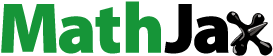
ABSTRACT
This study examined the effects of fatigue on hamstring muscles and gluteus maximus passive and active shear modulus in hip extension (HE) and knee flexion (KF) at 20% of maximal voluntary isometric contraction performed until task failure. Measurements were taken before and after the fatigue tasks and the delta (post-pre) was calculated. No differences in the fatigue effects on passive shear modulus were seen between muscles nor between tasks. For the active shear modulus: a task × muscle interaction was seen (p = 0.002; η2p = 0.401). The results for the tasks separately demonstrated only a significant effect for muscle in KF (p < 0.001; η2p = 0.598), with different individual contributions identified between BFlh-SM (p = 0.006; d = 1.10), BFlh-ST (p = 0.001; d = 1.35) and SM-ST (p = 0.020; d = 0.91). The comparisons between tasks for each muscle demonstrated significant differences for SM (p = 0.025; d = 0.60) and ST (p = 0.026; d = 0.60); however, no differences were seen for BFlh (p = 0.062; d = 0.46). Therefore, fatigue effects induce different patterns on the hamstring muscles in HE and KF tasks when performed at 20% MVIC.
Introduction
The hamstrings are a muscle group which act simultaneously at two joints by participating in knee flexion (KF) and hip extension (HE). Previous studies have reported that the patterns of hamstring muscle recruitment diverge significantly between different exercises. Indeed, exercises that mobilise the hip joint (e.g., stiff leg deadlift) seem to mobilise the biceps femoris long head (BFlh) and semimembranosus (SM) more (Fernandez-Gonzalo et al., Citation2016; Ono et al., Citation2011), while a greater recruitment of semitendinosus (ST) and biceps femoris short head (BFsh) seems to occur in knee-dominant exercises (e.g., prone leg curl) (Bourne et al., Citation2017; Fernandez-Gonzalo et al., Citation2016; Schuermans et al., Citation2014). However, differences in hamstring response between hip- and knee-dominant tasks have not been sufficiently explored from a mechanical perspective, especially when under fatigue.
Ultrasound-based shear wave elastography (SWE) has been proposed as a valid and reliable method to assess the hamstring muscles passive (i.e, at rest) and active (i.e., during contraction) shear modulus (Evangelidis et al., Citation2021; Miyamoto et al., Citation2020). Considering the muscle force-shear modulus (linear) relationship (Bouillard et al., Citation2011), previous studies have examined the muscle active shear modulus using SWE to determine if fatiguing exercises could alter could alter the neuromuscular coordination mechanism and lead to an adaptation in the control strategy over the synergistic muscles (Bouillard et al., Citation2012; Morel et al., Citation2019) i.e., the load sharing strategy (Stutzig & Siebert, Citation2015). It is speculated that this mechanism leads to a predominant activation of a muscle or group of muscles, which in turn can eventually cause tissue damage and manifest injury, especially under conditions of repeated effort.
Regarding active shear modulus assessment, two previous studies were conducted on two hamstring muscles (i.e., BFlh and ST) using SWE in isometric KF submaximal tasks (Mendes et al., Citation2018, Citation2020). The first assessed hamstrings’ active shear modulus across several submaximal intensities and noted that the ST generally showed a greater active shear modulus compared to BFlh, especially at low intensities (i.e., <40% of maximal voluntary isometric contraction, MVIC) (Mendes et al., Citation2018). The second found that after a KF fatigue task at 20% of MVIC, the active shear modulus of the ST decreases without changes in BFlh shear modulus, thus increasing the BFlh/ST ratio (Mendes et al., Citation2020). Together, these two studies indicate that BFlh/ST active shear modulus ratio is sensitive to both contraction intensity and fatigue. However, as the hamstrings shear modulus pattern seems to be different between KF and HE exercises (Bourne et al., Citation2017; Fernandez-Gonzalo et al., Citation2016; Ono et al., Citation2011; Schuermans et al., Citation2014), it should be interesting to examine whether these findings also apply to HE exercises. In addition, several aspects were not considered in the aforementioned studies. Firstly, passive muscle shear modulus was not assessed; thus, the resting state of the tested muscles on HE remains unclear. Secondly, only the BFlh and ST were examined, and the effect of fatigue was not tested between hip- and knee-dominant tasks in other hamstring muscles (i.e., SM and BFsh). Furthermore, it is well known that HE involves a great contribution from gluteus maximus (GM) (Neto et al., Citation2020), which has been reported to be crucial in the acceleration phase of sprinting, playing a key role in the forward orientation ground force (horizontal force) production (Dorn et al., Citation2012; Hamner & Delp, Citation2013). It should be noted that the main mechanism of hamstring strain injury is the sprint (Brooks et al., Citation2006). However, it is unknown whether fatigue could alter the GM passive and active shear modulus and be considered in a load sharing mechanism, which could be important in understanding hamstring strain injuries and developing rehabilitation strategies.
