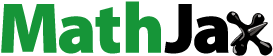
ABSTRACT
The study objective is to test general- and sport-specific adaption during a single training on the Flexibility Trainer. The device is designed to trigger residual muscle tone decreases of the hip-joint muscles by providing (nearly) isokinetic resistance during a full range of motion strength training in adduction/abduction and flexion/extension direction. Static hip flexion and abduction as well as kinematics of double side kicks were analysed on 15 participants before and after training (or rest for controls) to assess general flexibility and sport-specific movement range. Tests were recorded by a Vicon® motion capturing system. Static hip flexion and abduction as well as leg vector spreading angles (VSA) at different nodes of the kick were selected to determine adaptions of active and passive flexibility. Normalised hip joint moments, movement velocities and VSA were calculated to evaluate the training with the device. ANOVAs with 4-repeated measures and Friedman tests were performed to identify time differences and Bonferroni post-hoc test to identify between-subject effects. Significant differences were found for both static flexibility tests (Flexion = 13.65%; Abduction = 9.94%) and the VSA at specific action phases (≤15.15%). Results indicate that short-term adaptions when training with the Flexibility Trainer are exceeding comparable literature showing improved flexibility and sport-specific performance.
Introduction
Flexibility, as ‘the ability to move a joint smoothly through its complete range of motion … is determined by the nature of the joint structure, the condition of the ligaments and fascia that surround the joint, and muscle extensibility’ (Kent, Citation2006, p. 214), whereas active flexibility additionally depends on antagonistic muscle strength. However, joint stability decreases with the reduction of residual muscle tone, which increases injury risk. Therefore, strength and flexibility in combination with sufficient joint stability constitute essential synergising abilities for certain sport techniques. Particularly hip joints determine movement stability in various sports (Rahman & Islam, Citation2020) as their active range of motion (ROM) is a particularly important performance parameter. For example, martial artists need superior hip joint flexibility and strength, while performing kicking techniques at chest/head level of their opponents (Hoelbling et al., Citation2017). Furthermore, flexibility significantly decreases with age and periods of immobility (Roaas & Andersson, Citation1982), which renders active hip joint ROM training challenging, particularly for older athletes. Recent research established four methodical approaches (2 reflexes, namely autogenic and reciprocal inhibition; serial/longitudinal hypertrophy as physiological adaption, describing increases in the number of sarcomeres in series; isokinetic training mode, describing a force-independent training with constant velocity) that may support the effectiveness of this type of training (Brown, Citation2000; Csapo et al., Citation2011; Kent, Citation2006, p. 62, 458; Sharman et al., Citation2006).
Autogenic inhibition (or inverse myotatic reflex) describes that after a maximum contraction, a muscle is forced to lower the residual tension. Therefore, the reflex is used to force significant short-term adaptions in ROM. Reciprocal inhibition is “a process that inhibits the stretch reflex in antagonistic pairs of muscles (Kent, Citation2006, p. 458). Both reflexes combined are used in a special form of proprioceptive neuromuscular facilitation (PNF), called contract-relax-agonist-contract (CRAC) training, which appears extraordinarily effective for short-term training adaptions (Sharman et al., Citation2006). Furthermore, recent studies show that full ROM strength training results in physiological adaptions of muscles and fascias related to flexibility improvement (Baker et al., Citation2006; Csapo et al., Citation2011; Franchi et al., Citation2014; Reid & McNair, Citation2004). This physiological adaption is called serial (or longitudinal) hypertrophy. It counts as a long-term flexibility enhancement method. The training device presented in this work is designed to trigger all effects, described above. The isokinetic training mode was chosen to increase training effectiveness (Brown, Citation2000) and reduce the risk of injury as execution forces are solely determined by voluntary contraction, instead of specific resistance, which consequently decreases dangerous peak forces in certain unsupported joint angles. As stated above, a combination of all 4 methodical approaches is believed to result in superior adaptions of flexibility and strength, particularly if combined in one sports device for targeted semi-isolated training. Therefore, a sports device prototype, called Flexibility Trainer (FT) based on the concepts by Hoelbling (Citation2016) was manufactured for testing.
The device is designed to enhance both general and sport-specific ROM, by increasing active and passive flexibility as well as strength. Its effectiveness needs to be tested using common sport motoric assesments as well as a sports-specific technique analysis, which highly depends on hip joint flexibility to evaluate the effectiveness of the device. Both are based on the paper by Hoelbling et al. (Citation2020a), which analyses correlations between 2 static flexibility tests (standing toe touch and sitting side split) with leg vector spreading angles (VSA) during the double side kick (DSK) kicking combination. Results indicated that the movement range during the DSK execution is highly related to the static hip abduction flexibility, as well as the overall performance of the kick and tournament success of the fighter. Therefore, increased flexibility does not simply help to reach the target but also to achieve superior technique execution by enabling the athlete to increase the horizontal distance between the support foot and COM, which consequently leads to a higher impact potential and other tactical advantages (Hoelbling et al., Citation2020a). For sport-specific testings, the double side kick proved to constitute a frequently used and sufficiently researched martial arts competition technique combination (Ouergui et al., Citation2013; Siska & Brodani, Citation2017). The fact that it is a single-leg double kick but still a very common competition technique ranks it as one of the most difficult ‘standard’ techniques, which renders it ideal for distinction between skill levels.
