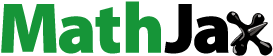
ABSTRACT
In volleyball, the overhead pass is an important skill for passing a ball and making a set to attackers. In the present study, we analysed the overhead pass motion and involved muscle activity with special attention to the stretch-shortening cycle (SSC). Electromyogram was recorded from the wrist and elbow joint flexors and extensors. The movement period when the ball touched the hand was divided into the pull and push phases with the moment when the ball was at the lowest position. In the pull phase, although the wrists were extended, the activity of wrist flexor muscle was higher than the extensor muscle in both skilled and unskilled groups. And the activity in the wrist flexor muscle in the pull phase was higher in the skilled participants than that of the unskilled participants. Thus, the skilled participants pushed the ball by utilising the SSC of the wrist, while the unskilled participants did this in a less degree. In addition, the kinetic chain was observed from elbow to wrist in the skilled participants. We conclude that it is important for an overhead pass that the wrist flexor muscles accumulate elastic energy during the pull phase which is then released in the push phase.
Introduction
In volleyball, the overhead pass is an important skill, both for passing the ball and for setting the ball for the attackers. Fine motor control is required for the involved sequence of movements. Nikos, Karolina, and Elissavet (Citation2009) noted that the higher the performance level of the setters, the better the execution of the attackers (Nikos et al., Citation2009). When performing an overhead pass, it is necessary for the players to control the appropriate distance and direction of a ball. These factors change depending upon the particular situation and can change in a very short time. Catching is illegal in volleyball, and thus the overhead pass does not involve simple ‘grasping and throwing’. According to Mary, Ridgway, & Jerry (Citation1987), the average time of ball contact during an overhead pass is 72 ms. Although an overhead pass does not require a high degree of power, it is repeated many times in games as well as training, so the pass should be as efficient as possible. Currently, the way to teach the skill of overhead pass is not necessarily evidence-based because the overhead pass motion has not yet been analysed, although other motion sequences in volleyball such as attacking, jumping, and blocking (Eom & Schutz, Citation1992; Ficklin, Lund, & Schipper, Citation2014; Hadzic, Sattler, Veselko, Markovic, & Dervisevic, Citation2014; Notarnicola et al., Citation2012). If the mechanism of an overhead pass was well described, it could provide players with a theoretical background which would aid in the development of this skilful motion.
Overhead pass is characterised by the movement in which the wrist is first dorsiflexed with a dropping ball and then flexed to push the ball. When a muscle is eccentrically contracted before the concentric contraction begins, the muscle can produce greater power than when there is only a concentric contraction (Cavagna, Saibene, & Margaria, Citation1965; Cavagna, Komarek, & Mazzoleni, Citation1971). This occurs because the elastic energy stored in the muscle and tendon during the eccentric contraction can be utilised (Bosco et al., Citation1982; Ishikawa & Komi, Citation2004; Norman & Komi, Citation1979; Padulo et al., Citation2013). Current research on the SSC has largely involved high power movements such as sprinting (Carr, McMahon, & Comfort, Citation2015), and the jumping (Bosco, Komi, & Ito, Citation1981; Hassani et al., Citation2013; Ishikawa & Komi, Citation2004), and has shown that utilisation of the SSC in the calf and thigh muscles is an effective method for producing considerable power (Baker & Nance, Citation1999; Bosco et al., Citation1981). Morse, Degens, Seynnes, Maganaris, and Jones (Citation2008) suggested that muscle-tendon unit stiffness is related to SSC performance (Morse et al., Citation2008). The muscle activity has a strong correlation with muscle stiffness (Leonard, Brown, Price, Queen, & Mikhailenok, Citation2004). While a large body of evidence supports the utility of the SSC in high power movements mostly in the lower limbs (Carr et al., Citation2015; Ishikawa & Komi, Citation2004; Ishikawa, Komi, Finni, & Kuitunen, Citation2006; Skurvydas, Dudoniene, Kalvenas, & Zuoza, Citation2002; Suchomel, Sole, & Stone, Citation2016), there is no information on SSC in the upper limbs for lower power movements and fine motor skills like the overhead pass. The main purpose of this study was to elucidate the movement sequences of this important skill in volleyball and to use this information in an analysis of the biomechanical differences between skilled and unskilled participants. The hypothesis was that utilisation of the SSC would lead to a better performance of the overhead pass in the skilled participants.