This study analysed the effects of fatigue on BFlh, BFsh, GM, SM, and ST passive and active shear modulus in HE and KF tasks at 20% MVIC until task failure. We hypothesised that: i) the effects of the fatigue tasks would induce different changes in the active shear modulus pattern. To test this, we conducted two experiments. We expected ii) no changes would be seen in passive shear modulus; iii) a decrease in active shear modulus of the BFlh and SM after the HE task; iv) an increased active shear modulus of the BFlh and a decrease of the ST after the KF task.
Methods
Participants
Fifteen physically active males (age: 23.3 ± 3.5 years; height: 174.3 ± 5.2 cm; body mass: 70.7 ± 9.5 kg) without a hamstring strain injury history were invited to participate in this study. The sample size was based on a previous methodological study, with 80% statistical power, effect size of 0.69 (G*Power software, v3.1.9.2). Participants visited the laboratory twice at least seven days apart. In each session, individuals performed either a KF or an HE task until failure in a random order. All participants read and signed an informed consent form prior to participating in the study. The local ethics committee approved the study (#9/2019).
Procedures
Dynamometry
Experiment I
An isokinetic dynamometer (System 3; Biodex Medical Systems, Shirley, NY) was used to assess the joint torque during an MVIC. For the hip dominant task (Experiment I) participants were positioned in the prone position with both knees fully extended and the measured hip flexed at 10° (). Participants were instructed verbally throughout the task to perform hip extension without flexing. The dynamometer axis was aligned with the greater trochanter. Moreover, this position was chosen based on the comfort and ability of the individuals to produce force.
Figure 1. Shear modulus assessment at 20% of MVIC before the fatigue task for semimembranosus (SM), semitendinosus (ST), biceps femoris long head (BFlh), biceps femoris short head (BFsh) and gluteus maximus (GM) for the hip extension (left) and knee flexion (right) exercises. Plastic casts (fixed to the skin with bi-adhesive tape) were used to ensure that repeated muscle shear modulus measurements would be performed at the same site. For the hip extension task, the attachment position should be as proximal to the knee joint as possible to avoid a knee flexion contribution; however, in assessing the shear modulus of all hamstrings muscles the attachment position could not be placed closer due to the cast’s placement which is critical to ensure a stable measure. Moreover, the femur was not fixed during the KF task to allow for cast placement and avoid external pressure from influencing SWE measurements. The red line within the elastogram window corresponds to the area used for calculating the average shear modulus.
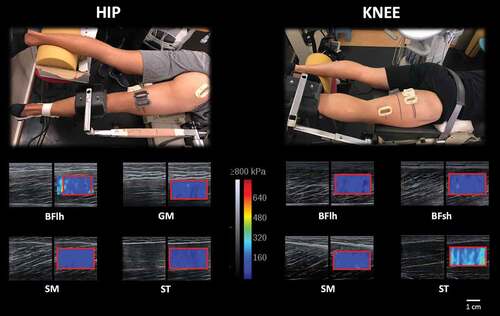
Experiment II
An isokinetic dynamometer (System 3; Biodex Medical Systems, Shirley, NY) was used to assess the joint torque at a sampling rate of 1000 Hz. For experiment II, participants were positioned in the prone position with the hip in neutral anatomical position, the measured knee flexed at 30° (), and with an orthosis positioned in the ankle~15º of plantar flexion to restrain dorsiflexion movements during contraction. This position was chosen since hamstrings are in a slack length (Silder et al., Citation2007). Moreover, hamstring injuries occur more frequently during the late swing phase of sprint at 30º of KF (Kenneally‐Dabrowski et al., Citation2019), being the most common injury mechanism (Garrett et al., Citation1984; Stanton & Purdham, Citation1989).
The dynamometer axis was aligned with the lateral femoral condyle. This position was chosen based on the ease in performing the shear wave measurements reliably (Mendes et al., Citation2018).
Protocol
In each experiment, testing began by assessing the muscles’ passive (i.e., muscles in rest condition) shear modulus; two 10-s video clips were obtained for each muscle at rest. Participants then performed 20 submaximal contractions, followed by two MVICs with 3-s duration and 1 min between trials. Based on the MVIC highest peak torque, individuals familiarised themselves with the 20% of MVIC trials using visual feedback. Each trial lasted~30 s. The active shear modulus at 20% of MVIC was then measured twice for each muscle. An isometric contraction at 20% of MVIC was then performed until task failure, which was defined as when the participant could not sustain the given intensity (i.e., a decrease higher than 5% of MVIC, visually controlled on a screen) for more than 3 s, even after verbal and visual feedback. Immediately after the fatigue task, the same passive and active shear modulus testing was conducted. The order of tasks and measurements in each muscle pair were randomised. To ensure peripheral fatigue, the intensity of the task failure was determined at 20%MVIC since a higher percentage would lead to a lower time until exhaustion and consequently less peripheral effects (Bigland-Ritchie & Woods, Citation1984; Gandevia, Citation2001). Moreover, higher intensities would mean the impossibility of performing 8 trials with~30s of contraction to measure the shear modulus values, considering that it was reported that a minimum of 25 s of contraction are needed to achieve a stable measurement (Mendes et al., Citation2018).
