ABSTRACT
This study investigated the impact of performing a closed kinetic chain with the lower limbs on isometric upper-limb pull and push strength. Sixty-two elite handcyclists were assessed with the Manual Muscle Test and allocated to groups with partial to normal (LLF) or no lower-limb (no-LLF) function. Both groups performed upper-limb strength measurements under two kinetic-chain conditions. During the closed-chain condition, the lower limbs were attached to two footrests, providing horizontal and vertical support. During the open-chain condition, the footrests were removed and the limbs were supported vertically by a horizontal plate. Repeated-measures ANOVA were conducted to investigate main effects (open vs. closed chain, LLF vs. no-LLF) and their interaction. During pull, LLF performed better (p < 0.001, +11%) by pushing against the footrests. However, this increase in pulling strength during a closed-chain condition was not observed in the no-LLF. Therefore, findings suggest an advantage for the least impaired athletes by being able to perform lower-limb closed chains during pulling. Handcyclists with LLF can maximise pulling performance by adjusting the footrests. The classification system should consider the implications of these findings on the allocation of athletes with different levels of LLF and/or on the equipment regulation.
Introduction
In Paralympic sports, a classification system aims to allocate athletes to different competition groups based on how much the sport-specific activity is limited as a consequence of the athlete’s impairment. By doing so, the classification system aims to minimise the impact of impairment on sports performance and to contribute to fair competition (International Paralympic Committee, Citation2015). Handcycling is composed of five sports classes starting with H5, the sport class consisting of athletes with the least activity limitation, to H1, the sports class including athletes with the greatest activity limitation (Union Cycliste Internationale, Citation2021). Athletes classified in H5 compete in an arm-trunk-powered handbike from an upright kneeling/sitting position, while athletes classified in the remaining classes compete in a recumbent position, in arm-powered handbikes (Union Cycliste Internationale, Citation2021).
The recumbent classes with the least impaired athletes (H3 and H4) present the greatest inter-class overlap in terms of handcycling performance, and differences among these groups’ average time-trial velocities appear to be small (R. E. A. Muchaxo et al., Citation2020). In accordance with the para-cycling regulations, the allocation of athletes to classes H3 or H4 is mainly centred on the athlete’s trunk strength and stability (Union Cycliste Internationale, Citation2021). Although trunk strength is an important determinant during class allocation, recent findings have suggested that trunk flexion strength and lesion level-based trunk function may only play a minor role in recumbent handcycling performance of elite athletes with no upper-limb strength impairments (Muchaxo et al., Citation2021).
One functional capacity that may be relevant in recumbent handcycling performance is the lower-limb function as part of a potential kinetic chain. The body segments are connected through joints and ligaments forming a kinetic chain that connects the whole body as a motor mechanism able to generate, sum, and transfer forces to the muscles responsible for the final action (Karandikar & Vargas, Citation2011). In tennis, for example, maximum power during a service can be achieved by transference and summation of ground reaction forces and muscle activity from the lower limbs and core to the hand (Van Der Hoeven & Kibler, Citation2006). Kinetic chains can be roughly categorised as open or closed depending on the body stabilisation during a movement and resistance/loading applied to the body extremities (Karandikar & Vargas, Citation2011). It has been demonstrated that the stabilisation of upper-limbs and trunk, i.e., forming a closed kinetic chain, leads to greater knee torque production in comparison with no stabilisation of these body segments, i.e., forming an open kinetic chain (Magnusson et al., Citation1993).
In H4, the athletes’ lower-limb muscle power varies, for example, from partial as a result of incomplete T12 spinal cord injury (SCI) to normal in the healthy leg of an athlete with a single amputation. This leads to an H4 class which includes athletes with no lower-limb function due to motor complete SCI, athletes with partial lower limb-function due to a motor incomplete SCI, and athletes with normal lower-limb function due to single lower-limb amputations. In addition to the lower-limb function range within this class, the para-cycling regulation stipulates that the use of leg and footrests must be used for protection of the lower limbs (Union Cycliste Internationale, Citation2021), but it does not refer to the implication of its use in combination with the different lower-limb function profiles.
