ABSTRACT
Introduction
The use of novel adjuvants in human vaccines continues to expand as their contribution to preventing disease in challenging populations and caused by complex pathogens is increasingly understood. AS01 is a family of liposome-based vaccine Adjuvant Systems containing 2 immunostimulants: 3-O-desacyl-4’-monophosphoryl lipid A and the saponin QS-21. AS01-containing vaccines have been approved and administered to millions of individuals worldwide.
Areas covered
Here we report advances in our understanding of the mode of action of AS01 that contributed to the development of efficacious vaccines preventing disease due to malaria, herpes zoster, and respiratory syncytial virus. AS01 induces early innate immune activation that induces T cell-mediated and antibody-mediated responses with optimized functional characteristics and induction of immune memory. AS01-containing vaccines appear relatively impervious to baseline immune status translating into high efficacy across populations. Currently licensed AS01-containing vaccines have shown acceptable safety profiles in clinical trials and post-marketing settings.
Expert opinion
Initial expectations that adjuvantation with AS01 could support effective vaccine responses and contribute to disease control have been realized. Investigation of the utility of AS01 in vaccines to prevent other challenging diseases, such as tuberculosis, is ongoing, together with efforts to fully define its mechanisms of action in different vaccine settings.
PLAIN LANGUAGE SUMMARY
Adjuvants are added to vaccines to increase the immune response produced after vaccination. Adjuvant Systems contain 2 or more molecules that stimulate the immune system. AS01 is an Adjuvant System that contains 2 components, MPL and QS-21, that stimulate the immune system. AS01 is included in 3 approved vaccines: a malaria vaccine for children, a herpes zoster vaccine for older adults, and a respiratory syncytial virus vaccine also for older adults. Vaccines containing AS01 have been extensively evaluated in clinical trials and administered to millions of individuals during market use. These vaccines are effective in preventing disease and have acceptable safety in different age groups. Experiments have been done to investigate how AS01 works in vaccines to produce an efficient immune response that helps to protect against the disease being targeted. A key effect of AS01 is to encourage specific immune cells to produce chemicals that stimulate the immune system. We now know that this effect is due to co-operation between MPL and QS-21. Experiments have shown that AS01 induces a sophisticated immune ‘gene signature’ in blood within 24 hours after vaccination, and people who developed this ‘gene signature’ had a stronger response to vaccination. AS01 seems to be able to stimulate the immune system of most people – even if they are older or have a weakened immune system. This means that AS01 could be included in other vaccines against other challenging diseases, such as tuberculosis, or could be used in the treatment of some disease, such as chronic hepatitis B.
Disclaimer
As a service to authors and researchers we are providing this version of an accepted manuscript (AM). Copyediting, typesetting, and review of the resulting proofs will be undertaken on this manuscript before final publication of the Version of Record (VoR). During production and pre-press, errors may be discovered which could affect the content, and all legal disclaimers that apply to the journal relate to these versions also.1. INTRODUCTION
Adjuvants are components added to vaccines to increase the magnitude, durability, and/or breadth of the immune response to vaccine antigens. Aluminum salts were the first adjuvants used in human vaccines and their use has spanned a century [Citation1]. New adjuvants and Adjuvant Systems (AS, combinations of 2 or more immunomodulatory agents) were initially developed during the 1990s to enhance immunogenicity in vulnerable populations with typically poor responses to vaccination. Today, adjuvants and AS have been included in numerous licensed vaccines (). In this review we focus on the Adjuvant System AS01 (GSK). AS01 refers to a family of adjuvants: AS01B (50 µg of 3-O-desacyl-4’-monophosphoryl lipid A (MPL) and 50 µg of Quillaja saponaria Molina, fraction 21 (QS-21) per injected dose) and AS01E (25 µg of MPL and 25 µg of QS-21 per injected dose), both in liposomal formulation.
Table 1 Adjuvants and Adjuvant Systems used in a range of licensed prophylactic vaccines.
Seven years ago, in this journal, a review summarized the unique aspects of AS01 and concluded that it showed good prospects for disease control through vaccination [Citation2]. At that time, the authors opined that “over the next 5 years, we will see new vaccines containing novel adjuvants, such as the CpG-adjuvanted candidate hepatitis B vaccine, designed to be used against pathogens for which aluminum salt as adjuvant proved insufficient. These new adjuvanted vaccines will aim to provide improved protection to vulnerable populations such as the elderly and immunocompromised.” [Citation2]. Since that publication, the onset of the coronavirus disease 2019 (COVID-19) pandemic stimulated the production and rollout of vaccines employing a range of existing and novel technologies including mRNA, adenovirus vectors, cytosine phosphoguanosine (CpG)-aluminum hydroxide, Alhydroxiquim-II, AS03, and Matrix-M (Novavax AB). Additionally, several other vaccines containing AS01 were approved; the RTS,S/AS01E malaria vaccine (Mosquirix, GSK) for use in children, gE/AS01B recombinant herpes zoster vaccine (RZV, Shingrix, GSK) for adults aged ≥50 years and immunocompromised persons aged ≥18 years, and most recently in a respiratory syncytial virus (RSV) vaccine (RSVPreF3-AS01 E vaccine, AREXVY, GSK) for use in adults from 60 years of age, and is under review for approval for adults at risk from 50 years of age. RTS,S/AS01E has been rolled out and administered to around 2 million children since 2019, and its efficacy and acceptable safety profile have been confirmed and continue to be actively monitored in large surveillance studies [Citation3–5]. Long-term efficacy of RZV in adult age groups demonstrated in phase III trials has been confirmed in post-marketing real-world effectiveness studies [Citation6–13]. The extensive clinical experience with AS01-containing vaccines gained since our last review has allowed us to expand and update our knowledge on the mode of action of this adjuvant system, and to re-emphasize its importance in driving effective immune responses to vaccine targets.
2. WHAT IS ADJUVANT SYSTEM AS01?
Combinations of adjuvants were first explored in the 1990’s after unsuccessful attempts to develop a human immunodeficiency virus (HIV) vaccine using classical methods [Citation14]. Adjuvants such as MPL (3-O-desacyl-4’-monophosphoryl lipid A) and QS-21 (Quillaja saponaria Molina, fraction 21 licensed by GSK from Antigenics LLC, a wholly owned subsidiary of Agenus Inc., a Delaware, USA corporation) were known from the 1980s to boost different aspects of the immune response. Combinations of these immune-modulating molecules and classical adjuvants such as aluminum salts, emulsions and liposomes, were expected to have additive or possibly synergistic effects in inducing specific immune responses [Citation14].
AS01 is an Adjuvant System containing MPL, QS-21 and liposome. MPL (produced by GSK) is a detoxified lipopolysaccharide obtained by hydrolysis of lipopolysaccharide (LPS) from Salmonella minnesota. QS-21 is a triterpene glycoside purified from the bark of Quillaja saponaria Molina. The liposomes contain cholesterol, and act as delivery vehicles. The cholesterol in the liposome formulation quenches the intrinsic hemolytic activity of QS-21 [Citation15]. AS01 induced strong CD4+ and humoral responses in animal studies and was therefore initially tested as adjuvant in the RTS,S malaria vaccine [Citation14].
2.1. The interaction between MPL and QS-21 is important to drive AS01 adjuvanticity
MPL binds to the innate immune receptor Toll-like receptor 4 (TLR4), which belongs to the family of pattern recognition receptors (PRRs) [Citation16]. PRRs such as TLR4 recognize pathogen-associated molecular patterns (PAMPs) and use them to identify threats to immune homeostasis [Citation17,Citation18]. Upon activation, TLR4 activates a proinflammatory immune response through a complex signaling pathway that involves 2 adapter molecules, Myd88 (myeloid differentiation primary response 88) that controls the release of cytokines, and TRIF (TIR-domain-containing adapter-inducing interferon [IFN]-β) that induces release of type 1 IFN [Citation18,Citation19]. However, in contrast to findings in mice [Citation20], MPL signaling in human cells is inefficient in engaging the interferon regulatory transcription factor 3 pathway and does not result in the induction of type I IFN in vitro [Citation19].
The signaling pathways triggered by QS-21 are less well understood. QS-21 accumulates in resident macrophages in the draining lymph node (dLN) and activates caspase-1, a converting enzyme that cleaves other proteins such as interleukin-1beta (IL-1β) and IL-18 into their active, mature forms. These events stimulate the production of proinflammatory cytokines and recruitment and activation of innate immune cells such as dendritic cells [Citation21]. The adjuvant effect of QS-21 depends on the integration of caspase-1 and Myd88 pathways and release of HMGB1 (high-mobility group protein B1) [Citation22] ().
Figure 1: Schematic representation of the cellular mechanism signaling pathways triggered by MPL and QS-21.
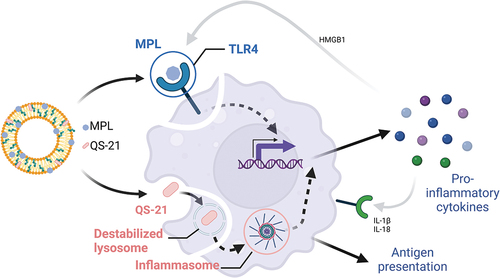
Importantly, combining MPL and QS-21 induces an innate signature that is not observed when individual components are administered [Citation23,Citation24]. This is likely to emerge from two combined effects. First, MPL and QS-21 share signaling pathways, such that when an innate cell is exposed to both immunostimulants, complex signal integration is likely to occur. Secondly, MPL and QS-21 activate distinct cell types, creating a network of innate effector functions. Both these mechanisms have been reported to serve as a means for signal amplification in the initiation of an antimicrobial response [Citation25], and could result in an improved response to vaccine antigens.