Shear wave elastography
Muscle shear modulus was assessed using two similar ultrasound scanners (Aixplorer, v11; Supersonic Imagine, Aix-en-Provence, France) in SWE mode [musculoskeletal preset, penetrate mode, smoothing level 5, opacity 100%, scale: 0–800 kPa for active (i.e., during contraction) and 0–100 kPa for passive (i.e., at rest) conditions), coupled with a linear transducer array (SL10–2, 2–10 MHz. Super Linear, Vermon, Tours, France). The transducer was placed at~43% (SM), ~55% (BFlh), ~55% (ST), and~20% (BFsh) of the distal-to-proximal femur length (Mendes et al., Citation2018). For the GM, the transducer was placed at 50% of the length between the ipsilateral posterior superior iliac spine and greater trochanter (Tayashiki et al., Citation2017). Shear modulus calculations assume isotropy of the underlying tissue; it is known, however, that muscle is anisotropic, since the mechanical properties of muscle fibres are direction-dependent (Brandenburg et al., Citation2014; Kotadia et al., Citation2020). The anisotropy of skeletal muscle therefore requires a longitudinal transducer orientation in SWE, aligned with the fascicle’s orientation (since the shear waves preferably propagate in their direction), to obtain accurate shear modulus values (Couade et al., Citation2011; Gennisson et al., Citation2010; Lee et al., Citation2012). Therefore, caution was taken to align the transducer with the muscle’s fascicles orientation, and to perform minimal pressure during the measurements.
Data acquisition and processing
Dynamometry and elastography data were synchronised using an external switch that simultaneously started data collection for all equipment and acquired using a Biopac MP100 acquisition system (Biopac, Santa Barbara, CA). Data were processed using automated MATLAB routines (The Mathworks Inc., Natick, MA). The shear modulus values were calculated from shear wave velocity (V). The velocity of shear waves generated by an ultrasonic beam (i.e, ‘pushing beam’ emitted by the probe) within the muscle are measured by a time-of-flight algorithm in each pixel of the elastogram map in ultrafast ultrasound sequences. Shear modulus was estimated using the following equation (Bercoff et al., Citation2004):
where is the muscle mass density (1000 kg/m3). It should be noted that the machine output is Young’s modulus, which corresponds to a linear displacement, whereas the shear modulus corresponds to an angular displacement. Notice that Young’s modulus and shear modulus are related (Bercoff et al., Citation2004):
being E the Young’s modulus and G the shear modulus (Shiina et al., Citation2015; Sigrist et al., Citation2017). The shear modulus is the ratio of shear stress over shear strain. Since muscle strains occur due to a shear force on the muscle fibres, shear modulus is often used in muscle rigidity analysis and consequent injury. For the shear modulus calculation, each clip exported from Aixplorer’s software was sequenced in.jpeg images. Image processing converted each pixel of the colour map into a value of the Young’s modulus based on the recorded (ultrasound) colour scale. The largest ROI in the elastogram window was determined manually by an experienced examiner avoiding aponeuroses and tissue artefacts (e.g., vessels) and the values were averaged to obtain a representative muscle value. Within each trial, the most stable values with ~ 20 s duration were averaged and divided by 3 to estimate the muscle shear elastic modulus (Bercoff et al., Citation2004).
Statistical analysis
Data analysis was performed using IBM SPSS Statistics 25.0 (IBM Corporation, Armonk, NY). Normality of data distribution was assessed using the Shapiro—Wilk test. To verify the existence of fatigue a paired sample t-test was performed on MVC for each task. To assess the effect of fatigue in each muscle a delta (Δ) active shear modulus (post-pre) was calculated for each muscle. The effect of fatigue in each task (HE and KF) on the biarticular muscles (BFlh, SM and ST) in passive and active shear modulus were examined by conducting a two-way ANOVA (task muscle) for each condition (passive and active). Post-hoc analysis was conducted using Bonferroni correction to determine the differences between muscles when a significant main effect was observed. Where an interaction was found, the two tasks were analysed separately using a one-way ANOVA followed by post-hoc analysis using Bonferroni correction. For each muscle a paired sample t-test was conducted. Additionally, for the comparison of the Δ active shear modulus of the monoarticular muscles (BFsh and GM) a one way ANOVA was performed for each task (HE and KF) and condition (passive and active). Post-hoc analysis was conducted using Bonferroni correction to determine the differences between muscles when a significant main effect was observed. The partial eta square (η2p) values were reported as a measure of the effect size of the ANOVA’s findings, classified as small (η2p = 0.01–0.05), medium (η2p = 0.06–0.013), and large (η2p>0.14) effects (Cohen, Citation1988). Cohen’s d effect sizes were determined and classified as small (d = 0.2–0.5), medium (d = 0.5–0.8), and large (d ≥ 0.8) for normally distributed data based on benchmarks suggested by Cohen (Citation1988). Data are presented as mean ± standard deviation. Significance was set at p < 0.05.
