ABSTRACT
Introduction
The two currently licensed surface protein non-capsular meningococcal serogroup B (MenB) vaccines both have the purpose of providing broad coverage against diverse MenB strains. However, the different antigen compositions and approaches used to assess breadth of coverage currently make direct comparisons complex.
Areas covered
In the second of two companion papers, we comprehensively review the serology and factors influencing breadth of coverage assessments for two currently licensed MenB vaccines.
Expert opinion
Surface protein MenB vaccines were developed using different approaches, resulting in unique formulations and thus their breadth of coverage. The surface proteins used as vaccine antigens can vary among meningococcal strains due to gene presence/absence, sequence diversity, and differences in protein expression. Assessment of the breadth of coverage provided by vaccines is influenced by the ability to induce cross-reactive functional immune responses to sequence diverse protein variants; the characteristics of the circulating invasive strains from specific geographic locations; methodological differences in the immunogenicity assays; differences in human immune responses between individuals; and the maintenance of protective antibody levels over time. Understanding the proportion of meningococcal strains, which are covered by the two licensed vaccines, is important in understanding protection from disease and public health use.
1. Introduction
The Gram-negative bacterium Neisseria meningitidis is the cause of invasive meningococcal disease (IMD) and remains a significant global cause of morbidity and mortality [Citation1]. Differences in the capsular polysaccharide allow classification of the meningococcus into 12 serogroups, of which five (A, B, C, W, and Y) have historically been responsible for 96% of IMD cases worldwide [Citation2,Citation3]. Serogroup X has recently emerged and caused outbreaks within the African meningitis belt [Citation2]. Vaccination is the most effective approach for the prevention of IMD [Citation4] with vaccines now available for the five most prevalent disease-causing serogroups and a serogroup X containing vaccine is under development [Citation5–7].
The capsular polysaccharides of serogroups A, C, W, and Y have been successfully used as vaccine antigens, initially as free polysaccharides and more recently in formulations conjugated to a carrier protein [Citation3]. As reviewed in detail in the accompanying manuscript, the responses induced by each polysaccharide antigen are protective against IMD caused by strains of the corresponding serogroup [Citation8]. This is due to capsular expression being required for survival of the meningococcus during invasive disease in almost all immunocompetent hosts and the limited structural variation in the capsular polysaccharide among strains of a given serogroup [Citation9]. The serologic evaluation of these vaccines in the serum bactericidal antibody (SBA) assay is uncomplicated as it may be undertaken using a representative strain for each serogroup [Citation10].
The serogroup B (MenB) polysaccharide is not a suitable vaccine antigen as it is poorly immunogenic and may potentially induce auto-antibodies [Citation11]. Vaccines for MenB have therefore had to use alternative antigens found on the bacterial surface [Citation12]. Outer membrane vesicle (OMV) vaccines were the first successfully developed and implemented in response to clonal MenB outbreaks in multiple countries [Citation13–15]. Despite OMVs containing many cell membrane–associated antigens that are presented to the immune system, the surface exposed epitopes can be highly variable and certain antigens are immunodominant. Specifically, protective immune responses are well documented against Porin A (PorA). This antigen is immunodominant in meningococcal OMV vaccines; however, the regions of PorA that are immunologically accessible are small and highly variable [Citation16]. These monovalent OMV vaccines only provide a limited breadth of coverage. Although they were effective in controlling localized outbreaks due to a single clonal strain expressing a PorA variant matching the OMV vaccine, they have little utility in other geographic locations where disease is caused by diverse strains expressing different PorA variants [Citation16–18]. In an attempt to increase the breadth of coverage, multi-valent OMV vaccine formulations have been developed [Citation19–22]. However, none of these successfully progressed through to licensure [Citation16].
To date, the search for a conserved, single or combination of meningococcal surface protein antigens that could provide complete coverage of all invasive MenB strains has proved challenging. Despite this, two meningococcal surface protein vaccines are now licensed for the prevention of MenB disease and are expected to confer broad protection. In 2013, the first meningococcal surface protein vaccine 4CMenB (Bexsero®, GSK Vaccines, Srl; Sovicille, Italy) was licensed in Europe and was immediately followed by MenB-FHbp (Trumenba®, bivalent rLP2086; Pfizer Inc, Philadelphia, PA, USA) in 2014 in the United States [Citation6,Citation23]. The proportion of MenB strains that are killed by immune sera elicited in response to these surface protein vaccines (i.e. their breadth of coverage) becomes fundamental to understanding their utility. The breadth of coverage afforded by each vaccine is driven by the choice of protein antigens and the ability of these antigens to induce cross-reactive immune responses to diverse variants, which can vary between individuals and be age-related [Citation24,Citation25]. Other considerations are the susceptibility of circulating invasive MenB strains, notably whether the protein(s) recognized by vaccine-induced antibody is expressed at levels sufficient for complement-mediated bactericidal killing.
The significantly different formulations of 4CMenB and MenB-FHbp affect their immunogenicity and therefore the breadth of coverage they will likely provide. The serologic evaluation of these vaccines has been undertaken measuring the same immune correlate of protection used for meningococcal ACWY polysaccharide conjugate vaccines, the SBA assay using human complement (hSBA) [Citation10]. The hSBA assays used for 4CMenB and MenB-FHbp vaccines have methodological differences and completely different strategies for selection of MenB assay strains [Citation12]. These differences impact the amount of information provided on breadth of coverage by each approach and result in an inability to directly compare the vaccines. Vaccine effectiveness data recently published for 4CMenB confirms that protein-based vaccines, licensed on the basis of hSBA, can prevent a substantial proportion of MenB disease cases [Citation26–28]. As exemplified by the data from England following the introduction of 4CMenB into infant and toddler age groups, these data have also demonstrated that 4CMenB does not provide ubiquitous coverage and has emphasized the importance of understanding breadth of coverage [Citation28].
This paper is the second of two companion papers that comprehensively review the complex area of serology and breadth of coverage assessment for MenB vaccines. The first reviewed the historical and transferable lessons; here we review the approaches undertaken for the licensure and assessment of 4CMenB and MenB-FHbp [Citation8]. Collectively, these two reviews provide the knowledge to understand and interpret the currently available data and provide considerations for the evaluation of current and future meningococcal surface protein vaccines.
2. Meningococcal surface protein vaccines
Whereas meningococcal conjugate vaccines containing the same serogroup polysaccharide are broadly similar to each other, the two licensed meningococcal surface protein vaccines are significantly different. To provide broad coverage, 4CMenB is comprised of four main antigens () [Citation29]. Three of these are recombinant-produced meningococcal proteins and were identified from their genomic sequence; factor H binding protein (FHbp), Neisserial heparin binding antigen (NHBA) and Neisseria adhesin A (NadA). The fourth component is delivered through an OMV preparation from the New Zealand meningococcal outbreak strain NZ 98/254, which is often simplified as a PorA antigen (P1.4) due to the immunodominance of this protein in the OMV [Citation6]. The NZ 98/254 derived OMVs include a core set of 21 additional proteins that comprise >90% of the OMV, as well as other minor proteins. Two accessory proteins, 936 (also termed GNA2091) and 953 (also termed GNA1030), originally identified as candidate antigens from genomic sequence and shown to induce SBA responses, are expressed as fusion proteins with FHbp and NHBA, respectively [Citation30]. These fusion proteins were shown to improve the immunogenicity of FHbp and NHBA antigens compared to the unfused presentation [Citation31].
Table 1. Current meningococcal surface protein vaccines and antigens
The FHbp antigen was also independently identified as a promising antigen by a traditional vaccinology approach of biochemical and immunological screening. In this effort, multiple meningococcal strains were used in SBA assay screens so that PorA-independent protein fractions that confer broad protection against diverse strains could be identified. In doing this, FHbp was identified as an antigen that could confer broad protection against MenB strains, which led to the development of MenB-FHbp [Citation23]. Initial research confirmed that recombinant FHbp was immunogenic and capable of inducing SBA cross-reactive against MenB strains expressing different FHbp sequence variants [Citation32–34]. Further pivotal research demonstrated that this broad protection was driven by the inclusion of the antigen’s lipidated tail as immunogenicity and breadth of coverage were reduced with its removal [Citation32–34]. By utilizing this benefit of lipidation, two diverse antigenic FHbp variants were identified that could provide broad coverage across the diversity of FHbp and therefore the diversity of disease-causing MenB strains. Thus, MenB-FHbp contains two lipidated FHbp antigens, one from each of the two immunologically distinct FHbp subfamilies A and B (; ) [Citation35,Citation36]. Although both 4CMenB and MenB-FHbp contain FHbp antigens, their different presentations as a non-lipidated fusion protein in 4CMenB and as a lipidated non-fusion protein in MenB-FHbp directly influences their relative immunogenicity.
Figure 1. Phylogenetic tree for FHbp.
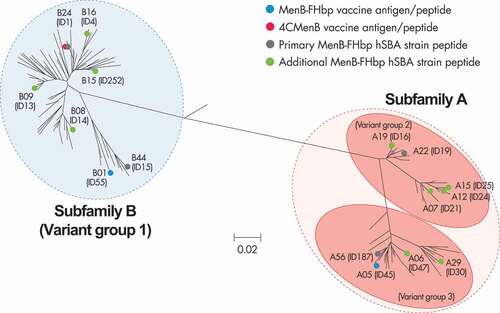
3. Importance of breadth of coverage for current meningococcal surface protein vaccines
Defining the breadth of coverage of individual surface protein antigens and collective vaccine formulations is crucial and unique among the meningococcal vaccines and is not a consideration for capsular-based vaccines. In the first of the companion papers, we explored the generic drivers impacting coverage, including those associated with strains such as gene presence/absence, sequence diversity, and expression, and those associated with the vaccine such as immunogenicity, cross-reactivity of SBA, and age-related immunogenicity () [Citation8]. Here, we focus on the protein antigens used in 4CMenB and MenB-FHbp.