Methods
Participants
Twenty male participants participated in this study. Their characteristics are shown in . Half of them were top-level university volleyball players (skilled). The other half had no experience with volleyball (unskilled). All of them were right-handed according to the Edinburgh Inventory (Oldfield, Citation1971). In accordance with the Declaration of Helsinki, the experimental procedure was explained to all participants and each participant signed a written informed consent. The study was approved by the ethics committee of Tokai University.
Table 1. Characteristics of participants and number of attempts. The position: L, Libero; MB, Middle Blocker; S, Setter; and WS, Wing Spiker. *, significantly lower than the unskilled group (p < 0.001). Skilled.
Procedure
Participants were first told to do a warm-up at their own pace, This included performing some trials of the experimental task. Participants were told to stand still and then to pass a ball with an overhead pass through a ring (diameter: 1 m, height: 2.43 m) which was set 6 m directly in front of them (). A custom-made ball launching machine located halfway between the target ring and the participant was used to supply the participant with a ball. If the propelled ball missed the ring, the trial was not included in the biomechanical analysis. Participants repeated the attempts until they succeeded in passing a ball through the ring. The number of trials required for success was utilised as an index of performance.
Measurements
Sixteen reflective markers were placed on the participant in order to calculate the joint centre and joints angles. The positions of markers on the body were: shoulder (acrominon) × 2, upper arm (between the shoulder and elbow for offset) × 2, elbow (humeral lateral epicondyle and medial epicondyle) × 4, forearm (the place between the elbow and wrist for offset) × 2, wrist (styloid process of radius and ulna) × 4, and hand (the third metacarpophalangeal joint of the hands) × 2. Five reflective markers on the ball were attached and four them were used to calculate the ball centre; the remaining one was used for offset. The four ball markers were decided by utilising two pairs of two points forming a line segment passing through the volleyball centre. The fifth marker was offset marker. And the offset marker was utilised for filling of gap markers and identifing all the other markers. So the offset marker was not utilised for calculating the ball centre and it is placed on the asymmetrical place on the ball. A three-dimensional automatic digitising system (Mac3D, Motion Analysis Co., USA; 10 cameras, sampling frequency: 250 Hz, shutter speed: 1/500 s) was used to quantify the motion of each point. The global coordinate system was determined with X, Y and Z axes, where the X-axis was directed from the participant to the target, the Y-axis was at a right angle in the horizontal plane, and the Z-axis denoted the vertical direction.
The elbow and wrist joint angles (θ) were obtained by the dot product. For the elbow joint, vector x was that from the elbow to the shoulder, and vector y was that from the elbow to the wrist. For the wrist joint, vector x was from the wrist to the elbow, and vector y was from the wrist to the hand. Where the wrist angle was greater than 180 degrees, the location was determined by observing the data. The angles were expressed mathematically as:
We collected electromyographic (EMG) data from the number of surface muscles on the right arm; Biceps Brachii (BB), Triceps Brachii (TB), Flexor Carpi Radialis (FCR), and Extensor Carpi Ulnalis (ECU). The recording from each area was accomplished with a wired bipolar electrode set (DL-141, S&ME Company, Japan) with a 12 mm separation. Sampling frequency for the EMG recordings was 1000 Hz. Before attaching the electrodes, the skin was shaved and scrubbed to make a good electrical connection and to minimise the introduction of noise. Motion and EMG data were fed into a personal computer (Endeavor, EPSON Company, Japan) and could be synchronised using a known constant delay. At the end of each experimental session, we recorded EMG data for more than 3 s isometric maximum voluntary contraction (MVC).
Data analysis
Division of phases
The main phase of passing action was defined as the period from ball touch to ball release. Ball touch and release were detected from tracking data of the ball. First, ball acceleration in the Z-axis was obtained. The time of ball touch was defined as when the acceleration started to change from −9.8 m/s2. Ball release was defined as when the acceleration returned to −9.8 m/s2. The main phase of the overhead pass motion was further divided into pull and push phases as defined. ‘Ball catch’, defined as the point when the ball was at its lowest position in the main phase, divides the pull and push phases. The duration of the main phase was standardised as 100% to compare motions in the skilled and unskilled groups ().