Results
Two participants were excluded in the data processing due to the quality requirements (age: 23.9 ± 3.2 years; height: 174.1 ± 5.2 cm; body mass: 70.6 ± 9.9 kg). For the GM, the transducer was positioned at 45.3 ± 6.5% of the length between the ipsilateral posterior superior iliac spine and greater trochanter. The testing sessions were separated by 12 ± 10 days.
Task performance
Time until failure was 946.2 ± 769.2 s and 504.7 ± 257.3 s in the HE and KF tasks, respectively. The MVIC decreased 22.9% after the HE task (pre: 179.2 ± 58.6 Nm; post: 138.2 ± 58.5 Nm; p = 0.004; d = 0.70), and 19.8% after the KF task (pre: 84.4 ± 23.5 Nm; post: 67.7 ± 22.1 Nm; p = 0.001; d = 0.73).
Passive shear modulus
In respect to the monoarticular muscles, no significant effect was found in KF between muscles (p = 0.452; η2p = 0.070), however a significant effect in HE (p = 0.038; η2p = 0.207) with the post-hoc analysis demonstrated no differences across all hamstrings muscles. Regarding the biarticular () muscles no significant task muscle interaction was found (p = 0.437; η2p = 0.067) as well as for task (p = 0.404; η2p = 0.059) and muscle (p = 0.079; η2p = 0.191) effects.
Active shear modulus
In respect to the monoarticular muscles, no significant effect was found in HE between muscles (p = 0.268; η2p = 0.102). A significant effect for muscle was observed in the KF task (p < 0.001; η2p = 0.445), however the post-hoc analysis showed no differences between the BFsh and all the other hamstrings muscles. In relation to the biarticular muscles (), a significant task muscle interaction was seen (p = 0.002; η2p = 0.401). Since an interaction was found, the two tasks were analysed separately and no effect was found for HE (p = 0.099; η2p = 0.175), whereas for KF a effect for muscle was seen (p < 0.001; η2p = 0.598). The post-hoc analysis showed differences between BFlh-SM (p = 0.006; d = 1.10), BFlh-ST (p = 0.001; d = 1.35) and SM-ST (p = 0.020; d = 0.91). The comparisons between tasks for each muscle demonstrated significant differences for SM (p = 0.025; d = 0.60) and ST (p = 0.026; d = 0.60); however, no differences were seen for BFlh (p = 0.062; d = 0.46).
Discussion and practical implications
To the best of our knowledge, this is the first study to examine the effects of fatigue on passive and active (at 20% of MVIC) shear modulus of all hamstring muscles plus the GM before and after both HE and KF fatigue protocols. The main findings were (i) no differences were detected for the fatigue effects (post-pre) on passive shear modulus between tasks and muscles; (ii) for the active shear modulus of biarticular muscles, the fatigue effects (post-pre) showed differences between all hamstring muscles in KF, with no significant fatigue effects between muscles in HE; (iii) the SM and ST demonstrated significantly different contributions between tasks.
Concerning the effects of fatigue on passive shear modulus, as hypothesised, no differences were seen between muscles, indicating that in the rest condition the contribution of all hamstring muscles is similar. In respect to the KF position, this is expected since the hamstrings are at a slack length (Silder et al., Citation2007). Despite the hamstrings being in a more lengthening position during HE, the contribution was similar, suggesting that the pattern tends to adapt to be homogenous in rest condition across all the hamstrings.
It is interesting that in the active shear modulus, no changes were seen in the mono-articular muscles (BFsh and GM) in both tasks, indicating that fatigue minimally impacted these muscles which did not lose nor reinforce their ability to produce work during the corresponding fatigue tasks.
Regarding the differences in contribution by each muscle between tasks, the SM and ST demonstrated opposite behaviour: while the ST showed a higher contribution in KF (negative Δ) without changes in HE, the SM showed a higher contribution after the task for HE (negative Δ) without changes in KF. Moreover, the BFlh showed no differences between tasks. It should be noted that a decrease in active shear modulus seems to reflect changes in localised muscle fatigue (Bouillard et al., Citation2014; Morel et al., Citation2019). This means that the ability of the contractile element to generate sarcomere cross-bridges is reduced. These results are mostly concordant with previous literature as exercises mobilising the knee joint seems to have a greater contribution of ST (Bourne et al., Citation2017; Fernandez-Gonzalo et al., Citation2016; Schuermans et al., Citation2014) and exercises mobilising the hip joint tend to have a greater contribution from the BFlh and SM (Fernandez-Gonzalo et al., Citation2016; Ono et al., Citation2011). Thus, it was expected that fatigue would impact the contribution of these muscles in each task; however, this was only verified for the ST (KF) and SM (HE). The SM has a greater capacity to produce force due to having the largest cross-sectional area among the hamstring muscles (Woodley & Mercer, Citation2005) and shorter pennated fibres (Kellis et al., Citation2012). Indeed, the SM is the greatest contributor for hip active force (Kellis & Blazevich, Citation2022). Moreover, the number and type of muscle fibres in the SM and ST could be relevant in the contribution observed between fatigue tasks. Regarding ST, it has been reported to have a considerably higher proportion of fast-twitch fibres (Smith et al., Citation2011) which led to a lower resistance of the ST to the fatigue during the KF task, as reflected in the decrease in active shear modulus. It should be noted that these types of observations are scarce in the literature for the hamstrings, especially regarding the SM—only Garrett et al. (Citation1984) has reported an average of 51% type II fibres in the SM across 10 individuals (Garrett et al., Citation1984). However, these 10 individuals (7 males and 3 females) were an average of 60 years of age, and it has been shown that the fast-twitch fibre (type II) content decreases with age (Larsson, Citation1978). Furthermore, sex differences have been demonstrated in the fast-twitch fibre content (Fournier et al., Citation2022) – with a higher fast-twitch fibre content in males for other hamstring muscles (ST) – further supported by the higher fatigue rate exhibited by males in knee flexion and extension (Pincivero et al., Citation2003). Since the participants in this study were young and physically active males, a higher percentage of fast-twitch fibres in the SM is expected, resulting in a lower resistance of the SM to the fatigue during the HE task, as reflected in the decrease in active shear modulus. This warrants further investigation.