In a study with able-bodied participants, it was shown that the ability to produce a closed kinetic chain by pushing off against the footrests () leads to a positive effect on the power output of able-bodied participants during handcycling isokinetic sprint tests (Kouwijzer et al., Citation2018). The ability to push off against the footrests with both legs, thus forming a closed kinetic chain, was compared to the condition in which they could not push off against the footrests, i.e., open chain. The closed-chain condition showed significantly increased peak (25%) and mean (11%) sprint power outputs. Moreover, the ability to push off with only one foot led to a similar increase in the peak and the mean power output of 22% and 10%, respectively, compared to not being able to push off (Kouwijzer et al., Citation2018). To the best of our knowledge, the study of Kouwijzer et al. (Citation2018) is the only study that investigated the potential effects of closed and open kinetic chains in a handcycling context. Although the effect sizes of the findings were small and derived from an able-bodied population, they initiated a debate around the possibly overlooked role of lower-limb function on recumbent handcycling performance and classification.
Figure 1. Examples of closed-chain (left pictures) and open-chain (right pictures) conditions on handbike footrests.
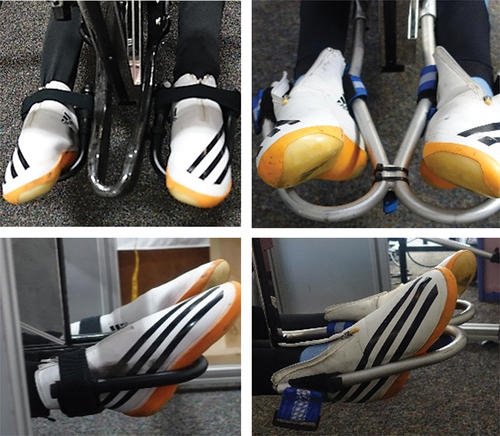
In a recent study investigating the upper-limb push and pull isometric strength characteristics in elite H1-H5 handcycling athletes, the maximum push and pull horizontal force was strongly associated with handcycling performance in a closed chain condition, explaining almost one-third of the variance in average time-trial velocity and 80% of the variance in isokinetic sprint power output (Muchaxo et al., Citation2022). It is the aim of the current study to investigate—within the same population and experimental design—the impact of closed-chain (CC) and open-chain (OC) conditions on the peak upper-limb force produced by elite handcyclists—according to the Participant Classification Framework of (McKay et al., Citation2022)—with different levels of lower-limb function, during standardised pull and push isometric strength tests. Furthermore, our subaim was to investigate the effect of performing a pull test with additional stability on the pelvic region but without footrests attached to the lower limbs, i.e., creating a CC condition on a pelvic contact point (PlvCC), on the peak upper-limb force produced.
We hypothesise that athletes who present partial to normal lower-limb function in at least one of the limbs will have an advantage on pull strength in a CC vs. OC condition (i.e., with vs. without a footrest attached) compared with athletes with no lower-limb function. During a push test, we hypothesise that the reaction force from pushing with the upper body against the backrest will compensate for the OC condition with the lower limbs and, therefore, the lower-limb kinetic chain will not lead to significantly greater forces. Lastly, in a subsample performing a pull test while creating a CC condition on a pelvic contact point (PlvCC), we hypothesise that the pelvic stabilisation partly compensates for the absence of footrests but that it is not as advantageous as the lower-limb CC in the group with partial to normal lower-limb function.
Methods
Participants
A convenience sample of 62 international handcycling athletes, recruited at the 2019 Para-cycling Road World Cup (Ostend, Belgium) and at the 2019 Para-cycling Road World Championship (Emmen, the Netherlands), participated in this study. As inclusion criteria, participants had to meet the following requirements: a) internationally classified in one of the existing handcycling sports classes; b) aged 18 years or older. In addition, exclusion criteria comprised a) presenting a health condition or active medical treatment that could influence the testing outcomes or the participant’s health during measurements; b) not being able to fully understand the aim and procedures. This study was approved by the Scientific and Ethical Review Board of the Faculty of Behavioural and Movement Sciences, of the Vrije Universiteit Amsterdam (VUA), with the number VCWE-2019-052, and all athletes provided written informed consent prior to voluntary participation.
