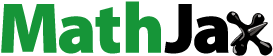
ABSTRACT
This study describes asymmetry in the main running mechanical variables during repeated treadmill sprints in elite female athletes and examines whether inter-limb differences in sprinting mechanics increase with fatigue. Eighteen elite female players (French national Rugby Sevens team) performed 8 × 5-s sprints (25-s rest) on an instrumented motorised sprint treadmill. The group mean ‘symmetry angle’ (SA) scores were ~1–2% for contact time (1.6 ± 0.6%), aerial time (2.1 ± 0.8%), step frequency (1.3 ± 0.5%) and step length (1.6 ± 0.6%). Mean vertical and horizontal forces, vertical and leg stiffness presented SA values of 1.7 ± 1.5%, 2.4 ± 1.2%, 2.6 ± 0.2% and 2.5 ± 0.2%, respectively. The SA scores were ~2–8% for duration of braking (6.9 ± 5.0%) and propulsive (6.5 ± 4.4%) phases, peak braking (6.5 ± 2.5%) and propulsive (1.6 ± 0.9%) forces as well as net (5.8 ± 5.6%), braking (7.7 ± 5.3%) and propulsive (2.7 ± 1.6%) impulses. However, there was no influence of sprint repetition number on SA scores for tested variables (P > 0.05). In elite female Rugby Sevens players, there was no noticeable difference in asymmetries for the great majority of stride mechanical variables during repeated treadmill sprints.
Introduction
The ability to repeat very short (<6 s) ‘all out’ efforts interspersed with incomplete (<30 s) recoveries during intense periods of a game is crucial in many team sports. From a biomechanical viewpoint, performance decrement during repeated-sprint exercise (RSE) is associated with substantial alterations in stride variables (i.e., longer contact time, slower step frequency) and spring-mass characteristics (i.e., decreased vertical stiffness) (Girard et al., Citation2017; Morin et al., Citation2011). However, changes in braking and/or propulsive forces have never been explored during RSE. This information would be helpful to understand, when fatigue develops during RSE, whether athletes slow down by reducing propulsive force in the direction of the movement as opposed to braking more in the early stance phase (Morin et al., Citation2015).
Measuring biomechanical locomotor asymmetry in the lower extremity has proven to be relevant for athletic populations because excessive inter-limb differences may decrease physical performance (Bishop et al., Citation2018) and/or be indicative of injury risk (Jacobs et al., Citation2005). Fatigue can increase knee joint asymmetries (i.e., knee internal rotation and knee stiffness) (Radzak et al., Citation2017), while studies identifying functional deficiencies during sport-specific actions are rare (Girard et al., Citation2017). The ‘Symmetry Angle’ (SA) is a dimensionless measure of asymmetry (Zifchock et al., Citation2008). The SA is calculated by plotting a measure for the right side against a measure for the left side. A vector line is drawn from this point through the intersection of the x and y axes, and the angle with respect to the x-axis is calculated. Two identical values will create a 45° angle, indicating perfect symmetry. The vector angle can be converted to a percentage, with SA values ranging from 0% (no asymmetry) to 100% (perfect asymmetry) (Zifchock et al., Citation2008). One advantage is that it does not suffer from artificial inflation, unlike other options (i.e., limb symmetry index, bilateral strength asymmetry, asymmetry index) that require a reference value (Bishop et al., Citation2016). Using a single, 8-s treadmill-based sprint test of uninjured rugby union athletes we previously revealed that SA scores in force generation were three times larger in horizontal in reference to vertical direction (Brown et al., Citation2017).