Regarding the comparison between muscles when the tasks were analysed separately, it is interesting to observe that the active shear modulus of all bi-articular muscles (i.e., BFlh, SM, and ST) was only affected by the fatigue protocol in KF, although in different directions (). In relation to HE similar changes of contribution were seen for all muscles between pre-post conditions suggesting a minimal effect of fatigue. A sharing strategy was detected in KF since a negative Δ was seen for the ST, reflecting a decrease in ST, whereas a positive Δ was observed for the BFlh, reflecting an increase of BFlh shear modulus after the fatigue task without changes in the SM. The significant difference between SM-BFlh and between SM-ST can be explained due to the maintained contribution pre-to-post task by SM with the opposite behaviours of BFlh and ST. More specifically, the BFlh-ST pair also display almost identical fatigue-induced effects in the HE task (similar Δ), emphasising the importance of their differential response to fatigue in the KF task. This load-sharing strategy has been previously reported (Schuermans et al., Citation2014). One possible explanation for these results in KF could be the muscle fibres composition for each muscle. Indeed, the BFlh seems to have a considerable high number of type I/IIa fibres (Dahmane et al., Citation2005; Evangelidis et al., Citation2017). On other hand ST has been reported to have a considerably higher proportion of fast-twitch fibres (Smith et al., Citation2011). Therefore, both muscles could have a consistently different proportion of slow/fast twitch fibres, making the ST a less fatigue-resistant muscle and a less fatigable muscle as the case of BFlh (i.e., with a higher proportion of slow-twitch fibres) should play a greater role in maintaining workload when fatigue is installed. Moreover, in the previous study by Mendes et al. (Citation2020), the BFlh active shear modulus was unaltered while the ST active shear modulus decreased after a KF fatigue protocol. In the present study, the ST active shear modulus decreased and the BFlh increased in the KF task. In the study by Mendes et al. (Citation2020), only a small to moderate MVIC decrease was observed after the KF fatigue task (i.e., between −3.8% and −7.8% in two different days). In the present study, a large decrease was observed in both tasks (−22.9% and −19.8% after HE and KF, respectively). This indicates a greater fatigue effect, which might have potentiated the higher post-task contribution of BFlh active shear modulus. It should be noted that the muscle shear modulus during contraction has been well correlated with electromyographic activity (Nordez & Hug, Citation2010) and with force production independently of the fatigue effects (Bouillard et al., Citation2012). Thus, we speculate that the BFlh workload increases with hamstring fatigue, possibly elevating its injury risk. It is interesting to note that, among the hamstring muscle components, the injury incidence is higher for the BFlh (Koulouris et al., Citation2007; Malliaropoulos et al., Citation2010). Therefore, physical activities involving the hamstrings by mobilising both knee and hip and leading to fatigue (e.g., repeated sprinting) might favour a higher contribution of BFlh active shear modulus and, consequently, put the BFlh at higher risk of injury. This hypothesis should be examined in a future study.
The present study has some limitations. Firstly, although a considerable number of muscles were assessed, we cannot disregard the participation of other synergistic muscles crossing both the knee and hip joints which might have affected the results. Secondly, shear modulus measurements were performed at a single site within each muscle, and it is known that active shear modulus differences exist within the muscle (Vaz et al., Citation2021). Thus, we are unaware whether the fatigue effects on muscle shear modulus are also region dependent. Thirdly, the contraction intensity used in the fatigue protocol and for shear wave measurements during contraction was submaximal. We are unaware whether responses could be different at other contraction intensities. Fourthly, the positions adopted in KF and HE tasks placed the hamstrings at different lengths, influencing their point on the force-length relationship. Additionally, as the absence of knee flexion in HE and of hip extension in KF were done via visual inspection, the influence of either on the other could not be objectively confirmed. As such, interpretation of the study findings should be performed under this consideration. However, it should be considered that the attachment position was placed to be able measure all the four hamstrings’ muscles and the gluteus maximus, and the placed strap during knee flexion immobilised the hip. Finally, since the study sample only included young males, the results cannot be generalised to other groups.