Experimental protocol
Data collection took place in a controlled field-lab setting at the competition events and comprised a set of two measurements: a clinical method of strength assessment (Manual Muscle Test (MMT)), and an objective ratio-scale strength assessment in a ‘handcycle-based’ isometric testing device. Prior to testing, participants were interviewed regarding health and sports characteristics in addition to demographic information.
Manual muscle test
Following the assessment methodology approach during handcycling classification, an MMT was applied by a medical professional/handcycling classifier. According to Daniels and Worthingham’s techniques (Hislop & Montgomery, Citation2007), the clinical assessment of muscle strength is based on a break test, where the examiner applies manual resistance to the body structure tested. It comprises a grading scale from 0, no muscle activity, to 5, normal muscle response. Upper-limb strength was assessed regarding elbow flexion, elbow extension, and wrist flexion. In addition, a simplified hand function test (impaired/non-impaired) was used and consisted of a combined fingers flexion assessment. Lower-limb strength during hip flexion, hip extension, and knee extension was assessed.
Isometric ‘handcycle-based’ ratio-scale force assessment
The upper-limb strength was assessed using a ‘handcycle-based’ isometric device (ICC = O.99, CI = [0.99,0.99]), which was custom-made in collaboration with the technical department of Human Movement Sciences, VUA. The setup simulated a recumbent handcycling position, with a backrest, leg support, and a rigid beam with two 3D force-instrumented handlebars (MC3A-100, AMTI, USA) (). The handlebars had a diameter of 0.04 m, were tilted inwards to an angle of 30° with the vertical axis, and placed 0.47 m apart. The device allowed individual adjustments of the angle and height of the backrest, height of the handlebar frame, horizontal position of the seat with respect to the rigid beam, and lower-limb support.
Figure 2. Isometric force setup (left) and examples of upper-limb pull and push tasks (right). The upper graph (right) resembles the posture during the pulling phase of handcycling, i.e., hands low/close to the legs. The lower graph (right) resembles the posture during the pushing phase of handcycling when the hands are furthest away from the legs.
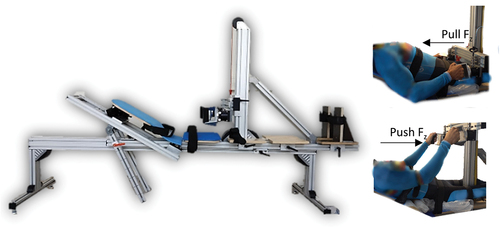
During the test, the participants were lying in a recumbent position, with the backrest set at 30° with the horizontal axis and a neck support provided so the participants could rest their head between trials. The lower limbs were placed in knee extension on top of wooden plates, positioned under the thighs and calves. Velcro straps were used at the thigh, pelvis and chest level, and for participants with hand impairment, a gripping aid glove (General Purpose Gripping Aid, The Active Hands Company Limited, Solihull, England) was used and attached to the handlebar. During the CC condition, two independent footrests were attached to the feet (or to the stump of the residual limb at the thigh level for an above-knee amputation and at the shank level for a below-knee amputation), providing vertical (horizontal heel plate) and horizontal (vertical plantar plate) support. During the OC condition, both footrests were removed, leaving only the horizontal heel plate support ( Top).
Figure 3. Top: examples of closed chain (left) and open chain (right) with the footrest of the isometric strength setup. Bottom: examples of the pelvic closed chain (left) and no pelvic closed chain (right) in the isometric strength setup.
