8.4% and 9.3%, respectively, of all IPD cases in vaccinated children). These rates are consistent with the high PCV efficacy and effectiveness estimates observed in clinical studies [Citation48,Citation49] and real-world effectiveness data [Citation6,Citation7,Citation9,Citation25,Citation29,Citation32,Citation34,Citation36,Citation37,Citation41,Citation46]. Our estimated vaccine failure rate is a little higher than that observed in the systematic review by Oligbu et al., which focused primarily on PCV7 (2.1% of all IPD cases in vaccinated children being vaccine failures) [Citation19].
While only two studies evaluating a 3+0 schedule (both in Australia) allowed calculation of vaccine failure rates for this schedule, the average vaccine failure rate across these two studies was substantially higher (19.0%) than the average rate across studies assessing 2+1 or 3+1 schedules (4.2% and 5.2%, respectively). Comparisons between schedules should, however, be interpreted with caution because we did not control for possible confounders, such as differences in circulating serotypes (different serotypes may be more or less prevalent in different regions of the world [Citation17,Citation50,Citation51]), study periods in relation to when PCV13 or PCV10 were introduced, presence and duration of prior PCV7 programs, children’s age and the prevalence of IPD risk factors in the study populations. Nevertheless, the higher number of vaccine failures in Australia with a 3+0 schedule [Citation28,Citation36] as compared to the United Kingdom’s 2+1 experience [Citation52] was noted previously and prompted a review of the program, which ultimately led to a switch to a 2+1 schedule in Australia [Citation28,Citation53]. Previous systematic reviews on PCV dosing schedules concluded that 3+0, 2+1 and 3+1 schedules are all effective in reducing VT IPD, and that based on the available data, differences between schedules are difficult to determine [Citation11,Citation54]. An analysis of vaccine breakthrough cases identified through Active Bacterial Core (ABC) surveillance in children <5 years old in 10 states in the United States compared vaccine breakthrough after 2+0, 3+0, 2+1 and 3+1 schedules. The study found significantly fewer breakthrough cases after 3 than after 2 primary PCV13 doses in the first year of life before administration of the booster dose. This difference disappeared after the first year of life regardless of whether a booster was administered [Citation55].
Serotypes 19A, 3 and, to a lesser extent, 19F were the main serotypes associated with vaccine failures or breakthrough IPD across studies, both in the overall analysis and in the sub-analysis on case-control studies. This reflected the most commonly isolated VTs in children fully or partially vaccinated with PCV13 (as most studies included in our review evaluated PCV13). Two studies on vaccine failure or breakthrough in children <5 years old, published after our search end date, confirmed these results. The above-mentioned study based on ABC surveillance showed that serotypes 19A and 3 together contributed to nearly 90% (60% and 30%, respectively) of PCV13 breakthrough cases identified between 2010 and 2016, and that 19F was isolated in 7% of cases [Citation55]. In a prospective study on IPD in children treated between 2012 and 2016 in one of three hospitals in Catalonia, Spain, the most common serotypes identified in PCV13 failure/breakthrough cases were serotypes 3 (67%) and 19A (21%) [Citation56]. Vaccine failure/breakthrough cases described in these two studies likely overlapped with the cases included in our review based on the articles by Moore et al. [Citation41] and Dominguez at al [Citation32]. With PCV10, the most frequent vaccine failure and breakthrough VTs in our analysis were serotypes 19A, 14, 6B and 6A. Effectiveness of PCV13 against IPD caused by serotype 3 has been low or absent in several studies [Citation25,Citation32,Citation36,Citation57,Citation58], consistent with the immunologic hyporesponsiveness observed for this serotype [Citation59,Citation60]. A lower effectiveness of both PCV13 and PCV10 against 19A IPD compared to IPD caused by other VTs has also been observed [Citation6,Citation25,Citation32,Citation36,Citation41,Citation46,Citation57,Citation58]. Higher correlates of protection have been estimated for IPD caused by serotypes 3 (2.83 µg/mL), 19A (1.00 µg/mL) and 19F (1.17 µg/mL) compared to the other VTs (0.14–0.87 µg/mL) [Citation57], indicating that higher serum antibody concentrations may be needed for protection against IPD caused by these serotypes compared to others. Serotype 19F (together with 6B) was also commonly isolated in vaccine failures with PCV7 [Citation19]. The data for the PCV10 analysis originated largely from a single study in Brazil [Citation9], which did not have an infant PCV7 program in place before introduction of PCV10. Hence, it may not be surprising that PCV7 and PCV7-related serotypes (such as 6A, 6B and 14) were identified as these would still have been prevalent in the population shortly after PCV10 introduction. By contrast, the PCV13 analysis is largely driven by data from the United Kingdom [Citation25], Australia [Citation28,Citation36] and the United States [Citation41], which all had an established PCV7 program in place before PCV13 was introduced. The prevalence of PCV7 serotypes would therefore have already been low in these populations at the start of the PCV13 period. Other factors hampering comparisons between the two vaccines include epidemiological differences between the study populations, differences in the duration of the PCV programs when data were collected, inclusion (or not) of children with a high risk of IPD, and underreporting of 6A and 19A vaccine failure or breakthrough IPD for PCV10 in studies where these were not considered VTs. However, the latter likely did not impact our results as all studies included in our review that evaluated PCV10 considered serotypes 6A and 19A in their analyses.