Figure 2. Definition of pull and push phases The solid and broken lines are ball position (above) and ball acceleration (below). The shaded zone is the main phase. “Ball Touch” and “Ball Release” are defined as the moment when ball acceleration starts to increase from and returns to -9.8 (m/s2), respectively. “Ball Catch” is the moment when the ball position is at its lowest
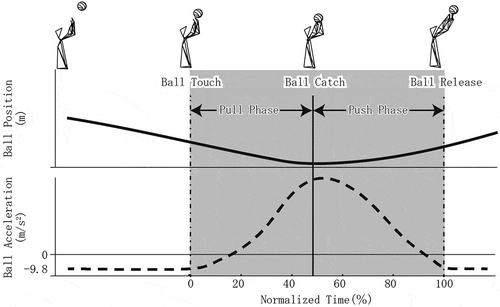
A Butterworth low-pass filter set at 10 Hz was used to remove high-frequency random interference on marker positions. Angular velocities were calculated by differentiating the involved angles.
EMG data were passed through a Butterworth band-pass-filter with a 10–500 Hz bandwidth in order to remove high-frequency random interference and motion artefacts of low frequency. The signal was then rectified. The EMG data for each muscle were standardised as %EMG using the value of the MVC. This can be expressed mathematically as:
where EMCt is the EMG data at time t, and EMGMVC is the averaged MVC EMG over the 3 s period according to Philipou et al. (Citation2009).
Then, we calculated the percent root-mean-square value (%RMS); this value has been related to constant force for a non-fatiguing contraction (Phinyomark, Hirunviriya, Limsakul, & Phukpattaranont, Citation2010). %RMS is expressed as:
The actual calculations were performed using Mathematica (Wolfram, America).
Data in skilled and unskilled groups were compared with unpaired non-parametric Mann–Whitney test. Right and left arms angle and angular velocity were compared using Two-way repeated measures analysis of variance. The significant level was at p < 0.05.
Results
The average number of trials that the skilled group took to succeed in passing a ball into the ring was significantly lower than that of the unskilled group (, p = 0.00001). There was no significant difference in height between skilled and unskilled groups (p= 0.234) While four skilled participants succeeded in the first trial, no unskilled participant accomplished this. The duration of the main phase for all the participants was 87 ± 23 ms and was not statistically different for the skilled and unskilled groups. The timing of ‘ball catch’ (48 ± 7%) in the main phase was also not statistically different for the two groups. There was no significant difference between angles and angular velocities of right and left arms in the main phase.
depicts typical examples of the raw-data for joint movement and EMG activity during an overhead pass for a skilled and an unskilled participant. shows group data for elbow angular velocity and angle as well as for activity of the BB and TB, and in the main phase. There was no significant difference in elbow angle between skilled and unskilled groups ()). At the start of the main phase, angular velocity has a positive value in both groups ()). This indicates that when the ball touched the participant’s hands the elbow had already started to extend. In the skilled group, the angular velocity had a peak at 60 ~ 80% of the normalised time, while in the unskilled group the angular velocity continued to increase during the entire main phase. The rate of the increase for the unskilled group did slowdown in the push phase. In the pull phase, the magnitude of TB activity was large for both groups (47 ± 27%), while BB activity was small for both (4 ± 3%, )). There was no statistical difference between the groups for either TB or BB activity in the pull phase. During the push phase, TB activity in the skilled group (16 ± 10%) was significantly lower than that of the unskilled group (31 ± 12%, ), p = 0.009).