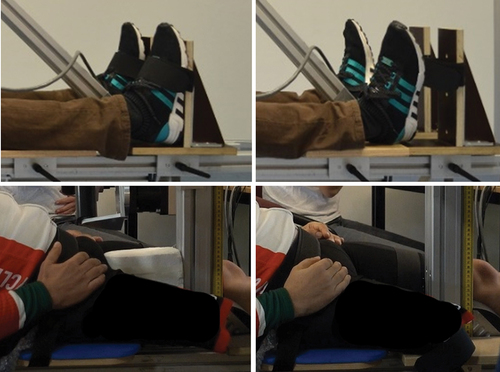
The test consisted of standardised isometric push and pull strength measurements under two kinetic chain conditions. Prior to the measurements, a 30-s calibration trial was conducted. The first trial was considered a familiarisation trial, followed by a set of 2 × 2 (push vs. pull; OC vs. CC) maximal voluntary contractions (MVC). Each isometric test comprised a 2- to 3-s build-up submaximal contraction followed by a 3-s MVC. A computer-based data acquisition control system (SCXI 1000, National Instruments, Texas, USA) collected force data signals at 200 Hz in three dimensions (horizontal (Z)—forward/backward (); transversal (X)—inward/outward; vertical (Y)—upward/downward).
Resembling the pull and push vertical positions on a handbike, strength tests were conducted at two different heights. To assess pull strength, the handlebars were placed at a height of 0.13 m from the seat (i.e., the lowest position possible without the cranks touching the participant’s body), and the seat was moved towards the rigid beam to the closest position possible, aiming to mimic handcycling-related elbow angles (see , upper right picture). A demonstration was provided, and the participant was instructed to pull backward with both arms simultaneously. To assess push strength, a similar procedure was conducted with the exception of the handlebars being placed at a height of 0.52 m from the seat, and the participant being instructed to push forward with both arms (see , lower right picture). Order of pull and push conditions were randomised among participants. The arm length and the elbow angle during the pulling and pushing conditions were measured for sample description.
A third kinetic chain condition was added later and, therefore, only assessed at the 2019 Para-cycling Road World Championship in Emmen, leading to a subsample of 36 athletes. In this condition, hereafter referred to as pelvic-closed-chain (PlvCC) condition, the footrests were removed, but additional stabilisation was provided to the pelvis. The additional stabilisation was achieved using a styrofoam block (different sizes available) that better filled the empty space between the pelvis, the inner thighs and the rigid frame ( Bottom). Pushing was not tested in this additional condition.
Data analyses
Manual muscle test
Participants were grouped based on the lower-limbs MMT scores. The group Lower-Limb Function (LLF) included athletes with partial to normal lower-limb strength in at least one of the limbs (MMT scores 4–5 presented at hip flexion, hip extension, and knee extension). The group No Lower-Limb Function (NO-LLF) included athletes who presented no or highly impaired strength in both legs (MMT scores 0–3 for hip flexion, hip extension and knee extension).
The MMT scores for elbow extension and elbow flexion ranged from 0 to 5. Participants who scored ≤3 in one of these muscle actions were considered to have impaired upper limbs.
Isometric ‘handcycle-based’ ratio-scale strength assessment
Data were processed using Matlab Software (Version R 2019b, MathWorks, Natick, Massachusetts, USA) and filtered with a 4th order Butterworth prior to the calculation of the outcome variables. For each trial, a moving average function was used to obtain the 2-s with the maximal average force in the horizontal direction (Fz) () from the dominant upper limb (as indicated by the athlete).
Prior to data analyses, a visual inspection of the force signals was conducted to control for deviations from the expected pattern, as conducted in a previous study (R. Muchaxo et al., Citation2022). It was expected that the participant would maintain an MVC period with a plateau effect; however, given the unknown influence of the physical impairment on isometric strength, pattern deviations could be a representation of the impact of impairment. Following this rationale, trials were selected for analysis when a) trials showed a typical MVC plateau ( left); b) a plateau was not shown but both trials from the same condition presented a similar pattern ( right). When a participant showed, for the same condition, a deviation from the typical plotting in one trial ( right) and a plateau effect in the other trial ( left), only the trial with the typical plateau effect was selected. Following this visual inspection, each participant’s outcome from the same condition was averaged and used as such in the statistical analyses.