For some serotypes (1, 6A, 7F), despite low numbers of cases, we noted a pattern of a higher relative proportion of VT IPD cases due to these serotypes in children receiving 1 dose of PCV than in those receiving 2 doses. In contrast, serotypes 3, 6B, 14 (small number of cases), 19A and 19F appear to be represented as much or more among breakthrough cases after 2 doses, perhaps suggesting higher antibody levels are required compared to other serotypes. For serotypes 3, 19A and 19F (but not 6B and 14), this is in line with the higher estimated correlates of protection [Citation57].
While it is difficult to compare schedules given the differing number and sizes of studies included in this review and the inherent differences between study populations and settings, the serotypes associated with the most common vaccine failures or breakthrough cases were mostly consistent across 2+1, 3+0 or 3+1 schedules. Among the more common vaccine failure serotypes, only serotype 3 was associated with a higher proportion of vaccine failures after a 3+1 schedule when compared to 2+1 or 3+0 (although only a small number of cases contributed to the assessment of the 3+1 schedule). This may be consistent with the observation that in both our overall analysis and the sub-analysis on case-control studies, serotype 3 was relatively more represented among vaccine failures than breakthrough cases. While clinical trial data suggest a minimal difference between a 2+1 and 3+1 schedule in terms of effectiveness against VT IPD [Citation49,Citation54], it is likely that a reduction to only 1 priming dose may be associated with an increase in VT IPD. The recent decision to switch to a 1+1 schedule in the United Kingdom [Citation61,Citation62] will soon provide a real-world setting to evaluate the impact of a reduced schedule on VT IPD.
Our review has several limitations. The search was limited primarily to English language articles; it is therefore likely that we did not consider possibly relevant publications in other languages. In addition, as the primary keyword search was focused on vaccine failures, we may have not considered publications reporting breakthrough cases while excluding vaccine failures. Not all studies included in our review provided data on all serotypes; some only reported the most frequent serotypes (e.g. Agier et al. [Citation23]) and some specifically reported serotypes 3 and/or 19A (e.g. Antachopoulos et al. [Citation26], De Wals et al. [Citation30]). This may have caused reporting bias that may have led to a bias in the estimates of vaccine failure or breakthrough. However, as these studies contributed less than 5% of cases in our analysis, the impact of this is likely minimal. In addition, reporting bias might have been present for 19A vaccine failures or breakthrough IPD in countries with a high level of concern over serotype 19A and for serotypes 6A and 19A vaccine failures or breakthrough in studies for which they were not considered as vaccine-related serotypes of PCV10 (although, as stated earlier, this effect may have been negligible). Where possible, we excluded cases in immunocompromised children or children with other comorbidities that may increase the risk of IPD. However, information on comorbidities for vaccine failures and breakthrough cases was not available for several studies. It is therefore likely that a portion of cases occurred in children with underlying conditions. In their review, focusing primarily on PCV7 failures, Oligbu et al. found that almost half of vaccine failures occurred in children with significant underlying comorbidities [Citation19]. As most studies did not report the exact age of breakthrough or vaccine failure cases, we could not assess the impact of time since last dose. It is possible that a substantial number of vaccine failures occurred many months up to years after the last dose and could have occurred due to waning immunity. Furthermore, we could not include all datasets in the calculations of vaccine failure and breakthrough rates because denominator data (number of IPD cases in vaccinated children) were not available for all studies. Finally, the studies included in our analysis did not contain information on sequence types or clonal complexes of the serotypes reported as vaccine failure or breakthrough. We could therefore not evaluate if certain genotypes were more likely to be associated with vaccine failure or breakthrough than others.
