ABSTRACT
Nanosecond pulsed electric fields, also known as Nano-Pulse Stimulation or NPS, can trigger regulated cell death to clear skin lesions that are cellular in nature. Before treating facial lesions, it is important to demonstrate the effects of these pulses on normal facial skin. Here we have applied a range of NPS energies to the epidermis and dermis of normal facial skin scheduled for excision to establish a safe dose range of energies prior to use in clinical applications. This was an open-label, non-randomized study under the direction of a single Principal Investigator. The time course of the treated tissue changes was determined by histological analysis. All energy settings generated a delayed epidermal loss followed by re-epithelialization by day 7 and a normal course of healing. One day after NPS treatment, the cellular membranes of the treated epidermis were intact, but their nuclei no longer stained with H&E, resulting in a hollow appearance that has been referred to as “ghost cells.” Cellular structures in the dermis, such as sebaceous glands and melanocytes, exhibited regulated cell death observed by 1 day post treatment. Melanocytes recovered to their normal density within 7 days. The 60-day samples indicated that epidermis, hair follicles, and eccrine glands appeared normal. The selective effect of NPS treatment on cellular structures in the epidermal and dermal layers suggests that this non-thermal modality of energy delivery is ideal for treating cellular targets including benign and malignant skin lesions. NPS skin treatments provide a promising method for clearing skin lesions with a cellular basis.
Background
NPS treatments have been applied to many different biological systems over the past two decades (Citation1,Citation2,Citation3). These pulses are so short that they have the unique ability to penetrate into cells and organelles before charge rearrangements can respond to neutralize the imposed field. This electric field penetration can “stimulate” many different cellular responses as varied as secretion (Citation4,Citation5), differentiation (Citation6), and regulated cell death (Citation7), depending on the amount of energy applied and cell type treated. The form of NPS applied in this study was tuned to deliver a non-thermal signal to induce regulated cell death (RCD) within a localized treatment zone determined by the placement of treatment tips with two rows of bipolar microneedles (). The pulse generator used was the PulseTx manufactured by Pulse Biosciences. The cell-specific NPS mechanism avoids damage to acellular tissue components mainly because it is non-thermal due to the low energy and ultra-short pulses used (Citation8,Citation9). A previous study treating pre-abdominoplasty abdominal skin confirmed this lack of damage to the acellular dermis for most NPS energy levels (Citation10). This is in contrast with other common treatments, such as laser resurfacing, electrodesiccation and cryotherapy, whose primary mechanism of action is thermal necrosis (Citation11) with skin effects visible almost immediately. Rather than heating the skin, NPS therapy generates nanopores in intracellular organelles to stimulate RCD. This results in a signaling cascade leading to apoptotic cell death in which the treated epidermis slowly dies over a day and within a week is replaced by a regenerated epidermis without any changes in the underlying dermal collagen fibers. Extensive pre-clinical work on animal models has demonstrated that this results in a favorable, scarless healing profile as well as a broad systemic and sustained antitumor immune response to malignant lesions (Citation12–19). Thus, NPS therapy has a potential dual effect in malignant cells of eliminating tumor cells via a RCD mechanism, followed by a systemic immune response triggered by the immune system’s detection of antigens that are released during RCD. Extensive preclinical research by several investigators has revealed that the RCD signaling cascade initiated by NPS therapy includes increases in intracellular calcium (Citation20,Citation21) reactive oxygen species (Citation22,Citation23), caspase-3 activation (Citation13,Citation14,Citation15,Citation16,Citation17,Citation18), and the initiation of DNA fragmentation (Citation24).
Methods
Study design
This study of NPS effects on preauricular facial skin was designed as an open-label, non-randomized study under the direction of a single Principal Investigator, James Newman, under IRB oversight (Biomedical Research Institute of America, protocol NP-PT-003) with clinical monitoring and data analysis by Pamela Buckman. Candidates who had previously decided to undergo an elective facelift procedure were extended an invitation to participate in this study. The study was designed to meet two objectives: (1) to establish a range of NPS exposure that could be delivered safely to facial tissue with predictable damage to the skin with associated predictable recovery, and (2) to perform a histological analysis of the NPS-treated tissue to correlate clinical recovery scores and images with histology scores and observations for the respective energy levels tested.
The degree of effect of the various energy treatment levels was assessed by evaluating the clinical appearance and histologic response of each treatment region. The time course of the wound response and the degree of injury were evaluated through histologic examination and scored evaluation of samples collected on the day of surgery representing 1, 7,15, 25, 30, and 60 days post-NPS treatment.