Figure 3. Typical raw data involving changes in the joint angles and EMGs of a skilled participant (A) and an unskilled participant (B). In the motion recordings, solid lines show data from the right arm, and broken lines show that of the left arm. The vertical lines depict ball touch (left) and ball release (right). The data involving the wrist are shaded. BB: Biceps Brachii, TB: Triceps Brachii, FCR: Flexor Carpi Radialis ECU: Extensor Carpi Ulnalis
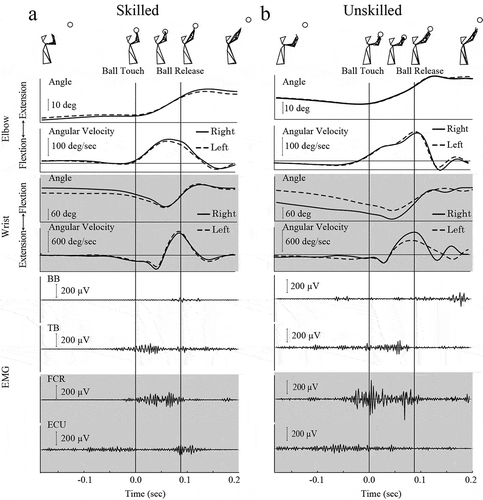
Figure 4. Motion and EMG activity of the elbow Graph A shows elbow angular velocity and angle data against the main phase means normalised for time in the main phase. The solid lines and closed circles depict the skilled group and the broken lines and open circles the unskilled group. Solid and broken vertical lines denote the mean times of ball catch in the skilled and the unskilled groups, respectively. Sections B and C illustrate activities of BB and TB in the pull phase (B), and in the push phase (C). The black and white bars delineate skilled and unskilled groups, respectively
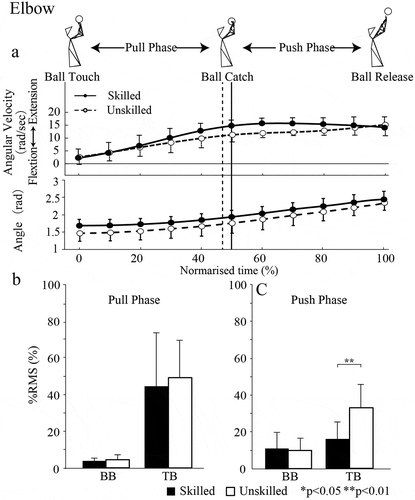
shows group data for wrist angular velocity and activities of the FCR and ECU in the main phase. There was no significant difference in wrist angle between skilled and unskilled groups ()). Both groups had already started to extend the wrist when the ball touched the participants’ hands ()). Before the ball catch, the wrist joint angle remained extended, and after the ball catch, the movement changed from extension to flexion at around 60% of the normalised time ()). The FCR had high activity in the pull phase for both groups ()). FCR activity in the skilled group was significantly higher than in the unskilled group (), p = 0.028). ECU activity did not differ between both groups in the pull phase nor in the push phase.
Figure 5. Motion and EMG activity of the wrist Graph A: shows wrist angular velocity and angle data against the main phase means normalised for time. The solid lines and closed circles denote the skilled group and the broken lines and open circles the unskilled group. Solid and broken vertical lines depict the mean times of ball catch in the skilled and the unskilled groups, respectively. Sections B and C illustrate activities of FCR and ECU in the pull phase (B), and in the push phase (C). The black and white bars delineate skilled and unskilled groups, respectively
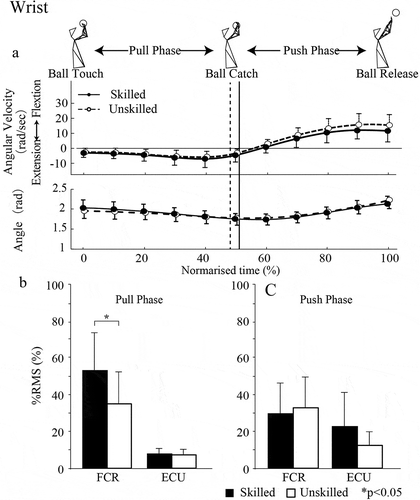
Discussion and implications
Although an overhead pass is the standard method for ‘setting’ or passing a volleyball, it is in one sense a somewhat unique motion in that it does not involve a grasp (catch) and subsequent throw. A detailed biomechanical analysis of this important skill has not been done. The main purpose of the present study was to elucidate the movement sequences of this important skill and to use this information in an analysis of the biomechanical differences between skilled and unskilled participants.