Statistical analyses
Analyses were conducted using IBM SPSS Statistics (Version 25). Data normality was assessed using the Shapiro Wilk test and homogeneity of variance with a Levene’s test. A Mauchly’s test was conducted to assess sphericity in the subsample with the three different conditions. A repeated-measures ANOVA was conducted to investigate whether there was an interaction effect between the within-subjects effect type of chain (CC vs. OC) and the between-subjects effect level of lower-limb function (LLF vs NO-LLF) on the pushing and pulling strength. The main effect of the type of kinetic chain (CC vs. OC) on the upper-limb force was examined as well. When the interaction effect was statistically significant, simple effects within each group separately were analysed to describe the effect of the kinetic chain at the different levels of lower-limb function. In the subsample with the three different conditions, simple contrasts were examined with PlvCC condition as reference (i.e., PlvCC vs. CC; PlvCC vs. OC).
In addition, a sensitivity analysis was conducted by removing athletes that presented additional characteristics that could influence the interpretation of the kinetic chain results, e.g., athletes with impaired upper limbs or athletes with pain in lower-limb joints besides normal strength in lower-limb muscles. Post-hoc Bonferroni tests were conducted to correct for multiple comparisons, and the alpha level was set a 0.05 for all tests.
Results
From the initial sample (n = 62), all participants performed the pulling task and 59 performed the pushing task (three participants were not willing to perform pushing tasks prior competition event due to pain or personal motivation). The groups based on the lower-limb MMT assessment had different sample sizes, with the group with the athletes presenting the greatest impairments being considerably larger (pull: LLF n = 21, NO-LLF n = 41; push: LLF n = 20, NO-LLF n = 39). Group characteristics are presented in . From the individual visual inspection, 8 of the 62 athletes presented atypical, i.e., no plateau effect during the MVC, but consistent force patterns during strength trials. Six athletes presented irregularities in only one of the strength trials, leading to the exclusion of these specific strength trials from further analysis. In the sensitivity analysis (n = 48; 13 women (21%)), six athletes from the LLF and eight athletes from the NO-LLF were removed.
Table 1. Demographic characteristics of the sample and average pulling and pushing forces for each condition and group.
Effect of a CC condition on pull force
There was a significant main effect of the type of kinetic chain on pull force with participants achieving higher forces in the CC condition compared to the OC condition (F(1,60) = 17.59, p < 0.001, η2 = 0.23). A significant interaction effect was observed between the type of kinetic chain and lower-limb function group, F(1,60) = 8.52, p = 0.005, η2 = 0.12 (), indicating that only in the LLF group higher (+11%) pull forces were achieved in the CC compared to the OC condition (F(1,60) = 19.12, p < 0.001, η2 = 0.24) (, Top Left). The results from the sensitivity analysis (LLF n = 15; NO-LLF n = 33) were comparable to the main analyses (main effect (F(1,46) = 24.19, p < 0.001, η2 = 0.35), interaction effect (F(1,46) = 11.88, p = 0.001, η2 = 0.21)).
Figure 5. Graphs showing average upper-limb forces of the LLF group, NO-LLF group, and total group, during closed and open chain conditions. The error bars represent the standard deviations. LLF partial to good lower-limb function; NO-LLF no lower-limb function. *=significant interaction effect.
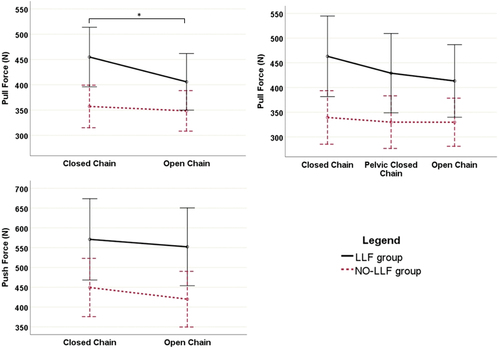
Table 2. Main effects and interaction effects of the type of kinetic chain and level of lower-limb function on pull and push forces.