Table 2. Breadth of coverage of meningococcal surface protein vaccines: factors impacting immunogenicity and the ability of induced antibody to provide protection across diverse strains
Both vaccines incorporate FHbp, although the number of variants included and their presentation differ. Almost all invasive MenB strains harbor the FHbp gene encoding a single sequence variant as evidenced by a global study where all 1814 isolates possessed FHbp and a European study where only one of 1052 isolates did not [Citation37,Citation38]. The protein plays an integral role in bacterial survival as it binds the complement down-regulatory protein factor H, leading to evasion of complement-mediated bacterial lysis [Citation12]. This protective role has been confirmed in studies where deletion of FHbp resulted in decreased bacterial survival [Citation39,Citation40]. Despite the near ubiquitous nature of the FHbp gene in MenB isolates there is considerable sequence diversity; 1728 alleles encoding 1335 peptide variants have been identified as of October 2020 [Citation41]. Due to concurrent discovery at different institutions, there are different FHbp nomenclature schemes in use. The first scheme, based on overall phylogenetic distribution of FHbp sequence data and supported by immunological analysis of the lipidated antigens, clusters peptides into two subfamilies (A and B) [Citation32]. A second scheme, based on an initial evaluation of a small number of strains, clusters peptides into three variant groups (1, 2, and 3) () [Citation12]. In this review, we detail FHbp using the subfamily followed by the peptide identifier and also provide the peptide ID from the PubMLST website in brackets e.g. A05 (ID45) [Citation42].
Expression of surface proteins by MenB strains is critical when considering breadth of coverage as sufficient protein must be present to enable binding of SBA for complement-mediated lysis to occur. A study of a large global collection of MenB isolates showed that FHbp surface expression varies with approximately a 65-fold difference between the lowest and highest expression. Despite this variation, the expression was normally distributed among isolates with relatively few expressing very low or very high levels [Citation38].
The ability of the non-lipidated FHbp fusion protein antigen contained in 4CMenB to induce cross-reactive responses to other FHbp peptides remains to be fully elucidated. A small early study of the non-lipidated B24 (ID1) antigen provided evidence that it induced SBA cross-reactive to MenB strains expressing other subfamily B FHbp peptides [Citation43]. The induced antibody, however, is generally not cross-reactive with all subfamily B peptides and a reduced level of SBA activity has been shown with increasing FHbp sequence divergence from the vaccine peptide [Citation44–46]. In addition, the non-lipidated subfamily B FHbp antigen does not generally induce SBA activity against subfamily A FHbp peptides in humans [Citation43,Citation44].
During MenB-FHbp development, lipidation of FHbp was shown to increase immunogenicity and the cross-reactive profile of induced SBA against diverse FHbp peptides, in part due to presentation of the antigen closer to its native lipoprotein structure [Citation32–34]. This not only included strains expressing peptides from the same FHbp subfamily but also strains expressing peptides from the alternative subfamily, albeit at lower levels [Citation36]. To provide coverage irrespective of the FHbp subfamily expressed, MenB-FHbp was formulated with the lipidated subfamily A and B antigens A05 (ID45) and B01 (ID55) [Citation32–34].
The two remaining main recombinant protein 4CMenB antigens vary among the proportion of MenB isolates that harbor the corresponding gene. A European study reported that all 1052 (100%) MenB isolates harbor the gene that codes for NHBA whereas only 22.3% had nadA. A subset of the nadA-positive strains did not include a full length gene with 8% having an interrupted or frame shifted gene preventing protein expression [Citation37]. As of October 2020 there have been 1404 and 130 peptides of NHBA and NadA identified, respectively [Citation47,Citation48]. The NadA peptides are categorized into two distinct groups, I and II, with 4CMenB containing a NadA3 peptide from group I [Citation49]. The SBA response induced by the 4CMenB NadA antigen is generally considered cross-reactive to MenB strains expressing peptides belonging to the same group, but not to group II peptides [Citation50]. Limited data detailing the relative proportion of group I and II NadA peptides in disease-causing isolates have been published; however, one study of 535 English MenB isolates reported that only 33% of those with NadA had intact genes coding group I NadA peptides [Citation37].
Despite high sequence variability, the NHBA peptides have not been grouped into any overarching structure such as variant groups or subfamilies. The 4CMenB NHBA peptide 2 has been demonstrated to induce cross-reactive SBA to some but not all strains with heterologous NHBA peptides [Citation51]. Expression levels of NHBA and NadA on MenB isolates have been almost exclusively investigated using the Meningococcal Antigen Typing System (MATS), which is covered in detail later in this review. As MATS concurrently assesses the ability of 4CMenB-induced antibody to cross-react with NHBA or NadA proteins, results are not solely a measure of isolate expression levels [Citation52]. In the absence of alternative data, expression of NHBA and NadA must be interpreted from the available MATS data. A European MATS evaluation predicted that approximately 59% of MenB strains expressed sufficient levels of a NHBA peptide that would be susceptible to 4CMenB immune sera [Citation31]. Only 7% of the isolates harboring nadA expressed sufficient levels of protein to be killed by 4CMenB immune sera, equivalent to 1.5% of all isolates overall. This low proportion of strains expressing sufficient NadA may in part be due to the MATS in vitro growth conditions, which are not optimal for NadA expression and not reflective of in vivo conditions [Citation53].
The final main antigenic component of 4CMenB are OMVs containing the immunodominant PorA peptide. Three variable regions (VRs) of amino acid sequence are collectively used to determine the PorA subtype; e.g. P1.7–2,4,37 [Citation54]. Depending on the PorA subtype, one of the VRs is often immunodominant, which for 4CMenB is the VR2 P1.4 [Citation55]. The anti-PorA SBA induced by 4CMenB OMVs has limited cross-reactivity to other PorA subtypes; coverage of 4CMenB is therefore considered to be restricted to isolates possessing the matched P1.4 VR2 [Citation55,Citation56]. The proportion of MenB strains with this PorA subtype varies geographically, with 20.2% of European MenB isolates and 5.9% of US isolates possessing the P1.4 PorA [Citation37,Citation57]. In contrast to the other protein antigens, the level of PorA expression is generally consistent across MenB strains, meaning that expression is usually not considered when evaluating vaccine coverage [Citation52]. The OMV component of 4CMenB may also have adjuvant properties that potentially increase the antigenicity of recombinant proteins [Citation24,Citation25,Citation58]. However, the other minor OMV protein components have been demonstrated to contribute significantly to the immunogenicity of 4CMenB, making it difficult to apportion the adjuvant effect [Citation52,Citation59].
Additional factors may contribute to the breadth of coverage of a surface protein vaccine being greater than predicted by individual antigens. Antibody populations against more than one protein antigen can have synergistic functional activity when in combination [Citation60]. This synergy can broaden a vaccine’s breadth of coverage among isolates that express more than one protein where SBA activity is not achieved against either protein in isolation. The surface proteins in MenB vaccines are not only harbored by MenB strains but are also found on many meningococcal strains, irrespective of capsular group [Citation45,Citation61]. Extension of MenB vaccine coverage beyond MenB strains has been shown in multiple studies but will be most beneficial in the absence of corresponding meningococcal conjugate vaccines; the latter vaccines have a superior breadth of coverage due to the capsular polysaccharide antigens and induction of herd protection [Citation45,Citation61,Citation62].
As breadth of immune coverage is reliant upon the cross-reactivity of induced antibody to diverse protein peptides, differences in individual responses can have significant impact on the coverage afforded to that individual. Such differences against different peptides have been shown for both 4CMenB and MenB-FHbp, demonstrating the importance of assessing individual and overall population responses [Citation24,Citation25,Citation63–66]. Age-related immunogenicity may also be another important consideration as indicated with 4CMenB responses and the cross-reactive profile of induced antibody to FHbp and NadA peptides being lowest with an early infant schedule and improving with age [Citation24,Citation25,Citation66]. These data emphasize that predicting breadth of coverage is enormously complex and must consider the individual responses, host factors such as complement deficiencies, subjects that may be receiving complement inhibitor therapy, the age group being vaccinated, and the MenB strains representative of the local epidemiology.
4. Evaluation of 4CMenB immunogenicity and breadth of coverage
The immune response to 4CMenB was evaluated in early studies using an IgG ELISA or the SBA assay using baby rabbit complement [Citation30,Citation67]. These were superseded by an indicator strain approach where three and subsequently four MenB strains were used in the gold standard SBA assay using human complement (hSBA) () [Citation68,Citation69]. Each indicator strain is proposed to measure SBA against one of the four main vaccine antigens and is considered ‘matched’ for that respective antigen [Citation70]. This is based on the premise that the respective indicator strains either did not harbor the gene coding for the other antigens, expressed a diverse peptide unrecognized by 4CMenB-induced antibody, or expressed insufficient protein to support SBA killing. These indicator strains were used throughout the clinical development providing immunogenicity data for each of the four main antigens against a single peptide [Citation68,Citation69]. While providing a strong indication of the vaccines ability to induce bactericidal antibodies against matched strains (rather like anti-capsular antibodies killing a meningococcal strain with the corresponding capsule, these data do not provide comprehensive information on the breadth of coverage). This is due to the assessment of breadth of coverage depending upon both the ability of vaccine-induced antibodies to cross-react with sequence diverse peptides that may also vary in the level of surface expression.
Table 3. Meningococcal serogroup B strains frequently used in the serum bactericidal antibody assay for the analysis of 4CMenB
To address the lack of data on breadth of coverage and the practical limitations in serum availability for hSBA assays, the manufacturer of 4CMenB developed MATS [Citation52]. This is an in vitro assay designed to predict 4CMenB coverage by using an ELISA to test MenB isolates to determine protein expression in conjunction with the affinity of vaccine-induced antibodies against FHbp, NadA, and NHBA. Relative potency (RP) values are calculated by comparison to a reference strain and do not require any clinical sera. Correlation of RP values with hSBA titers ≥1:8 (or ≥4-fold rises where pre-vaccination titers were ≥1:4) using pooled sera from immunized toddlers allowed the derivation of positive bactericidal thresholds (PBTs) for each of the three antigens. Using MATS, 4CMenB is predicted to protect against a MenB isolate with an RP ≥PBT for ≥1 of the 3 antigens or if the isolate harbors a P1.4 PorA. The assay has been used to predict 4CMenB coverage of country-specific MenB strain collections; these have ranged from 66% to 91% [Citation71].
Different aspects of the MATS methodology have been associated with either underestimating or overestimating 4CMenB coverage. The conservative nature of how the PBTs were assigned, lower levels of NadA expression in vitro vs in vivo, the potential for antibody synergy, and SBA against minor OMV components have all been suggested as reasons for underestimation [Citation52,Citation71]. Conversely, there are reasons why the MATS assay may over-predict the breadth of coverage [Citation72]. The PBTs were established using toddler sera where subjects had received three 4CMenB priming doses as infants followed by a toddler booster. Consequently, age-related immunogenicity is not considered and MATS has been associated with over-prediction and under-prediction of coverage in infants and adolescents, respectively [Citation52]. A final consideration is that the binary MATS ‘covered’ or ‘not covered’ result provides no information on the proportion of subjects in an age group with protective titers, which is known to vary significantly [Citation25,Citation46,Citation73]. These aspects mean that MATS predictions must be carefully interpreted, as the binary ‘covered’ or ‘not covered’ result does not inform if an individual vaccine recipient will be protected against that isolate.