In conclusion, this study provides scientific evidence that HE and KF exercises seem to have a different active shear modulus response pattern to a submaximal isometric contraction at 20% of MVIC. The HE task produced a similar behaviour across all hamstring muscles, and the KF task resulted in a Δ decrease of the ST, an Δ increase of the BFlh and a similar Δ of the SM which showed that the active shear modulus pattern is changed with a submaximal isometric fatigue protocol. Future researchers should consider the effect of fatigue on the hamstrings active shear modulus response pattern in different joints, in order to translate these results into not only improving sports performance as well as in the process of rehabilitation, but also exploring a potential link to the occurrence of hamstring strain injuries.
Acknowledgments
The authors thank the study participants for their participation, to Sport Lisboa e Benfica for lending one Aixplorer unit, and to Professor Mauricio Cerda for the MATLAB code.
Disclosure statement
No potential conflict of interest was reported by the author(s).
Additional information
Funding
References
- Bercoff, J., Tanter, M., & Fink, M. (2004). Supersonic shear imaging: A new technique for soft tissue elasticity mapping. IEEE Transactions on Ultrasonics, Ferroelectrics, and Frequency Control, 51(4), 396–409. https://doi.org/10.1109/TUFFC.2004.1295425
- Bigland-Ritchie, B., & Woods, J. J. (1984). Changes in muscle contractile properties and neural control during human muscular fatigue. Muscle & Nerve, 7(9), 691–699. https://doi.org/10.1002/mus.880070902
- Bouillard, K., Hug, F., Guével, A., & Nordez, A. (2012). Shear elastic modulus can be used to estimate an index of individual muscle force during a submaximal isometric fatiguing contraction. Journal of Applied Physiology, 113(9), 1353–1361. https://doi.org/10.1152/japplphysiol.00858.2012
- Bouillard, K., Jubeau, M., Nordez, A., & Hug, F. (2014). Effect of vastus lateralis fatigue on load sharing between quadriceps femoris muscles during isometric knee extensions. Journal of Neurophysiology, 111(4), 768–776. https://doi.org/10.1152/jn.00595.2013
- Bouillard, K., Nordez, A., & Hug, F. (2011). Estimation of individual muscle force using elastography. PLoS ONE, 6(12), e29261. https://doi.org/10.1371/journal.pone.0029261
- Bourne, M. N., Williams, M. D., Opar, D. A., Al Najjar, A., Kerr, G. K., & Shield, A. J. (2017). Impact of exercise selection on hamstring muscle activation. British Journal of Sports Medicine, 51(13), 1021–1028. https://doi.org/10.1136/bjsports-2015-095739
- Brandenburg, J. E., Eby, S. F., Song, P., Zhao, H., Brault, J. S., Chen, S., & An, K. -N. (2014). Ultrasound elastography: The new frontier in direct measurement of muscle stiffness. Archives of Physical Medicine and Rehabilitation, 95(11), 2207–2219. https://doi.org/10.1016/j.apmr.2014.07.007
- Brooks, J. H. M., Fuller, C. W., Kemp, S. P. T., & Reddin, D. B. (2006). Incidence, risk, and prevention of hamstring muscle injuries in professional rugby union. The American Journal of Sports Medicine, 34(8), 1297–1306. https://doi.org/10.1177/0363546505286022
- Cohen, J. (1988). Statistical power analysis for the behavioral sciences (2nd ed.). Routledge.
- Couade, M., Pernot, M., Messas, E., Bel, A., Ba, M., Hagege, A., Fink, M., & Tanter, M. (2011). In vivo quantitative mapping of myocardial stiffening and transmural anisotropy during the cardiac cycle. IEEE Transactions on Medical Imaging, 30(2), 295–305. https://doi.org/10.1109/TMI.2010.2076829
- Dahmane, R., Djordjevič, S., Šimunič, B., & Valenčič, V. (2005). Spatial fiber type distribution in normal human muscle. Journal of Biomechanics, 38(12), 2451–2459. https://doi.org/10.1016/j.jbiomech.2004.10.020
- Dorn, T. W., Schache, A. G., & Pandy, M. G. (2012). Muscular strategy shift in human running: Dependence of running speed on hip and ankle muscle performance. The Journal of Experimental Biology, 215(Pt 11), 1944–1956. https://doi.org/10.1242/jeb.064527
- Evangelidis, P. E., Massey, G. J., Ferguson, R. A., Wheeler, P. C., Pain, M. T. G., & Folland, J. P. (2017). The functional significance of hamstrings composition: Is it really a “fast” muscle group? Scandinavian Journal of Medicine & Science in Sports, 27(11), 1181–1189. https://doi.org/10.1111/sms.12786
- Evangelidis, P. E., Shan, X., Otsuka, S., Yang, C., Yamagishi, T., & Kawakami, Y. (2021). Hamstrings load bearing in different contraction types and intensities: A shear-wave and B-mode ultrasonographic study. PLoS ONE, 16(5), e0251939. https://doi.org/10.1371/journal.pone.0251939