In a wider context, one of the biggest challenges professional team sports face is to prevent injury, likely increasing during the latter stages of matches (Ekstrand et al., Citation2011). Whereas men are more predisposed to hamstring strains and hip/groin injuries, a higher incidence of quadriceps strains and ankle syndesmosis injuries exists in women football players at the elite European club level (Larruskain et al., Citation2018). To date, most of our knowledge on stride mechanical asymmetries has been derived from trained males tested in ‘fresh’ conditions (e.g., single sprint, without accounting for fatigue perturbation) (Brown et al., Citation2017). Running asymmetry has been investigated from an injury perspective, with asymmetry suggested as a possible contributor to injury predisposition in one side (Zifchock et al., Citation2006). A complete analysis of asymmetry that combines multiple biomechanical variables, known to change in different proportion with sprints repetition (fatigue) (Morin et al., Citation2011), is required. Given the magnitude impact forces experienced during sprint running, and that force production capacity in the lower limbs decreases with sprints repetition (Perrey et al., Citation2010), such analysis could give greater insight into the potential increased predisposition to injury caused by asymmetries (Schache et al., Citation2009).
The aim of this study was to characterise asymmetry of the main running mechanical variables (with special reference to horizontal force production) during repeated treadmill sprints in elite female Rugby Sevens players. We hypothesised that inter-limb differences in sprinting mechanics would differ according to the variable of interest (larger for horizontally vs. vertically derived variables) and increase with fatigue.
Methods
Participants
Eighteen uninjured elite female players belonging to the French national Rugby Sevens team (mean±SD, age: 23.8 ± 3.6 years; height: 172 ± 7 cm; body mass: 68.9 ± 6.9 kg) participated in this study. Ethical approval for the study was provided by the Anti-Doping Laboratory Ethics Committee in Qatar (IRB approval E2017000254) and was undertaken according to the principles outlined in the Declaration of Helsinki.
Experimental approach to the problem
After completing ‘familiarization’ sprints and a standardised warm-up (Girard et al., Citation2017), participants performed 8 × 5-s sprints (25-s rest) on an instrumented motorised treadmill (ADAL3D-WR, Medical Development, France). Briefly, this treadmill allows athletes to complete accelerations from a still position, and it is mounted on a highly rigid metal frame, set at 0 ° grade incline, fixed to the ground through four piezoelectric force transducers (KI 9077b; Kistler, Switzerland). It was installed on a specially engineered concrete slab to ensure maximal rigidity of the supporting ground (Girard et al., Citation2017; Morin et al., Citation2011). Participants were instructed to perform maximally during all sprints and received strong verbal encouragement, while no specific information about performance was provided after each effort.
Running mechanics
Data were continuously sampled at 1,000 Hz, and after appropriate filtering (Butterworth-type 30 Hz low-pass filter), instantaneous data of vertical, net horizontal and resultant ground reaction forces were averaged for each support phase (vertical force >30 N). These data were completed by measurements of the main step kinematic variables: contact time (s), aerial time (s), swing time (s), step frequency (Hz) and step length (m). Foot strike and toe-off instants were determined as the vertical ground reaction forces rose above and fell below 30 N. Step frequency was calculated as the inverse of step duration, which was the time from the foot strike of one leg to the next foot strike of the other leg. Contact time was the duration from the foot strike to the toe off, and flight time was the duration from the toe-off to the foot strike. A linear spring-mass model of running was used to investigate the mechanical integrative variables characterising the lower limbs behaviour during running (Coleman et al., Citation2012). Finally, peak braking and peak propulsive forces (BW), duration of braking and propulsive phases (s) along with absolute (N.s) and relative (m/s) net braking and propulsive impulses were determined. Specifically, braking and propulsive impulses (negative and positive values of the horizontal force signal) were obtained by integrating braking and propulsive ground reaction forces over the corresponding periods, respectively, at each contact phase (Morin et al., Citation2015). Participants completed between 15 and 18 steps during each 5-s sprint (Girard et al., Citation2017). The initial step was systematically omitted since it does not correspond to a complete push-off. For final analysis, values of the 2nd to the 15th step were averaged during the 5-s sprint and then averaged for each leg.
Symmetry angle
For each participant, inter-leg symmetry was measured using the symmetry angle (SA) equation (Zifchock et al., Citation2008):
Symmetry angle (SA) =
but if
then
The SA is an arctan function of the ratio of two bilateral values, where a SA score of 0% indicates perfect symmetry and 100% indicates perfect asymmetry.