Prediction of 4CMenB coverage using genotypic methods alone have also been explored thus negating the need to use live meningococcal isolates as required by MATS. Genotypic methods also have utility for cases where bacterial DNA but not isolates are recovered from the patient [Citation74]. Genetic MATS (gMATS) is an evolution of MATS for the genotypic prediction of 4CMenB coverage [Citation75]. Each FHbp and NHBA peptide with historical MATS results for ≥5 isolates were categorized as either ‘not covered,’ ‘unpredictable’ or ‘covered’ in instances where <40%, 40–60%, or >60% had MATS results ≥PBT, respectively. Coverage by the NadA antigen is not considered. A MenB isolate is considered gMATS ‘covered’ in instances where ≥1 of the FHbp and NHBA peptides are individually categorized as ‘covered’ and/or when the strain harbors P1.4 PorA. If a strain does not harbor P1.4 PorA and FHbp and NHBA peptides are categorized as ‘not covered’ then the isolate is considered gMATS ‘not covered.’ In all other cases where an FHbp or NHBA peptide is individually categorized as ‘unpredictable’ or there are fewer than five historical MATS results, the isolate is considered gMATS ‘unpredictable.’ The gMATS scheme was shown to have an accuracy of 92% when compared with MATS for overall country 4CMenB coverage predictions, and it can be easily applied by anyone with available genetic typing data without the need for additional laboratory work [Citation75]. It does, however, maintain all MATS attributes previously covered and classifies a proportion of isolates as ‘covered’ or ‘not covered’ based on genetic information when their individual MATS results might be the opposite.
Several manufacturer-independent genotypic 4CMenB coverage schemes have been established. The Bexsero Antigen Sequence Typing (BAST) scheme was incorporated into the PubMLST database and in common with gMATS, relied on MATS data [Citation76]. As the amount of available MATS data during development was limited, the scheme classifies many of the less frequent peptides as ‘unknown,’ limiting the utility of BAST. More recently, a replacement in the form of the Meningococcal Deduced Vaccine Antigen Reactivity (MenDeVAR) index has been reported that benefits from the inclusion of additional MATS data [Citation77]. In each case, the reliance of these genotypic methods on MATS data remains a limitation, and consideration must be given to these assays being a further step removed from the accepted surrogate of protection.
5. Linking 4CMenB indicator strains to breadth of coverage
The indicator strain hSBA results for 4CMenB are increasingly reported against a given antigen rather than against the strain as a whole and without considering the synergistic effects from multiple antibody populations [Citation78–80]. However, hSBA killing of indicator strains by 4CMenB immune sera is mediated against many components. For example, the NZ 98/254 PorA indicator strain is the same strain used for OMV production and has repeatedly been shown to provide the highest hSBA results and responses compared to other PorA P1.4 strains due to homologous minor OMV proteins [Citation24,Citation25,Citation55,Citation81]. The NZ 98/254 subfamily B FHbp peptide is recognized by 4CMenB-elicited antibody [Citation30,Citation44], and the strain expresses FHbp above the MATS PBT [Citation82,Citation83]. The PorA indicator strain also expresses a vaccine-homologous NHBA peptide above the MATS PBT [Citation82,Citation83], and pre-clinical work showed that the NHBA accessory protein induced SBA against NZ 98/254 [Citation30]. The collective ability of the recombinant antigens to induce hSBA against the NZ 98/254 strain was demonstrated in phase 1 and 2 studies with a recombinant vaccine formulation without OMVs [Citation58,Citation84].
The 44/76-SL FHbp indicator strain also measures hSBA against components other than FHbp. 44/76-SL expresses NHBA above the MATS PBT [Citation82], and the 4CMenB OMV component has been demonstrated to induce hSBA against this strain via minor OMV proteins [Citation59]. Furthermore, 44/76-SL (FHbp) and 5/99 (NadA) indicator strains express the relevant protein at levels considerably higher than reported for the majority of other MenB strains with the same FHbp/NadA peptide [Citation24,Citation25,Citation58,Citation84]. This may be an explanation for why these strains give higher hSBA responses following 4CMenB than other strains with identical and near identical FHbp or NadA peptides but with lower expression [Citation25,Citation58,Citation84]. Interpretation of the indicator strain serology must be made carefully especially when attempting to apply data to diverse strains, which may have different protein peptides and/or surface expression levels. This is because lower protein expression and increasing sequence divergence from the peptide used as a vaccine antigen are likely to correspond with lower responses as seen in clinical studies [Citation25,Citation58,Citation84]. Another issue is that although hSBA responses are primarily against a single antigen, responses are also against other components and thus may act synergistically. This is, however, how a multi-valent vaccine is expected to confer protection in vivo when an invading meningococcus is matched for more than one antigen.
6. Evaluation of MenB-FHbp immunogenicity and breadth of coverage
Determination of the immune response to MenB-FHbp has relied upon four primary and 10 additional MenB strains in the hSBA assay collectively representing the sequence diversity of FHbp () [Citation85]. Selection of these strains was informed by investigations into FHbp sequence diversity and expression across a representative collection of 1814 MenB strains from the U.S.A. and Europe [Citation86]. Phenotypic FHbp expression data were generated using the Meningococcal Antigen Surface Expression (MEASURE) assay which determines surface expression irrespective of the peptide via the use of a cross-reactive monoclonal antibody [Citation36].
Table 4. Meningococcal serogroup B strains used throughout the clinical development of MenB-FHbp
The four primary test strains were selected following a stringent step-wise approach applying set criteria in order to eliminate bias: (1) isolates harboring vaccine-homologous FHbp peptides (A05 (ID45) and B01 (ID55)) were excluded; (2) isolates with very low and very high FHbp surface expression were excluded; (3) isolates that were not compatible with the hSBA assay were excluded; (4) isolates that did not demonstrate low proportions of subjects with pre-vaccination SBA activity (i.e. baseline seropositivity) were excluded; and (5) selection of the final four strains was randomly undertaken from the remaining isolates. Exceptions were the inclusion of strains expressing A22 (ID19) and B24 (ID1) FHbp peptides as indicated by regulatory agencies due to their prevalence in MenB disease isolates in the U.S.A. and European Union [Citation34,Citation86]. The four selected primary strains included two expressing subfamily A and two expressing subfamily B FHbp peptides () and were used throughout the clinical development of MenB-FHbp to generate breadth of coverage information.
In selected studies, 10 additional strains were used in the hSBA assay to further demonstrate breadth of coverage and confirm the responses obtained with the primary strains. These strains were predictive of other strains expressing diverse FHbp peptides. Their selection was identical to that for primary strains with the following modifications: (1) isolates with the same FHbp peptide as the primary strains were excluded; (2) only strains with FHbp peptides prevalent among disease causing isolates in the U.S.A and Europe and which were of the predominant clonal complex for that FHbp peptide (if a predominant clonal complex existed) were included [Citation87]. The 10 selected additional strains included six that expressed subfamily A and four that expressed subfamily B FHbp peptides [Citation86]. At the time of selection, the FHbp peptides of these 14 strains collectively accounted for approximately 80% of all invasive MenB isolates in the U.S.A. and Europe. Furthermore, all 10 of the most common FHbp peptides among MenB disease isolates from the U.S.A. and Europe are expressed by one of these 14 strains [Citation34,Citation35]. Recent epidemiological studies from across the globe have confirmed the representative nature of these FHbp peptides selected for assessing the breadth of coverage of MenB-FHbp [Citation88–92].
The hSBA results against the 14 epidemiologically relevant diverse strains have confirmed the ability of MenB-FHbp to induce cross-reactive antibodies regardless of the FHbp peptide expressed by the test strains. This has been demonstrated at both the individual and population level [Citation86]. The use of strains with low-medium in vitro FHbp expression means that SBA responses are representative, with the exception of isolates with insufficient FHbp surface expression. The MEASURE assay has therefore been used to investigate the proportions of isolates with FHbp surface expression above a predictive threshold that correlates with hSBA killing [Citation38]. This predictive threshold corresponds to a 91.2% probability that MenB-FHbp immunization will induce a consistent ≥4-fold rise in hSBA titer from pre- to post-series completion. This approach was intentionally conservative as hSBA killing was considered using ≥4-fold rises in hSBA titer and not a titer threshold such as ≥1:4 or 1:8.
In a globally representative collection of MenB isolates, >91% were shown to express FHbp on the surface above this threshold indicating their likely susceptibility to MenB-FHbp–induced antibody. More recently, country-specific predictions have supported this figure, with 91% of Canadian MenB isolates (largely clonal complex [cc] 269 or cc41/44) recovered between 2010 and 2012 expressing FHbp above the predictive threshold, although there were differences observed between geographic regions where these strains were not dominant [Citation89]. The most contemporaneous report is for Greek MenB isolates recovered between 2010 and 2017 where 96% (cc32, cc269, cc41/44, cc213, and cc35) expressed FHbp above the predictive threshold [Citation92]. Further consideration must also be given to the conservative nature of the MEASURE predictive threshold as a subset of isolates with FHbp expression below this level can also be killed in the hSBA assay by MenB-FHbp–immune sera [Citation38].
Genetic schemes for the prediction of MenB-FHbp coverage of isolates have not been developed by the manufacturer and an interim scheme on the PubMLST website has now been superseded by the MenDeVAR index [Citation77]. MenDeVAR coverage is presumed for MenB-FHbp if the FHbp peptide of an isolate is homologous to a MenB-FHbp antigen, expressed by a strain killed in the SBA assay in published literature, or has been shown to be expressed above the MEASURE predictive threshold for ≥75% of previously assayed isolates. The utility of the MenDeVAR index remains to be determined especially as the scheme only currently classifies a limited number of FHbp peptides for MenB-FHbp coverage. This is in contrast to the collective interpretation of SBA data against four primary strains, the 10 additional strains, as well as MenB outbreak strains, demonstrating the ability of MenB-FHbp to provide protection against the full diversity of FHbp [Citation93].