The aim of the study is to analyse the general and sport-specific short-term responses to a training with The Flexibility Trainer, by applying selected tests and models related to Martial Arts performance (Hoelbling et al., Citation2020a). This objective leads to following hypotheses:
The training with The Flexibility Trainer results in a significant improvement of hip flexion during the standing toe-touch test.
The training with The Flexibility Trainer results in a significant improvement of hip abduction during the sitting side split test.
The training with The Flexibility Trainer results in a significantly higher leg spreading angle during specific nodes (INI; FLX1; MP1; KE1; FLX2; MP2; KE2; FC; as defined in Methods section) of the DSK technique execution.
The training with The Flexibility Trainer is performed as an active movement, even when the gravity component acts in motion direction (spreading).
Materials and methods
Experimental approach
The laboratory was equipped with a 3x1m2 tatamis (martial arts mats) with a punching bag at the end of the technique execution area and a 3x2m2 tatami with wooden panels on it as the foundation for The Flexibility Trainer as suggested by Hoelbling et al. (Citation2020). The entire motion analysis volume was surrounded by 12 infrared Vicon® 3D motion capturing cameras. During the tests and device training, the participants were equipped with 39 reflective markers, which were placed on specific landmarks on the body according to the plug-in-gait marker setup (Vicon®, Citation2016). The marker positions within the observation volume were digitalised and labelled, using Vicon® Nexus. After recording, data was prepared (filling gaps) and smoothed, using Woltring filter routine (Woltring, Citation1986) for marker trajectories. Force sensor data was filtered with a Butterworth filter (Winter, Citation2009), using a 1 Hz cut-off frequency (due to the long and slow motions), for performance analysis during device training. Then, plug-in-gait static and dynamic gait models were applied, to establish joint angles and joint centre positions. Data was extracted, using specially developed Python® routines for unification of the data extraction process, and exported into Microsoft Excel.
Subjects
The sample group consisted of 15 low to high achieving male martial artists (kickboxing, sport karate and taekwondo), including 2 world championship finalists and (additionally) 6 national champions. Each fighter participated in both the control (first test day) and the training group (second test day) at 2 different days within 1 week at a similar daytime. Participants (Age: 30.13 years ± 6.97; Mass: 84.93 kg ± 10.55; Height 182.27 cm ± 4.58) were healthy, without current or chronic hip or knee joint pain, and each of them had at least 1 year of experience in their sport.
Device
Based on the preliminary design published by Hoelbling et al. (Citation2020), a working prototype was produced for this study. The device, shown and described in was designed to enable (near-) isokinetic training in abduction/adduction and flexion/extension (foot mounts are rotated 90° and each leg can be mounted in front or back). The deceleration mechanism works for leg spreading and closing motion, so the athlete can actively work with maximum strength in both directions.
Figure 1. Design illustration of Flexibility Trainer, with the braking system (isokinetic hydraulic dampers) and the force sensor (connection between dampers and left slide). Rotational damper (front of foot mount), steel cable with guide rollers (from left to right) and its opposing sided mounts on the sliders (Hoelbling et al., Citation2020)
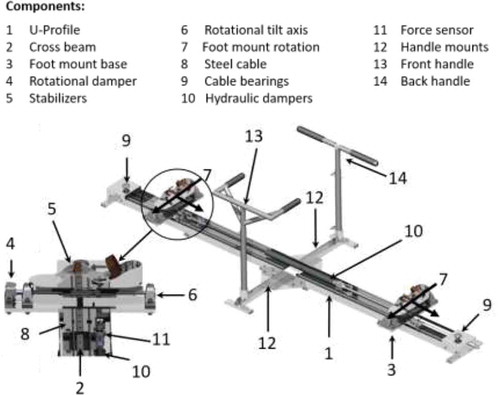
Procedures
After their declaration of informed consent (approved by the ethics committee), the athletes were instructed to do a standardized warm-up, consisting of 5 minutes of cycling at 60–80 watts on an exercise bike and two different types of hip-joint swing gymnastics (20 repetitions of lateral and front swings with straight and bended knee), followed by the marker placement for the Vicon® motion capturing analysis. The testing procedures started with the standing toe-touch test for the assessment of hip flexion (Kippers & Parker, Citation1987) and the sitting side-split (Amigó et al., Citation2010) test for hip abduction, see .