The skilled participants were able to successfully ‘set’ a volleyball through a target ring with less attempts than were the unskilled participants (). Four of the skilled participants succeeded in the first attempt, while all unskilled participants needed from four to thirteen attempts to succeed. Clearly, the skilled participants were more proficient at the overhead pass than the unskilled ones. There was no significant difference between right and left arm movements changes in angles and angular velocities in the main phase even in the unskilled group. Therefore, the symmetry of movement and EMG activity was likely to be maintained although EMG data were recorded from only right hand. There was also no significant differences in both wrist and elbow motion between skilled and unskilled groups. This would be because the analysis was made only for the succeeded trials in both groups, probably resulting in very similar ball trajectory, and does not indicate that there was no difference in the skilled of an overhead pass between the groups.
Motion and EMG during the pull phase
In the pull phase, the activity of FCR exhibited statistical differences between the skilled and unskilled groups ()). Thus, there are likely to be some distinctive characteristics in the wrist function for the pull phase of the skilled participants. On the other hand, there was no difference in the elbow motion nor in EMG in the pull phase (). Thus, during the pull phase, both groups resisted to the dropped ball and pushed it by elbow extension in a similar way.
Although both groups extended the wrist during the pull phase, the FCR showed a higher level of EMG activity () in the skilled participants. That is, the FCR showed a stronger eccentric contraction in this phase, and after the ball catch, the FCR switched to a concentric contraction. This indicates that in the overhead pass motion a stretch-shortening cycle (SSC) functions in the FCR. Many researches have been done on the SSC during motions that involve high power mainly in the lower limbs (Carr et al., Citation2015; Harrison, Keane, & Coglan, Citation2004; Hassani et al., Citation2013; Ishikawa & Komi, Citation2004; Levenez et al., Citation2013; Norman & Komi, Citation1979; Serrien & Baeyens, Citation2017; Skurvydas et al., Citation2002; Suchomel et al., Citation2016). However, although there are several studies on SSC during low power motions, the studies did not focus on the movement using the upper arms (Fukunaga et al., Citation2001; Lloyd, Oliver, Hughes, & Williams, Citation2012; Yaghoubi, Esfehani, Hosseini, Alikhajeh, & Shultz, Citation2015). Thus, the SSC during overhead pass is the first finding of SSC utilisation in a quickly switching motion that involves low power. Harrison et al. (Citation2004) and Horita et al. (1996) suggested that the effects of SSC function increased with the increases in leg stiffness. In their study, the leg stiffness was assessed from the torque data but the increases in the torque should accompany the increase in muscle activity because muscle activity has strong correlation with muscle stiffness (Leonard et al., Citation2004). Likewise, because the FCR activity in the skilled group in the present study was higher than that of the unskilled group, the skilled group should have greater wrist stiffness, and the SSC of the FCR would function more effectively than the unskilled group. That is, the skilled group was able to utilise the stored elastic energy more efficiently than was the unskilled group.
Motion and EMG during the push phase
In the push phase, there was no statistical significance between the groups in the motion of the elbow and wrist joints (). A higher TB activity in the unskilled group ()) indicates that they used the elbow joint more strongly than did the skilled group. On the other hand, the skilled group would have been able to use the elastic energy of the FCR that was accumulated during the pull phase. This is an important overhead pass capability that the skilled group had developed. In a previous study, the activity of the gastrocnemius during the push off of drop jump in quick drop jump group was shown to be higher than slow drop jump group (Bobbert, Mackay, Schinkelshoek, Huijing, & van Ingen Schenau, Citation1986). The function of the wrist joint during the overhead pass is closed to that of the ankle joint during drop jump. The unskilled group would provide the supplementary energy needed for pushing the ball by elbow extension since they could not accumulate sufficient elastic energy in the wrists.