Statistical analysis
Descriptive statistics are presented as mean values±SD. Data were tested using a one-factor (time) ANOVA for repeated measures (sprint 1 to 8), while a Bonferroni post hoc multiple comparison was performed if a significant main effect was observed. Effect-sizes were described in terms of partial eta-squared (ηp2, with ηp2 ≥ 0.06 representing a moderate effect and ηp2 ≥ 0.14 a large effect). All statistical calculations were performed using SPSS statistical software V.24.0 (IBM Corp., Armonk, USA). The significance level was set at P < 0.05.
Results
Changes in performance, kinetics and kinematics variables and spring mass characteristics with sprints repetition are displayed in , while horizontal force production data are depicted in .
Table 1. Performance and running mechanics during repeated treadmill sprints.
Table 2. Horizontal force production data during repeated treadmill sprints.
Mean SA scores for all sprints were ~1–2% for contact time (1.6 ± 0.6%), aerial time (2.1 ± 0.8%), step frequency (1.3 ± 0.5%) and step length (1.6 ± 0.6%). Mean vertical and horizontal forces as well as vertical and leg stiffness presented mean SA values of 1.7 ± 1.5%, 2.4 ± 1.2%, 2.6 ± 0.2% and 2.5 ± 0.2%, respectively. Mean SA scores were ~2–8% for duration of braking (6.9 ± 5.0%) and propulsive (6.5 ± 4.4%) phases, peak braking (6.5 ± 2.5%) and propulsive (1.6 ± 0.9%) forces as well as net (5.8 ± 5.6%), braking (7.7 ± 5.3%) and propulsive (2.7 ± 1.6%) impulses. However, there was no influence of sprints repetition on asymmetry scores for the large majority (except for running velocity, contact time and leg compression, P < 0.05) of variables tested (P > 0.05) ().
Discussion and implications
Our main finding is that, with the exception of running velocity and two biomechanical variables (i.e., the fifth sprint had more and less asymmetry for contact time and leg compression, respectively, in reference to the initial bout), SA scores did not change across sprints repetition for 20 gait variables in elite female Rugby Sevens players. As with the present study, we previously observed in physically active males that performing five, 5-s treadmill sprints with 25-s recovery do not amplify kinetics, kinematics and spring-mass-model asymmetries (Girard et al., Citation2017). No direct comparison with female athletes is possible, since the literature is currently lacking investigations of between-leg differences during RSE in high-calibre (i.e., professional/national team, as tested here) female athletes. Interestingly, Brown et al. (Citation2014), who tested 20 active females during over ground running (~12 km/h), concluded that dominant and non-dominant limbs also fatigued at a similar rate after completing a self-paced treadmill run to exhaustion. Collectively, this implies that lower extremities behave similarly during RSE, and that unilateral and bilateral sprint mechanical assessments may offer similar information when it comes to quantify the effects of fatigue, at least in injury-free and inter-limb symmetric athletes. We extend these observations to mechanical variables derived from the horizontal force signal to indicate, for the first time, that RSE was not accompanied by apparent alteration in forward-oriented asymmetrical responses with fatigue.
As with previous studies (Girard et al., Citation2017; Brown et al., Citation2017), averaged SA values were small (<3%) across most kinetics/kinematics and spring mass variables. Additionally, horizontal force measures during both braking and propulsive phases (except for peak propulsive forces) displayed twice larger deviations from symmetry. A qualitative inspection of the average bilateral asymmetries further indicates that scores can range from as low as 0.7% (peak vertical forces) and up to 6.9% (duration of braking phase). In addition to being protocol-, sprint distance- and population-dependent, these data reinforce the notion that asymmetry ultimately depends on the biomechanical variable of interest.