Figure 2. Visualisation of flexibility tests recorded with a 3D Vicon® motion capturing system, based on (Hoelbling et al., Citation2020a)
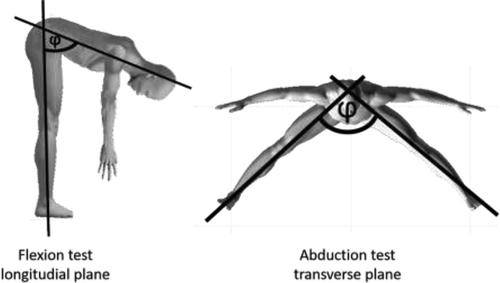
After about 1 minute, 3 trials of 3 repetitions of the double side kick (as described in Double side kick technique execution) were performed. Participants were instructed to reach the target at chin height at the second kick; however, lower kick heights were also accepted for analysis. If markers detached during the trial, the participant was instructed to stop and perform another full trial after replacement. Incomplete or insufficient trials (e.g., if the first kick was not fully performed) were excluded from the analysis.
For the device training, athletes were instructed to wear shoes, and foot markers were placed accordingly, so the participants’ feet could be fixed to the foot mounts of the sliders. The training started with the abduction position shown in . The athlete performed the exercise, as described in . After another short rest of about 2 minutes the participants executed the training in both front split directions () in the same sequence, with another 2 minutes of rest in between.
Table 1. Flexibility trainer exercise description for total ~60s
Figure 3. Phases and nodes illustration of the double side kick, with specifications of kicking movement 1 and 2 (KIM1; KIM2), which together forms the total kicking movement (TKIM)
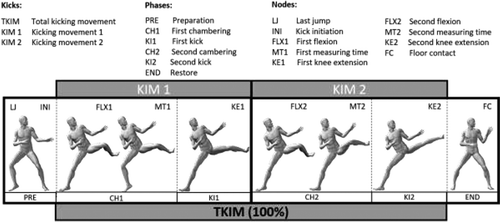
The exercise sequences were followed by 15 minutes of rest for the control group testing day, in order to exclude a possible test-habituation effect or any effects from the kick exercise on the flexibility tests and vice versa. After the training, athletes were instructed to take off their shoes, and markers were placed accordingly. The post-exercise (or rest) flexibility and (3 trials of 3) double side kick tests were repeated in the same sequence as in the pre-exercise (or rest) tests on both testing days (control and training).
Double side kick technique execution
The technique (see, ) is executed from a double foot jumping movement (BNC) called ‘bouncing’ (Young-Kwan & Yoon Hyuk, Citation2011) or ‘hopping’ (Yuncza, Citation2011). After a short initiation action (PRE, between nodes LJ and INI (Hoelbling et al., Citation2021)), the total kicking movement (TKIM) starts with a lateral pendulum-like front leg elevation (CH1, between nodes INI and MT1). During this movement, a knee flexion (FLX1) is performed to decrease the lever arm of the kicking leg. Afterwards, the leg is extended again, which leads to the first kicking action (KI1, between nodes MT1 and KE1) without target contact. After maximum knee extension, a second leg elevation (CH2, between nodes KE1 and MT2), with another knee flexion (FLX2) and second kick (KI2, between nodes MT2 and KE2) with target contact are executed, which constitutes the end of KIM2 and consequently TKIM. Finally, the kicking leg is restored on the floor (END, between nodes KE2 and FC). Detailed phase and note definition was used from Hoelbling et al. (Citation2017) and Hoelbling et al. (Citation2020b).
Variable definition
To analyse both general and specific flexibility before and after the intervention (or rest) as well as to describe the device training modalities, a specific set of variables was established for each test.
Static flexibility test data
To identify changes in static hip flexibility, the combined abduction angles of both hip joints and the maximum average of right and left hip flexion angles, as given by Vicon®, were chosen for analysis.
Double side kick technique execution
To analyse sport-specific changes in dynamic flexibility through device training, the leg vector spreading angle (VSA; angle between vectors from hip to ankle joint centres of both legs) was calculated, using Equation 1, according to Hoelbling et al. (Citation2020a):
Equation 1: Maximum leg vector spreading angle (VSA) calculation for each spreading repetition at device training. φ = Leg vector spreading angle; LL = Left leg vector angle; RL = Right leg vector angle ().