5. Conclusion
Vaccine failures and breakthrough IPD are uncommon in children vaccinated with PCV13 or PCV10, irrespective of the administered schedule. Vaccine failures appear to be more common after 3+0 than after 2+1 or 3+1 schedules. However, data are too limited for a robust comparison, and other factors may have confounded these results. Serotypes 19A, 3 and, to a lesser extent, 19F were the most common serotypes associated with vaccine failures and breakthrough IPD in PCV13 studies, and serotypes 14, 6B and vaccine-related 19A and 6A in PCV10 studies. The analysis for PCV10 was however limited by the small number of studies evaluating this vaccine.
6. Expert opinion
The low rate of vaccine failure and breakthrough observed with the two PCVs currently used globally confirms the high effectiveness of both PCVs in preventing VT IPD. This information is valuable for countries evaluating and interpreting their own data to provide context to assess if their current programs are meeting their objectives, particularly in the context of PCV schedules implemented. Despite differences in the vaccine formulations, this analysis suggests similar serotypes are responsible for the majority of vaccine failure and breakthrough for both vaccines.
This is important as the constant discussion over which vaccine is better or more appropriate is distracting from the more important objective of increasing coverage and preventing the most disease possible. The World Health Organization has concluded that ‘there is currently insufficient evidence that the two vaccines differ in their impact on overall pneumococcal disease burden’ and recommends that ‘the choice of product to be used in a country should be based on programmatic characteristics, vaccine supply, vaccine price, the local and regional prevalence of vaccine serotypes and antimicrobial resistance patterns’ [Citation1].
With extended-valency PCVs likely to be introduced in the next couple of years (e.g. the 15-valent and 20-valent PCVs currently under development [Citation63,Citation64]), the prediction is that these will continue to help prevent additional pneumococcal disease. As the serotypes targeted by vaccination are generally assumed to be the most important, both epidemiologically and clinically, this expansion of theoretical serotype coverage is valuable and will provide incremental impact in some countries according to their local epidemiology. It is important to note that in the absence of vaccine pressure, PCV7 serotypes were the most frequent causes of disease in North America, Oceania and Europe, while some of the additional PCV13 serotypes were already prominent in other parts of the world [Citation50,Citation51]. As PCV7 serotypes were suppressed through PCV7, the share of additional PCV13 serotypes in IPD increased, demonstrating a second tier of priority serotypes [Citation65–67]. This tiered serotype priority process will continue with extended-valency PCVs, which will be effective in reducing disease, provided that the protection offered to all VTs is maintained. This evolution of VTs included in vaccines has been mirrored in the serotypes most frequently associated with vaccine failures or breakthrough disease while moving from PCV7 to PCV10/PCV13 periods. With a future change to 15-valent or 20-valent PCVs [Citation63,Citation64], this evolution is likely to continue. Central to understanding this evolution is the possibility of immune interference as a greater number of serotypes are added into vaccine formulations. Reduced serotype-specific antibody responses have been observed at higher CPS and higher carrier doses [Citation68]. Consistently, the 15-valent PCV failed non-inferiority versus PCV13 for three serotypes in terms of the percentage of responders and for up to five serotypes (for a non-adjuvanted formulation) in terms of geometric mean concentration ratios [Citation64]. Immune interference has the potential to compromise the antibody responses to individual serotypes and may be reflected as higher rates of vaccine failures or breakthrough IPD following vaccine introduction. If this phenomenon continues to increase with extended-valency vaccines, other PCV13 serotypes may see a potential recurrence, reflected as either vaccine failure or breakthrough due to lower relative vaccine pressure provided by the newer vaccines. Continued surveillance and evaluation of vaccine failures and breakthrough disease will be important to ensure that optimal prevention of all VTs is maintained.