6.1. Additional MenB breadth of coverage data
Most reported hSBA immunogenicity data have been generated with indicator strains for 4CMenB and the 14 primary and additional strains for MenB-FHbp, as these were agreed-upon licensure pathways with regulatory agencies. There is, however, an increasing volume of additional hSBA assay data that can be used to inform our understanding of the vaccines’ breadth of coverage. The earliest hSBA data are those from two phase 2 studies of 4CMenB that used four UK MenB strains in addition to the indicator strains [Citation24,Citation25]. Responses to a UK strain expressing a P1.4 PorA were lower than those against the NZ 98/254 indicator strain, confirming earlier observations that the highest responses are seen against the vaccine strain [Citation55]. Another important observation was found using the UK strain M01 240364 which has an identical NadA to the 5/99 indicator strain except for a difference in the leader peptide and, importantly, expressing a ‘high’ compared to ‘very high’ level of NadA. While sera from infants following three 4CMenB doses had strong SBA responses in assays using the 5/99 NadA indicator strain, there was almost no response in assays performed with the alternative UK strain even though it expressed NadA at significant levels [Citation25]. Functional responses to this UK strain were only detected using sera collected following the fourth booster dose at 12 months of age, highlighting the age-related responses [Citation25]. Further evaluation of the responses against the four UK strains used in these phase 2 studies indicate that despite protein expression, limited responses were induced against a strain with an FHbp belonging to the same subfamily as the vaccine antigen (B44 (ID15)) and various NHBA peptides [Citation24,Citation25].
Vaccine responses to MenB strains that have been responsible for localized outbreaks have also been determined for both vaccines. Taha and colleagues reported that MenB-FHbp induced SBA in adolescents against six French outbreak strains expressing five different FHbp peptides [Citation63]. Lujan and colleagues reported that following vaccination of healthcare workers and microbiologists with MenB-FHbp, between 73% and 100% achieved ≥4-fold rises in hSBA titer against 14 MenB strains, including six from US university outbreaks and two from Canadian disease outbreaks [Citation64]. In an almost identical study, 70–95% of 4CMenB-vaccinated adults achieved ≥4-fold rises in hSBA titer against the three indicator strains, but only 20–45% achieved ≥4-fold rises for 10 of the 15 strains tested [Citation46]. Greater responses were detected against the three strains that expressed a vaccine-homologous FHbp, although these were lower than the responses reported for the FHbp indicator strain. The study evaluated the same three B15 (ID252) strains used by Lujan and colleagues, which, despite expressing FHbp, were not killed by 4CMenB immune sera [Citation46,Citation64]. Basta and colleagues investigated hSBA titers in US university students who were vaccinated with 4CMenB in an outbreak response [Citation73]. Although no cases of MenB disease occurred among vaccinated students, 87% and 100% of students had hSBA titers ≥1:4 against the FHbp and NadA indicator strains, respectively, whereas only 66% had hSBA titers ≥1:4 against the outbreak strain that expressed both FHbp and NHBA above the MATS PBT [Citation73,Citation94]. A follow-up observational study taking pre- and post-4CMenB samples undertaken at the same university further confirmed the discrepancy in responses between the outbreak and indicator strains. Fold rises in hSBA geometric mean titer (GMT) of 22.8 and 227.9 were reported for the FHbp and NadA indicator strains, but only a 2.4-fold rise was gained against the outbreak strain [Citation95]. These hSBA data from non-indicator strains highlight that breadth of coverage can be specific to the vaccinated individual’s response. This is due to differing individual immune responses and how cross-reactive and functional induced antibodies are. This confirms that a binary assessment of a strain being covered or not covered by a vaccine is often an oversimplification.
A recent study reported the ability of 4CMenB to provide protection in adolescents against 14 MenB outbreak strains [Citation45]. This study did not report ≥4-fold rises in hSBA titer but showed that for 11/14 strains, ≥50% of subjects had hSBA titers ≥1:4 following vaccination. However, seven of these 11 strains expressed the FHbp peptide homologous to the vaccine antigen and two of the others had a vaccine homologous P1.4 PorA or harbored NadA. This study confirmed earlier findings by Giuntini and colleagues of the inability of 4CMenB immune sera to provide coverage against the B15 (ID252) FHbp peptide even when expressed by the test strain [Citation46]. By way of comparison, MenB-FHbp has been shown to induce a cross-reactive response against the B15 (ID252) FHbp peptide, expressed by one of the additional strains used in SBA assays during clinical testing [Citation12]. The reason for the discrepancy in the ability of the two vaccines to kill strains expressing this FHbp peptide is not clearly understood but a contributing factor may be the lipidation of MenB-FHbp antigen, which has been associated with increased immunogenicity [Citation32–34].
Breadth of coverage information following infant priming and toddler boosting with 4CMenB can be gained from the study by Biolchi and colleagues comparing two- and three-dose priming series [Citation96]. A pooled sample following each series resulted in hSBA titers ≥1:4 against 40% and 87.5% of a panel of 40 UK MenB strains following the priming series and the booster, respectively. These data confirm the age-related immunogenicity profile of 4CMenB and are aligned with the earlier individual subject data against the subset of UK strains used in initial phase 2 studies [Citation24,Citation25].
To date, there have not been head-to-head studies comparing the immunogenicity and breadth of coverage of 4CMenB and MenB-FHbp. The studies of Lujan et al. and Giuntini et al. were undertaken in the same laboratory using the same eight US and Canadian outbreak strains but the samples came from independent studies of a relatively small size [Citation46,Citation64]. Another study investigated the hSBA responses in adolescents and adults receiving 4CMenB but against the four primary strains used for MenB-FHbp analysis [Citation97,Citation98]. Following completion of a 2 dose 4CMenB series given at 0 and 2 months, the greatest response was seen against the B24 (ID1) FHbp strain that expressed the 4CMenB vaccine-homologous FHbp and a group I NadA with 91.8% of subjects achieving ≥4-fold rises in hSBA titer (). Similarly, 73% of subjects achieved a ≥4-fold rise against the A22 (ID19) FHbp strain, which is the same clonal complex as the OMV vaccine strain. However, only 37.3% of subjects achieved a ≥4-fold rise for the B44 (ID15) FHbp strain, matching the results from an infant phase 2 study that also showed low responses against a strain expressing the B44 (ID15) FHbp [Citation25]. For the A56 (ID187) FHbp strain, 37.5% of subjects achieved a ≥4-fold rise, likely due to non-FHbp responses from either the NHBA or minor-OMV proteins.
Table 5. Human serum bactericidal antibody assay titers 1 month following two doses of 4CMenB on a 0–2-month schedule in adolescents 12–18 years of age against primary meningococcal serogroup B strains used throughout the MenB-FHbp clinical program [Citation97,Citation98]
6.2. Additional breadth of coverage data for other meningococcal capsular groups
The surface proteins included as antigens in the 4CMenB and MenB-FHbp vaccines are meningococcal proteins that induce SBA and are able to provide protection irrespective of serogroup [Citation45,Citation61]. This potential has now been demonstrated for both vaccines. Studies using 147 European and Brazilian meningococcal serogroups C, W, and Y (MenC, MenW, and MenY) isolates showed that pooled serum samples taken post-4CMenB series completion in adolescents and toddlers gave hSBA titers ≥1:4 against 61.9% and 74.1% of isolates, respectively [Citation99,Citation100]. Another study utilizing Italian isolates showed that pooled post-4CMenB sera from adolescents and toddlers had hSBA titers ≥1:4 against nine MenW, meningococcal serogroup X (MenX), and MenY isolates [Citation100]. This was in contrast to 11 MenC isolates where the adolescent pool achieved hSBA titers ≥1:4 against five isolates and the toddler pool demonstrated hSBA titers ≥1:2 against two isolates [Citation100]. Specific investigations have also been undertaken with post-4CMenB sera and its ability to kill MenW cc11 isolates that express a NadA class I peptide. The first of these showed the ability of four serum pools generated from toddler and young children post-4CMenB booster samples to kill six UK MenW cc11 isolates [Citation101]. A more recent study investigated 20 individual samples taken from adolescents post-4CMenB and 19 had hSBA titers ≥1:4 against one of the UK MenW cc11 isolates used in the previous study [Citation102]. Similar data have been reported for MenB-FHbp where hSBA responses were shown to MenC, MenW, MenY, and MenX isolates expressing five different FHbp peptides [Citation61]. Collective interpretation of non-MenB data and the breadth of coverage provided by the respective MenB vaccines must be undertaken with the same considerations as those used when interpreting MenB serological data. For example, the data showing the ability of 4CMenB immune sera to kill a UK MenW strain may not be transferable to other MenW strains that do not harbor the same NadA peptide or express the protein to the same extent.
6.3. Real-world data and breadth of protection
In unison with previous capsular polysaccharide-containing meningococcal vaccines, the surface protein vaccines were licensed using the hSBA ≥1:4 correlate or ≥4-fold increase in SBA titers in the absence of proven efficacy or effectiveness [Citation103]. Ratification of this approach has been previously achieved for polysaccharide-containing and OMV vaccines, confirming the SBA assay as the appropriate surrogate or correlate of protection [Citation74]. Vaccine impact and effectiveness data for surface protein vaccines are now being generated that confirm the appropriateness of the SBA surrogate for these recombinant meningococcal protein antigens. Early information came from the use of 4CMenB and MenB-FHbp at different US universities in response to MenB disease outbreaks where no cases occurred in vaccinated individuals after sufficient time for immune responses to develop, but did occur in those who were unvaccinated [Citation104].
In 2014, in the Saguenay-Lac-Saint-Jean region of Quebec, Canada, a short-term mass vaccination campaign with 4CMenB was conducted for participants between 2 months and 20 years of age [Citation26]. This was in response to a clonal ST-269 outbreak of MenB disease. While interpretation of the data is complicated by significant declines in MenB cases in surrounding regions not using the vaccine, the low number of cases, and the clonality of this outbreak, 4CMenB introduction led to a 92% reduction in MenB cases. Vaccine effectiveness of 91% and 94% against MenB disease has been recently reported for two Italian regions using 4CMenB in infant programs, although the number of cases and the target population are relatively small [Citation27]. Perhaps the most robust data to be reported have come from national surveillance in England following 4CMenB introduction in 2015. During the first 3 years of the program there was a 75% reduction in MenB cases and a vaccine effectiveness of 52.7% and 59.1% following the two infant priming doses and two priming doses plus toddler booster, respectively [Citation28]. The immunization program was also reported to have a vaccine effectiveness of 34% and 88% against MenW following 4CMenB priming and booster doses, respectively [Citation62].