Figure 4. Illustration of forces and moments acting during device training, adapted from. Hoelbling et al. (Citation2020)
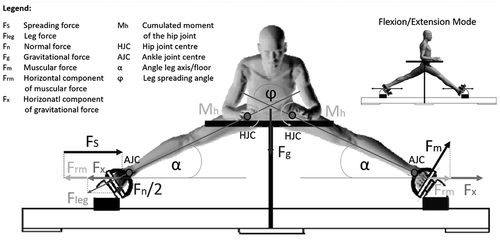
Kinetic analysis of device training
To determine the physical performance during the device training, the average relative hip joint moment during the spreading motion (for more detailed description, see Appendix A), on the basis of force sensor data and Vicon® plug-in-gait model outputs (Vicon®, Citation2016), we used the equations outlined below (all scalars):
Cumulated hip spreading moment (Mh), based on , was calculated by subtracting the gravity component from the sensor force and translating it to the hip, using Equation 2, as described in Hoelbling et al. (Citation2020):
Equation 2: Calculation of leg spreading hip moment during device training. Legend: Mh = Hip joint spreading moment; FS = Sensor Force (for 1 leg); Fg = Gravitation force (m*g); α = Leg/floor angle; lleg = Leg length.
It is noted that this equation does not apply to the leg closing motion, as the body weight at this exercise was significantly supported on the handle mounts (in contrast to the spreading motion, where the handles are mainly used to ensure balance), so the authors were not able to calculate the gravity component.
To calculate each component (flexion/extension, abduction/adduction, ext./int. rotation), the cumulated hip joint moment Mh was divided in the same ratio as the Vicon® model outputs, which are based on the segmental movement direction but do not include the resistance of the Flexibility Trainer. Equation 3 (Hoelbling et al., Citation2020) was used for this calculation:
Equation 3: Calculation of hip flexion/extension moment. Legend: Mi = Hip joint moment in preferred movement direction (Mx = Flexion/Extension moment; My = Abduction/adduction moment; Mz = Int./ext. rotation moment); MVi = Vicon calculated moment in preferred movement direction; Mh = Cumulated hip moment; MVx = Vicon hip flexion/extension moment; MVy = Vicon hip adduction/abduction moment; MVz = Vicon hip int./ext. rotation moment.
Finally, the average, relative abduction or flexion/extension moment (for device adduction/abduction and both flexion/extension trials) was calculated separately for leg spreading motion, using Equation 4:
Equation 4: Calculation of average normalised hip moment. Legend: ØMi = Average normalised moment in preferred movement direction (Mx = flexion moment; My = abduction moment); Mir = Hip moments right leg (Mxr = flexion/extension moment right leg; Myr = Abduction/adduction moment right leg); Mil = Hip moments left leg (Mxl = flexion/extension moment left leg; Myl = Abduction/adduction moment left leg); nMi = Number of frames used for calculation; m = body mass.
Furthermore, average horizontal execution velocity (per leg) for both spreading and closing, as well as maximum VSA of all 3 separate executions (including rest) were determined. VSA were calculated as in Equation 1.
Statistical analysis
Statistical analysis was performed using SPSS ver. 22 (IBM Corp., Chicago, Illinois). Normal distribution for all variables was checked, using the Shapiro–Wilk test for sample size < 50 participants. Descriptive statistics of all pre- and post-tests for both groups were performed.
To identify significant differences in changes between both, training, and control as well as pre and post-tests, analysis of variance (ANOVA; for normally distributed variables) for 4 repeated measures (control group pre; control group post; training group pre; training group post), or the Friedman test (for variables significantly differing from normal distribution) were used. Bonferroni post hoc test was used if significant differences were detected.
For all tests, a significance level of p = 0.05 was used.
Results
All 15 participants performed two flexibility tests each and a total of 417 kicks for all participants. ANOVA tests show significant differences in hip flexion (hypothesis 1) and abduction (hypothesis 2) between the test times ().
Table 2. Results of main (within-subject) effects and group differences of ANOVA (or Friedman test for variables, which significantly differ from normal distribution). Variables: φ = leg spreading angle; INI = Kick initiation; FLX1 = First flexion; MT1 = First measuring time; KE1 = First knee extension; FLX2 = Second flexion; MT2 = Second measuring time; KE2 = Second knee extension; FC = Floor contact; KIM = Kicking motion (Hoelbling et al., Citation2017)
Furthermore, the post-test for flexion and abduction showed significant differences to all other related previous test times ().
Figure 5. Static flexibility test results training (TR) and control (CO) group before (Pre) and after (Post) intervention or rest. * above bars marks significant differences of Bonferroni post-hoc test between test times, whereas * between the bars on the bottom means significant difference of the ANOVA test between test times (further details in )
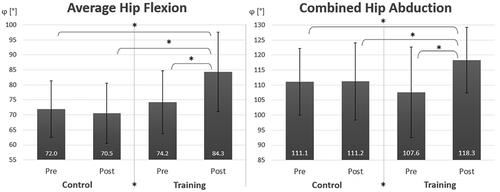
Averaged vector spreading angles (hypothesis 3) showed significant differences at ANOVA tests between sessions for MT1, KE1, FLX2, and KE2 as well as for the maxima at KIM1 and KIM2, but not for INI, FLX1, MT2 and FC, as shown in and .