An additional consideration, beyond the scope of this discussion, relates to the observed replacement disease seen with PCVs following their implementation [Citation65,Citation69]. While short-term reductions in overall IPD are observed following PCV introductions due to reductions in VT IPD, replacement with non-VTs has been progressively observed, although the magnitude of this replacement does appear to vary by country, program or PCV used [Citation17,Citation18]. This has implications for the cost-effectiveness of introducing higher-valency, and likely more expensive, vaccines in the future. Development of serotype-independent pneumococcal protein-based vaccines that could be used in parallel with higher-valency PCVs might ultimately be the preferred way to combat pneumococcal disease [Citation70].
Abbreviations
CPS, capsular polysaccharides; IPD, invasive pneumococcal disease; PCV, pneumococcal conjugate vaccine; PCV7, 7-valent pneumococcal conjugate vaccine; PCV13, 13-valent pneumococcal conjugate vaccine; PHiD-CV/PCV10, pneumococcal non-typeable Haemophilus influenzae protein D-conjugate vaccine; PRISMA, Preferred Reporting Items for Systematic reviews and Meta-Analyses; ROBINS-I, Risk Of Bias In Non-randomized Studies - of Interventions; VT, vaccine serotype.
Article highlights
Although PCV10 and PCV13 are highly effective against vaccine-serotype invasive pneumococcal disease (VT IPD), fully or partially vaccinated children can still develop VT IPD (i.e. vaccine failure or breakthrough, respectively).
We performed a systematic review of vaccine failures and breakthrough IPD in children ≤5 years old after PCV10 or PCV13 vaccination.
Vaccine failure and breakthrough were uncommon with both vaccines (each <10% of all IPD in vaccinated children), confirming the vaccines’ high effectiveness.
The main serotypes associated with vaccine failure or breakthrough were 19A, 3 and 19F in PCV13 studies and 14, 6B and vaccine-related 19A and 6A in PCV10 studies.
Author contributions
All authors contributed to the conception and design of the analyses. Bruce A. Mungall and Javier Nieto Guevara performed the systematic review. All authors contributed to the interpretation of the results and helped draft or revised the manuscript. All authors have reviewed and approved the final article.
Trademarks
Synflorix is a trademark of the GSK group of companies. Prevnar/Prevenar and Prevnar 13/Prevenar 13 are trademarks of Pfizer Inc.
Declaration of interests
Javier Nieto Guevara and Lamine Soumahoro are, and Bruce A. Mungall and Bernard Hoet were employed by the GSK group of companies and declare financial and non-financial relationships and activities. All authors hold shares in the GSK group of companies. The authors have no other relevant affiliations or financial involvement with any organization or entity with a financial interest in or financial conflict with the subject matter or materials discussed in the manuscript.
Reviewer disclosures
A reviewer on this manuscript has disclosed a current research grant to their institution from Pfizer. Peer reviewers on this manuscript have no relevant financial or other relationships to disclose.
Acknowledgments
The authors thank Modis Life Sciences, Belgium, for editorial assistance and manuscript coordination, on behalf of GSK: Natalie Denef provided writing support and Stéphanie Deroo provided support with extraction of data, verification of extracted data and manuscript coordination. The authors also thank Nicolas Lecrenier (NL) for his contributions to the early stages of the systematic literature search, data extraction and analysis. Preliminary results of our analysis were presented at the 11th International Symposium on Pneumococci & Pneumococcal Diseases (ISPPD-11), 15–19 April 2018, Melbourne, Australia.
Additional information
Funding
References
- World Health Organization. Pneumococcal conjugate vaccines in infants and children under 5 years of age: WHO position paper – february 2019. Wkly Epidemiol Rec. 2019;94(8):85–104.
- Cohen O, Knoll M, O’Brien K, et al. Pneumococcal conjugate vaccine (PCV) product assessment. [Cited 2021 Jan 19] Available from: https://www.jhsph.edu/ivac/wp-content/uploads/2018/05/pcv-product-assessment-april-25-2017.pdf.