Subjects
There were two cohorts of three subjects each. The first group had 3 regions mapped on each side of the face and two regions were each treated with one of eight different energy levels at each pre-surgery visit using either a 2.5 × 2.5 mm applicator or a 5 × 5 mm applicator (). The second cohort had 11 regions mapped and a third applicator was added (1.5x1.5 mm) () along with earlier time points.
Treatment
Six energy levels were evaluated in the initial cohort of three patients enrolled in the 60-day longitudinal study. Treatment areas were demarcated on the skin on each treatment day using a plastic overlay and medical grade ink. Prior to treatments, 2% lidocaine injections were applied to facilitate patient comfort during energy delivery. The treatment levels (TL) represented different amounts of NPS energy, where the number of pulses, applied voltage, pulse duration, and tip area combined to produce a particular TL. The total electric energy applied to each treatment region ranged between 2 and 5 J.
Histologic sample preparation
The clinical subject’s skin was marked with gentian violet marker confirming previously treated NPS sites with the aid of the plastic templates immediately prior to beginning their planned surgery. The patients were then prepared for surgery with intravenous sedation in an accredited outpatient surgical facility. After sterile prepping and draping the patients’ face and neck, local anesthetic of 2% lidocaine with epinephrine (1:100,000) was injected along marked incision lines along with field infiltration of dilute anesthetic subcutaneously for facelift surgery. A punch biopsy ranging from 4 to 6 mm was then used to completely excise the treatment areas through the subcutaneous fat with an orientation suture when possible noting the anterior superomedial edge of each treatment zone. There was minimal bleeding encountered during the biopsy process and no electrocautery was used during the harvesting of the specimens. Once all specimens were harvested, the patients underwent their planned surgical facelift procedure under intravenous sedation. The individual samples collected were placed in collection jars with 10% neutral formalin for preservation and shipped to a histopathology lab, Pinkus Dermatopathology Laboratory, for staining and evaluation by a dermatopathologist.
Samples were bisected perpendicular to the epidermal surface to create full-thickness sections and were mounted to expose the epidermal and dermal cross-section. Hematoxylin and Eosin (H&E) staining preparations were made of all time points. Preparations were also made for selected time points using elastic Orcein Giemsa, microphthalmia transcription factor (MITF), and Fontana Mason. Samples prepared with these stains were examined by a board-certified dermatopathologist (DM) and graded using methods designed to evaluate dermal inflammation, elastic fiber integrity, adnexal structure effects, and melanocyte density. The assessment of melanocytes was performed by counting melanocytes identifiable in three randomly selected, 1-mm2 treatment zones of the sample and taking the average of the three.
Results
Clinical experience
All treated areas healed without exudate or significant discomfort to the patient during the clinical evaluation period. No signs of infection were present, and no adverse events occurred. A representative photographic montage is shown in . Localized epidermal damage and erythema is apparent post-treatment for all treatment levels in all subjects. Formation of eschar is evident 5 days post-treatment. Sites treated at higher TLs appeared more affected. Epidermal recovery was evident by 7 days and complete by 15 days. The skin harvesting at the date of surgery added 20 minutes to the total operating time and there was no impact on all of the subjects’ healing after their procedures were complete. There were no adverse events or alterations in patient healing during the regular post-operative clinic visits from their respective surgical procedures.
Figure 2. Long-term images of facial skin treated with NPS energy. (a) Fitzpatrick type 1 skin treated with 150 mJ/mm3; (b) Fitzpatrick type 2 skin treated with 150 mJ/mm3. (c) Fitzpatrick type 1 skin treated with 300 mJ/mm3; (d) Fitzpatrick type 2 skin treated with 300 mJ/mm3. The scale bars in each image are 2 mm long and the day after treatment on which each photo was taken is indicated in the upper left of each photo
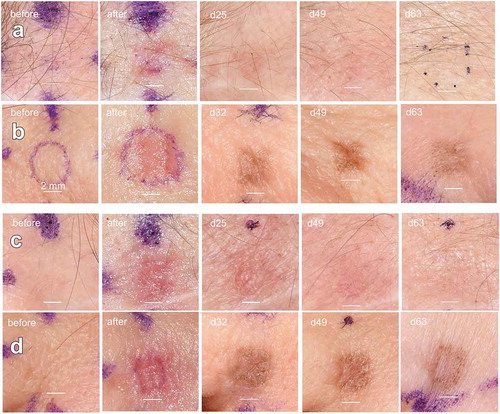
Histological findings
Epidermal changes
One day after NPS treatment, the nuclei of the treated epidermis no longer stained blue in H&E. The cellular membranes were intact, but the cell interiors had a hollow appearance that is sometimes referred to as “ghost cells” (). Seven days after NPS treatment, the treated epidermis had formed an eschar () and a regenerated epidermis was present. The samples at day 15 timepoints showed full re-epithelialization of the epidermis. The rete ridge patterns which often show flattening with chronic photoaging, also showed patterns similar to control specimens. Evidence of epidermal inflammation such as spongiosis or exocytosis of lymphocytes was not observed. The samples at the 30-day timepoints showed similar changes to the 15-day samples.