Figure 6. Results for vector spreading angles (VSA) during the kicking movements (KIM) of training (TR) and control (CO) group before (Pre) and after (Post) intervention or rest. * marks significant differences between variables and groups (further details to group differences for VSA at the nodes are shown in )
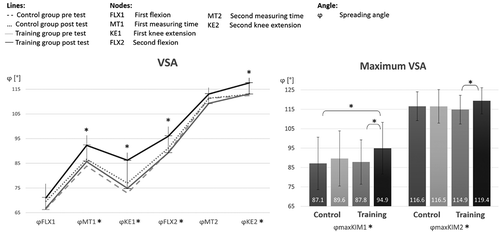
Table 3. Bonferroni post-hoc test for ANOVA for comparison of group differences of the nodes with significant ANOVA outcomes during the kick execution. Mean and standard deviation of VSAs are given for each group and brackets below mark significant differences of the Bonferroni test and the respective test times. Nodes: MT1 = first measuring time 1; KE1 = first knee extension; FLX2 = second knee flexion; KE2 = Second knee extension
Results of Bonferroni post-hoc tests for analysis of between-subject differences of MT1, KE1, FLX2, KE2, KIM1 and KIM2 are shown in .
At the device training, all participants performed three spreading and three closing movement and one CRAC contraction set (during the last repetition) in total, for all 3 exercises. Descriptive statistics are given in Mean ± SD or Median ± IR (interquartile range) if the variable (marked with ⁺) differs significantly from normal distribution. To describe the performance during device training (hypothesis 4), average values for spreading velocity (vSpAbd = 111.23 mm/s ± 23.39; vSpFL = 120.64 mm/s ± 29.52; vSpFR = 114.25 mm/s ± 23.75), closing velocity (vClAbd = 80.04 mm/s ± 11.45; vClFL = 93.71 mm/s ± 20.14; vClFR = 91.97 mm/s ± 16.18) and relative spreading (ϕMAbd = 1.54 Nm/kg ± 0.60; ϕMFL = 1.43 Nm/kg ± 0.76; ϕMFR = 2.15 Nm/kg ± 0.68) moment, as well as the maximum VSA (φmaxAbd1 = 125.12°± 15.71; φmaxAbd2 = 126.59°± 14.97; φmaxAbd3 = 125.18°± 19.35; φmaxFL1 = 130.19°± 12.51; φmaxFL2⁺ = 132.61°± 11.39; φmaxFL3 = 133.68°± 8.31; φmaxFR1 = 128.25°± 11.95; φmaxFR2 = 131.58°± 9.01; φmaxFR3 = 133.74°± 8.60) for all 3 repetitions and exercises (Abduction = Abd; left front split = FL; right front split = FR), were calculated.
Discussion and implications
Firstly, it is noted that no injuries or health impairments were reported during or within 1 week after the sessions, despite high physical strain during the device training, determined by hip joint moments of up to 2.15 Nm/kg in average. Furthermore, results clearly show that training with the Flexibility Trainer (FT) significantly enhances both general and sport-specific flexibility/ROM within a single training session. The general flexibility tests showed average improvements of 10.1° (13.65%) for static hip flexion and 10.7° (9.94%) for combined abduction (). In comparison, Feland et al. (Citation2001) reported median improvements on hamstring flexibility of 4° from static and 6° from PNF training, while Fjerstad et al. (Citation2018) found 1° for passive and 1.2° for active static stretching and the athletes of Rubini et al. (Citation2011) achieved 2.7° after static and 4.4° PNF training of the hip adductors. Sharman et al. (Citation2006) on the other hand describe an expected range of 3°-9° for one PNF treatment based on their systematic review. A comparison of absolute values of the training groups’ pre- and post-tests to Hoelbling et al. (Citation2020a) also provides interesting insights. This study compared static flexibility of 3 groups, which could be described as absolute world elite (1), elite (2) and advanced (3) kickboxers and karateka. The current pre-test of the FT training group showed slightly lower mean values (Flx: 74.2°; Abd: 84.3°) than their advanced group (Flx: 78.53°; Abd: 110.24°), while the post-test values were higher (Flx: 84.3°; Abd: 118.3°), partially even close to the mean values of their elite group (Flx: 86.6°; Abd: 132.59°). Furthermore, significant differences were found between the post tests of the training group to all other test times, while no differences were found between the other test times. In fact, all participants improved both static hip joint flexibility components after the training with the Flexibility Trainer, which leads to the acceptance of hypotheses 1 and 2.