2.2. How AS01 modulates the immune response – what’s new?
It has been long understood that adjuvants, including AS01, exert their effects on the immune response at the level of the innate response, which are then translated into an optimized adaptive response. The mode of action by which AS01 enhances the immune response has been well studied in animal models. In animals, the adjuvant effects of AS01 rely on the spatial and temporal co-localization of AS01 and the antigen, indicating that the adjuvant acts locally in muscle and dLNs [Citation26]. Multiple features of AS01-induced innate response have been described () [Citation21–23,Citation26].
Figure 2: Schematic representation of the mode of action of AS01.
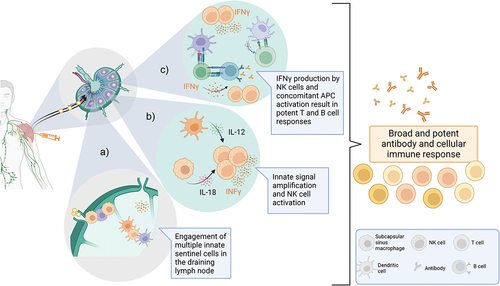
Shortly after injection, AS01 drains from the muscle to reach the dLN [Citation22,Citation26]. Rapid production of cytokines is detected both in the injected muscle and in the dLN as early as 3 hours after immunization [Citation23,Citation26]. This inflammatory response is transient, peaking after 1–2 days and returning to baseline after 7 days. Specific chemokines associated with cell recruitment are released, resulting in innate cell infiltration in muscle and dLN.
Specifically, the numbers of dendritic cells and lymphocytes increase rapidly in the dLN [Citation26], probably resulting in increased frequency of antigen presenting cells (APC) and T cell interactions and ultimately favoring antigen-specific T cell priming. AS01 also increases the diversity of dendritic cell subsets in the dLN and enhances their expression of crucial costimulatory molecules such as CD86 and CD40. Overall, these effects result in efficient priming of antigen-specific T cells, which in turn supports activation and maturation of B cells, promoting humoral responses to vaccination [Citation27,Citation28].
Animal studies of innate effector cells in muscle and dLN have identified that both migratory and tissue resident innate lymphoid cells contribute to shaping of adaptive immune responses by AS01 within dLNs through rapid effector cytokine release [Citation29]. Recent studies in mice showed that TLR4 expression in hematopoietic cells in muscle and dLN is sufficient to mediate the adjuvant effect of AS01, with little input from non-hematopoietic cells in either location [Citation30].
Unlike other adjuvants such as emulsions or aluminum salts, AS01 does not directly enhance antigen uptake by monocytes or dendritic cells [Citation26]. However, recent evidence suggests that AS01 does increase antigen uptake by dLN B cells in mice, highlighting the complex interaction between AS01 and the dLN cellular milieu. While this effect was related to an impact on lymph flow and antigen entry in the dLN for a different, nanoparticle based, saponin/MPL formulation, the authors did not investigate these parameters for AS01 [Citation31].
The hallmark of the AS01 innate signature is now known to be the robust production of IFNγ and IFNγ-related cytokines, such as CXCL10, CXCL9. In mice, IFNγ production in the dLN is detectable as early as 3 hours post-vaccination and peaks at 6 hours, demonstrating an extremely rapid innate immune activation by AS01 [Citation32,Citation33]. In primary responses, this effect is a direct consequence of the synergy between MPL and QS-21 and is likely dependent on the complex network of cells activated by these components. For instance, the IFNγ response is dependent on IL-12 (likely produced by dendritic cells) and IL-18, released by lymph node resident macrophages. Resident natural killer (NK) cells, which are the main producers of IFNγ in the dLN, represent an attractive ‘signal integration hub’ for early AS01-mediated innate immune stimuli.
We propose that the early production of IFNγ is a key step in the amplification of immune activation, as IFNγ is an important cytokine involved at different steps of the immune response, including activation of macrophages and NK cells, as well as T and B cell differentiation [Citation34,Citation35]. The importance of the role of IFNγ in mediating the adjuvant effect of AS01 has been demonstrated in animal challenge models for the RTS,S/AS01E malaria vaccine, where blocking IFNγ resulted in loss of vaccine-induced protection [Citation23].
Importantly, many of the findings on AS01 mode of action uncovered in animal models were confirmed by clinical experience with AS01-containing vaccines. The conduct of exploratory clinical trials comparing different Adjuvant Systems (AS01, AS03, AS04, plus the adjuvant aluminum hydroxide) formulated with the hepatitis B surface antigen (HBsAg) allowed testing of at least some of the above proposed mechanisms of action in humans and recapitulated many earlier findings () [Citation36]. Vaccination of hepatitis B virus-naïve individuals with two doses (1 month apart) of AS01/HBsAg resulted in robust antibody titers and, importantly, CD4+ T cell responses, which were higher than those induced by other adjuvant formulations tested. All adjuvanted formulations were able to induce T cells with a high degree of polyfunctionality, but the number of polyfunctional CD4+ T cells was highest with AS01/HBsAg [Citation36]. Similarly innate immune activation, as measured by early serum cytokine responses, was highest in subjects receiving AS01/HBsAg. Importantly, AS01 drove an IFNγ response in the serum, including high levels of the downstream chemokine CXCL10. Transcriptional analysis of peripheral blood mononuclear cells identified a core innate gene signature that emerged within 24 hours after vaccination [Citation37], including genes related to the IFN pathway and NK cell-related functions. Expression of this core signature was associated with early activation of the innate response and with the magnitude of the adaptive response and more recently with humoral response durability (manuscript in preparation). Importantly, and in contrast to other Adjuvant Systems investigated in this study, AS01 not only induced the highest average expression of this signature, but also resulted in expression of the core signature in a majority of subjects. This suggests that the stronger innate response induced by AS01 is capable of overcoming individual immune heterogeneity, thus reducing the impact of poor immune fitness on the vaccine response [Citation37]. This hypothesis is consistent with the robust immune responses observed in older adults >50 years of age vaccinated with AS01-containing RZV, and RSVPreF3-AS01E [Citation38,Citation39], and with evidence that 2 doses of an AS01-containing tuberculosis (TB) vaccine overcome differences between individuals in their baseline immunity to TB [Citation40].
Figure 3: Clinical trial designs comparing different adjuvants formulated with the hepatitis B surface antigen (HBsAg) (panel A) to decipher the mechanisms of action in humans (panel B) [Citation36].
![Figure 3: Clinical trial designs comparing different adjuvants formulated with the hepatitis B surface antigen (HBsAg) (panel A) to decipher the mechanisms of action in humans (panel B) [Citation36].](/cms/asset/ad7fc92a-3a96-49e0-ba5c-fc7267d8d802/ierv_a_2382725_f0003_oc.jpg)
When compared to HBsAg/Aluminum hydroxide, significant differences in the quantity and quality of the humoral immune response induced by HBsAg/AS01were observed [Citation41,Citation42]. Specifically (i) HBsAg/AS01 induced higher titers of HBsAg-specific antibodies; (ii) HBsAg/AS01 induced antibodies of higher quality, such as increased avidity, increased breadth of antibody-mediated effector functions, and increased B cell memory responses; (iii) the proportion of subjects showing high avidity antibodies was higher with HBsAg/AS01 than with HBsAg/Aluminum hydroxide. Intriguingly, the emulsion Adjuvant System AS03 induced similar effects on HBsAg-specific humoral responses in spite of different composition and signaling. As such and considering the differential effects of AS01 and AS03 on T cell responses to HBsAg, the functional differences between these two adjuvants remain partially to be elucidated.
While invaluable information can be drawn from exploratory clinical research studies, these are resource intensive. In vitro modelling of adjuvant effects using primary human cells is emerging as an avenue to complement animal and clinical adjuvant mode of action data [Citation43]. For instance, stimulation of peripheral blood mononuclear cells with AS01 resulted in strong monocyte activation. Importantly, in spite of age-associated declines in immune function, monocytes from older individuals were still capable of producing inflammatory and co-stimulatory signals in response to AS01 [Citation44].
Overall, the body of evidence accumulated in the clinical development of AS01-containing vaccines suggests that many of the features of AS01 mode of action described above are involved in promoting efficient adaptive immune responses, ultimately contributing to vaccine induced protection.
3. GLOBAL EXPERIENCE WITH AS01-CONTAINING VACCINES
3.1. Update on RTS,S/AS01E malaria vaccine
RTS,S/AS01E contains a form of the Plasmodium falciparum circumsporozoite protein (CSP) fused to HBsAg and formulated with AS01E. RTS,S/AS01E has now undergone evaluation in the Malaria Vaccine Implementation Programme (MVIP), a multi-partner collaboration to assess real-world effectiveness and safety of RTS,S/AS01E in the target age group of 5–17- month-old children living in malaria endemic regions of Africa [Citation45]. Based on results of the 4-year pilot program, the World Health Organization (WHO) recommended RTS,S/AS01E for all children living in regions with moderate to high P. falciparum transmission [Citation46]. Nearly 2 million children have received their first RTS,S/AS01E dose [Citation3]. Data from the MVIP found that the effectiveness of vaccination against hospital admission due to severe malaria was 30% (95% confidence interval [CI] 8–46%), and effectiveness against hospital admission with a positive malaria test was 21% (95% CI 7–32%) [Citation5], which is consistent with findings from phase III efficacy studies [Citation47,Citation48]. More recently, the combination of seasonal malaria vaccination with seasonal chemoprevention demonstrated significantly higher protection against disease that any of the 2 interventions alone in young children in Burkina Faso and Mali, opening the door to new prevention strategies for malaria control [Citation49].