Figure 3. H&E histology of the changes in facial skin following NPS treatment using an energy setting of 500 mJ/mm3 with a 1.5 × 1.5 mm applicator. (a) Untreated facial skin biopsy from patient 5; (b) biopsy of facial skin from patient 5 one day after NPS treatment. Ghost cells lacking nuclear stain are present in the epidermis; (c) biopsy of facial skin from patient 5 one week after NPS treatment. Note the formation of an eschar (arrow) resulting from the treated epidermis in “(b)” (ellipse) and the newly regenerated, thinner epidermis that has formed on the dermis; (d) biopsy of facial skin from patient 5 thirty days after NPS treatment; (e) control facial skin sample from patient 5; (f) skin biopsy from patient 5 taken 1 day post NPS treatment. Arrows point to sebaceous glands undergoing regulated cell death. SK indicates a necrotic seborrheic keratosis that was present on treated skin
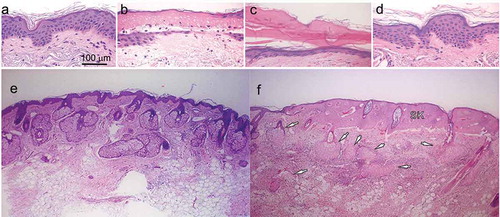
Changes in the dermis
Sebaceous glands and melanocytes exhibited the greatest changes. Within 1 day they were observed to undergo regulated cell death and form ghost cells (). Sebaceous glands did not recover during the 60 days in this study, but melanocytes began to recover within 7 days and returned to normal by 30 days (). The number of melanocytes was observed using a MITF immunostain and averaging the number found in 3 different areas of 1 mm2. Staining for melanocytes shows the number of melanocytes return to normal density, comparable to the control specimens, for most energy levels tested. The two highest energy levels show a slightly lower count of melanocytes compared to controls, which may be indicative of a slower recovery of melanocytes. The rapid return of the melanocytic density to levels comparable to control should be consistent with a relative normalization of skin pigmentation over time.
Figure 4. Mean melanocyte density determined in preauricular tissue samples treated with a 2.5 × 2.5 mm tip, applying indicated energies and scored at indicated sample time. Bars indicate melanocyte density and gray line indicates the energy density that was applied. Total applied energy is indicated below each bar
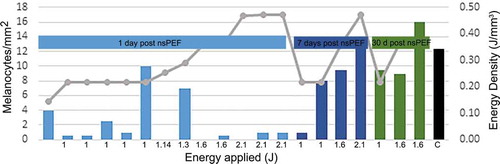
Hair follicles and eccrine ducts
In specimens in which hair follicles and eccrine ducts were visible, there was no apparent effect to the adnexal structures. This is consistent with previous histologic analysis of tissue exposed to this technology, which showed partial healing seen at 5 days and complete recovery by 15 days. Eccrine ducts often showed focal squamous metaplasia, a sign of re-epithelialization. The epidermal layer had returned to normal in all cases with a normal thickness and preservation of rete ridge pattern similar to controls. The samples at the 60-day timepoint showed the epidermis, hair follicles, and eccrine glands had all completely returned to normal.
Dermal collagen
Alterations in the dermal collagen observed were minimal, with no evidence of thermal injury. In some tissue samples exposed to 300 mJ/mm3 there was a mild effect on the elastin fibers, as there was evidence of a loss of elastic fibers in the papillary dermis. In one of these patients, there was also some flattening of the dermo-epidermal junction which improved by day 60.