Sport-specific movement range measured with vector spreading angles (VSA) also improved at most nodes during the double side kick execution. Mean VSA of MT1 (+7.67%), KE1 (+15.15%), FLX2 (+7.55%), and KE2 (+3.94%), as well as the maxima of KIM1 (+8.08%) and KIM2 (+3.94%), showed significant improvements, particularly within the training group, while the control group test times and the pre-test of the training group, showed no significant differences at all (). Furthermore, all these values showed significant differences between-subject effects (), which leads to the acceptance of hypothesis 3. Comparisons of these results with those from the 3 groups of Hoelbling et al. (Citation2020a) showed that the FT training caused slightly lower maximum angles before (114.9°) and slightly higher maximum angles (119.4°) after the intervention than their advanced group (116.45°). These values are again just slightly below their elite group (122.35°). A different situation has been found during the kick executions, where both the pre- and post-test values are between their advanced and elite group at MT1 and KE1. Post-test values of the FT training group at MT2 are very close to those of their elite group and at KE2, the values are even above the VSA of their elite group. This may indicate that the sport-specific performance of the current study is above their advanced group and the short-term effect of the device training could improve the kicking technique even to elite level. However, to support this statement, many other factors must be considered.
While the closing movement must be an active movement (due to the usage of the hydraulic dampers), the hip moment calculation for the spreading motion clearly showed that the athletes contracted their respective muscles (with up to 2.15 Nm/kg in average) during the exercise, which leads to the acceptance of hypothesis 4. It is further reported that traditional stretching methods often have no or even negative effects on performance of ballistic movements (Curry et al., Citation2009; Perrier et al., Citation2011; Young & Elliott, Citation2001), which can be attributed to a decrease in musculotendinous unit stiffness leading to a lower rate of force production and a delay in muscle activation (Paradisis et al., Citation2014). However, it is noted that activating follow-up exercise can substantially mitigate this effect (Behm & Chaouachi, Citation2011). The current method, on the other hand, showed highly significant improvements after device training sessions. This effect could either be attributed to the mitigating effect (as described above) or even to a short-term improvement in muscle strength, due to maximum voluntary activation or solely to the decrease of antagonistic inhibition. However, these potential hypotheses are beyond the scope of the current study and will be investigated in future studies.
To summarise, both general and sport-specific flexibility has improved more than expected after the training with the FT, by up to 15.15% on average. These effects might be based not solely on an increased reciprocal and autogenic inhibition effect, due to full ROM resistance (hip moments up to 2.15 Nm/kg on average), but also on the release of fascia bondings due to strong contractions in maximum positions and increased stress tolerance. Given the fact that improvements were found across the entire participant population, from highly flexible and highly successful martial artists to fighters with lower performance levels.
Based on the rapid ROM increase during the device training, with the first execution (with 125.12° on average) already above the maximum static flexibility result (107.55° on average before the intervention) and the strong contractions throughout the exercise, we want to introduce the descriptive methodical term of Supra-ROM strength training (SROM) for this exercise type. It describes a strength exercise, performed in a ROM higher than the previous static maximum.
Therefore, we conclude that the Flexibility Trainer is potentially an effective device to improve the hip-joint flexibility. It enables the athlete to effectively perform SROM strength training by using respective reflexes within the training session. This is expected to lead to novel training effects by forcing the targeted muscles to a serial hypertrophy and increased strength in the opposing muscles, which consequently may help to overcome a performance plateau, even for high-performing athletes. However, the device design is work in progress and future studies need to assess long-term adaptions and optimise the training sequence. To provide an outlook on planned future research, we would expect that long-term adaptions due to a combined effect of CRAC PNF and SROM to be significantly higher than in previously reported. For example, Sharman et al. (Citation2006) report improvements of up to 21° in 12 weeks, Rowlands et al. (Citation2003) found 33.6° in 6 weeks of CRAC training, whereas in Valamatos et al. (Citation2018), fascia length increased by 4.9% in a 15 week intervention, and in Reid and McNair (Citation2004), the joint angle improved by 10.1° after 6 weeks of full range of motion training.
Limitations
The device prototype requires improvement in stability, particularly at the handle constructions (side movements) and foot mounts, mainly due to tolerances of the used tools and standard parts. Furthermore, the foot mounts are not optimised for a self-training configuration.
Different training sequences with the device should be tested for determining an optimum. The results only show short-term adaptions by the device, compared to a non-training setting. A training study should be performed to analyse short- and long-term adaptions compared to other flexibility trainings with similar load durations, in order to determine cumulated benefits and advantages of a training with the device. Furthermore, some sport specific variables showed significant difference between pre- and post-test in the training group but not between control tests and post-test of the training groups, which may be due to a limited learning or adaption effect. This effect, however, did not hold on until the next test day, as some variables decreased again until the pre-test of the training group.