Kinetic link in the overhead pass
Generally, for a skilled throw-like motion such as pitching (Seroyer et al., Citation2010), kicking (Chapman & Sanderson, Citation1990), and a push-like motion such as shot put (Coh, Stuhec, & Supej, Citation2008), the peak velocity appears, in the order, from the proximal to the distal joints. This leads to an efficient flow of energy, which has been termed the kinetic chain (Kreighbaum & Barthels, Citation1996). In the present study, the peak angular velocity for elbow joint in the skilled group occurred at 60–80% of the normalised time, while the angular velocity in the unskilled group kept increasing until the end of the push phase ()). On the other hand, wrist joint angular velocity peaked at 90% of the normalised time for both groups ()). Thus, while the skilled group demonstrated a kinetic chain-like order of peaks in angular velocity (first the elbow then the wrist) (Chapman & Sanderson, Citation1990), the unskilled group did not. This suggests that the unskilled participants did not utilise the kinetic chain, and would thus suffer an inevitable energy loss. In addition, Chapman and Sanderson (Citation1990) suggested utilising the kinetic chain enables the SSC of muscles effectively to work. The overhead pass in the skilled players would be done by efficient utilisation of kinetic chain with the SSC in the wrist.
The present study revealed that the skilled participants pushed the ball by wrist flexion with the SSC, but unskilled participants did that in a less degree. When unskilled novices are coached for the overhead pass, the importance of the spring function of the wrist should be emphasised. For example, it would be effective to let unskilled novices practice the overhead pass with the elbows kept at the fully extended position so that they cannot use the elbow.
Limitations
We restricted our analysis to an overhead pass to propel a volleyball through a ring 6 m from the participants. Thus, the mechanisms involved in controlling the distance should be analysed in future studies, utilising targets set at various distances from the participants. We also analysed only one successful attempt, but the accuracy of the movement should also be verified by analysing multiple trials including failed ones.
In volleyball overhead pass the fingers should work to push and control a ball. In the present research, however, we analysed only the movements of wrist and elbow joints because finger movements are too complex and small with many muscles involved. To draw a conclusion on the role of SSC, future studies concerning the behaviour of muscle-tendon unit of the wrist is necessary. And we could only suggest that the SSC was utilised in wrist movement during an overhead pass from motion and EMG data.
Conclusion
In summary, we clarified biomechanical characteristics of the overhead pass in skilled volleyball players by comparing the joint motion and EMG activity in skilled and unskilled participants. Ball propulsion by the skilled participants included elastic energy from the wrist which was stored during the pull phase. Moreover, a kinetic chain was observed – sequential development of joint angular velocity from the elbow to the wrist – only in the skilled participants. Consequently, to develop an efficient, skilful overhead pass, it is important that the wrist flexors accumulate elastic energy during the pull phase. This energy can then be released in the push phase.
Acknowledgments
The authors wish to thank the players who volunteered to participate in this study, as well as their coaches for allowing usage of the data. Especially, we are thankful for the cooperation of Prof. Tsumiyama, who is the head coach of the Tokai University Volleyball team. We also thank Dr Larry Crawshaw for editing the English in the manuscript.
Disclosure statement
No potential conflict of interest was reported by the authors.
References
- Baker, D., & Nance, S. (1999). The relation between running speed and measures of strength and power in professional rugby league players. The Journal of Strength & Conditioning Research, 13, 230–235. doi:https://doi.org/10.1519/00124278-199908000-00009
- Bobbert, M. F., Mackay, M., Schinkelshoek, D., Huijing, P., & van Ingen Schenau, G. (1986). Biomechanical analysis of drop and countermovement jumps. European Journal of Applied Physiology and Occupational Physiology, 54, 566–573. doi:https://doi.org/10.1007/BF00943342
- Bosco, C., Ito, A., Komi, P., Luhtanen, P., Rahkila, P., Rusko, H., & Viitasalo, J. (1982). Neuromuscular function and mechanical efficiency of human leg extensor muscles during jumping exercises. Acta Physiologica, 114, 543–550. doi:https://doi.org/10.1111/j.1748-1716.1982.tb07022.x
- Bosco, C., Komi, P. V., & Ito, A. (1981). Prestretch potentiation of human skeletal muscle during ballistic movement. Acta Physiologica, 111, 135–140. doi:https://doi.org/10.1111/j.1748-1716.1981.tb06716.x
- Carr, C., McMahon, J. J., & Comfort, P. (2015). Relationships between jump and sprint performance in first-class county cricketers. Journal of Trainology, 4, 1–5. doi:https://doi.org/10.17338/trainology.4.1_1
- Cavagna, G., Saibene, F., & Margaria, R. (1965). Effect of negative work on the amount of positive work performed by an isolated muscle. Journal of Applied Physiology, 20, 157–158. doi:https://doi.org/10.1152/jappl.1965.20.1.157
- Cavagna, G. A., Komarek, L., & Mazzoleni, S. (1971). The mechanics of sprint running. The Journal of Physiology, 217, 709–721.