Elastic tissue staining
Elastic tissue remained intact in all the patients. In tissue samples exposed to 300 mJ/mm3 there was occasional slight decrease in elastic fibers. Minimal effects were noted on the dermis. This predicts a very low risk of scarring.
Inflammation
Overall, the degree of dermal inflammation was minimal compared to other thermal or physical methods of intentionally damaging surface epidermal tissue. There was a small amount of inflammation seen at day 30 for some tissue exposed to the highest treatment levels; however, the amount of inflammation appears to be sparse, primarily perivascular and focally perifollicular. The samples examined showed no evidence of fibrin deposition in the blood vessels to suggest vascular injury.
Presence of macrophages
Macrophages containing melanin were sparse but present in the papillary dermis. These were present at all treatment levels but are few in numbers.
Fat
There was no effect on the subcutaneous fat at any of the timepoints evaluated.
Hyperpigmenation
Presence of macrophages containing melanin correlates with hyperpigmentation. In the first cohort of patients, 6 of the 12 treatment sites exhibited hyperpigmentation correlating to subjects with higher Fitzpatrick skin classification III. No hyperpigmentation was noted for Fitzpatrick class I skin type. In the second cohort, hyperpigmentation was observed in 30% of the treated sites and was associated with Fitzpatrick score of IV.
Discussion
This study represents the first controlled clinical trial to evaluate the effects of NPS treatment of facial skin. This novel method of using low energy NPS treatment at nine different energy settings was observed to lead to a predictable recovery of the epidermal layer of facial skin at all energy levels at all timepoints observed. Lesions residing in the epidermis became part of the eschar when the renewed epidermal layer appeared. Cellular structures in the dermis underwent regulated cell death and exhibited variable amounts of regeneration. The melanocytes began to reappear at 7 days after NPS treatment, but the sebaceous glands did not regenerate during the 60 days studied.
The lack of observed effect on dermal collagen and relatively low level of inflammation is consistent with the non-thermal, nature of these ultra-short pulses of energy. This lack of inflammatory effect is consistent with the preservation of fibroblasts, elastic tissue, and melanocyte recovery.
Hyperpigmentation
Patients with Fitzpatrick skin types II, III, and IV exhibited hyperpigmentation in the treated skin tissue. Ongoing studies using lower energy levels suggest that hyperpigmentation is reduced at lower levels. Another approach to reduce hyperpigmentation would be the use of hydroquinone in darker pigmented subjects post-procedure as is commonly used to reduce post-inflammatory hyperpigmentation caused by other therapies.
Conclusion
These results demonstrate that the NPS treatment of facial skin is safe and effective for removing lesions residing in the epidermis. In addition, all cellular structures in the epidermis and dermis can be eliminated without damaging the non-cellular fibrous components of the dermis. Many of the cellular structures such as the melanocytes and hair follicles regenerate within a week or so while the sebaceous glands do not regenerate. The treatment time is very short and highly localized to the skin area within the applicator tip.
Acknowledgments
This study was funded by Pulse Biosciences Inc. LJ, WK, EE, DU, RN are employed by Pulse Biosciences. JN and DM were compensated for their time required to conduct the procedures described here or to analyze the histological data generated by these procedures.
Disclosure statement
All authors have completed and submitted the ICMJE Form for Disclosure of Potential Conflicts of Interest. LJ, EE, WAK, DU and RN are employed by Pulse Biosciences Inc., the company that fabricated the pulse generator used in this study. None of the other authors reported conflicts.
Additional information
Funding
References
- Schoenbach KH. From the basic science of biological effects of ultrashort electrical pulses to medical therapies. Bioelectromagnetics. 2018;39(4):257–76. doi:10.1002/bem.22117.
- Batista NT, Rebersek M, Vernier PT, Mali B, Miklavcic D. Effects of high voltage nanosecond electric pulses on eukaryotic cells (in vitro): a systematic review. Bioelectrochemistry. 2016;110:1–12. doi:10.1016/j.bioelechem.2016.02.011.
- Beebe SJ, Sain NM, Ren W.Induction of cell death mechanisms and apoptosis by nanosecond pulsed electric fields (nsPEFs). Cells. 2013;2(1):136–62.
- Zhang J, Blackmore PF, Hargrave BY, Xiao S, Beebe SJ, Schoenbach KH. Nanosecond pulse electric field (nanopulse): a novel non-ligand agonist for platelet activation. Arch Biochem Biophys. 2008;471(2):240–48. doi:10.1016/j.abb.2007.12.009.