For the first time with RSE, we continuously monitored braking and propulsive peak forces, phase durations and resulting impulses in the horizontal direction (), as previously done for single running sprint (Morin et al., Citation2015). Lengthening of braking versus propulsive phase duration was approximately three times larger (~11% vs. 4%), and thereby was mainly responsible for progressively longer contact times with sprints repetition. While alteration in peak braking and propulsive forces was of similar magnitude (~12–13%), this resulted in progressively lower values for propulsive, but not braking, absolute/relative impulses. This implies that during RSE, athletes primarily slow down, as evidenced by lower net absolute/relative impulses, by pushing less forcefully forward (while also braking less) in the horizontal direction. Consistent with previous RSE studies (male participants) also performed on an instrumented treadmill (Girard et al., Citation2017; Morin et al., Citation2011), our data in elite female athletes showed that changes in kinetics/kinematics and spring-mass characteristics was substantial across repetitions (). Nonetheless, it is difficult to directly compare our results with other experimental conditions since the details of the RSE performed (i.e., number of repetitions, exercise-to-rest ratio), in addition to characteristics of tested participants, differ. Additionally, there is a lack of research validating the force output provided by instrumented treadmills relative to the forces generated whilst sprinting on the competition ground (grass). Although true running velocity was probably slightly underrepresented and subtle biomechanical differences (i.e., external assistance provided by the moving belt) may exist, our motorised instrumented treadmill is a useful device to investigate realistic sprint performance from zero to almost maximal velocity and associated mechanics that were found comparable to field linear sprinting (Morin & Sève, Citation2011).
Our main finding was that lower extremities behave in a similar manner with fatigue, with in general constant SA scores across sprints repetition. Previous sprint running analyses have collected unilateral, biomechanical data due to constraints on positioning of cameras (lateral view; Gittoes & Wilson, Citation2010) or because a single force plate is available (Hunter et al., Citation2004). When a bilateral analysis is not an option, collecting unilateral data may therefore be acceptable to describe the biomechanical manifestation of fatigue during repeated running sprints. However, a step-based approach may provide an incomplete description of sprinting technique as opposed to a stride-based analysis. Hence, SA scores were found to be inconsistent between variables. Variables derived from the horizontal force signal were the most asymmetrical, with almost all SA scores exceeding 5% that represents nearly a doubling compared to remaining measurements. Using a fixed cut-off threshold, for instance, an inter-limb differences of ~15% for injury predisposition (Zifchock et al., Citation2006), is an arbitrary approach since SA scores vary greatly between variables. From a diagnostic perspective, specific inter-limb analyses of kinetics, kinematic or spring-mass characteristics are encouraged to ensure variable-specific bilateral differences are not overlooked. This implies that different biomechanical variables should not be used interchangeably to assess asymmetry. While the present SA scores provide reference data, these values should not be used as cut off points to determine injury risk, as these values ultimately are both population- and task-specific.
Conclusion
In elite female Rugby Sevens players, there was no noticeable difference in asymmetries for the great majority of running kinetics/kinematics (i.e., phases duration, mean/peak forces and impulses in both vertical and horizontal directions) and spring-mass characteristics (i.e., vertical and leg stiffness) during repeated treadmill sprints. Adjustments occurring during the braking and propulsive phases had larger asymmetry scores than other stride mechanical variables.
Disclosure statement
No potential conflict of interest was reported by the author(s).