Plasmodium species have complex life-cycles that include mosquito vector, human liver, and human blood stages [Citation50]. Mosquitoes inject sporozoites when having a blood meal which then spend several hours in the blood stream prior to infecting liver cells. By inducing a robust antibody response against sporozoites, RTS,S/AS01E aims to prevent liver infection and clinical malaria disease [Citation51]. The magnitude of the antibody response induced by RTS,S/AS01E correlates with protection against malaria [Citation52,Citation53]. RTS,S/AS01E induces increased IFNγ levels in the serum and IFN-related transcriptional responses in peripheral blood mononuclear cells which are also associated with protection from controlled malaria challenge [Citation53]. Antibodies induced by RTS,S/AS01E appear to act by blocking transit of sporozoites to the liver and by restricting their infectivity in this organ () [Citation54].
Figure 4: Schematic representation of RTS,S/AS01E malaria vaccine mode of action.
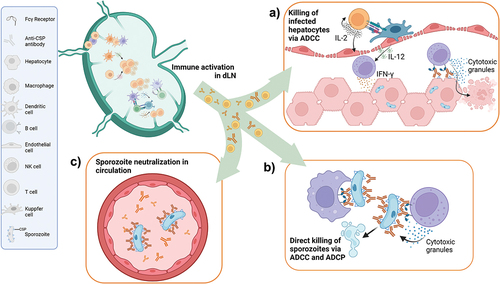
More recently the quality of the immune response in terms of antibody specificity, subclass distribution and antibody avidity were found to be important in predicting protection in malaria challenge studies [Citation54,Citation55]. In particular, the ability of RTS,S/AS01E to induce CSP-specific antibodies promoting antibody dependent cellular cytotoxicity (ADCC) played an important role in predicting protection in challenge studies. ADCC is mostly mediated by NK cells, an important cell type in the mode of action of AS01. The presence of NK cells in peripheral blood is negatively correlated with the antibody response to RTS,S/AS01E and with protection against malaria. This observation could be caused by RTS,S/AS01E vaccination stimulating NK cells to leave the circulation and move to lymphoid organs and the liver, where they interact with CSP-specific antibodies to inhibit sporozoite invasion by killing infected hepatocytes () or direct killing () [Citation53,Citation54].
If the CSP-mediated mechanism of protection is mostly antibody mediated, how does the potent CD4+ T cell response induced by RTS,S/AS01E contribute to protection? First of all, CD4+ T cells play an essential role in promoting antibody quality. A specialized subset of CD4+ T cells in the lymph node, follicular helper T cells provide help for optimal B cell maturation and antibody production. RTS,S/AS01E induced strong follicular helper T cell responses [Citation56], and when linked to a different malaria antigen, AS01 induced higher levels of antigen-specific CD4+ T follicular than other vaccine platforms [Citation57]. Appropriate help from follicular helper T cells will result in antibodies with multiple effector functions beyond neutralization of circulating sporozoites (). By interacting with different innate immune cells, highly functional antibodies can mediate direct killing of sporozoites either by ADCC or by antibody dependent cellular phagocytosis (ADCP) () [Citation58]. Secondly, effector CD4+ T cells may indirectly contribute to protective effector functions by promoting ADCC in the liver (). CSP-specific antibodies bind to CSP on the surface of infected hepatocytes. CSP-specific CD4+ T cells secrete IL-2 and cause activation of liver NK cells. Liver NK cells are further activated by binding of the surface FcγRIII receptor by CSP-specific antibodies [Citation59]. As such, activating multiple arms of the immune responses, RTS,S/AS01E is able to unleash cooperative adaptive and innate effector functions.
3.2. Update on recombinant zoster vaccine (RZV)
RZV contains recombinant varicella zoster virus glycoprotein E (gE) antigen with AS01B (gE/AS01B). RZV is highly effective in preventing herpes zoster and its most common complication, post-herpetic neuralgia, even at the extremes of older age where immune senescence usually results in sub-optimal immune responses and poor efficacy [Citation60,Citation61]. In the United States (US) and Europe, RZV is approved for the prevention of herpes zoster and post-herpetic neuralgia in adults aged 50 years and over and more recently for prevention of herpes zoster and post-herpetic neuralgia in immunodeficient or immunosuppressed adults aged 18 years and older [Citation62,Citation63]. Vaccine efficacy was 68.2% (95% CI, 55.6–77.5%) in autologous hematopoietic cell transplant recipients, 87.2% (44.3–98.6%) in persons with hematological malignancies, and 90.5% (73.5–97.5%) in those with pre-existing potential immune-mediated diseases (pIMDs) [Citation64,Citation65].
Protective efficacy after RZV appears to be durable. After vaccination with 2 RZV doses, vaccine efficacy was sustained at 73.2% (95% CI 46.9–87.6%) during the 10th year [Citation13]. Immune responses had waned modestly by year 10, with anti-gE antibodies remaining 5-fold higher than pre-vaccination levels, and there was little change in gE-specific CD4+ T cell frequency [Citation13]. Modelling studies predict that humoral and cell-mediated immune (CMI) responses should persist for more than 20 years after vaccination [Citation66]. An additional vaccination administered to adults in their 80’s 10 years after the initial immunization course induced marked increases in antibody levels and CD4+ T cell frequencies, indicating a robust anamnestic response [Citation66].
The contribution of AS01B to the immune response induced by RZV has been well documented. Compared to administration of gE alone, the addition of AS01B results in strikingly higher antibody levels and CD4+ T cell responses [Citation67]. Further insights about the contribution of AS01B to RZV-mediated cellular immune responses can be gleaned by comparing RZV with live attenuated zoster vaccine (ZVL, Zostavax, MSD). RZV induced a much higher gE and varicella zoster virus VZV-specific memory Th1 response than ZVL, 30 days after the last vaccination. RZV induced a marked memory T cell response which was associated with persistence, while gE-specific T cells induced by ZVL had an effector phenotype, which is associated with a shorter duration of the immune response. Importantly, these findings hold true not only for CD4+ helper T cells, but also for CD8+ T cells [Citation68,Citation69]. Recent data comparing ZVL and RZV vaccines also showed that RZV expanded more gE-specific CD4+ T cells with greater breadth [Citation70]. Intriguingly, analysis of T cell receptor sequences and of gE-specific persistent CD4+ T cells suggests that the durability of the immune responses elicited by RZV may rely in part on its ability to recruit naïve CD4+ T cells during the early stages of the vaccine response. Finally, both RZV or ZVL have been shown to generate anti-gE and anti-VZV ADCC, with evidence that RZV had greater ability to induce ADCC antibodies than ZVL [Citation71].
3.3. Respiratory syncytial virus (RSV) vaccine
There is growing appreciation that RSV causes a substantial burden of disease in older adults, immunocompromised persons, and individuals with chronic medical conditions, including cardiovascular diseases. RSV is a driver of morbidity and mortality in these populations, although the true disease burden remains incompletely described [Citation72]. RSV has two major subtypes, A and B, that may cocirculate. The fusion protein (F) is a major glycoprotein found on the RSV envelop and the pre-fusion (PreF) conformation is the major target for neutralizing antibodies [Citation73,Citation74].
No correlates of protection exist for RSV, but it is likely that multiple mechanisms contribute to preventing the illness, specifically in older adults. Neutralizing antibodies play a major role in the prevention of lower respiratory tract disease caused by RSV, and low serum neutralization titers in older adults has been linked to an increased risk of symptomatic RSV infection and hospitalization [Citation75,Citation76]. Beyond the humoral response, RSV-specific CD4+ and CD8+ T cell responses in older adults occur at lower frequency and have lower functionality and proliferative capacity compared to younger adults, that likely contributes to the increased susceptibility of older adults to severe disease [Citation77,Citation78].
RSVPreF3-AS01E is a combination of the recombinant RSVPreF3 protein and AS01E designed to induce a robust humoral and cellular immune response, to help protect older adults and those adults aged 50–59 years with chronic medical conditions leading to an increased risk for RSV disease against RSV-related lower tract respiratory disease. As observed for other AS01-containing vaccines, the combination of the RSVPreF3 antigen with AS01E induced statistically higher Th1 CD4+ T cell responses compared with administration of RSVPreF3 alone [Citation39]. The AS01-containing formulation boosted cellular immune responses and restored RSVPreF3 Th1 CD4+ T cell levels in older adults to within the range seen in young adults. There was no adjuvant effect on the magnitude of the antibody response. However, further investigation suggests that compared to unadjuvanted RSVPreF3, RSVPreF3-AS01E induced a broader neutralizing antibody booster response that covered antigenically distant RSV strains, including those that currently dominate in the circulation [Citation79].
In a phase III efficacy study performed across consecutive RSV seasons, RSVPreF3-AS01E showed statistically significant and clinically meaningful overall efficacy of 82.6% (96.95% CI, 57.9–94.1%) in preventing RSV lower respiratory tract disease in the target population [Citation80]. Vaccine efficacy was 94.1% (95% CI, 62.4–99.9%) against severe RSV disease, and 94.6% (95% CI, 65.9–99.9%) in older adults with underlying cardiorespiratory and endocrine-metabolic conditions. Vaccine efficacy was similar against the RSV A and B subtypes. More recent results showed that efficacy was sustained over two seasons, with cumulative vaccine efficacy of 67.2% (97.5% CI 48.2–80.0) against RSV lower respiratory tract disease and 78.8% (95% CI 52.6–92.0) again severe lower respiratory tract disease [Citation81].