Acknowledgments
The authors express their gratitude to all athletes who participated in the study and further to the students who constructed the first two versions of the Flexibility Trainer as well as the engineers who finally manufactured the prototype.
Disclosure statement
No potential conflict of interest was reported by the author(s).
References
- Amigó, A. I., Faciabén, A. B., Marginet, M. C., Ferrer, B., & Evrard, M. M. (2010). Flexibility testing in young competing gymnasts using a trigonometric method: One-year follow-up. Apunts: Medicina de l’esport, 45(168), 235–242. https://www.raco.cat/index.php/Apunts/article/download/217291/298466
- Baker, M. J., Wu, Y. Z., Green, S., & Caiozzo, V. J. (2006). Is longitudinal growth of skeletal muscle dependent upon satellite cell activation [Paper presented]. The 52nd annual meeting of the orthopaedic research society, Chicago, USA.
- Behm, D. G., & Chaouachi, A. (2011). A review of the acute effects of static and dynamic stretching on performance. European Journal of Applied Physiology, 111(11), 2633–2651. https://doi.org/10.1007/s00421-011-1879-2
- Brown, L. E. (2000). Isokinetics in human performance. Human Kinetics.
- Csapo, R., Alegre, L. M., & Baron, R. (2011). Time kinetics of acute changes in muscle architecture in response to resistance exercise. Journal of Science and Medicine in Sport, 14(3), 270–274. https://doi.org/10.1016/j.jsams.2011.02.003
- Curry, B. S., Chengkalath, D., Crouch, G. J., Romance, M., & Manns, P. J. (2009). Acute effects of dynamic stretching, static stretching, and light aerobic activity on muscular performance in women. The Journal of Strength & Conditioning Research, 23(6), 1811–1819. https://doi.org/10.1519/JSC.0b013e3181b73c2b
- Feland, J. B., Myrer, J., & Merrill, R. (2001). Acute changes in hamstring flexibility: PNF versus static stretch in senior athletes. Physical Therapy in Sport, 2(4), 186–193. https://doi.org/10.1054/ptsp.2001.0076
- Fjerstad, B. M., Hammer, R. L., Hammer, A. M., Connolly, G., Lomond, K. V., & O’Connor, P. (2018). Comparison of two static stretching procedures on hip adductor flexibility and strength. International Journal of Exercise Science, 11(6), 1074. https://www.ncbi.nlm.nih.gov/pmc/articles/PMC6179425/
- Franchi, M. V., Atherton, P. J., Reeves, N. D., Fluck, M., Williams, J., Mitchell, W. K., Selby, A., Beltran Valls, R. M., & Narici, M. V. (2014). Architectural, functional and molecular responses to concentric and eccentric loading in human skeletal muscle. Acta Physiologica, 210(3), 642–654. https://doi.org/10.1111/apha.12225\r10.1111/apha.12225
- Hoelbling, D. (2016). EP000003269429A1. U. WIEN.
- Hoelbling, D., Baca, A., & Dabnichki, P. (2020a). A kinematic model for assessment of hip joint range-of-motion in fast sport movements using spreading angles. Sports Biomechanics, 1–13. https://doi.org/10.1080/14763141.2020.1795237
- Hoelbling, D., Baca, A., & Dabnichki, P. (2020b). Sequential action, power generation and balance characteristics of a martial arts kick combination. International Journal of Performance Analysis in Sport, 20(5), 766–781. https://doi.org/10.1080/24748668.2020.1774730
- Hoelbling, D., Grafinger, M., Baca, A., & Dabnichki, P. (2020). The flexibility trainer: Feasibility analysis, prototype- and test station development for a sports device for hip-joint flexibility and strength enhancement [Paper presented]. The proceedings of the 8th international conference on sport sciences research and technology support (icSPORTS 2020), Budapest, Hungary.
- Hoelbling, D., Smiech, M., Mattaeus, Cizmic, D., Baca, A., & Dabnichki, P. (2021). Exploration of Martial Arts Kick Initiation Actions and Telegraphs International Journal of Performance Analysis in Sport [Paper presented]. https://doi.org/10.1080/24748668.2021.1920314.
- Hoelbling, D., Preuschl, E., Hassmann, M., & Baca, A. (2017). Kinematic analysis of the double side kick in pointfighting, kickboxing. Journal of Sports Sciences, 35(4), 317–324. https://doi.org/10.1080/02640414.2016.1164333
- Kent, M. (2006). The Oxford dictionary of sports science and medicine. Oxford University Press.