- Fernandez-Gonzalo, R., Tesch, P. A., Linnehan, R. M., Kreider, R. B., DiSalvo, V., Suarez-Arrones, L., Alomar, X., Mendez-Villanueva, A., & Rodas, G. (2016). Individual muscle use in hamstring exercises by soccer players assessed using functional MRI. International Journal of Sports Medicine, 37(7), 559–564. https://doi.org/10.1055/s-0042-100290
- Fournier, G., Bernard, C., Cievet-Bonfils, M., Kenney, R., Pingon, M., Sappey-Marinier, E., Chazaud, B., Gondin, J., & Servien, E. (2022). Sex differences in semitendinosus muscle fiber-type composition. Scandinavian Journal of Medicine & Science in Sports, 32(4), 720–727. https://doi.org/10.1111/sms.14127
- Gandevia, S. C. (2001). Spinal and supraspinal factors in human muscle fatigue. Physiological Reviews, 81(4), 1725–1789. https://doi.org/10.1152/physrev.2001.81.4.1725
- Garrett, W. E., Jr., Califf, J. C., & Bassett, F. H., 3rd. (1984). Histochemical correlates of hamstring injuries. The American Journal of Sports Medicine, 12(2), 98–103. https://doi.org/10.1177/036354658401200202
- Gennisson, J. -L., Deffieux, T., Macé, E., Montaldo, G., Fink, M., & Tanter, M. (2010). Viscoelastic and anisotropic mechanical properties of in vivo muscle tissue assessed by supersonic shear imaging. Ultrasound in Medicine & Biology, 36(5), 789–801. https://doi.org/10.1016/j.ultrasmedbio.2010.02.013
- Hamner, S. R., & Delp, S. L. (2013). Muscle contributions to fore-aft and vertical body mass center accelerations over a range of running speeds. Journal of Biomechanics, 46(4), 780–787. https://doi.org/10.1016/j.jbiomech.2012.11.024
- Kellis, E., & Blazevich, A. J. (2022). Hamstrings force-length relationships and their implications for angle-specific joint torques: A narrative review. BMC Sports Science, Medicine and Rehabilitation, 14(1), 166. https://doi.org/10.1186/s13102-022-00555-6
- Kellis, E., Galanis, N., Kapetanos, G., & Natsis, K. (2012). Architectural differences between the hamstring muscles. Journal of Electromyography and Kinesiology: Official Journal of the International Society of Electrophysiological Kinesiology, 22(4), 520–526. https://doi.org/10.1016/j.jelekin.2012.03.012
- Kenneally‐Dabrowski, C. J. B., Brown, N. A. T., Lai, A. K. M., Perriman, D., Spratford, W., & Serpell, B. G. (2019). Late swing or early stance? A narrative review of hamstring injury mechanisms during high‐speed running. Scandinavian Journal of Medicine & Science in Sports, 29(8), 1083–1091. https://doi.org/10.1111/sms.13437
- Kotadia, I., Whitaker, J., Roney, C., Niederer, S., O’neill, M., Bishop, M., & Wright, M. (2020). Anisotropic cardiac conduction. Arrhythmia & Electrophysiology Review, 9(4), 202–210. https://doi.org/10.15420/aer.2020.04
- Koulouris, G., Connell, D. A., Brukner, P., & Schneider-Kolsky, M. (2007). Magnetic resonance imaging parameters for assessing risk of recurrent hamstring injuries in elite athletes. The American Journal of Sports Medicine, 35(9), 1500–1506. https://doi.org/10.1177/0363546507301258
- Larsson, L. (1978). Morphological and functional characteristics of the ageing skeletal muscle in man: A cross-sectional study.
- Lee, W. -N., Pernot, M., Couade, M., Messas, E., Bruneval, P., Bel, A., Hagege, A. A., Fink, M., & Tanter, M. (2012). Mapping myocardial fiber orientation using echocardiography-based shear wave imaging. IEEE Transactions on Medical Imaging, 31(3), 554–562. https://doi.org/10.1109/tmi.2011.2172690
- Malliaropoulos, N., Papacostas, E., Kiritsi, O., Papalada, A., Gougoulias, N., & Maffulli, N. (2010). Posterior thigh muscle injuries in elite track and field athletes. The American Journal of Sports Medicine, 38(9), 1813–1819. https://doi.org/10.1177/0363546510366423
- Mendes, B., Firmino, T., Oliveira, R., Neto, T., Cruz-Montecinos, C., Cerda, M., Correia, J. P., Vaz, J. R., & Freitas, S. R. (2020). Effects of knee flexor submaximal isometric contraction until exhaustion on semitendinosus and biceps femoris long head shear modulus in healthy individuals. Scientific Reports, 10(1). https://doi.org/10.1038/s41598-020-73433-1
- Mendes, B., Firmino, T., Oliveira, R., Neto, T., Infante, J., Vaz, J. R., & Freitas, S. R. (2018). Hamstring stiffness pattern during contraction in healthy individuals: Analysis by ultrasound-based shear wave elastography. European Journal of Applied Physiology, 118(11), 2403–2415. https://doi.org/10.1007/s00421-018-3967-z