- Poolman JT, Peeters CCAM, van Den Dobbelsteen GPJM. The history of pneumococcal conjugate vaccine development: dose selection. Expert Rev Vaccines. 2013;12(12):1379–1394.
- Ganaie F, Saad JS, McGee L, et al. A new pneumococcal capsule type, 10D, is the 100th serotype and has a large cps fragment from an oral Streptococcus. mBio. 2020;11(3):e00937–20.
- De Wals P, Lefebvre B, Markowski F, et al. Impact of 2+1 pneumococcal conjugate vaccine program in the province of Quebec, Canada. Vaccine. 2014;32(13):1501–1506.
- Deceuninck G, De Serres G, Boulianne N, et al. Effectiveness of three pneumococcal conjugate vaccines to prevent invasive pneumococcal disease in Quebec, Canada. Vaccine. 2015;33(23):2684–2689.
- Domingues CMAS, Verani JR, Montenegro Renoiner EI, et al. Effectiveness of ten-valent pneumococcal conjugate vaccine against invasive pneumococcal disease in Brazil: a matched case-control study. Lancet Respir Med. 2014;2(6):464–471.
- Jokinen J, Rinta-Kokko H, Siira L, et al. Impact of ten-valent pneumococcal conjugate vaccination on invasive pneumococcal disease in Finnish children–a population-based study. PLoS One. 2015;10(3):e0120290.
- Verani JR, Domingues CMAS, de Moraes JC. Indirect cohort analysis of 10-valent pneumococcal conjugate vaccine effectiveness against vaccine-type and vaccine-related invasive pneumococcal disease. Vaccine. 2015;33(46):6145–6148.
- Prymula R, Schuerman L. 10-valent pneumococcal nontypeable Haemophilus influenzae PD conjugate vaccine: synflorix. Expert Rev Vaccines. 2009;8(11):1479–1500.
- Cohen O, Knoll M, O’Brien K, et al. Pneumococcal conjugate vaccine (PCV) review of impact evidence (PRIME): summary of findings from systematic review, October 2017. [ Cited 2021 19 January] Available from: https://www.who.int/immunization/sage/meetings/2017/october/3_FULL_PRIME_REPORT_2017Sep26.pdf?ua=1.
- Cohen R, Cohen JF, Chalumeau M, et al. Impact of pneumococcal conjugate vaccines for children in high- and non-high-income countries. Expert Rev Vaccines. 2017;16(6):625–640.
- de Oliveira LH, Camacho LAB, Coutinho ESF, et al., Impact and effectiveness of 10 and 13-valent pneumococcal conjugate vaccines on hospitalization and mortality in children aged less than 5 years in Latin American countries: a systematic review. PLoS One. 2016;11(12):e0166736.
- Mrkvan T, Pelton SI, Ruiz-Guinazu J, et al. Effectiveness and impact of the 10-valent pneumococcal conjugate vaccine, PHiD-CV: review of clinical trials and post-marketing experience. Expert Rev Vaccines. 2018;17(9):797–818.
- Ngocho JS, Magoma B, Olomi GA, et al. Effectiveness of pneumococcal conjugate vaccines against invasive pneumococcal disease among children under five years of age in Africa: a systematic review. PLoS One. 2019;14(2):e0212295.
- Naucler P, Galanis I, Morfeldt E, et al., Comparison of the impact of pneumococcal conjugate vaccine 10 or pneumococcal conjugate vaccine 13 on invasive pneumococcal disease in equivalent populations. Clin Infect Dis. 2017;65(11):1780–1789.
- Balsells E, Guillot L, Nair H, et al. Serotype distribution of Streptococcus pneumoniae causing invasive disease in children in the post-PCV era: a systematic review and meta-analysis. PLoS One. 2017;12(5):e0177113.
- Izurieta P, Bahety P, Adegbola R, et al. Public health impact of pneumococcal conjugate vaccine infant immunization programs: assessment of invasive pneumococcal disease burden and serotype distribution. Expert Rev Vaccines. 2018;17(6):479–493.
- Oligbu G, Hsia Y, Folgori L, et al., Pneumococcal conjugate vaccine failure in children: a systematic review of the literature. Vaccine. 2016;34(50):6126–6132.
- Page MJ, McKenzie JE, Bossuyt PM, et al. The PRISMA 2020 statement: an updated guideline for reporting systematic reviews. BMJ. 2021;372:n71.