The high efficacy observed in older adults with underlying co-morbidities could have marked impacts on morbidity and mortality due to RSV in the most vulnerable populations. In addition to the induction of robust levels of broadly neutralizing antibody titers and restoration of RSVPreF3-specific CD4+ T cells, other immune parameters may be contributing to the high efficacy observed in the phase III study and are currently under investigation.
RSVPreF3-AS01E was first approved for prevention of lower respiratory tract disease caused by RSV in individuals ≥60 years of age in the US in May 2023, and thereafter in other countries including Europe, Canada and Japan [Citation82–85]. RSVPreF3-AS01E is the first RSV vaccine ever to be approved.
3.4. Safety and reactogenicity
AS01-containing vaccines have been given to millions of people and there is now broad published evidence of their safety profiles. Reactogenicity refers to the physical manifestations of the inflammatory response to vaccination [Citation86]. Adjuvanted vaccines are typically more reactogenic than unadjuvanted formulations containing the same antigen. For example, AS01 induces more reactogenicity than aluminum hydroxide when used in combination with HBsAg, with higher rates of pain and other adverse reactions at the injection site [Citation87,Citation88]. This is likely a consequence of the enhanced innate immune responses elicited by the adjuvant components at the site of injection. Most of these reactions are mild to moderate and transient. A meta-analysis of the safety of new adjuvants (AS01, AS02, AS03 and MF59) in older adults, as reported in clinical trials concluded that despite increased reactogenicity observed for newly adjuvanted vaccines compared to control groups, there was no overall increase in serious adverse events or fatalities [Citation89].
3.4.1. RTS,S/AS01E
The evaluation of RTS,S/AS01E in clinical trials involved approximately 15,000 children aged 6–12 weeks and 5–17 months who received a 3 dose-schedule of either RTS,S/AS01E or control vaccines (rabies vaccine in children and meningococcal serogroup C conjugate vaccine in young infants), and more than 4,200 who received a fourth dose of RTS,S/AS01E 18 months after dose 3 [Citation47]. In a phase III trial, the frequency of solicited reports of local adverse events symptoms was similar among infants after RTS,S/AS01E and after the childhood vaccines used in the study. Systemic reactogenicity was higher in the RTS,S/AS01E group than in the control group [Citation90,Citation91]. The fourth RTS,S/AS01E dose was also more reactogenic than the comparator vaccine in both children and young infants, with a higher frequency of both systemic and local reactions [Citation47]. Severe (Grade 3) solicited adverse events (i.e., drowsiness, irritability and loss of appetite) were rare, except for Grade 3 fever (>39°C) that occurred in 5.3% of children and 1.5% of infants following a fourth dose of RTS,S/AS01E. As observed during the primary series, the incidence of fever within 7 days post-vaccination was higher in both infants and children who received a fourth dose of RTS,S/AS01E vaccine than in those who received the control vaccine, and a small number of these febrile reactions were accompanied by generalized convulsive seizures [Citation47]. This study noted an imbalance between the RTS,S/AS01E and control group in the incidence of meningitis and cerebral malaria cases occurring in children (but not infants), and higher mortality in girls vaccinated with RTS,S/AS01E versus girls who received control vaccines [Citation92]. None of these signals have been observed in the phase I/II studies, in other phase III studies, or in the MVIP pilot evaluations. On this basis, the WHO concluded that these were chance findings [Citation46]. A systematic review of 21 clinical trials of RTS,S/AS01E concluded that most adverse events observed after RTS,S/AS01E were also observed after other vaccines [Citation93].
3.4.2. RZV
In clinical trials in older adults, RZV was more reactogenic than placebo. Solicited or unsolicited reports of Grade 3 symptoms were reported by 17.0% of RZV recipients and 3.2% of placebo recipients in adults aged >50 years [Citation61]. In adults >70 years, solicited reports of injection-site and systemic reactions were also more common among RZV recipients than among placebo recipients. Adverse events were generally mild to moderate in intensity and transient, and the overall incidences of serious adverse events, pIMDs, and deaths were similar across the study groups [Citation60,Citation61]. An open-label study found that vaccine-related reactogenicity did not impact the quality of life in the majority of vaccinees [Citation94]. Post-marketing safety data with RZV is consistent with the safety profile observed in clinical trials, with commonly reported adverse events indicative of reactogenicity [Citation95,Citation96].
In an overview of six clinical trials of RZV in immunocompromised populations (≥18 years of age) that included patients with autologous hematopoietic stem cell transplant, renal transplant, hematologic malignancies, solid tumors, or HIV who are at an increased risk of herpes zoster [Citation97], the percentage of adults reporting serious adverse events, deaths, and pIMDs was balanced across RZV and placebo recipients. No safety concerns were identified in the safety overview [Citation97]. A meta-analysis of 17 clinical trials and 19 cohort studies that aimed to investigate the efficacy, effectiveness, and safety of RZV and zoster vaccine live in immunocompetent and immunocompromised subjects found that the incidences of injection site symptoms, systemic adverse events, serious adverse events, and deaths in RZV recipients were not statistically significantly different between immunocompromised and immunocompetent subjects [Citation12].
3.4.3. RSVPreF3-AS01E
In the large phase III placebo-controlled trial in adults ≥60 years of age, RSVPreF3-AS01E was more reactogenic than placebo but these reactions were generally transient and mild to moderate in severity [Citation80]. The overall incidences of pIMDs and serious adverse events were similar in vaccine and placebo groups.
4. TUBERCULOSIS VACCINE: AN EXAMPLE OF A NEW AS01-CONTAINING VACCINES IN DEVELOPMENT
Globally, TB is the second most common cause of death due to infectious diseases after COVID-19 and 1.3 million people died from TB in 2022 [Citation98]. Multi-resistant TB is a public health crisis that threatens global disease control. An effective vaccine that could prevent TB reactivation in infected adults would have profound implications for reducing disease transmission and reducing the disease burden due to active pulmonary TB.
Vaccine development against TB has been hampered by the complex nature of the disease, its various clinical manifestations, and the ability for Mycobacterium tuberculosis to reside dormant for long periods in granulomas shielded from the immune system [Citation99]. Traditionally it was though that strong T cell responses were required to mediate protection against active TB but growing evidence points to an important contribution of humoral immunity [Citation100].
The AS01-containing investigational TB vaccine contains the M72 antigen, a recombinant fusion protein derived from 2 immunogenic M. tuberculosis antigens; Mtb32A and Mtb39A. A proof-of-concept phase 2b study in HIV-negative adults (18–50 years) with TB infection found that vaccine efficacy of M72/AS01E (2 doses administered 1 month apart) at month 36 was 49.7% (95% CI 2.1–74.2%) [Citation101].
In mice, M72/AS01E induced polyfunctional, Th1-expressing M72-specific CD4+ and CD8+ T cells, with high levels observed in the lungs [Citation102]. Two doses of M72/AS01E induced high levels of M72-specific antibody responses and polyfunctional M72-specific CD4+ T cells that express Th1 cytokines; namely IFNγ, TNFα and IL-2 that remain detectable after 3 years [Citation103]. Humoral and CD4+ T cell responses were markedly enhanced by the addition of AS01 compared to administration of M72 alone. As observed for other AS01-containing vaccines, M72/AS01E also induced a transient IFNγ response in serum 24 hours after vaccination. Moreover, humoral and CMI responses to vaccination appeared to be similar in healthy non- bacille Calmette-Guérin (BCG)-vaccinated individuals, and BCG-vaccinated adolescents living in TB endemic areas and therefore more likely to be infected [Citation104]. This further illustrates the ability of AS01 to overcome individual differences in baseline immune memory responses. Indeed, two vaccine doses induced a homogenous transcriptional signature in groups of individuals who had been treated for TB and who were disease-naïve [Citation40].
5. CONCLUSIONS
While the mode of action of AS01 continues to be elucidated in animal models and through clinical trials, it has become clear that AS01-containing vaccines are able to overcome many of the limitations of the immune response brought about by immune senescence, chronic disease, and immunosuppression. Since our last review, three AS01-containing vaccines have been approved for use; two for the prevention of severe viral illnesses in adults (herpes zoster and RSV), and one for the prevention of a complex parasitic disease, malaria, in children. The efficacy of RZV and RTS,S/AS01E has been confirmed in post-marketing/implementation settings and RSV post-marketing vaccination of older adults with RSVPreF3-AS01 has started in the 2023 season. Available clinical and post-marketing data with currently licensed AS01-containing vaccines continue to show acceptable safety profiles.
6. EXPERT OPINION
The investigation and use of AS01-containing vaccines has expanded across diverse disease states, including acute respiratory infection (RSV), dermatological manifestations of viral reactivation (herpes zoster), parasitic infection (malaria), and chronic granulomatous infection (TB). As a result, new features of the mode of action of AS01 have become apparent. AS01 induces T follicular helper cells, broad antibody responses with optimized functional characteristics and induction of immune memory, consistent induction of polyfunctional T cell responses in a broad range of populations including children, older adults, and immunocompromised individuals. AS01-containing vaccines appear relatively impervious to baseline immune status, seeming to consistently induce robust responses across age groups and disease states and in populations (elderly and immunocompromised) in whom vaccine responses are typically attenuated [Citation37,Citation40], translating into high efficacy in these populations. How this ‘flattening’ effect of pre-existing immune defects occurs and how it could be further leveraged in vaccine design, is not totally known. It is feasible that AS01 engages multiple immune pathways simultaneously, ensuring redundancy in protection mechanisms, so that defects in one arm are compensated by another.