- Chapman, A. E., & Sanderson, D. J. (1990). Muscular coordination in sporting skills. In Winters, Jack, M., Woo, Savio, L-Y. (Eds.), Multiple muscle systems (pp. 608–620). Springer.
- Coh, M., Stuhec, S., & Supej, M. (2008). Comparative biomechanical analysis of the rotational shot put technique. Collegium antropologicum, 32, 249–256.
- Eom, H. J., & Schutz, R. W. (1992). Statistical analyses of volleyball team performance. Research Quarterly for Exercise and Sport, 63, 11–18. doi:https://doi.org/10.1080/02701367.1992.10607551
- Ficklin, T., Lund, R., & Schipper, M. (2014). A comparison of jump height, takeoff velocities, and blocking coverage in the swing and traditional volleyball blocking techniques. Journal of Sports Science & Medicine, 13, 78.
- Fukunaga, T., Kubo, K., Kawakami, Y., Fukashiro, S., Kanehisa, H., & Maganaris, C. N. (2001). In vivo behaviour of human muscle tendon during walking. Proceedings of the Royal Society of London B: Biological Sciences, 268, 229–233. doi:https://doi.org/10.1098/rspb.2000.1361
- Hadzic, V., Sattler, T., Veselko, M., Markovic, G., & Dervisevic, E. (2014). Strength asymmetry of the shoulders in elite volleyball players. Journal of Athletic Training, 49, 338–344. doi:https://doi.org/10.4085/1062-6050-49.2.05
- Harrison, A. J., Keane, S. P., & Coglan, J. (2004). Force-velocity relationship and stretch-shortening cycle function in sprint and endurance athletes. The Journal of Strength & Conditioning Research, 18, 473–479. doi:https://doi.org/10.1519/13163.1
- Hassani, A., Kotzamanidou, M. C., Fotiadou, E., Patikas, D., Evagelinou, C., & Sakadami, N. (2013). Neuromuscular differences between boys with and without intellectual disability during squat jump. Research in Developmental Disabilities, 34, 2856–2863. doi:https://doi.org/10.1016/j.ridd.2013.05.046
- Ishikawa, M., & Komi, P. V. (2004). Effects of different dropping intensities on fascicle and tendinous tissue behavior during stretch-shortening cycle exercise. Journal of Applied Physiology, 96, 848–852. doi:https://doi.org/10.1152/japplphysiol.00948.2003
- Ishikawa, M., Komi, P. V., Finni, T., & Kuitunen, S. (2006). Contribution of the tendinous tissue to force enhancement during stretch–Shortening cycle exercise depends on the prestretch and concentric phase intensities. Journal of Electromyography and Kinesiology, 16, 423–431. doi:https://doi.org/10.1016/j.jelekin.2005.08.006
- Kreighbaum, E., & Barthels, K. M. (1996). Biomechanics: A qualitative approach for studying human movement (pp. 338–369). Allyn and Bacon.
- Leonard, C. T., Brown, J. S., Price, T. R., Queen, S. A., & Mikhailenok, E. L. (2004). Comparison of surface electromyography and myotonometric measurements during voluntary isometric contractions. Journal of Electromyography and Kinesiology, 14, 709–714. doi:https://doi.org/10.1016/j.jelekin.2004.06.001
- Levenez, M., Theunissen, S., Bottero, A., Snoeck, T., Bruyère, A., Tinlot, A., … Provyn, S. (2013). The effect of a passive stretch training protocol on performance during a drop jump in humans. The Journal of Sports Medicine and Physical Fitness, 53, 319–326.