The real-world data reaffirm that SBA is predictive of protection for meningococcal surface protein vaccines and that protection will be afforded against MenB and non-MenB capsular groups. However, these real-world data highlight the importance of breadth of coverage and why we need to better understand and predict such coverage. The 4CMenB vaccine effectiveness data from England against MenB disease show a higher effectiveness following the toddler booster and may be explained by the age-related immunogenicity demonstrated in the few SBA studies providing breadth of coverage information in this age group [Citation25,Citation96]. The effectiveness against MenW was similarly age-related and is probably explained by the expression of NadA by the MenW cc11 clone and the age-related anti-NadA response previously shown [Citation25,Citation62]. The MenW effectiveness data may therefore not be transferable to other localities where MenW disease is not caused by the MenW cc11-expressing NadA [Citation62]. The same applies to MenB where both breadth of coverage and vaccine effectiveness can be location specific.
6.4. Antibody persistence and meningococcal carriage
Studies of monovalent MenC conjugate vaccines highlighted the critical importance of maintaining protective levels of anti-capsular SBA in meningococcal disease protection even when immunological memory had been imparted by immunization [Citation105]. This is explained by the rapid progression of invasive meningococcal infections outpacing the kinetics of immunological memory in the absence of protective SBA levels. Although there is currently no information demonstrating the same requirement for maintenance of protective SBA levels for meningococcal surface protein antigens, it is likely to be the same. The immune response and associated benefit of maintaining protective SBA levels in response to natural exposure through carriage could be different between conjugate capsular and surface protein vaccinees. As an example, MenC strains are infrequently carried, when carried they do not all express capsule or express less capsule than other serogroups, and carriage rates are further reduced where MenC programs have achieved indirect or herd protection [Citation106]. In contrast, FHbp is harbored and often expressed in meningococcal strains irrespective of serogroup and even by unencapsulated meningococci [Citation107–109]. The potential of meningococcal acquisition and carriage as events able to trigger immunological memory imparted by surface protein vaccines may be worthy of investigation.
The persistence of the breadth of coverage provided by meningococcal surface protein vaccines is equally important as the titers achieved immediately following series completion. There is currently no evidence to suggest that the ability of antibody to cross-react to heterologous and homologous peptides is different as the antibody population wanes or following a booster dose. There is the potential that memory B-cell maturation, which occurs over time, may increase antibody-cross reactivity, but this remains to be determined. Antibody persistence and boosting studies for 4CMenB have maintained the use of SBA indicator strains with interpretation of data relying on MATS coverage estimates, which in turn are linked to immediately post-vaccination antibody cross-reactivity [Citation78–80,Citation110–112]. Use of the indicator strains at these persistence time points also fails to elucidate the impact of lower protein expression on the bactericidal activity as antibody wanes. Investigation of MenB-FHbp antibody persistence and the demonstration of anamnestic booster responses was evaluated using the primary SBA strains, providing information of antibody-cross reactivity and breadth of coverage at persistence time points [Citation113]. Use of the primary SBA strains that express vaccine-heterologous FHbp peptides does not provide an opportunity to identify whether the waning of antibodies recognizing homologous and heterologous proteins following MenB-FHbp is the same.
The benefit of indirect protection achieved through carriage interruption has been a significant advantage of meningococcal conjugate vaccines and has led to implementation of programs where this is a specific objective [Citation114]. The ability of surface protein vaccines to impact asymptomatic carriage has been investigated in multiple studies. A large study of 4CMenB in Australian adolescents concluded that the vaccine was unlikely to impart herd protection as there was no impact on meningococcal carriage acquisition or carriage density [Citation115,Citation116]. The use of two FHbp variants that are lipidated in MenB-FHbp means that results from the Australian 4CMenB study are not necessarily transferable to MenB-FHbp. The only data for MenB-FHbp are associated with two small, opportunistic studies in the setting of college campus outbreaks in the U.S.A. These studies did not identify any impact on carriage but interpretation is hindered by the very limited number of participants that had completed the full dosing series and the timing of sampling [Citation117,Citation118]. A study comparing the impact of 4CMenB and MenB-FHbp in UK adolescents has been interrupted by the COVID-19 pandemic potentially rendering data interpretation compromised [Citation119].
Historical meningococcal epidemiology highlights that there are natural cyclic changes in circulating strains and clones causing disease interspersed with outbreaks and hyperendemic periods [Citation1]. This can impact the breadth of coverage afforded by individual surface protein antigens and vaccine formulations as variation can occur in gene presence, peptide variant, and expression level. Vaccine pressure from surface protein vaccines could accelerate such changes, but this has not been observed for other meningococcal capsular-based or OMV vaccines. Continued surveillance of meningococcal epidemiology and breakthrough cases occurring following surface protein vaccine use are vital to identify any changes that could impact potential breadth of coverage.
7. Conclusion
The concept of the breadth of coverage for meningococcal surface protein vaccines is unique to these vaccines and has never needed consideration for meningococcal capsular-based vaccines. This is due to the variation in presence, sequence, and/or expression of the surface proteins in disease-causing strains and the requirement for the induction of functional cross-reactive immune responses against diverse variants. As the two currently licensed surface protein vaccines contain different antigens with different presentations, the breadth of protection each will provide varies. The methodologies for assessing breadth of coverage and the strains used can significantly impact both the results and the interpretation of these results in terms of perceived breadth of coverage against diverse disease-causing MenB strains. This applies equally to the vaccine impact and effectiveness data as well as the immunological assays. Key factors and components that independently and collectively influence the breadth of coverage assessments of surface protein vaccines are summarized in . Collective assessment of these factors is crucial to understanding how to interpret and assess the breadth of coverage of current and future surface protein vaccines.
Table 6. Key factors influencing laboratory assays assessing the breadth of coverage of meningococcal surface protein vaccines
8. Expert opinion
Meningococcal surface protein vaccines are licensed to provide broad coverage against diverse MenB disease-causing strains. An understanding of the proportion of strains that are covered by these vaccines as well as those that are not are equally important. The best information that estimates coverage is gained from the immunological testing in hSBA assays (the accepted surrogate of protection), and from real-world data using these vaccines, or a combination of the two.
Due to differences in the clone(s) of local disease-causing strains, the breadth of coverage afforded by a vaccine could vary depending on geographical location. For example, the breadth of coverage in a region where disease is predominantly due to an ongoing outbreak or hyperendemic disease caused by one or a limited number of meningococcal clones could be very different compared to regions with diverse endemic disease. Importantly, meningococcal epidemiology is continually changing and requires ongoing surveillance and isolate characterization, which may lead to changes in the breadth of coverage provided by specific MenB vaccines. Breadth of coverage predictions must also be carefully interpreted in terms of relevance and transferability. Differences in immunogenicity and cross-reactivity of induced bactericidal antibody due to subject age and between individuals of the same age also must be considered. The representativeness of the strains used in SBA assessments also requires careful consideration and has limitations. For hSBA immunogenicity studies, it will never be possible to test isolates from all cases as there is often a significant proportion of cases where an isolate is not recovered. Selection of strains always has the possibility of introducing bias, even when unintentional, and can result in the final selection being unrepresentative. Studies using multiple replicates of strains that express the same surface proteins at similar levels as opposed to diverse strains can give a false representation of coverage. Similarly, studies with large numbers of strains do not necessarily provide more robust data, especially when these strains do not capture the full known diversity.
With hSBA studies there are many methodological caveats that must be collectively considered to allow the interpretation of results as discussed in the companion manuscript [Citation8]. In some instances we have also seen a loss of hSBA assay sensitivity by using a lower limit of quantification (LLOQ) of 1:8 or 1:16, which is above the accepted correlate of protection [Citation10]. The use of these LLOQs is inherently conservative and has the potential to underestimate vaccine immunogenicity and efficacy. Calculating proportions with hSBA titer ≥1:8 or 1:16 also impacts the proportion of subjects calculated as achieving ≥4-fold rises. Choosing SBA strains that have low rates of baseline seropositivity is likely another way in which assay sensitivity may be lost. These aspects must be collectively compared against the longstanding knowledge that while hSBA titers ≥1:4 are predictive of protection from disease, hSBA titers <1:4 are not predictive of susceptibility [Citation120].
Vaccine immunogenicity and breadth of coverage must be holistically evaluated, and studies only reporting the proportion of subjects with an hSBA titer cutoff ≥1:4/LLOQ may not provide a full understanding. This is especially true where there is baseline seropositivity, as these studies fail to elucidate the vaccine response that could otherwise be achieved by reporting fold rises or GMTs that account for baseline SBA levels. The use of a ≥4-fold rise in hSBA titer was originally proposed as a way of confirming vaccine response and is a more conservative measure than applying an hSBA titer cutoff of ≥1:4/LLOQ [Citation6,Citation121]. This is because a sample with a seropositive post-vaccination hSBA titer of 1:4 could have been 1:2 pre-vaccination, only a single titer below. The impact of assay variation must also be considered when such a fine margin is used to signify a vaccine response.
Different genetic schemes have been developed to help predict breadth of coverage, but their utility and accuracy remain uncertain. These schemes provide a binary prediction that may or may not be correct and do not account for differences in immune responses between individuals and age groups. In contrast, there is increasing data confirming that immune coverage of surface protein vaccines will extend to other meningococcal serogroups, an added benefit to the use of these vaccines [Citation62,Citation93,Citation99–102]. Current surface protein vaccines will not be a direct replacement of meningococcal serogroups A, C, W, and Y (MenACWY) vaccines and explains the development of pentavalent meningococcal vaccines where surface protein vaccines are being combined with MenACWY conjugate vaccines [Citation122,Citation123]. Surface protein vaccines may also be useful in situations such as the recent emergence of unencapsulated meningococcal strains causing outbreaks of urogenital tract disease [Citation124].
Further data on the current surface protein vaccines will continue to emerge and so will data on new vaccines in development, including pentavalent MenABCWY formulations. Careful assessment of the breadth of coverage data is critical for an informed forecast of the utility of meningococcal protein-containing vaccines in different age groups and geographic regions.
Article highlights
In the second of two companion papers, we compare how the two currently available meningococcal surface protein vaccines, 4CMenB and MenB-FHbp, have been assessed for breadth of coverage.
The breadth of coverage afforded by meningococcal surface protein vaccines is driven by (1) the choice of protein antigens included in the vaccines and the ability to induce cross-reactive immune responses to diverse protein variants – this immune response can vary between individuals and be age-related; and (2) the susceptibility of circulating invasive MenB and other meningococcal strains from a geographic location – do these strains sufficiently express the protein(s) recognized by vaccine induced antibodies.