Many of the immune features of AS01 are similar across vaccines using different antigens, but several are not. For example, CD8+ T cell activation by AS01-containing vaccines has been observed in pre-clinical models, but consistent evidence of this effect in humans has been lacking. More recently, AS01-induced antigen-specific CD8+ T cell responses were observed in studies of M72/AS01E and RZV [Citation68,Citation69,Citation104,Citation105] but not in studies of RSVPreF3-AS01 or RTS,S/AS01E [Citation39,Citation106]. In a study of an investigational AS01-containing HIV nanoparticle vaccine, CD8+ T cells responses were induced against the nanoparticle ‘scaffold’ but not the HIV antigen [Citation107]. Levels of neutralizing antibodies boosted after administration of RSVPreF3 were similar regardless of whether RSVPreF3 was administered alone or combined with AS01 [Citation39], whereas marked increases in antibody responses have been observed with other antigen-AS01 administrations compared to antigen alone. These observations highlight that the effect of adjuvantation with AS01 will vary depending on the vaccine antigen, the target pathogen and the vaccinees’ immune status with regards to the infection (naïve vs prime, latent vs recurrent infection).
As yet, the mechanisms underlying the reactogenicity of AS01 remain incompletely explained. IL-6 and IFNγ responses are linked to antibody and T cells responses to HBsAg/AS01 and also appear to be associated with systemic reactogenicity [Citation87]. While the data from the AS01-adjuvanted vaccines presented here appear to indicate that the adjuvant effect on the antigen-specific immune response is also associated with reactogenicity, the ‘no pain no gain’ hypothesis – that reactogenicity is a hallmark of a good adaptive immune response – has previously been shown to be inconsistent [Citation108]. As such, more work is needed to understand the mechanisms of reactogenicity and their relationship with the induction of protective immune responses.
AS01-licensed vaccines have so far been used for prophylaxis, yet there is a range of opportunities to be explored for AS01-based therapeutic vaccination that could provide further benefits to public health. For example, 2 clinical trials are investigating the use of for AS01-based therapeutic vaccines for the treatment of chronic hepatitis B (NCT03866187, NCT05276297). Chronic hepatitis B infection is characterized by dysfunctional and diminished CD4+ and CD8+ cells. It is thought that adjuvants could contribute to stimulation of CD4+ T cells and B cells, thereby helping to restore the immune response and promote viral clearance [Citation109]. In addition to the prevention of infectious disease, there is growing understanding that vaccines can have non-specific effects on the immune system unrelated to the disease they are designed to prevent [Citation110,Citation111]. When beneficial, these effects can reduce susceptibility to unrelated infections, impact the development of inflammation and cancer, slow or reverse age-related immune deterioration, and impact overall mortality. These benefits are thought to result from epigenetic reprogramming of innate immune cells, referred to as trained immunity [Citation110]. Recently, epigenetic reprogramming of monocytes by AS01//HBsAg vaccination have been described in human [Citation111]. How these effects might be leveraged to improve immune fitness and human health is a subject of intense investigation [Citation112].
As a result of the COVID-19 pandemic, there is now an array of new adjuvants and vaccine technologies such as viral vectors and mRNA vaccines, that are considered self-adjuvanted. Over the next 5 years we anticipate that significant work will be done to improve our understanding in 3 key areas. The first is understanding the nuances of individual antigen/adjuvant interactions that can guide selection of antigen and adjuvant combinations to obtain the desired immune response. This will also expand to comparisons of mRNA-lipid nanoparticles vs adjuvanted protein vaccination strategies, in the hope to select the best vaccine technology to achieve protective vaccination early on in vaccine development. The second is to explore and leverage the interaction between pre-existing immunity and adjuvant-induced immune modulation, and the third is to optimize dose and dose schedules in different populations. For example, AS01E used in the malaria, RSV, and investigational TB vaccines, contains half the amount of adjuvant components than AS01B used in RZV. A delayed fractional dose of RTS,S/AS01E improved vaccine efficacy in adults but not in children for reasons that are not clear, although the study settings were markedly different [Citation113,Citation114]. Dose and schedule optimization is also likely to minimize the reactogenicity experienced by vaccinees upon adjuvanted vaccination. Deep understanding of the different immune pathways driven by adjuvants in different age groups and populations with heterogenous prior immunological experiences could potentially allow the development of immunological models that could predict likely outcomes for different antigen-adjuvant combinations and schedules. This pathway has been explored in the development of therapeutics and vaccines for COVID-19 [Citation115]. Finally, data showing that older adults vaccinated with RZV had fewer and less severe COVID-19 infections [Citation116] is an intriguing finding that raises the question when AS01 could have a future role in promoting immune fitness. The impact of RZV on trained immunity is being explored in a phase I study (NCT04523246). The results of this and a range of other studies that are underway and investigating whether and how trained immunity can be leveraged to improve human health, could potentially expand the paradigm under which we currently view adjuvants and vaccines.
In summary, AS01 is one of several adjuvant systems being used to develop vaccines that are highly effective in populations with compromised immune systems, and against complex disease against which classical approaches were unsuccessful. The role of adjuvants, including AS01, in the prevention of human infectious diseases is expected to continue to expand into novel areas in the foreseeable future.
ARTICLE HIGHLIGHTS
Efficacy and acceptable safety profiles of AS01-containing tuberculosis, malaria, herpes zoster and respiratory syncytial virus vaccines have been demonstrated in clinical trials in multiple age groups and in post-marketing setting for the licensed AS01-containing vaccines.
The adjuvant effects of AS01 rely on the spatial and temporal co-localization of AS01 and the antigen. Shortly after injection, AS01 drains from the muscle to the proximal lymph node where rapid production of cytokines and influx of innate effector cells occur.
The downstream signaling activities of 3-O-desacyl-4’-monophosphoryl lipid A (MPL) and saponin QS-21 interact to drive the adjuvant effects of AS01. MPL and QS-21 share signaling pathways that promote complex signal integration, and together they activate distinct cell types, creating a network of innate effector functions.
The hallmark of the AS01 innate signature is the rapid production of interferon (IFN)-γ in the draining lymph node. AS01 also induces a core innate gene signature in the blood of vaccinees within 24 hours after vaccination, including genes related to the IFN pathway and natural killer (NK) cell-related functions. The core innate gene signature was associated with the magnitude of the adaptive response and could predict persistence of the humoral response in experimental trials.
Importantly, AS01 induced the core signature in most subjects, regardless of their underlying immune status. Thus, AS01 is capable of overcoming individual immune heterogeneity, reducing the impact of poor immune fitness on the vaccine response, even in older adults and immunocompromised.
AS01 induces T follicular helper cells, broad antibody responses with optimized functional characteristics and induction of immune memory, consistent induction of polyfunctional T cell responses in infants, older adults and the immunocompromised.
Declaration of Interests
All authors are employees of GSK and hold financial equities in GSK. The authors have no other relevant affiliations or financial involvement with any organization or entity with a financial interest in or financial conflict with the subject matter or materials discussed in the manuscript apart from those disclosed.
Trademarks
MF59 is a trademark owned by or licensed to Novartis AG. AS01and AS03, AREXVY, Mosquirix and Shingrix are trademarks owned by or licensed to GSK. Matrix-M is a trademark owned by or licensed to Novavax AB. Zostavax is a trademark of Merck Sharp & Dohme Corp
Contributorship
All authors were involved in the analysis and interpretation of the relevant literature and the development of the manuscript. All authors gave final approval before submission.
Acknowledgements
The authors thank Business and Decision Life Sciences Medical Communication Service Center for editorial assistance and manuscript coordination, on behalf of GSK. Joanne Wolter provided medical writing support. are generated with Scientific Image and Illustration Software | BioRender (https://www.biorender.com/).
Data Availability Statement
Data sharing is not applicable to this article as no new data were created or analyzed in this study.