Effect of a PlvCC condition on pull force
The subsample performing the PlvCC condition consisted of 36 athletes (14% women; LLF n = 11, NO-LLF n = 25). The main effect of the type of kinetic chain on pull force was significant (F(1,68) = 6.87, p = 0.002, η2 = 0.17), revealing a significant effect of the kinetic chain on the pull force between CC and PlvCC (F(1,34) = 5.69, p = 0.02, η2 = 0.14), with higher (+6%) pull forces in the CC condition. Yet, the kinetic chain effect between OC and PlvCC was not significant (p = 0.27; 5% higher forces in PlvCC). No significant interaction effect was observed between the type of kinetic chain and the level of lower-limb function, F(2,68) = 2.97, p = 0.058, η2 = 0.08 () (, Top Right). The results from the sensitivity analysis (LLF n = 9; NO-LLF n = 19) were comparable to the main analysis (main effect (F(2,52) = 6.17, p = 0.04, η2 = 0.19) and no interaction effect (p = 0.13)). However, the differences between the conditions were opposite to what was found in the N = 36 sample, i.e., no significant effect between CC and PlvCC (p = 0.18; 3% higher in CC), but a significantly higher (+4%) pull force in PlvCC compared to OC (F(1,26) = 5.86, p = 0.02, η2 = 0.18).
Table 3. Main effects and interaction effects of the type of kinetic chain and the level of lower-limb function on pull force in the pelvic-closed-chain subsample.
Effect of a CC condition on push force
Regarding push force (), participants achieved higher (+5%) forces in the CC condition than in the OC condition (F(1,57) = 13.37, p = 0.001, η2 = 0.19), which was similar for the 2 groups (no significant interaction effect) (, Bottom Left). The results from the sensitivity analysis (LLF n = 14; NO-LLF n = 32) were comparable (main effect (F(1,44) = 11.98, p = 0.001, η2 = 0.21), and no interaction effect (p = 0.57)).
Discussion and implications
This study is the first study to investigate the difference in upper-limb force production between CC and OC conditions in a large group of handcycling athletes with a different lower-limb function. As hypothesised, our findings suggest that pull strength is significantly higher in handcyclists when they can actively form a closed chain using their legs. This is in line with the findings of Kouwijzer et al. in able-bodied participants, who found higher power output values during a handcycling sprint test when athletes pushed against the footrest (i.e., closed chain) (Kouwijzer et al., Citation2018). However, in our strength measurements, the advantage provided by the CC condition was not observed in the NO-LLF athletes, i.e., athletes who cannot form an active CC condition do not benefit in an isometric strength test. These results suggest that the interaction between the level of lower-limb function and the athlete-handbike interface (allowing or not allowing a CC) plays a role in maximum upper-limb pull strength.
Stabilising the pelvic region during the pull strength measurement, to prevent sliding of the body in the isometric strength setup, appears to partly compensate for the removal of the footrests during a lower-limb OC condition in the LLF group. The results indicate that the inclusion of a PlvCC condition reduces the interaction effect between the level of lower-limb function and the type of kinetic chain, suggesting that the pelvis is an important part of the chain. However, it is seen in (Top Right) that the condition led to the highest average pull forces in the LLF group and that average pull force was lower when using the PlvCC condition and was the lowest in the OC condition. This change in average pull force between kinetic chain conditions was less evident in the NO-LLF group (, Top Right).
As the PlvCC condition was only performed by a smaller subsample of participants, results need to be interpreted with caution. It could be expected that, even under an optimal PlvCC condition, the addition of a footrest to push against still provides greater advantage for maximal pull strength in athletes with LLF, compared with not being able to push against it. This assumption is in line with previous findings in able-bodied individuals that suggested that combined back and hand stabilisation leads to greater knee torque production than back stabilisation alone (Magnusson et al., Citation1993). Achieving maximum power production requires an intact stable kinetic chain function (Van Der Hoeven & Kibler, Citation2006). Yet, those studies’ findings are limited to the inclusion of able-bodied participants, thus, future studies should deepen the understanding of the different characteristics of athlete-handbike interface in athletes with diverse physical impairments by investigating the impact that optimisation of adapted equipment, such as additional straps and other stabilisation aids, may have on handcycling performance.