Predicting the breadth of coverage is also influenced by the methodologic differences in the immune correlate of protection, which is the SBA assay using human complement (hSBA). Genotypic methods of predicting coverage are less reliable.
Different approaches have been undertaken for the immunological assessment and prediction of breadth of coverage for 4CMenB and MenB-FHbp.
4CMenB assessment has primarily used four MenB indicator strains in the hSBA assay to independently measure responses against one of the four antigens. Responses against these ‘matched’ indicator strains do not provide information on cross-protection to diverse strains with different protein variants or lower expression levels. Coverage of 4CMenB against MenB isolates has therefore been predicted using the Meningococcal Antigen Typing System (MATS) which provides a binary ‘covered’ or ‘not covered’ outcome but which does not account for differences in responses between individuals or different age groups.
Assessment of MenB-FHbp has primarily been undertaken in the hSBA assay using four primary and 10 additional MenB strains. All of these strains expressed a unique heterologous FHbp to those contained within the vaccine and all expressed the FHbp protein at low-medium levels. Responses against these 14 MenB strains was intended to provide breadth of coverage information as the FHbp variants and expression levels were representative of global diversity.
Real-world effectiveness data are confirming that the meningococcal protein-based vaccines, licensed on the basis of hSBA, can prevent meningococcal disease caused by MenB and other serogroups; but have also demonstrated that meningococcal surface protein vaccines do not provide ubiquitous coverage. This evidence further emphasizes the importance of understanding factors influencing coverage and longitudinally assessing breadth of coverage.
List of abbreviations
Author contribution
All authors participated equally and substantially in manuscript development, including interpretation of literature, writing, revision, and intellectual contribution.
Declarations of Interest
J Findlow, P Liberator, AS Anderson, P Balmer and L Jodar are employees of Pfizer Inc and may hold stock or stock options. R Borrow performs contract research on behalf of Public Health England for GSK, Pfizer, and Sanofi Pasteur. DS Stephens performs research on N. meningitidis funded by the US National Institute of Allergy and Infectious Diseases of the National Institutes of Health. The authors have no other relevant affiliations or financial involvement with any organization or entity with a financial interest in or financial conflict with the subject matter or materials discussed in the manuscript.
Reviewer disclosures
Peer reviewers on this manuscript have no relevant financial or other relationships to disclose.
Acknowledgments
This publication made use of the PubMLST website available at https://pubmlst.org [42]. We would also like to thank the participants of study B1971048, the coordinating investigator Professor Lars Østergaard, and the research team.
Additional information
Funding
References
- Parikh SR, Campbell H, Bettinger JA, et al. The everchanging epidemiology of meningococcal disease worldwide and the potential for prevention through vaccination. J Infect. 2020;81(4):483–498.
- Purmohamad A, Abasi E, Azimi T, et al. Global estimate of Neisseria meningitidis serogroups proportion in invasive meningococcal disease: a systematic review and meta-analysis. Microb Pathog. 2019; 134:103571.
- Tan LK, Carlone GM, Borrow R. Advances in the development of vaccines against Neisseria meningitidis. N Engl J Med. 2010;362(16):1511–1520.
- Rosenstein NE, Perkins BA, Stephens DS, et al. Meningococcal disease. N Engl J Med. 2001;344(18):1378–1388.
- Cohn AC, MacNeil JR, Clark TA, et al. Prevention and control of meningococcal disease: recommendations of the Advisory Committee on Immunization Practices (ACIP). MMWR Recomm Rep. 2013;62(RR–2):1–28.
- Bexsero (MenB-4C). GlaxoSmithKline Vaccines srl. Siena, Italy: 2018.
- Chen WH, Neuzil KM, Boyce CR, et al. Safety and immunogenicity of a pentavalent meningococcal conjugate vaccine containing serogroups A, C, Y, W, and X in healthy adults: a phase 1, single-centre, double-blind, randomised, controlled study. Lancet Infect Dis. 2018;18(10):1088–1096.
- Findlow J, Lucidarme J, Taha M-K, et al. Correlates of protection for meningococcal surface protein vaccines: lessons from the past. Expert Rev Vaccines. 2021;1–13. doi:https://doi.org/10.1080/14760584.2021.1940144.
- Stephens DS. Biology and pathogenesis of the evolutionarily successful, obligate human bacterium Neisseria meningitidis. Vaccine. 2009;27(suppl 2):B71–7.
- Borrow R, Carlone GM, Rosenstein N, et al. Neisseria meningitidis group B correlates of protection and assay standardization–international meeting report Emory University, Atlanta, Georgia, United States, 16-17 March 2005. Vaccine. 2006;24(24):5093–5107.
- Finne J, Leinonen M, Makela PH. Antigenic similarities between brain components and bacteria causing meningitis. Implications for vaccine development and pathogenesis. Lancet. 1983;2(8346):355–357.
- Findlow J, Bayliss CD, Beernink PT, et al. Broad vaccine protection against Neisseria meningitidis using factor H binding protein. Vaccine. 2020;38(49):7716–7727.
- Sierra GV, Campa HC, Varcacel NM, et al. Vaccine against group B Neisseria meningitidis: protection trial and mass vaccination results in Cuba. NIPH Ann. 1991;14(2):195–207. Discussion 208-10.
- Bjune G, Høiby EA, Grønnesby JK, et al. Effect of outer membrane vesicle vaccine against group B meningococcal disease in Norway. Lancet. 1991;338(8775):1093–1096.
- Holst J, Oster P, Arnold R, et al. Vaccines against meningococcal serogroup B disease containing outer membrane vesicles (OMV): lessons from past programs and implications for the future. Hum Vaccin Immunother. 2013;9(6):1241–1253.
- Wang NY, Pollard AJ. The next chapter for group B meningococcal vaccines. Crit Rev Microbiol. 2018;44(1):95–111.
- Holst J, Feiring B, Fuglesang JE, et al. Serum bactericidal activity correlates with the vaccine efficacy of outer membrane vesicle vaccines against Neisseria meningitidis serogroup B disease. Vaccine. 2003;21(7–8):734–737.
- Donald RG, Hawkins JC, Hao L, et al. Meningococcal serogroup B vaccines: estimating breadth of coverage. Hum Vaccin Immunother. 2017;13(2):255–265.
- Boutriau D, Poolman J, Borrow R, et al. Immunogenicity and safety of three doses of a bivalent (B:4:p1.19,15 and B:4:p1.7-2,4) meningococcal outer membrane vesicle vaccine in healthy adolescents. Clin Vaccin Immunol. 2007;14(1):65–73.
- de Kleijn ED, de Groot R, Labadie J, et al. Immunogenicity and safety of a hexavalent meningococcal outer-membrane-vesicle vaccine in children of 2-3 and 7-8 years of age. Vaccine. 2000;18(15):1456–1466.
- Kaaijk P, van Straaten I, van de Waterbeemd B, et al. Preclinical safety and immunogenicity evaluation of a nonavalent PorA native outer membrane vesicle vaccine against serogroup B meningococcal disease. Vaccine. 2013;31(7):1065–1071.
- Rots NY, Kleijne DE. Safety of a nonavalent meningococcal serogroup B vaccine in healthy adult volunteers in a randomised, controlled, single blind study. In 16th International Pathogenic Neisseria Conference. 2008. Rotterdam, Netherlands.
- US Food and Drug Administration. Summary Basis for Regulatory Action. 2014 October 29. [cited 2018 Oct 11]; Available from: https://wayback.archive-it.org/7993/20190425012126/https://www.fda.gov/downloads/BiologicsBloodVaccines/Vaccines/ApprovedProducts/UCM424125.pdf.
- Snape MD, Dawson T, Oster P, et al. Immunogenicity of two investigational serogroup B meningococcal vaccines in the first year of life: a randomized comparative trial. Pediatr Infect Dis J. 2010;29(11):71–79.
- Findlow J, Borrow R, Snape MD, et al. Multicenter, open-label, randomized phase II controlled trial of an investigational recombinant meningococcal serogroup B vaccine with and without outer membrane vesicles, administered in infancy. Clin Infect Dis. 2010;51(10):1127–1137.
- De Wals P, Deceuninck G, Lefebvre B, et al. Impact of an immunization campaign to control an increased incidence of serogroup B meningococcal disease in one region of Quebec, Canada. Clin Infect Dis. 2017;64(9):1263–1267.
- Azzari C, Moriondo M, Nieddu F, et al. Effectiveness and impact of the 4CMenB vaccine against group B meningococcal disease in two Italian regions using different vaccination schedules: a five-year retrospective observational study (2014-2018). Vaccines (Basel). 2020;8(3):469.
- Ladhani SN, Andrews N, Parikh SR, et al. Vaccination of infants with meningococcal group B vaccine (4CMenB) in England. N Engl J Med. 2020;382(4):309–317.
- Bexsero®. Summary of Product Characteristics. GlaxoSmithKline, in 4CMenB. 2019.
- Giuliani MM, Adu-Bobie J, Comanducci M, et al. A universal vaccine for serogroup B meningococcus. Proc Natl Acad Sci U S A. 2006;103(29):10834–10839.
- European Medicines Agency. Bexsero EPAR summary for the public. 2012. [cited 2017 Mar 27]; Available from: http://www.ema.europa.eu/docs/en_GB/document_library/EPAR_-_Summary_for_the_public/human/002333/WC500137857.pdf.
- Fletcher LD, Bernfield L, Barniak V, et al. Vaccine potential of the Neisseria meningitidis 2086 lipoprotein. Infect Immun. 2004;72(4):2088–2100.
- Luo Y, Friese OV, Runnels HA, et al, The dual role of lipids of the lipoproteins in Trumenba, a self-adjuvanting vaccine against meningococcal meningitis B disease. AAPS J. 2016;18(6):1562–1575.
- Zlotnick GW, Jones TR, Liberator P, et al. The discovery and development of a novel vaccine to protect against Neisseria meningitidis serogroup B disease. Hum Vaccin Immunother. 2015;11(1):5–13.
- Murphy E, Andrew L, Lee K-L, et al. Sequence diversity of the factor H binding protein vaccine candidate in epidemiologically relevant strains of serogroup B Neisseria meningitidis. J Infect Dis. 2009;200(3):379–389.
- Jiang HQ, Hoiseth SK, Harris SL, et al. Broad vaccine coverage predicted for a bivalent recombinant factor H binding protein based vaccine to prevent serogroup B meningococcal disease. Vaccine. 2010;28(37):6086–6093.