- Sterne JA, Hernan MA, Reeves BC, et al. ROBINS-I: a tool for assessing risk of bias in non-randomised studies of interventions. BMJ. 2016;355:i4919.
- Grant LR, O’Brien SE, Burbidge P, et al. Comparative immunogenicity of 7 and 13-valent pneumococcal conjugate vaccines and the development of functional antibodies to cross-reactive serotypes. PLoS One. 2013;8(9):e74906.
- Agier MS, Marchand S, Paret N, et al. [Post-licensure safety surveillance for Prevenar 13((R)) in France]. Arch Pediatr. 2017;24(5):439–444.
- Almeida AF, Sobrinho-Simoes J, Ferraz C, et al. Pneumococcal pneumonia vaccine breakthroughs and failures after 13-valent pneumococcal conjugated vaccine. Eur J Public Health. 2016;26(5):887–889.
- Andrews N, Kent A, Amin-Chowdhury Z, et al. Effectiveness of the seven-valent and thirteen-valent pneumococcal conjugate vaccines in England: the indirect cohort design, 2006–2018. Vaccine. 2019;37(32):4491–4498.
- Antachopoulos C, Tsolia MN, Tzanakaki G, et al. Parapneumonic pleural effusions caused by Streptococcus pneumoniae serotype 3 in children immunized with 13-valent conjugated pneumococcal vaccine. Pediatr Infect Dis J. 2014;33(1):81–83.
- Asner SA, Agyeman PKA, Gradoux E, et al. Burden of Streptococcus pneumoniae sepsis in children after introduction of pneumococcal conjugate vaccines: a prospective population-based cohort study. Clin Infect Dis. 2019;69(9):1574–1580.
- Blyth CC, Jayasinghe S, Andrews RM. A rationale for change: an increase in Invasive Pneumococcal Disease in fully vaccinated children. Clin Infect Dis. 2020;70(4):680–683.
- Cohen C, Von Mollendorf C, de Gouveia L, et al. Effectiveness of the 13-valent pneumococcal conjugate vaccine against invasive pneumococcal disease in South African children: a case-control study. Lancet Glob Health. 2017;5(3):e359–e369.
- De Wals P, Lefebvre B, Deceuninck G, et al., Incidence of invasive pneumococcal disease before and during an era of use of three different pneumococcal conjugate vaccines in Quebec. Vaccine. 2018;36(3):421–426.
- Diawara I, Zerouali K, Katfy K, et al. Invasive pneumococcal disease among children younger than 5 years of age before and after introduction of pneumococcal conjugate vaccine in Casablanca, Morocco. Int J Infect Dis. 2015;40:95–101.
- Dominguez A, Ciruela P, Hernandez S, et al. Effectiveness of the 13-valent pneumococcal conjugate vaccine in preventing invasive pneumococcal disease in children aged 7–59 months. A matched case-control study. PLoS One. 2017;12(8):e0183191.
- Godot C, Levy C, Varon E, et al. Pneumococcal meningitis vaccine breakthroughs and failures after routine 7-valent and 13-valent pneumococcal conjugate vaccination in children in France. Pediatr Infect Dis J. 2015;34(10):e260–263.
- Guevara M, Barricarte A, Torroba L, et al. Direct, indirect and total effects of 13-valent pneumococcal conjugate vaccination on invasive pneumococcal disease in children in Navarra, Spain, 2001 to 2014: cohort and case-control study. Euro Surveill. 2016;21(14):30186.
- Harboe ZB, Dalby T, Weinberger DM, et al. Impact of 13-valent pneumococcal conjugate vaccination in invasive pneumococcal disease incidence and mortality. Clin Infect Dis. 2014;59(8):1066–1073.
- Jayasinghe S, Chiu C, Quinn H, et al. Effectiveness of 7- and 13-valent pneumococcal conjugate vaccines in a schedule without a booster dose: a 10-year observational study. Clin Infect Dis. 2018;67(3):367–374.
- Latasa P, Ordobas M, Garrido-Estepa M, et al. Effectiveness of different vaccine schedules for heptavalent and 13-valent conjugate vaccines against pneumococcal disease in the Community of Madrid. Vaccine. 2017;35(40):5381–5387.