During the push strength measurements, the athletes’ trunk is pushed against the backrest (producing a reaction force vertically as well as horizontally, i.e., against gravity and material friction). It is likely that the fixed backrest forms a closed chain that minimises a potential impact of the lower-limb function. In addition, pushing against the footrests with the lower limbs seems counter-intuitive when aiming to push maximally against the handlebars with the upper limbs. It is plausible that the increased push force production observed in both groups during CC is a result of a greater stabilisation of the athlete’s lower extremities with the Velcro strap (). Although the isometric strength setup allowed for individual adjustments, it can be speculated that the personalised backrest of the athlete’s handbike would fixate the athlete’s body better than our standard backrest. Hence, while pushing the handbike cranks, the effect of the footrest may be reduced or minimal.
The pull strength was shown to be one of the strongest isometric strength characteristics to determine handcycling performance (Muchaxo et al., Citation2022). Its impact was particularly significant during the more standardised maximal-effort handcycling sprint performance, in comparison with a time-trial performance at submaximal (albeit high) velocities, which involves a greater contribution of the aerobic energy system. It could be that the advantage gained by athletes with lower-limb function when pushing against footrests would be greater during specific race moments that require (near) maximal effort, such as a sprint, as observed in the study of Kouwijzer et al. with able-bodied participants (Kouwijzer et al., Citation2018). Future studies should contribute to a better understanding of the athlete-handbike interface by assessing performance indicators in handcycling athletes racing with different kinetic chain conditions.
According to the International Paralympic Committee Athlete Classification Code, the use of equipment must be considered during sport class allocation, and regulated by the governing sports federation (International Paralympic Committee, Citation2015). In the para-cycling regulations, the use of footrests is regulated in terms of safety (Union Cycliste Internationale, Citation2021). However, the ability to form an active CC is not considered. As described previously, the H4 sport class may include athletes with lower-limb function varying from no function in both lower limbs to normal function in the non-impaired limb and in the residual limb of an athlete with a single amputation. The findings suggest that when the athletes with the least impairments in this class, i.e., with partial to full lower-limb function, are permitted to push against the footrest, they can maximise their performance and will have an advantage compared with their opponents who do not have lower-limb function but compete in the same sport class. When a closed chain cannot be made, the difference between groups is smaller, as the advantage of the athletes with the least impairments.
In a recent study, it was suggested that trunk flexion strength (a main determinant in sport allocation between H3 and H4) may play a minor role during recumbent handcycling performance in athletes without upper-limb impairments (R. Muchaxo et al., Citation2021). In addition, the difference in handcycling performance between the two classes has been found to be small (R. E. A. Muchaxo et al., Citation2020; Muchaxo et al., Citation2021). It could be hypothesised that the athletes with the greatest impairments from H4 (i.e., no lower-limb function and almost normal trunk flexion strength) present a more similar activity limitation to the athletes with the least impairments from H3 than with the respective H4 athletes with partial to good lower-limb function. We do not have sufficient evidence to recommend whether lower-limb function should have a main role in sport class allocation, as we have not studied the impact of kinetic chains during realistic handcycling performances (e.g., a time-trial race). However, the findings of Kouwijzer et al. (Citation2018) together with the current study point out that athletes with lower-limb function varying from partial to full are able to maximise their recumbent handcycling performance by pushing against the footrests forming closed kinetic chains, and therefore, leading to potential unfair advantages of the least impaired athletes when competing against athletes with no lower-limb function.
Limitations
A study limitation is the difference in sample size between the two lower-limb groups. As the group with partial to good lower-limb function was substantially smaller we could not make comparisons between partial and good lower-limb function or between function in one leg versus in both legs. However, the sensitivity analysis, excluding participants with additional health conditions, showed similar results and thereby indicates robustness of the main results. To better understand the impact of a lower-limb OC with a PlvCC condition, a subsample was tested; however, a larger sample is preferred to confirm the presented results.
To answer our research questions, an innovative customised ‘handcycle-based’ isometric strength setup was built. We have followed a standardised testing protocol and analyses, and the strength setup allowed adjustments. However, individualising to match muscle force-length relationship across participants with different anthropometric characteristics was not optimal. In addition, although the proposed strength setup mimics a recumbent handcycling position, variations exist in comparison with actual handbikes. For example, it is expected that the increment observed in push strength during a CC (i.e., feet strapped to the footrest) is compensated in the athlete’s handbike by a customised backrest. Further research efforts in customising a strength setup to an optimal athlete-device interface are needed to understand if an athlete takes advantage of other interface adjustments beyond the footrest CC during pull and push, as well as during other segments of the propulsion cycle, such as push-up and pull down.