- Miyamoto, N., Kimura, N., & Hirata, K. (2020). Non-uniform distribution of passive muscle stiffness within hamstring. Scandinavian Journal of Medicine & Science in Sports, 30(9), 1729–1738. https://doi.org/10.1111/sms.13732
- Morel, B., Hug, F., Nordez, A., Pournot, H., Besson, T., Mathevon, L., & Lapole, T. (2019). Reduced active muscle stiffness after intermittent submaximal isometric contractions. Medicine and Science in Sports and Exercise, 51(12), 2603–2609. https://doi.org/10.1249/MSS.0000000000002080
- Neto, W. K., Soares, E. G., Vieira, T. L., Aguiar, R., Chola, T. A., Sampaio, V. D. L., & Gama, E. F. (2020). Gluteus maximus activation during common strength and hypertrophy exercises: A systematic review. Journal of Sports Science & Medicine, 19(1), 195–203. https://doi.org/10.000/jssm-19-195.xml/3Eabst#
- Nordez, A., & Hug, F. (2010). Muscle shear elastic modulus measured using supersonic shear imaging is highly related to muscle activity level. Journal of Applied Physiology, 108(5), 1389–1394. https://doi.org/10.1152/japplphysiol.01323.2009
- Ono, T., Higashihara, A., & Fukubayashi, T. (2011). Hamstring functions during hip-extension exercise assessed with electromyography and magnetic resonance imaging. Research in Sports Medicine, 19(1), 42–52. https://doi.org/10.1080/15438627.2011.535769
- Pincivero, D. M., Gandaio, C. M. B., & Ito, Y. (2003). Gender-specific knee extensor torque, flexor torque, and muscle fatigue responses during maximal effort contractions. European Journal of Applied Physiology, 89(2), 134–141. https://doi.org/10.1007/s00421-002-0739-5
- Schuermans, J., Van Tiggelen, D., Danneels, L., & Witvrouw, E. (2014). Biceps femoris and semitendinosus–teammates or competitors? New insights into hamstring injury mechanisms in male football players: A muscle functional MRI study. British Journal of Sports Medicine, 48(22), 1599–1606. https://doi.org/10.1136/bjsports-2014-094017
- Shiina, T., Nightingale, K. R., Palmeri, M. L., Hall, T. J., Bamber, J. C., Barr, R. G., Castera, L., Choi, B. I., Chou, Y. -H., Cosgrove, D., Dietrich, C. F., Ding, H., Amy, D., Farrokh, A., Ferraioli, G., Filice, C., Friedrich-Rust, M., Nakashima, K., Schafer, F., … Kudo, M. (2015). WFUMB guidelines and recommendations for clinical use of ultrasound elastography: Part 1: Basic principles and terminology. Ultrasound in Medicine & Biology, 41(5), 1126–1147. https://doi.org/10.1016/j.ultrasmedbio.2015.03.009
- Sigrist, R. M. S., Liau, J., Kaffas, A. E., Chammas, M. C., & Willmann, J. K. (2017). Ultrasound elastography: Review of techniques and clinical applications. Theranostics, 7(5), 1303–1329. https://doi.org/10.7150/thno.18650
- Silder, A., Whittington, B., Heiderscheit, B., & Thelen, D. G. (2007). Identification of passive elastic joint moment-angle relationships in the lower extremity. Journal of Biomechanics, 40(12), 2628–2635. https://doi.org/10.1016/j.jbiomech.2006.12.017
- Smith, L. R., Lee, K. S., Ward, S. R., Chambers, H. G., & Lieber, R. L. (2011). Hamstring contractures in children with spastic cerebral palsy result from a stiffer extracellular matrix and increasedin vivosarcomere length. The Journal of Physiology, 589(10), 2625–2639. https://doi.org/10.1113/jphysiol.2010.203364
- Stanton, P., & Purdham, C. (1989). Hamstring injuries in sprinting - the role of eccentric exercise. The Journal of Orthopaedic and Sports Physical Therapy, 10(9), 343–349. https://doi.org/10.2519/jospt.1989.10.9.343
- Stutzig, N., & Siebert, T. (2015). Muscle force compensation among synergistic muscles after fatigue of a single muscle. Human Movement Science, 42, 273–287. https://doi.org/10.1016/j.humov.2015.06.001
- Tayashiki, K., Hirata, K., Ishida, K., Kanehisa, H., & Miyamoto, N. (2017). Associations of maximal voluntary isometric hip extension torque with muscle size of hamstring and gluteus maximus and intra-abdominal pressure. European Journal of Applied Physiology, 117(6), 1267–1272. https://doi.org/10.1007/s00421-017-3617-x
- Vaz, J. R., Neto, T., Correia, J. P., Infante, J., & Freitas, S. R. (2021). Regional Differences in Biceps Femoris Long Head Stiffness during Isometric Knee Flexion. Journal of Functional Morphology and Kinesiology, 6(1). https://doi.org/10.3390/jfmk6010018
- Woodley, S. J., & Mercer, S. R. (2005). Hamstring muscles: Architecture and innervation. Cells, Tissues, Organs, 179(3), 125–141. https://doi.org/10.1159/000085004