- Vogel U, Taha M-K, Vazquez JA, et al. Predicted strain coverage of a meningococcal multicomponent vaccine (4CMenB) in Europe: a qualitative and quantitative assessment. Lancet Infect Dis. 2013;13(5):416–425.
- McNeil LK, Donald RGK, Gribenko A, et al. Predicting the susceptibility of meningococcal serogroup B isolates to bactericidal antibodies elicited by bivalent rLP2086, a novel prophylactic vaccine. MBio. 2018;9(2):e00036–18.
- Seib KL, Serruto D, Oriente F, et al. Factor H-binding protein is important for meningococcal survival in human whole blood and serum and in the presence of the antimicrobial peptide LL-37. Infect Immun. 2009;77(1):292–299.
- Madico G, Welsch JA, Lewis LA, et al. The meningococcal vaccine candidate GNA1870 binds the complement regulatory protein factor H and enhances serum resistance. J Immunol. 2006;177(1):501–510.
- Neisseria factor H binding protein sequence typing. 2020. [cited 2020 Oct 12]; Available from: https://pubmlst.org/neisseria/fHbp/.
- Jolley KA, Bray JE, Maiden MCJ. Open-access bacterial population genomics: bIGSdb software, the PubMLST.org website and their applications. Wellcome Open Res. 2018;3:124. https://wellcomeopenresearch.org/
- Masignani V, Comanducci M, Giuliani MM, et al. Vaccination against Neisseria meningitidis using three variants of the lipoprotein GNA1870. J Exp Med. 2003;197(6):789–799.
- Brunelli B, Del Tordello E, Palumbo E, et al. Influence of sequence variability on bactericidal activity sera induced by factor H binding protein variant 1.1. Vaccine. 2011;29(5):1072–1081.
- Biolchi A, Tomei S, Santini L, et al. Four-component meningococcal serogroup B vaccine induces antibodies with bactericidal activity against diverse outbreak strains in adolescents. Pediatr Infect Dis J. 2021;40(2):e66–e71.
- Giuntini S, Lujan E, Gibani MM, et al. Serum bactericidal antibody responses of adults immunized with the MenB-4C vaccine against genetically diverse serogroup B meningococci. Clin Vaccin Immunol. 2017;24(1):e00430–16.
- NadA sequence typing. 2020. [cited 2020 Oct 12]; Available from: https://pubmlst.org/neisseria/NadA/.
- Neisserial heparin binding antigen (NHBA) protein sequence typing. 2020. [cited 2020 Oct 12]; Available from: https://pubmlst.org/neisseria/NHBA/.
- Bambini S, De Chiara M, Muzzi A, et al. Neisseria adhesin A variation and revised nomenclature scheme. Clin Vaccine Immunol. 2014;21(7):966–971.
- Liguori A, Dello Iacono L, Maruggi G, et al. NadA3 structures reveal undecad coiled coils and LOX1 binding regions competed by meningococcus B vaccine-elicited human antibodies. mBio. 2018;9(5):e01914–18.
- Partridge E, Lujan E, Giuntini S, et al. The role of anti-NHba antibody in bactericidal activity elicited by the meningococcal serogroup B vaccine, MenB-4C. Vaccine. 2017;35(33):4236–4244.
- Donnelly J, Medini D, Boccadifuoco G, et al. Qualitative and quantitative assessment of meningococcal antigens to evaluate the potential strain coverage of protein-based vaccines. Proc Natl Acad Sci U S A. 2010;107(45):19490–19495.
- Fagnocchi L, Biolchi A, Ferlicca F, et al. Transcriptional regulation of the nadA gene in Neisseria meningitidis impacts the prediction of coverage of a multicomponent meningococcal serogroup B vaccine. Infect Immun. 2013;81(2):560–569.
- Sacchi CT, Whitney AM, Popovic T, et al. Diversity and prevalence of PorA types in Neisseria meningitidis serogroup B in the United States, 1992–1998. J Infect Dis. 2000;182(4):1169–1176.
- Martin DR, Ruijne N, McCallum L, et al. The VR2 epitope on the PorA P1.7-2,4 protein is the major target for the immune response elicited by the strain-specific group B meningococcal vaccine MeNZB. Clin Vaccine Immunol. 2006;13(4):486–491.
- Ruijne N, Lea RA, O’Hallahan J, et al. Understanding the immune responses to the meningococcal strain-specific vaccine MeNZB measured in studies of infants. Clin Vaccine Immunol. 2006;13(7):797–801.
- Rajam G, Stella M, Kim E, et al. Meningococcal Antigen Typing System (MATS)-based Neisseria meningitidis serogroup B coverage prediction for the MenB-4C vaccine in the United States. mSphere. 2017;2(6):e00261-17.
- Toneatto D, Ismaili S, Ypma E, et al. The first use of an investigational multicomponent meningococcal serogroup B vaccine (4CMenB) in humans. Hum Vaccin. 2011;7(6):646–653.
- Wedege E, Bolstad K, Aase A, et al. Functional and specific antibody responses in adult volunteers in New Zealand who were given one of two different meningococcal serogroup B outer membrane vesicle vaccines. Clin Vaccin Immunol. 2007;14(7):830–838.
- Giuliani M, Bartolini E, Galli B, et al. Human protective response induced by meningococcus B vaccine is mediated by the synergy of multiple bactericidal epitopes. Sci Rep. 2018;8(1):3700.
- Harris SL, Tan C, Andrew L, et al. The bivalent factor H binding protein meningococcal serogroup B vaccine elicits bactericidal antibodies against representative non-serogroup B meningococci. Vaccine. 2018;36(45):6867–6874.
- Ladhani SN, Campbell H, Andrews N, et al. First real-world evidence of meningococcal group B vaccine, 4CMenB, protection against meningococcal group W disease; prospective enhanced national surveillance, England. Clin Infect Dis. 2021;73(7):e1661–e1668.
- Taha M-K, Hawkins JC, Liberator P, et al. Bactericidal activity of sera from adolescents vaccinated with bivalent rLP2086 against meningococcal serogroup B outbreak strains from France. Vaccine. 2017;35(11):1530–1537.
- Lujan E, Partridge E, Giuntini S, et al. Breadth and duration of meningococcal serum bactericidal activity in health care workers and microbiologists immunized with the MenB-FHbp vaccine. Clin Vaccine Immunol. 2017;24(8):e00121–17.
- Ostergaard L, Vesikari T, Absalon J, et al. A bivalent meningococcal B vaccine in adolescents and young adults. N Engl J Med. 2017;347(24):2349–2362.
- Santolaya ME, O'Ryan ML, Valenzuela MT, et al. Imunogenicity and tolerability of a multicomponent meningococcal serogroup B (4CMenB) vaccine in healthy adolescents in Chile: a phase 2b/3 randomised, observer-blind, placebo-controlled study. Lancet. 2012;379(9816):617–24.
- Pizza M, Scarlato V, Masignani V, et al. Identification of vaccine candidates against serogroup B meningococcus by whole-genome sequencing. Science. 2000;287(5459):1816–1820.
- O’Ryan M, Stoddard J, Toneatto D, et al. A multi-component meningococcal serogroup B vaccine (4CMenB): the clinical development program. Drugs. 2014;74(1):15–30.
- Masignani V, Pizza M, Moxon ER. The development of a vaccine against meningococcus B using reverse vaccinology. Front Immunol. 2019;10:751.
- Giuliani MM, Biolchi A, Serruto D, et al. Measuring antigen-specific bactericidal responses to a multicomponent vaccine against serogroup B meningococcus. Vaccine. 2010;28(31):5023–5030.
- Medini D, Stella M, Wassil J. MATS: global coverage estimates for 4CMenB, a novel multicomponent meningococcal B vaccine. Vaccine. 2015;33(23):2629–2636.
- da Silva RAG, Karlyshev, AV, Oldfield, NL. Variant signal peptides of vaccine antigen, FHbp, impair processing affecting surface localization and antibody-mediated killing in most meningococcal isolates. Front Microbiol. 2019;10:2847.
- Basta N, Mahmoud AAF, Wolfson J, et al. Immunogenicity of a meningococcal B vaccine during a university outbreak. N Engl J Med. 2016;375(3):220–228.
- Borrow R, Taha M-K, Giuliani MM, et al. Methods to evaluate serogroup B meningococcal vaccines: from predictions to real-world evidence J Infect. 2020;81(6):862–872.
- Muzzi A, Brozzi A, Serino L, et al. Genetic Meningococcal Antigen Typing System (gMATS): a genotyping tool that predicts 4CMenB strain coverage worldwide. Vaccine. 2019;37(7):991–1000.
- Brehony C, Rodrigues CMC, Borrow R, et al. Distribution of Bexsero® Antigen Sequence Types (BASTs) in invasive meningococcal disease isolates: implications for immunisation. Vaccine. 2016;34(39):4690–4697.
- Rodrigues CMC, Jolley, KA, Smith, A, et al. Meningococcal Deduced Vaccine Antigen Reactivity (MenDeVAR) index: a rapid and accessible tool that exploits genomic data in public health and clinical microbiology applications. J Clin Microbiol. 2020;59(1):e02161–20.
- Martinón-Torres F, Carmona Martinez A, Simkó R, et al. Antibody persistence and booster responses 24-36 months after different 4CMenB vaccination schedules in infants and children: a randomised trial. J Infect. 2018;76(3):258–269.
- Martinón-Torres F, Nolan T, Toneatto D, et al. Persistence of the immune response after 4CMenB vaccination, and the response to an additional booster dose in infants, children, adolescents, and young adults. Hum Vaccin Immunother. 2019;15(12):2940–2951.
- Nolan T, Santolaya ME, de Looze F, et al. Antibody persistence and booster response in adolescents and young adults 4 and 7.5 years after immunization with 4CMenB vaccine. Vaccine. 2019;37(9):1209–1218.
- Findlow J, Bai X, Findlow H, et al. Safety and immunogenicity of a four-component meningococcal group B vaccine (4CMenB) and a quadrivalent meningococcal group ACWY conjugate vaccine administered concomitantly in healthy laboratory workers. Vaccine. 2015;33(29):3322–3330.
- Read RC, Dull P, Bai X, et al. A phase III observer-blind randomized, controlled study to evaluate the immune response and the correlation with nasopharyngeal carriage after immunization of university students with a quadrivalent meningococcal ACWY glycoconjugate or serogroup B meningococcal vaccine. Vaccine. 2017;35(3):427–434.