- Mackenzie GA, Hill PC, Jeffries DJ, et al. Effect of the introduction of pneumococcal conjugate vaccination on invasive pneumococcal disease in The Gambia: a population-based surveillance study. Lancet Infect Dis. 2016;16(6):703–711.
- Madhi F, Godot C, Bidet P, et al. Serotype 3 pneumococcal pleural empyema in an immunocompetent child after 13-valent pneumococcal conjugate vaccine. Pediatr Infect Dis J. 2014;33(5):545–546.
- Mereckiene J, Fitzgerald M, Cotter S, et al. Invasive pneumococcal disease (IPD) and effect of pneumococcal conjugate vaccines in Ireland in 2008–2013. European Scientific Conference on Applied Infectious Disease Epidemiology; 2014;Stockholm, Sweden.
- Moore MR, Link-Gelles R, Schaffner W, et al. Effectiveness of 13-valent pneumococcal conjugate vaccine for prevention of invasive pneumococcal disease in children in the USA: a matched case-control study. Lancet Respir Med. 2016;4(5):399–406.
- Novak D, Lundgren A, Westphal S, et al. Two cases of hemolytic uremic syndrome caused by Streptococcus pneumoniae serotype 3, one being a vaccine failure. Scand J Infect Dis. 2013;45(5):411–414.
- Okonji Z, Kozakova J. Molecular characterization of Streptococcus pneumoniae isolates recovered from cases of pneumococcal vaccine failure in children under five years of age in the Czech Republic in 2012–2014. Epidemiol Mikrobiol Imunol. 2018;67(3):99–103.
- Ong R, Tan N, Chong C, et al. Pediatric invasive pneumococcal disease associated with pneumococcal conjugate vaccine failures in Singapore: a case series. 33rd Annual Meeting of the European Society for Paediatric Infectious Diseases; 2015;Leipzig, Germany.
- Sutcu M, Akturk H, Karagozlu F, et al. Empyema due to Streptococcus pneumoniae serotype 9V in a child immunized with 13-valent conjugated pneumococcal vaccine. Balkan Med J. 2017;34(1):74–77.
- van der Linden M, Falkenhorst G, Perniciaro S, et al. Effectiveness of pneumococcal conjugate vaccines (PCV7 and PCV13) against invasive pneumococcal disease among children under two years of age in Germany. PLoS One. 2016;11(8):e0161257.
- Corcoran M, Mereckiene J, Cotter S, et al. Invasive Streptococcus pneumoniae infections and vaccine failures in children in Ireland from the postvaccine era from 2007 to 2018. Pediatr Infect Dis J. 2020;39(4):339–344.
- Tregnaghi MW, Saez-Llorens X, Lopez P, et al. Efficacy of pneumococcal nontypable Haemophilus influenzae protein D conjugate vaccine (PHiD-CV) in young Latin American children: a double-blind randomized controlled trial. PLoS Med. 2014;11(6):e1001657.
- Palmu AA, Jokinen J, Borys D, et al. Effectiveness of the ten-valent pneumococcal Haemophilus influenzae protein D conjugate vaccine (PHiD-CV10) against invasive pneumococcal disease: a cluster randomised trial. Lancet. 2013;381(9862):214–222.
- Hausdorff WP, Bryant J, Paradiso PR, et al. Which pneumococcal serogroups cause the most invasive disease: implications for conjugate vaccine formulation and use, part I. Clin Infect Dis. 2000;30(1):100–121.
- Johnson HL, Deloria-Knoll M, Levine OS, et al. Systematic evaluation of serotypes causing invasive pneumococcal disease among children under five: the pneumococcal global serotype project. PLoS Med. 2010;7(10):e1000348.
- Oligbu G, Collins S, Andrews N, et al. Characteristics and serotype distribution of childhood cases of invasive pneumococcal disease following pneumococcal conjugate vaccination in England and Wales, 2006–2014. Clin Infect Dis. 2017;65(7):1191–1198.