Additional information
Funding
REFERENCES
- Marrack P, McKee AS, Munks MW. Towards an understanding of the adjuvant action of aluminium. Nat Rev Immunol. 2009;9:287–293. doi: 10.1038/nri2510
- Didierlaurent AM, Laupèze B, Di Pasquale A, et al. Adjuvant system AS01: helping to overcome the challenges of modern vaccines. Expert Rev Vaccines. 2017;16(1):55–63. doi: 10.1080/14760584.2016.1213632
- World Health Organisation. Malaria vaccine implementation programme [internet] [cited 2023 Mar 08] Available from: https://www.who.int/initiatives/malaria-vaccine-implementation-programme
- Praet N, Asante KP, Bozonnat MC, et al. Assessing the safety, impact and effectiveness of RTS,S/AS01E malaria vaccine following its introduction in three sub-Saharan African countries: methodological approaches and study set-up. Malar J. 2022;21(1):132. doi: 10.1186/s12936-022-04144-3
- World Health Organization. Full evidence report on the RTS,S/AS01 malaria vaccine. SAGE Yellow Book for October 2021. 5.1 malaria [internet] [cited 2022 Mar 08]. Available from: https://cdn.who.int/media/docs/default-source/immunization/mvip/full-evidence-report-on-the-rtss-as01-malaria-vaccine-for-sage-mpag-(sept2021).pdf?sfvrsn=c9737be_5
- Izurieta HS, Wu X, Forshee R, et al. Recombinant zoster vaccine (shingrix): real-world effectiveness in the first 2 years post-licensure. Clin Infect Dis. 2021;73(6):941–948. doi: 10.1093/cid/ciab125
- Lu A, Sun Y, Porco TC, et al. Effectiveness of the recombinant zoster vaccine for herpes zoster ophthalmicus in the United States. Ophthalmology. 2021;128(12):1699–1707. doi: 10.1016/j.ophtha.2021.04.017
- Mbinta JF, Nguyen BP, Awuni PMA, et al. Post-licensure zoster vaccine effectiveness against herpes zoster and postherpetic neuralgia in older adults: a systematic review and meta-analysis. Lancet Healthy Longev. 2022;3:e263–e275. doi: 10.1016/S2666-7568(22)00039-3
- Okoli GN, Al-Yousif Y, Reddy VK, et al. The number needed to vaccinate (NNV) against herpes zoster: a systematic review with meta-analysis. Infect Dis (Lond). 2022;54(5):356–366. doi: 10.1080/23744235.2021.2018493
- Sun Y, Jackson K, Dalmon CA, et al. Effectiveness of the recombinant zoster vaccine among kaiser permanente hawaii enrollees aged 50 and older: a retrospective cohort study. Vaccine. 2021;39(29):3974–3982. doi: 10.1016/j.vaccine.2021.05.056
- Sun Y, Kim E, Kong CL, et al. Effectiveness of the recombinant zoster vaccine in adults aged 50 and older in the United States: a claims-based cohort study. Clin Infect Dis. 2021;73(6):949–956. doi: 10.1093/cid/ciab121
- Xia Y, Zhang X, Zhang L, et al. Efficacy, effectiveness, and safety of herpes zoster vaccine in the immunocompetent and immunocompromised subjects: A systematic review and network meta-analysis. Front Immunol. 2022;13:978203. doi: 10.3389/fimmu.2022.978203
- Strezova A, Diez-Domingo J, Al Shawafi K, et al. Long-term protection against herpes zoster by the adjuvanted recombinant zoster vaccine: interim efficacy, immunogenicity, and safety results up to 10 years after initial vaccination. Open Forum Infect Dis. 2022;9(10):ofac485. doi: 10.1093/ofid/ofac485
- Garçon N, Di Pasquale A. From discovery to licensure, the Adjuvant System story. Hum. Vaccin. Immunother. 2017;13:19–33. doi: 10.1080/21645515.2016.1225635
- Fortpied J, Collignon S, Moniotte N, et al. The thermostability of the RTS,S/AS01 malaria vaccine can be increased by co-lyophilizing RTS,S and AS01. Malar J. 2020;19(1):202. doi: 10.1186/s12936-020-03253-1
- Didierlaurent AM, Morel S, Lockman L, et al. AS04, an aluminum salt- and TLR4 agonist-based adjuvant system, induces a transient localized innate immune response leading to enhanced adaptive immunity. J Immunol. 2009;183(10):6186–6197. doi: 10.4049/jimmunol.0901474
- Ishii KJ, Koyama S, Nakagawa A, et al.. Host innate immune receptors and beyond: making sense of microbial infections. Cell Host & Microbe. 2008;3(6):352–363. doi: 10.1016/j.chom.2008.05.003
- Molteni M, Gemma S, Rossetti C. The role of toll-like receptor 4 in infectious and noninfectious inflammation. Mediators Inflamm. 2016;2016:6978936. doi: 10.1155/2016/6978936
- Coler RN, Bertholet S, Moutaftsi M, et al. Development and characterization of synthetic glucopyranosyl lipid adjuvant system as a vaccine adjuvant. PLoS One. 2011;6(1):e16333. doi: 10.1371/journal.pone.0016333
- Mata-Haro V, Cekic C, Martin M, et al. The vaccine adjuvant monophosphoryl lipid a as a TRIF-biased agonist of TLR4. Science. 2007;316(5831):1628–1632. doi: 10.1126/science.1138963
- Welsby I, Detienne S, N’Kuli F, et al. Lysosome-dependent activation of human dendritic cells by the vaccine adjuvant QS-21. Front Immunol. 2016;7:663. doi: 10.3389/fimmu.2016.00663
- Detienne S, Welsby I, Collignon C, et al. Central role of CD169(+) lymph node resident macrophages in the adjuvanticity of the QS-21 component of AS01. Sci Rep. 2016;6:39475. doi: 10.1038/srep39475
- Coccia M, Collignon C, Herve C, et al. Cellular and molecular synergy in AS01-adjuvanted vaccines results in an early IFNγ response promoting vaccine immunogenicity. NPJ Vaccines. 2017;2(1):25. doi: 10.1038/s41541-017-0027-3
- Dendouga N, Fochesato M, Lockman L, et al. Cell-mediated immune responses to a varicella-zoster virus glycoprotein E vaccine using both a TLR agonist and QS21 in mice. Vaccine. 2012;30(20):3126–3135. doi: 10.1016/j.vaccine.2012.01.088
- Thaiss CA, Levy M, Itav S, et al. Integration of innate immune signaling. Trends Immunol. 2016;37(2):84–101. doi: 10.1016/j.it.2015.12.003
- Didierlaurent AM, Collignon C, Bourguignon P, et al. Enhancement of adaptive immunity by the human vaccine adjuvant AS01 depends on activated dendritic cells. J Immunol. 2014;193(4):1920–1930. doi: 10.4049/jimmunol.1400948
- Bosteels C, Fierens K, De Prijck S, et al. CCR2- and Flt3-Dependent inflammatory conventional type 2 dendritic cells are necessary for the induction of adaptive immunity by the human vaccine Adjuvant System AS01. Front Immunol. 2021;11:606805. doi: 10.3389/fimmu.2020.606805
- Palmer EM, van Seventer GA. Human T helper cell differentiation is regulated by the combined action of cytokines and accessory cell-dependent costimulatory signals. J Immunol. 1997;158(6):2654–2662. doi: 10.4049/jimmunol.158.6.2654
- Dutton EE, Gajdasik DW, Willis C, et al. Peripheral lymph nodes contain migratory and resident innate lymphoid cell populations. Sci Immunol. 2019;4(35):eaau8082. doi: 10.1126/sciimmunol.aau8082
- Van Maele L, Fougeron D, Cayet D, et al. Toll-like receptor 4 signaling in hematopoietic-lineage cells contributes to the enhanced activity of the human vaccine adjuvant AS01. Eur J Immunol. 2019;49(12):2134–2145. doi: 10.1002/eji.201948234
- Silva M, Kato Y, Melo MB, et al. A particulate saponin/TLR agonist vaccine adjuvant alters lymph flow and modulates adaptive immunity. Science Immunol. 2021;6:eabf1152. doi: 10.1126/sciimmunol.abf1152
- Burny W, Callegaro A, Bechtold V, et al. Different adjuvants induce common innate pathways that are associated with enhanced adaptive responses against a model antigen in humans. Front Immunol. 2017;8:943. doi: 10.3389/fimmu.2017.00943
- van den Berg RA, Coccia M, Ballou WR, et al. Predicting RTS,S vaccine-mediated protection from transcriptomes in a malaria-challenge clinical trial. Front Immunol. 2017;8:557. doi: 10.3389/fimmu.2017.00557
- Bradley LM, Dalton DK, Croft M. A direct role for IFN-gamma in regulation of Th1 cell development. J Immunol. 1996;157(4):1350–1358. doi: 10.4049/jimmunol.157.4.1350
- Schroder K, Hertzog PJ, Ravasi T, et al. Interferon-gamma: an overview of signals, mechanisms and functions. J Leukoc Biol. 2004;75:163–189. doi: 10.1189/jlb.0603252
- Leroux-Roels G, Marchant A, Levy J, et al. Impact of adjuvants on CD4(+) T cell and B cell responses to a protein antigen vaccine: results from a phase II, randomized, multicenter trial. Clin Immunol. 2016;169:16–27. doi: 10.1016/j.clim.2016.05.007
- De Mot L, Bechtold V, Bol V, et al. Transcriptional profiles of adjuvanted hepatitis B vaccines display variable interindividual homogeneity but a shared core signature. Sci Transl Med. 2020;12. doi: 10.1126/scitranslmed.aay8618
- Cunningham AL, Heineman TC, Lal H, et al. Immune responses to a recombinant glycoprotein E herpes zoster vaccine in adults aged 50 years or older. J Infect Dis. 2018;217(11):1750–1760. doi: 10.1093/infdis/jiy095
- Leroux-Roels I, Davis MG, Steenackers K, et al. Safety and immunogenicity of a respiratory syncytial virus prefusion F (RSVPreF3) candidate vaccine in older adults: phase 1/2 randomized clinical trial. J Infect Dis. 2023;227(6):761–772. doi: 10.1093/infdis/jiac327
- Coccia M, Burny W, Demoitie MA, et al. Subsequent AS01-adjuvanted vaccinations induce similar transcriptional responses in populations with different disease statuses. PLoS One. 2022;17(11):e0276505. doi: 10.1371/journal.pone.0276505
- Loos C, Coccia M, Didierlaurent AM, et al. Systems serology-based comparison of antibody effector functions induced by adjuvanted vaccines to guide vaccine design. NPJ Vaccines. 2023;8(1):34. doi: 10.1038/s41541-023-00613-1
- Budroni S, Buricchi F, Cavallone A, et al. Antibody avidity, persistence, and response to antigen recall: comparison of vaccine adjuvants. NPJ Vaccines. 2021;6(1):78. doi: 10.1038/s41541-021-00337-0
- Morrocchi E, van Haren S, Palma P, et al. Modeling human immune responses to vaccination in vitro. Trends Immunol. 2024;45(1):32–47. doi: 10.1016/j.it.2023.11.002
- Smith CL, Richardson B, Rubsamen M, et al. Adjuvant AS01 activates human monocytes for costimulation and systemic inflammation. Vaccine. 2024;42(2):229–238. doi: 10.1016/j.vaccine.2023.12.010
- World Health Organization. Malaria: The malaria vaccine implementation programme (MVIP). 2 March 2020. Q&A [internet] [cited 2022 Mar 08]. Available from:. https://www.who.int/news-room/questions-and-answers/item/malaria-vaccine-implementation-programme
- World Health Organization. Malaria vaccine: WHO position paper –March 2022. Wkly Epidemiol Rec. 2022;97 61–80.