- Szenborn L, Block SL, Jackowska T, et al. Immune responses to booster vaccination with meningococcal ABCWY vaccine after primary vaccination with either investigational or licensed vaccines: a phase 2 randomized study. Pediatr Infect Dis J. 2018;37(5):475–482.
- Toneatto D, Oster P, deBoer ACW, et al. Early clinical experience with a candidate meningococcal B recombinant vaccine (rMenB) in healthy adults. Hum Vaccin. 2011;7(7):781–791.
- Perez JL, Absalon J, Beeslaar J, et al. From research to licensure and beyond: clinical development of MenB-FHbp, a broadly protective meningococcal B vaccine. Expert Rev Vaccines. 2018;17(6):461–477.
- Harris SL, Tan C, Perez J, et al. Selection of diverse strains to assess broad coverage of the bivalent FHbp meningococcal B vaccine. NPJ Vaccines. 2020;5(1):8.
- Richmond PC, Marshall HS, Nissen MD, et al. Safety, immunogenicity, and tolerability of meningococcal serogroup B bivalent recombinant lipoprotein 2086 vaccine in healthy adolescents: a randomised, single-blind, placebo-controlled, phase 2 trial. Lancet Infect Dis. 2012;12(8):597–607.
- Abad R, García-Amil C, Navarro C, et al. Molecular characterization of invasive serogroup B Neisseria meningitidis isolates from Spain during 2015-2018: evolution of the vaccine antigen factor H binding protein (FHbp). J Infect. 2021;82(4):37–44.
- Bettinger JA, Liberator P, Halperin SA, et al. Estimated susceptibility of Canadian meningococcal B isolates to a meningococcal serogroup B vaccine (MenB-FHbp). Vaccine. 2020;38(8):2026–2033.
- Carannante A, Fazio C, Neri A, et al. Meningococcal B vaccine antigen FHbp variants among disease-causing Neisseria meningitidis B isolates, Italy, 2014-2017. PLoS One. 2020;15(11):e0241793.
- de Lemos APS, Sacchi CT, Gonçalves CR, et al. Genomic surveillance of Neisseria meningitidis serogroup B invasive strains: diversity of vaccine antigen types, Brazil, 2016-2018. PLoS One. 2020;15(12):e0243375.
- Tzanakaki G, Xirogianni A, Tsitsika A, et al. Estimated strain coverage of serogroup B meningococcal vaccines: a retrospective study for disease and carrier strains in Greece (2010-2017). Vaccine. 2021;39(11):1621–1630.
- Harris SL, Donald RGK, Hawkins JC, et al. Neisseria meningitidis serogroup B vaccine, bivalent rLP2086, induces broad serum bactericidal activity against diverse invasive disease strains including outbreak strains. Pediatr Infect Dis J. 2017;36(2):216–223.
- McNamara LA, Shumate AM, Johnsen P, et al. First use of a serogroup B meningococcal vaccine in the US in response to a university outbreak. Pediatrics. 2015;135(5):798–804.
- Basta N, Mahmoud, A, Wolfson, J, et al. Meningococcal B vaccine (4CMenB) immunogenicity pre- and postvaccination among university students in the US. Presented at: 36th Annual European Society for Paediatric Infectious Diseases (ESPID) Meeting; May 28-June 2, 2018; Malmo Sweden.
- Biolchi A, Tomei S, Santini L, et al. Evaluation of strain coverage of the multicomponent meningococcal serogroup B vaccine (4CMenB) administered in infants according to different immunisation schedules. Hum Vaccin Immunother. 2019;15(3):725–731.
- Pfizer Inc. Data on file: Final Clinical Study Report: a phase 4, single-arm, open-label study describing the safety and immunogenicity of Bexsero in healthy subjects aged between 12 and <19 years. B1971048. 2016.
- EU Clinical Trials Register. A Phase 4, Single-Arm, Open-Label Study Describing The Safety And Immunogenicity of Bexsero in Healthy Subjects Aged 12 Years to Less Than (<) 19 Years. [cited 2021 Mar 26]: Available from: https://www.clinicaltrialsregister.eu/ctr-search/trial/2014-003822-42/results.
- Biolchi A, De Angelis G, Moschioni M, et al. Multicomponent meningococcal serogroup B vaccination elicits cross-reactive immunity in infants against genetically diverse serogroup C, W and Y invasive disease isolates. Vaccine. 2020;38(47):7542–7550.
- Biolchi A, Tomei S, Brunelli B, et al. 4CMenB immunization induces serum bactericidal antibodies against non-serogroup B meningococcal strains in adolescents. Infect Dis Ther. 2021;10(1):307–316.
- Ladhani SN, Giuliani MM, Biolchi A, et al. Effectiveness of meningococcal B vaccine against endemic hypervirulent Neisseria meningitidis W strain, England. Emerg Infect Dis. 2016;22(2):309–311.
- Biolchi A, Tomei S, Santini L, et al. Four-component meningococcal serogroup B vaccine induces antibodies with bactericidal activity against diverse outbreak strains in adolescents. Pediatr Infect Dis J. 2021;40(2):e66–e71.
- Vaccines and Related Biologic Products Advisory Committee. Approaches to licensure of meningococcal vaccines for prevention of serogroup B invasive meningococcal disease: briefing document for the Vaccines and Related Biologic Product Advisory Committee Meeting, April 7. Silver Spring MD: US Food and Drug Administration; 2011.
- Soeters HM, McNamara LA, Blain AE, et al. University-based outbreaks of meningococcal disease caused by serogroup B, United States, 2013-2018. Emerg Infect Dis. 2019;25(3):434–440.
- Borrow R, Balmer P, Miller E. Meningococcal surrogates of protection–serum bactericidal antibody activity. Vaccine. 2005;23(17–18):2222–2227.
- Maiden MCJ, Ibarz-Pavón AB, Urwin R, et al. Impact of meningococcal serogroup C conjugate vaccines on carriage and herd immunity. J Infect Dis. 2008;197(5):737–743.
- Marsh JW, Shutt KA, Pajon R, et al. Diversity of factor H-binding protein in Neisseria meningitidis carriage isolates. Vaccine. 2011;29(35):6049–6058.
- Lemée L, Hong E, Etienne M, et al. Genetic diversity and levels of expression of factor H binding protein among carriage isolates of Neisseria meningitidis. PLoS One. 2014;9(9):e107240.
- Ala’aldeen DAA, Flint M, Oldfield NJ, et al. Human antibody responses to the meningococcal factor H binding protein (LP2086) during invasive disease, colonization and carriage. Vaccine. 2010;28(48):7667–7675.
- Sadarangani M, Sell T, Iro MA, et al. Persistence of immunity after vaccination with a capsular group B meningococcal vaccine in 3 different toddler schedules. CMAJ. 2017;189(41):E1276–E1285.
- Iro MA, Snape MD, Voysey M, et al. Persistence of bactericidal antibodies following booster vaccination with 4CMenB at 12, 18 or 24 months and immunogenicity of a fifth dose administered at 4 years of age-a phase 3 extension to a randomised controlled trial. Vaccine. 2017;35(2):395–402.
- Snape MD, Voysey M, Finn A, et al. Persistence of bactericidal antibodies after infant serogroup B meningococcal immunization and booster dose response at 12, 18 or 24 months of age. Pediatr Infect Dis J. 2016;35(4):113–123.
- Vesikari T, Østergaard L, Beeslaar J, et al. Persistence and 4-year boosting of the bactericidal response elicited by two- and three-dose schedules of MenB-FHbp: a phase 3 extension study in adolescents. Vaccine. 2019;37(12):1710–1719.
- Presa J, Findlow J, Vojicic J, et al. Epidemiologic trends, global shifts in meningococcal vaccination guidelines, and data supporting the use of MenACWY-TT vaccine: a review. Infect Dis Ther. 2019;8(3):307–333.
- Marshall HS, McMillan M, Koehler AP, et al. Meningococcal B vaccine and meningococcal carriage in adolescents in Australia. N Engl J Med. 2020;382(4):318–327.
- McMillan M, Walters L, Sullivan T, et al. Impact of meningococcal B (4CMenB) vaccine on pharyngeal Neisseria meningitidis carriage density and persistence in adolescents. Clin Infect Dis. 2021; 73(1):e99–e106.
- Soeters HM, Whaley M, Alexander-Scott N, et al. Meningococcal carriage evaluation in response to a serogroup B meningococcal disease outbreak and mass vaccination campaign at a college-Rhode Island, 2015-2016. Clin Infect Dis. 2017;64(8):1115–1122.
- McNamara LA, Thomas JD, MacNeil J, et al. Meningococcal carriage following a vaccination campaign with MenB-4C and MenB-FHbp in response to a university serogroup B meningococcal disease outbreak-Oregon, 2015-2016. J Infect Dis. 2017;216(9):1130–1140.
- Carr J, Plested E, Aley P, et al. ‘Be on the TEAM’ Study (Teenagers Against Meningitis): protocol for a controlled clinical trial evaluating the impact of 4CMenB or MenB-fHbp vaccination on the pharyngeal carriage of meningococci in adolescents. BMJ Open. 2020;10(10):e037358.
- Goldschneider I, Gotschlich EC, Artenstein MS. Human immunity to the meningococcus. I. the role of humoral antibodies. J Exp Med. 1969;129(6):1307–1326.
- TRUMENBA® (Meningococcal Group B Vaccine). Full Prescribing Information, Wyeth Pharmaceuticals LLC, a subsidiary of Pfizer Inc. Philadelphia PA; 2018.
- Sáez-Llorens X, Beltran-Rodriguez J, Novoa Pizarro JM, et al. Four-year antibody persistence and response to a booster dose of a pentavalent MenABCWY vaccine administered to healthy adolescents and young adults. Hum Vaccin Immunother. 2018;14(5):1161–1174.
- Peterson J, Drazan D, Czajka H, et al. Pentavalent meningococcal (MenABCWY) vaccine is safe and well tolerated with immunogenicity noninferior to coadministered MenB-FHbp and MenACWY-CRM in a phase 2 study of healthy adolescents and young adults. Open Forum Infect Dis. 2020;7(Suppl 1):S25–S26.
- Tzeng Y-L, Giuntini S, Berman Z, et al. Neisseria meningitidis urethritis outbreak isolates express a novel factor H binding protein variant that is a potential target of group B-directed meningococcal (MenB) vaccines. Infect Immun. 2020;88(12):e00462–20.