- Kippers, V., & Parker, A. W. (1987). Toe-touch test: A measure of its validity. Physical Therapy, 67(11), 1680–1684. https://doi.org/10.1093/ptj/67.11.1680
- Ouergui, I., Hssin, N., Franchini, E., Gmada, N., & Bouhlel, E. (2013). Technical and tactical analysis of high level kickboxing matches. International Journal of Performance Analysis in Sport, 13(2), 294–309. https://doi.org/10.1080/24748668.2013.11868649
- Paradisis, G. P., Pappas, P. T., Theodorou, A. S., Zacharogiannis, E. G., Skordilis, E. K., & Smirniotou, A. S. (2014). Effects of static and dynamic stretching on sprint and jump performance in boys and girls. The Journal of Strength & Conditioning Research, 28(1), 154–160. https://doi.org/10.1519/JSC.0b013e318295d2fb
- Perrier, E. T., Pavol, M. J., & Hoffman, M. A. (2011). The acute effects of a warm-up including static or dynamic stretching on countermovement jump height, reaction time, and flexibility. The Journal of Strength & Conditioning Research, 25(7), 1925–1931. https://doi.org/10.1519/JSC.0b013e3181e73959
- Rahman, M. H., & Islam, M. S. (2020). Stretching and flexibility: A range of motion for games and sports. European Journal of Physical Education and Sport Science, 6(8). https://oapub.org/edu/index.php/ejep/article/download/3380/6016
- Reid, D. A., & McNair, P. J. (2004). Passive force, angle, and stiffness changes after stretching of hamstring muscles. Medicine and Science in Sports and Exercise, 36(11), 1944–1948. https://doi.org/10.1249/01.MSS.0000145462.36207.20
- Roaas, A., & Andersson, G. B. (1982). Normal range of motion of the hip, knee and ankle joints in male subjects, 30–40 years of age. Acta Orthopaedica Scandinavica, 53(2), 205–208. https://doi.org/10.3109/17453678208992202
- Rowlands, A. V., Marginson, V. F., & Lee, J. (2003). Chronic flexibility gains: Effect of isometric contraction duration during proprioceptive neuromuscular facilitation stretching techniques. Research Quarterly for Exercise and Sport, 74(1), 47–51. https://doi.org/10.1080/02701367.2003.10609063
- Rubini, E. C., Souza, A. C., Mello, M. L., Bacurau, R. F. P., Cabral, L. F., & Farinatti, P. T. V. (2011). Immediate effect of static and proprioceptive neuromuscular facilitation stretching on hip adductor flexibility in female ballet dancers. Journal of Dance Medicine & Science, 15(4), 177–181. https://www.ingentaconnect.com/content/jmrp/jdms/2011/00000015/00000004/art00006
- Sharman, M. J., Cresswell, A. G., & Riek, S. (2006). Proprioceptive neuromuscular facilitation stretching: Mechanisms and clinical implications. Sports Medicine, 36(11), 929–939. https://doi.org/10.2165/00007256-200636110-00002
- Siska, L., & Brodani, J. (2017). Point-fight kickboxing match analysis. International Journal of Physical Education, Sports and Health, 4(4), 16–19. https://www.researchgate.net/profile/Jaroslav-Brodani/publication/318461923_Point-fight_kickboxing_match_analysis/links/596c702fa6fdcc18ea793b7a/Point-fight-kickboxing-match-analysis.pdf
- Valamatos, M. J., Tavares, F., Santos, R. M., Veloso, A. P., & Mil-Homens, P. (2018). Influence of full range of motion vs. equalized partial range of motion training on muscle architecture and mechanical properties. European Journal of Applied Physiology, 118(9), 1969–1983. https://doi.org/10.1007/s00421-018-3932-x
- Vicon®. (2016). Plug-in gait reference guide (pp. 95). Vicon Motion Systems. https://docs.vicon.com/display/Nexus25/PDF+downloads+for+Vicon+Nexus?preview=/50888706/50889377/Plug-in%20Gait%20Reference%20Guide.pdf
- Winter, D. A. (2009). Biomechanics and motor control of human movement. John Wiley & Sons.
- Woltring, H. J. (1986). A fortran package for generalized, cross-validatory spline smoothing and differentiation. Advances in Engineering Software, 8(2), 104–113. https://doi.org/10.1016/0141-1195(86)90098-7
- Young, W., & Elliott, S. (2001). Acute effects of static stretching, proprioceptive neuromuscular facilitation stretching, and maximum voluntary contractions on explosive force production and jumping performance. Research Quarterly for Exercise and Sport, 72(3), 273–279. https://doi.org/10.1080/02701367.2001.10608960
- Young-Kwan, K. I. M., & Yoon Hyuk, K. I. M. (2011). Taekwondo biomechanics: Intersegmental joint coordination and hopping effect [Paper presented]. The Spring conference, Daejeon, South Korea.
- Yuncza, E. (2011). Sport karate point sparring: An essential guide to the point fighting method. Indomitable.
Appendix A. Detailed explanation of the kinetic model for hip moment calculation during the spreading motion of the Flexibility Trainer exercise, see
Table