- RTS,S Clinical Trials Partnership. Efficacy and safety of RTS,S/AS01 malaria vaccine with or without a booster dose in infants and children in Africa: final results of a phase 3, individually randomised, controlled trial. Lancet. 2015;386:31–45. doi: 10.1016/S0140-6736(15)60721-8
- European Medicines Agency. Mosquirix H-W-2300. Product information [internet] [cited 2024 Feb 16]. Available from: https://www.ema.europa.eu/en/glossary/product-information
- Dicko A, Ouedraogo JB, Zongo I, et al. Seasonal vaccination with RTS,S/AS01(E) vaccine with or without seasonal malaria chemoprevention in children up to the age of 5 years in Burkina Faso and Mali: a double-blind, randomised, controlled, phase 3 trial. Lancet Infect Dis. 2023;24:75–86. doi: 10.1016/S1473-3099(23)00368-7
- Centers for Disease Control and Prevention. Malaria. Appendix A: Malaria Lifecycle. [internet] [cited 2024 July 03]. Available from: https://www.cdc.gov/malaria/php/surveillance/appendix-a-malaria-lifecycle.html
- Beeson JG, Kurtovic L, Valim C, et al. The RTS,S malaria vaccine: Current impact and foundation for the future. Sci Transl Med. 2022;14(671):eabo6646. doi: 10.1126/scitranslmed.abo6646
- Ockenhouse CF, Regules J, Tosh D, et al. Ad35.CS.01 - RTS,S/AS01 heterologous prime boost vaccine efficacy against sporozoite challenge in healthy malaria-naïve adults. PLoS One. 2015;10(7):e0131571. doi: 10.1371/journal.pone.0131571
- Kazmin D, Nakaya HI, Lee EK, et al. Systems analysis of protective immune responses to RTS,S malaria vaccination in humans. Proc Natl Acad Sci USA. 2017;114:2425–2430. doi: 10.1073/pnas.1621489114
- Suscovich TJ, Fallon JK, Das J, et al. Mapping functional humoral correlates of protection against malaria challenge following RTS,S/AS01 vaccination. Sci Transl Med. 2020;12(553):12. doi: 10.1126/scitranslmed.abb4757
- Seaton KE, Spreng RL, Abraha M, et al. Subclass and avidity of circumsporozoite protein specific antibodies associate with protection status against malaria infection. NPJ Vaccines. 2021;6(1):110. doi: 10.1038/s41541-021-00372-x
- Pallikkuth S, Chaudhury S, Lu P, et al. A delayed fractionated dose RTS,S AS01 vaccine regimen mediates protection via improved T follicular helper and B cell responses. eLife. 2020;9. doi: 10.7554/eLife.51889
- Nielsen CM, Ogbe A, Pedroza-Pacheco I, et al. Protein/AS01B vaccination elicits stronger, more Th2-skewed antigen-specific human T follicular helper cell responses than heterologous viral vectors. Cell Rep Med. 2021;2:100207. doi: 10.1016/j.xcrm.2021.100207
- Young WC, Carpp LN, Chaudhury S, et al. Comprehensive data integration approach to assess immune responses and correlates of RTS,S/AS01-mediated protection from malaria infection in controlled human malaria infection trials. Front. Big Data. 2021;4:672460. doi: 10.3389/fdata.2021.672460
- Moris P, Jongert E, van der Most RG. Characterization of T-cell immune responses in clinical trials of the candidate RTS,S malaria vaccine. Hum Vaccin Immunother. 2018;14(1):17–27. doi: 10.1080/21645515.2017.1381809
- Cunningham AL, Lal H, Kovac M, et al. Efficacy of the herpes zoster subunit vaccine in adults 70 years of age or older. N Engl J Med. 2016;375(11):1019–1032. doi: 10.1056/NEJMoa1603800
- Lal H, Cunningham AL, Godeaux O, et al. Efficacy of an adjuvanted herpes zoster subunit vaccine in older adults. N Eng J Med. 2015;372:2087–2096. doi: 10.1056/NEJMoa1501184
- Shingrix Summary of Product Characteristics. [internet] [cited 2023 Sept 05]. Available from: https://www.ema.europa.eu/en/documents/product-information/shingrix-epar-product-information_en.pdf 2018
- Shingrix Prescribing Information [internet] [cited 2023 Sept 05]. Available from: https://www.fda.gov/media/108597/download
- Anderson TC, Masters NB, Guo A, et al. Use of recombinant zoster vaccine in immunocompromised adults aged >/=19 Years: recommendations of the Advisory Committee on Immunization Practices - United States, 2022. MMWR. 2022;71:80–84. doi: 10.15585/mmwr.mm7103a2
- Dagnew AF, Rausch D, Herve C, et al. Efficacy and serious adverse events profile of the adjuvanted recombinant zoster vaccine in adults with pre-existing potential immune-mediated diseases: a pooled post hoc analysis on two parallel randomized trials. Rheumatology (Oxford). 2021;60(3):1226–1233. doi: 10.1093/rheumatology/keaa424
- Hastie A, Catteau G, Enemuo A, et al. Immunogenicity of the adjuvanted recombinant zoster vaccine: persistence and anamnestic response to additional doses administered 10 years after primary vaccination. J Infect Dis. 2021;224(12):2025–2034. doi: 10.1093/infdis/jiaa300
- Chlibek R, Bayas JM, Collins H, et al. Safety and immunogenicity of an AS01-adjuvanted varicella-zoster virus subunit candidate vaccine against herpes zoster in adults ≥50 years of age. J Infect Dis. 2013;208:1953–1961. doi: 10.1093/infdis/jit365
- Weinberg A, Kroehl ME, Johnson MJ, et al. comparative immune responses to licensed herpes zoster vaccines. J Infect Dis. 2018;218(suppl_2):S81–S87. doi: 10.1093/infdis/jiy383
- Levin MJ, Kroehl ME, Johnson MJ, et al. Th1 memory differentiates recombinant from live herpes zoster vaccines. J Clin Invest. 2018;128(10):4429–4440. doi: 10.1172/JCI121484
- Laing KJ, Ford ES, Johnson MJ, et al. Recruitment of naive CD4+ T cells by the recombinant zoster vaccine correlates with persistent immunity. J Clin Invest. 2023. doi: 10.1172/JCI172634 133 23
- Park SY, Levin MJ, Canniff J, et al. Development of antibody-dependent cellular cytotoxicity in response to recombinant and live-attenuated herpes zoster vaccines. NPJ Vaccines. 2022;7(1):123. doi: 10.1038/s41541-022-00545-2
- Savic M, Penders Y, Shi T, et al. Respiratory syncytial virus disease burden in adults aged 60 years and older in high-income countries: a systematic literature review and meta-analysis. Influenza Other Respir Viruses. 2023;17(1):e13031. doi: 10.1111/irv.13031
- Ngwuta JO, Chen M, Modjarrad K, et al. Prefusion F-specific antibodies determine the magnitude of RSV neutralizing activity in human sera. Sci Transl Med. 2015;7:309ra162. doi: 10.1126/scitranslmed.aac4241
- Rossey I, McLellan JS, Saelens X, et al. Clinical potential of prefusion RSV F-specific antibodies. Trends Microbiol. 2018;26(3):209–219. doi: 10.1016/j.tim.2017.09.009
- Walsh EE, Peterson DR, Falsey AR. Risk factors for severe respiratory syncytial virus infection in elderly persons. J Infect Dis. 2004;189(2):233–238. doi: 10.1086/380907
- Falsey AR, Walsh EE. Relationship of serum antibody to risk of respiratory syncytial virus infection in elderly adults. J Infect Dis. 1998;177(2):463–466. doi: 10.1086/517376
- Looney RJ, Falsey AR, Walsh E, et al. Effect of aging on cytokine production in response to respiratory syncytial virus infection. J Infect Dis. 2002;185(5):682–685. doi: 10.1086/339008
- Cherukuri A, Patton K, Gasser RA, Jr., et al. Adults 65 years old and older have reduced numbers of functional memory T cells to respiratory syncytial virus fusion protein. Clin Vac Immunol. 2013;20:239–247. doi: 10.1128/CVI.00580-12
- Sacconnay L, De Smedt J, Rocha-Perugini V, et al. The RSVPreF3-AS01 vaccine elicits broad neutralization of contemporary and antigenically distant respiratory syncytial virus strains. Sci Transl Med. 2023;15(710):eadg6050. doi: 10.1126/scitranslmed.adg6050
- Papi A, Ison MG, Langley JM, et al. Respiratory syncytial virus prefusion F protein vaccine in older adults. N. Engl. J. Med. 2023;388:595–608. doi: 10.1056/NEJMoa2209604
- Ison MG, Papi A, Athan E, et al. Efficacy and safety of respiratory syncytial virus (RSV) prefusion F protein vaccine (RSVPreF3 OA) in older adults over 2 RSV seasons. Clin Infect Dis. 2024;78(6):1732–1744. doi: 10.1093/cid/ciae010
- US FDA approves GSK’s Arexvy, the world’s first respiratory syncytial virus (RSV) vccine for older adults. [03 May 2023, cited 2023 Jun 19]. Available from: https://www.gsk.com/en-gb/media/press-releases/us-fda-approves-gsk-s-arexvy-the-world-s-first-respiratory-syncytial-virus-rsv-vaccine-for-older-adults/