Additional information
Funding
References
- Bishop, C., Read, P., Chavda, S., & Turner, A. (2016). Asymmetries of the lower limb: The calculation conundrum in strength training and conditioning. Strength and Conditioning Journal, 38, 27–32. doi: 10.1519/SSC.0000000000000264
- Bishop, C., Turner, A., & Read, P. (2018). Effects of inter-limb asymmetries on physical and sports performance: A systematic review. Journal of Sports Sciences, 36, 1135–1144. doi: 10.1080/02640414.2017.1361894
- Brown, A. M., Zifchock, R. A., & Hillstrom, H. J. (2014). The effects of limb dominance and fatigue on running biomechanics. Gait & Posture, 39, 915–919. doi: 10.1016/j.gaitpost.2013.12.007
- Brown, S. R., Cross, M. R., Girard, O., Brocherie, B., Samozino, P., & Morin, J.-B. (2017). Kinetic sprint asymmetries on a non-motorised treadmill in rugby union athletes. International Journal of Sports Medicine, 38, 1017–1022. doi: 10.1055/s-0043-117607
- Coleman, D. R., Cannavan, D., Horne, S., & Blazevich, A. J. (2012). Leg stiffness in human running: Comparison of estimates derived from previously published models to direct kinematic-kinetic measures. Journal of Biomechanics, 45, 1987–1999. doi: 10.1016/j.jbiomech.2012.05.010
- Ekstrand, J., Hagglund, M., & Walden, M. (2011). Epidemiology of muscle injuries in professional football (soccer). American Journal of Sports Medicine, 39, 1226–1232. doi: 10.1177/0363546510395879
- Girard, O., Brocherie, F., Morin, J.-B., & Millet, G. P. (2017). Lower limb mechanical asymmetry during repeated treadmill sprints. Human Movement Science, 52, 203–214. doi: 10.1016/j.humov.2017.02.008
- Gittoes, M. J. R., & Wilson, C. (2010). Intralimb joint coordination patterns of the lower extremity in maximal velocity phase sprint running. Journal of Applied Biomechanics, 26, 188–195. doi: 10.1123/jab.26.2.188
- Hunter, J. P., Marshall, R. N., & McNair, P. J. (2004). Interaction of step length and step rate during sprint running. Medicine and Science in Sports & Exercise, 36, 261–271. doi: 10.1249/01.MSS.0000113664.15777.53
- Jacobs, C., Uhl, T. L., Seeley, M., Sterling, W., & Goodrich, L. (2005). Strength and fatigability of the dominant and nondominant hip abductors. Journal of Athletic Training, 40, 203–206.
- Larruskain, J., Lekue, J. A., Diaz, N., Odriozola, A., & Gil, S. M. (2018). A comparison of injuries in elite male and female football players: A five-season prospective study. Scandinavian Journal of Medicine and Science in Sports, 28, 237–245. doi: 10.1111/sms.12860
- Morin, J.-B., Samozino, P., Edouard, P., & Tomazin, K. (2011). Effect of fatigue on force production and force application technique during repeated sprints. Journal of Biomechanics, 44, 2719–2723. doi: 10.1016/j.jbiomech.2011.07.020
- Morin, J.-B., & Sève, P. (2011). Sprint running performance: Comparison between treadmill and field conditions. European Journal of Applied Physiology, 111, 1695–1703. doi: 10.1007/s00421-010-1804-0
- Morin, J. B., Slawinski, J., Dorel, S., de Villareal, E. S., Couturier, A., Samozino, P., Brughelli, M., & Rabita, G. (2015). Acceleration capability in elite sprinters and ground impulse: Push more, brake less? Journal of Biomechanics, 48, 3149–3154. doi: 10.1016/j.jbiomech.2015.07.009
- Perrey, S., Racinais, S., Saimouaa, K., & Girard, O. (2010). Neural and muscular adjustments following repeated running sprints. European Journal of Applied Physiology, 109, 1027–1036. doi: 10.1007/s00421-010-1445-3
- Radzak, K. N., Putnam, A. M., Tamura, K., Hetzler, R. K., & Stickley, C. D. (2017). Asymmetry between lower limbs during rested and fatigued state running gait in healthy individuals. Gait & Posture, 51, 268–274. doi: 10.1016/j.gaitpost.2016.11.005
- Schache, A. G., Wrigley, T. V., Baker, R., & Pandy, M. G. (2009). Biomechanical response to hamstring muscle strain injury. Gait and Posture, 29, 332–338. doi: 10.1016/j.gaitpost.2008.10.054
- Zifchock, R. A., Davis, I., & Hamill, J. (2006). Kinetic asymmetry in female runners with and without retrospective tibial stress fractures. Journal of Biomechanics, 39, 2792–2797. doi: 10.1016/j.jbiomech.2005.10.003
- Zifchock, R. A., Davis, I., Higginson, J., & Royer, T. (2008). The symmetry angle: A novel, robust method of quantifying asymmetry. Gait & Posture, 27, 622–627. doi: 10.1016/j.gaitpost.2007.08.006