Conclusion
This is the first study addressing the potential impact of pushing with the lower-limbs against footrests on upper-limb isometric strength in handcycling athletes with different levels of lower-limb function. The significant advantage of using a CC was observed in athletes with partial to good lower-limb function, but not in those without. As such, these findings are particularly important for the athletes allocated to the handcycling sport class H4, where athletes with the least impairments can exploit the advantage provided by pushing off against the footrests, but athletes with no lower-limb function do not seem to benefit from the use of the footrest in an equal manner. Theoretically, this implies further benefits for optimisation of performance, especially during maximal effort like in a sprint, of athletes with some degree of lower-limb function. However, the ability to perform a CC is not considered in the current handcycling classification system nor in the handcycling technology paragraph of the UCI. To guarantee fair competition, the current classification system should therefore consider the implication of these results on the classification rules.
Acknowledgments
We would like to thank the technical department of the Department of Human Movement Sciences, VUA, for their contribution to the development of the isometric strength setup.
Disclosure statement
No potential conflict of interest was reported by the author(s).
Additional information
Funding
References
- Hislop, H., & Montgomery, J. (2007). Daniels and Worthingham’s muscle testing: Techniques of manual examination (8th ed.). Saunders Elsevier.
- International Paralympic Committee. (2015). IPC athlete classification code. International Paralympic Committe.
- Karandikar, N., & Vargas, O. O. O. (2011). Kinetic chains: A review of the concept and its clinical applications. PM and R, 3(8), 739–745. https://doi.org/10.1016/j.pmrj.2011.02.021
- Kouwijzer, I., Nooijen, C., Van Breukelen, K., Janssen, T., & De Groot, S. (2018). Effects of push-off ability and handcycle type on handcycling performance in able-bodied participants. Journal of Rehabilitation Medicine, 50(6), 563–568. https://doi.org/10.2340/16501977-2343
- Magnusson, S. P., Geismar, R. A., Gleim, G. W., & Nicholas, J. A. (1993). The effect of stabilization on isokinetic knee extension and flexion torque production. Journal of Athletic Training, 28(3), 221–225.
- McKay, A., Stellingwerff, T., Smith, E., Martin, D., Mujika, I., Goosey-Tolfrey, V., Sheppard, J., & Burke, L. M. (2022). Defining training and performance caliber: A participant classification framework. International Journal of Sports Physiology & Performance [Internet]. 17(2), 317–331. https://doi.org/10.1123/ijspp.2021-0451
- Muchaxo, R., de Groot, S., Kouwijzer, I., van der Woude, L. H. V., Janssen, T. W. J., & Nooijen, C. F. J. (2022). Association between upper-limb isometric force and handcycling performance in elite athletes. Sports Biomechanics, 1–20. https://doi.org/10.1080/14763141.2022.2071760
- Muchaxo, R., De, G. S., Kouwijzer, I., Van Der, W. L., Janssen, T., & Nooijen, C. F. J. (2021). A role for trunk function in elite recumbent handcycling performance? A role for trunk function in elite recumbent handcycling performance? Journal of Sports Sciences, 39(20), 2312–2321. https://doi.org/10.1080/02640414.2021.1930684
- Muchaxo, R. E. A., De, G. S., Van Der Woude, L. H. V., Janssen, T. W. J., & Nooijen, C. (2020). Do handcycling time-trial velocities achieved by para-cycling athletes vary across handcycling classes? Adapted Physical Activity Quarterly, 37(4), 461–480. https://doi.org/10.1123/apaq.2019-0143
- Union Cycliste Internationale. (2021). Part 16 para-cycling. In UCI cycling regulations (pp. 1–96).
- Van Der Hoeven, H., & Kibler, W. B. (2006). Shoulder injuries in tennis players. British Journal of Sports Medicine, 40(5), 435–440. https://doi.org/10.1136/bjsm.2005.023218