- Lloyd, R. S., Oliver, J. L., Hughes, M. G., & Williams, C. A. (2012). Age-related differences in the neural regulation of stretch–Shortening cycle activities in male youths during maximal and sub-maximal hopping. Journal of Electromyography and Kinesiology, 22, 37–43. doi:https://doi.org/10.1016/j.jelekin.2011.09.008
- Mary, E. Ridgway, & Jerry, W. (1987). A kinemamtic analysis of the front set and back set in volleyball. In Biomechanics in sport, ⅲ and ⅳ, (pp. 240–248).
- Morse, C. I., Degens, H., Seynnes, O. R., Maganaris, C. N., & Jones, D. A. (2008). The acute effect of stretching on the passive stiffness of the human gastrocnemius muscle tendon unit. The Journal of Physiology, 586, 97–106. doi:https://doi.org/10.1113/jphysiol.2007.140434
- Nikos, B., Karolina, B., & Elissavet, N. M. (2009). Performance of male and female setters and attackers on Olympic-level volleyball teams. International Journal of Performance Analysis in Sport, 9, 141–148. doi:https://doi.org/10.1080/24748668.2009.11868470
- Norman, R. W., & Komi, P. V. (1979). Electromechanical delay in skeletal muscle under normal movement conditions. Acta Physiologica, 106, 241–248. doi:https://doi.org/10.1111/j.1748-1716.1979.tb06394.x
- Notarnicola, A., Fischetti, F., Gallone, D., Moretti, L., Pignataro, P., Tafuri, S., & Moretti, B. (2012). Overload and neovascularization of shoulder tendons in volleyball players. BMC Research Notes, 5, 397. doi:https://doi.org/10.1186/1756-0500-5-397
- Oldfield, R. C. (1971). The assessment and analysis of handedness: The Edinburgh inventory. Neuropsychologia, 9, 97–113. doi:https://doi.org/10.1016/0028-3932(71)90067-4
- Padulo, J., Tiloca, A., Powell, D., Granatelli, G., Bianco, A., & Paoli, A. (2013). EMG amplitude of the biceps femoris during jumping compared to landing movements. Springerplus, 2, 520. doi:https://doi.org/10.1186/2193-1801-2-520
- Philippou, A., Maridaki, M., Bogdanis, G., Halapas, A., & Koutsilieris, M. (2009). Changes in the mechanical properties of human quadriceps muscle after eccentric exercise. In Vivo, 23, 859–865.
- Phinyomark, A., Hirunviriya, S., Limsakul, C., & Phukpattaranont, P. (2010). Evaluation of EMG feature extraction for hand movement recognition based on Euclidean distance and standard deviation. Paper presented at the Electrical Engineering/Electronics Computer Telecommunications and Information Technology (ECTI-CON), 2010 International Conference on, Chiang Mai, Thailand. 856–860.
- Seroyer, S. T., Nho, S. J., Bach, B. R., Bush-Joseph, C. A., Nicholson, G. P., & Romeo, A. A. (2010). The kinetic chain in overhand pitching: Its potential role for performance enhancement and injury prevention. Sports Health, 2, 135–146. doi:https://doi.org/10.1177/1941738110362656
- Serrien, B., & Baeyens, J.-P. (2017). The proximal-to-distal sequence in upper-limb motions on multiple levels and time scales. Human Movement Science, 55, 156–171. doi:https://doi.org/10.1016/j.humov.2017.08.009
- Skurvydas, A., Dudoniene, V., Kalvenas, A., & Zuoza, A. (2002). Skeletal muscle fatigue in long‐distance runners, sprinters and untrained men after repeated drop jumps performed at maximal intensity. Scandinavian Journal of Medicine & Science in Sports, 12, 34–39. doi:https://doi.org/10.1034/j.1600-0838.2002.120107.x
- Suchomel, T. J., Sole, C. J., & Stone, M. H. (2016). Comparison of methods that assess lower-body stretch-shortening cycle utilization. The Journal of Strength & Conditioning Research, 30, 547–554. doi:https://doi.org/10.1519/JSC.0000000000001100
- Yaghoubi, M., Esfehani, M. M., Hosseini, H. A., Alikhajeh, Y., & Shultz, S. P. (2015). Comparative electromyography analysis of the upper extremity between inexperienced and elite water polo players during an overhead shot. Journal of Applied Biomechanics, 31, 79–87. doi:https://doi.org/10.1123/jab.2014-0068