- European Medicines Agency. Arexvy. [cited 2023, Sept 23]. Available from: https://www.ema.europa.eu/en/medicines/human/EPAR/arexvy
- Press release. GSK’s Arexvy, the first respiratory syncytial virus (RSV) vaccine for older adults approved in Canada [internet] [cited 2023 Aug 04]. Available from: https://ca.gsk.com/en-ca/media/press-releases/gsk-s-arexvy-the-first-respiratory-syncytial-virus-rsv-vaccine-for-older-adults-approved-in-canada/
- Japan’s Ministry of Health, Labour and Welfare approves GSK’s Arexvy, the country’s first respiratory syncytial virus (RSV) vaccine for older adults [internet] [cited 2023 Sept 23]. Available from: https://www.gsk.com/en-gb/media/press-releases/japan-s-ministry-of-health-labour-and-welfare-approves-gsk-s-arexvy/
- Herve C, Laupeze B, Del Giudice G, et al. The how’s and what’s of vaccine reactogenicity. NPJ Vaccines. 2019;4(1):39. doi: 10.1038/s41541-019-0132-6
- Burny W, Marchant A, Herve C, et al. Inflammatory parameters associated with systemic reactogenicity following vaccination with adjuvanted hepatitis B vaccines in humans. Vaccine. 2019;37(14):2004–2015. doi: 10.1016/j.vaccine.2019.02.015
- Di Pasquale A, Preiss S, Tavares Da Silva F, et al. Vaccine adjuvants: from 1920 to 2015 and beyond. Vaccines (Basel). 2015;3:320–343. doi: 10.3390/vaccines3020320
- Baay M, Bollaerts K, Verstraeten T. A systematic review and meta-analysis on the safety of newly adjuvanted vaccines among older adults. Vaccine. 2018;36(29):4207–4214. doi: 10.1016/j.vaccine.2018.06.004
- RTS,S Clinical Trials Partnership. A phase 3 trial of RTS,S/AS01 malaria vaccine in African infants. N Eng J Med. 2012;367:2284–2295. doi: 10.1056/NEJMoa1208394
- RTS,S Clinical Trials Partnership. First results of phase 3 trial of RTS,S/AS01 malaria vaccine in African children. New Eng J Med. 2011;365:1863–1875. doi: 10.1056/NEJMoa1102287
- Guerra Mendoza Y, Garric E, Leach A, et al. Safety profile of the RTS,S/AS01 malaria vaccine in infants and children: additional data from a phase III randomized controlled trial in sub-Saharan Africa. Hum Vaccin Immunother. 2019;15(10):2386–2398. doi: 10.1080/21645515.2019.1586040
- Yihunie W, Kebede B, Tegegne BA, et al. Systematic review of safety of RTS,S with AS01 and AS02 Adjuvant Systems using data from randomized controlled trials in infants, children, and adults. Clin Pharmacol. 2023;15:21–32. doi: 10.2147/CPAA.S400155
- Schmader KE, Levin MJ, Chen M, et al. Impact of reactogenicity after two doses of recombinant zoster vaccine upon physical functioning and quality of life: an open phase III trial in older adults. J. Gerontol. A Biol Sci Med Sci. 2021;76:485–490. doi: 10.1093/gerona/glaa127
- Tavares-Da-Silva F, Co MM, Dessart C, et al. Review of the initial post-marketing safety surveillance for the recombinant zoster vaccine. Vaccine. 2020;38(18):3489–3500. doi: 10.1016/j.vaccine.2019.11.058
- Hesse EM, Shimabukuro TT, Su JR, et al. Postlicensure safety surveillance of recombinant zoster vaccine (Shingrix) - United States, October 2017-June 2018. MMWR. 2019;68:91–94. doi: 10.15585/mmwr.mm6804a4
- Lopez-Fauqued M, Co-van der Mee M, Bastidas A, et al. Safety profile of the adjuvanted recombinant zoster vaccine in immunocompromised populations: an overview of six trials. Drug Saf. 2021;44(7):811–823. doi: 10.1007/s40264-021-01076-w
- World Health Organisation. Fact sheet. Tuberculosis [internet] [updated 7 Nov 2023 cited 2023 Deb 16]. Available from https://www.who.int/news-room/fact-sheets/detail/tuberculosis 2023.
- Scriba TJ, Dinkele R, Warner DF, et al. Challenges in TB research. J Exp Med. 2022;219. doi: 10.1084/jem.20221334 12
- Stewart P, Patel S, Comer A, et al. Role of B vells in Mycobacterium tuberculosis infection. Vaccines (Basel). 2023;11. doi: 10.3390/vaccines11050955
- Tait DR, Hatherill M, Van Der Meeren O, et al. Final analysis of a trial of M72/AS01E vaccine to prevent tuberculosis. N Engl J Med. 2019;381(25):2429–2439. doi: 10.1056/NEJMoa1909953
- Ouaked N, Demoitie MA, Godfroid F, et al. Non-clinical evaluation of local and systemic immunity induced by different vaccination strategies of the candidate tuberculosis vaccine M72/AS01. Tuberculosis (Edinb). 2023;143:102425. doi: 10.1016/j.tube.2023.102425
- Leroux-Roels I, Forgus S, De Boever F, et al. Improved CD4+ T cell responses to mycobacterium tuberculosis in PPD-negative adults by M72/AS01 as compared to the M72/AS02 and Mtb72F/AS02 tuberculosis candidate vaccine formulations: a randomized trial. Vaccine. 2013;31(17):2196–2206. doi: 10.1016/j.vaccine.2012.05.035
- Penn-Nicholson A, Geldenhuys H, Burny W, et al. Safety and immunogenicity of candidate vaccine M72/AS01E in adolescents in a TB endemic setting. Vaccine. 2015;33(32):4025–4034. doi: 10.1016/j.vaccine.2015.05.088
- Day CL, Tameris M, Mansoor N, et al. Induction and regulation of T-cell immunity by the novel tuberculosis vaccine M72/AS01 in South African adults. Am J Respir Crit Care Med. 2013;188(4):492–502. doi: 10.1164/rccm.201208-1385OC
- Lalvani A, Moris P, Voss G, et al. Potent induction of focused Th1-type cellular and humoral immune responses by RTS,S/SBAS2, a recombinant Plasmodium falciparum malaria vaccine. J Infect Dis. 1999;180(5):1656–1664. doi: 10.1086/315074
- Cohen KW, De Rosa SC, Fulp WJ, et al. A first-in-human germline-targeting HIV nanoparticle vaccine induced broad and publicly targeted helper T cell responses. Sci Transl Med. 2023;15(697):eadf3309. doi: 10.1126/scitranslmed.adf3309
- Callegaro A, Burny W, Herve C, et al. association between immunogenicity and reactogenicity: a post hoc analysis of 2 phase 3 studies with the adjuvanted recombinant zoster vaccine. J Infect Dis. 2022;226(11):1943–1948. doi: 10.1093/infdis/jiab536
- Laupeze B, Vassilev V, Badur S. A role for immune modulation in achieving functional cure for chronic hepatitis B among current changes in the landscape of new treatments. Expert Rev Gastroenterol Hepatol. 2023;17(11):1135–1147. doi: 10.1080/17474124.2023.2268503
- Laupeze B, Del Giudice G, Doherty MT, et al. Vaccination as a preventative measure contributing to immune fitness. NPJ vaccines. 2021;6:93. doi: 10.1038/s41541-021-00354-z
- Smolen K, Bechtold V, Burny W, et al. AS01B-adjuvanted vaccine promotes functional and epigenetic modifications in monocytes. Presentation at: 5th International Symposium on Trained Immunity; 2023 May 29-31, Naples (Italy). Abstract E-Book [page 28]. Available from: https://www.innateimmunememory.org/images/Abstract%20International%20Symposium%20on%20Innate%20Immune%20Memory.pdf
- Dagenais A, Villalba-Guerrero C, Olivier M Trained immunity: A “new” weapon in the fight against infectious diseases. Front Immunol. 2023;14:1147476. doi: 10.3389/fimmu.2023.1147476
- Moon JE, Greenleaf ME, Regules JA, et al. A phase IIA extension study evaluating the effect of booster vaccination with a fractional dose of RTS,S/AS01E in a controlled human malaria infection challenge. Vaccine. 2021;39(43):6398–6406. doi: 10.1016/j.vaccine.2021.09.024
- Samuels AM, Ansong D, Kariuki SK, et al. Efficacy of RTS,S/AS01(E) malaria vaccine administered according to different full, fractional, and delayed third or early fourth dose regimens in children aged 5-17 months in Ghana and Kenya: an open-label, phase 2b, randomised controlled trial. Lancet Infect Dis. 2022;22:1329–1342. doi: 10.1016/S1473-3099(22)00273-0
- Desikan R, Padmanabhan P, Kierzek AM, et al. Mechanistic models of COVID-19: insights into disease progression, vaccines, and therapeutics. Int J Antimicrob Agents. 2022;60(1):106606. doi: 10.1016/j.ijantimicag.2022.106606
- Bruxvoort KJ, Ackerson B, Sy LS, et al. Recombinant adjuvanted zoster vaccine and reduced risk of COVID-19 diagnosis and hospitalization in older adults. J Infect Dis. 2021;225:1915–1922. doi: 10.1093/infdis/jiab633