- Australian Government Department of Health. Australian Technical Advisory Group on Immunisation. Public consultation on the infant pneumococcal vaccination schedule. [cited 2021 Mar 9]. Available from: https://consultations.health.gov.au/ohp-immunisation-branch/infant-pneumococcal-vaccination-schedule-recommend/supporting_documents/Public%20Consultation%20Document%20%20Infant%20Pneumococcal%20Vaccination%20Schedule%20Recommendations.pdf.
- Conklin L, Loo JD, Kirk J, et al. Systematic review of the effect of pneumococcal conjugate vaccine dosing schedules on vaccine-type invasive pneumococcal disease among young children. Pediatr Infect Dis J. 2014;33(Suppl 2):S109–118.
- Adebanjo TA, Pondo T, Yankey D, et al. Pneumococcal Conjugate Vaccine Breakthrough Infections: 2001–2016. Pediatrics. 2020;145(3):e20190836.
- Hernández S, Moraga-Llop F, Díaz A, et al. Failures of 13-Valent Conjugated Pneumococcal Vaccine in Age-Appropriately Vaccinated Children 2–59 Months of Age, Spain. Emerg Infect Dis. 2020;26(6):1147–1155.
- Andrews NJ, Waight PA, Burbidge P, et al. Serotype-specific effectiveness and correlates of protection for the 13-valent pneumococcal conjugate vaccine: a postlicensure indirect cohort study. Lancet Infect Dis. 2014;14(9):839–846.
- Weinberger R, van der Linden M, Imohl M, et al. Vaccine effectiveness of PCV13 in a 3+1 vaccination schedule. Vaccine. 2016;34(18):2062–2065.
- Linley E, Bell A, Gritzfeld JF, et al. Should pneumococcal serotype 3 be included in serotype-specific immunoassays? Vaccines (Basel). 2019;7(1):4.
- Poolman J, Borrow R. Hyporesponsiveness and its clinical implications after vaccination with polysaccharide or glycoconjugate vaccines. Expert Rev Vaccines. 2011;10(3):307–322.
- Joint Committee on Vaccination and Immunization (JCVI). Minute of the meeting on 04 October 2017. [Cited 2021 Jan 26] Available from: https://app.box.com/s/iddfb4ppwkmtjusir2tc/file/247634612957.
- Public Health England. Pneumococcal vaccination: infant schedule changes from January 2020 letter. [ Cited 2021 26 January] Available from: https://www.gov.uk/government/publications/pneumococcal-vaccination-infant-schedule-changes-from-january-2020-letterr.
- Thompson A, Lamberth E, Severs J, et al. Phase 1 trial of a 20-valent pneumococcal conjugate vaccine in healthy adults. Vaccine. 2019;37(42):6201–6207.
- Greenberg D, Hoover PA, Vesikari T, et al. Safety and immunogenicity of 15-valent pneumococcal conjugate vaccine (PCV15) in healthy infants. Vaccine. 2018;36(45):6883–6891.
- Feikin DR, Kagucia EW, Loo JD, et al. Serotype-specific changes in invasive pneumococcal disease after pneumococcal conjugate vaccine introduction: a pooled analysis of multiple surveillance sites. PLoS Med. 2013;10(9):e1001517.
- Hicks LA, Harrison LH, Flannery B, et al. Incidence of pneumococcal disease due to non-pneumococcal conjugate vaccine (PCV7) serotypes in the United States during the era of widespread PCV7 vaccination, 1998–2004. J Infect Dis. 2007;196(9):1346–1354.
- Isaacman DJ, McIntosh ED, Reinert RR. Burden of invasive pneumococcal disease and serotype distribution among Streptococcus pneumoniae isolates in young children in Europe: impact of the 7-valent pneumococcal conjugate vaccine and considerations for future conjugate vaccines. Int J Infect Dis. 2010;14(3):e197–209.
- Dagan R, Poolman J, Siegrist CA. Glycoconjugate vaccines and immune interference: a review. Vaccine. 2010;28(34):5513–5523.
- Weinberger DM, Malley R, Lipsitch M. Serotype replacement in disease after pneumococcal vaccination. Lancet. 2011;378(9807):1962–1973.
- Scott NR, Mann B, Tuomanen EI, et al. Multi-Valent Protein Hybrid Pneumococcal Vaccines: a Strategy for the Next Generation of Vaccines. Vaccines (Basel). 2021;9(3):209.