- Hargrave B, Li F.Nanosecond pulse electric field activation of platelet-rich plasma reduces myocardial infarct size and improves left ventricular mechanical function in the rabbit heart. J Extra Corpor Technol. 2012;44(4):198–204.
- Bai F, Gusbeth C, Frey W, Nick P. Nanosecond pulsed electric fields trigger cell differentiation in chlamydomonas reinhardtii. Biochim Biophys Acta Biomembr. 2017;1859(5):651–61. doi:10.1016/j.bbamem.2017.01.007.
- Nuccitelli R, McDaniel A, Anand S, Cha J, Mallon Z, Berridge JC, Uecker D. Nano-pulse stimulation is a physical modality that can trigger immunogenic tumor cell death. J Immunother Cancer. 2017;5:32. doi:10.1186/s40425-017-0234-5.
- Nuccitelli R, Pliquett U, Chen X, Ford W, James SR, Beebe SJ, Kolb JF, Schoenbach KH. Nanosecond pulsed electric fields cause melanomas to self-destruct. Biochem Biophys Res Commun. 2006;343(2):351–60. doi:10.1016/j.bbrc.2006.02.181.
- Pliquett U, Nuccitelli R. Measurement and simulation of Joule heating during treatment of B-16 melanoma tumors in mice with nanosecond pulsed electric fields. Bioelectrochemistry. 2014;100:62–68. doi:10.1016/j.bioelechem.2014.03.001.
- Kaufman D, Martinez M, Jauregui L, Ebbers E, Nuccitelli R, Knape WA, Uecker D, Mehregan D. A dose-response study of a novel method of selective tissue modification of cellular structures in the skin with nanosecond pulsed electric fields. Lasers Surg Med. 2020;52:315–22. doi:10.1002/lsm.23145.
- Orringer JS, Rittie L, Baker D, Voorhees JJ, Fisher G. Molecular mechanisms of nonablative fractionated laser resurfacing. Br J Dermatol. 2010;163(4):757–68. doi:10.1111/j.1365-2133.2010.09998.x.
- Nuccitelli R, Tran K, Lui K, Huynh J, Athos B, Kreis M, Nuccitelli P, De Fabo EC. Non-thermal nanoelectroablation of UV-induced murine melanomas stimulates an immune response. Pigment Cell Melanoma Res. 2012;25:618–29. doi:10.1111/j.1755-148X.2012.01027.x.
- Chen R, Sain NM, Harlow KT, Chen YJ, Shires PK, Heller R, Beebe SJ. A protective effect after clearance of orthotopic rat hepatocellular carcinoma by nanosecond pulsed electric fields. Eur J Cancer. 2014;50(15):2705–13. doi:10.1016/j.ejca.2014.07.006.
- Nuccitelli R, Berridge JC, Mallon Z, Kreis M, Athos B, Nuccitelli P.Nanoelectroablation of murine tumors triggers a CD8-dependent inhibition of secondary tumor growth. PLoS One. 2015;10(7):e0134364.
- Skeate JG, Da Silva DM, Chavez-Juan E, Anand S, Nuccitelli R, Kast WM. Nano-pulse stimulation induces immunogenic cell death in human papillomavirus-transformed tumors and initiates an adaptive immune response. PloS One. 2018;13(1):e0191311. doi:10.1371/journal.pone.0191311.
- Guo S, Jing Y, Burcus NI, Lassiter BP, Tanaz R, Heller R, Beebe SJ. Nano-pulse stimulation induces potent immune responses, eradicating local breast cancer while reducing distant metastases. Int J Cancer. 2018;142(3):629–40. doi:10.1002/ijc.31071.
- Guo S, Burcus NI, Hornef J, Jing Y, Jiang C, Heller R, Beebe SJ. Nano-pulse stimulation for the treatment of pancreatic cancer and the changes in immune profile. Cancers. 2018;10(7):217. doi:10.3390/cancers10070217.
- Lassiter BP, Guo S, Beebe SJ. Nano-pulse stimulation ablates orthotopic rat hepatocellular carcinoma and induces innate and adaptive memory immune mechanisms that prevent recurrence. Cancers. 2018;10(3): 69-88.
- Nuccitelli R, Wood R, Kreis M, Athos B, Huynh J, Lui K, Nuccitelli P, Epstein EH Jr. First-in-human trial of nanoelectroablation therapy for basal cell carcinoma: proof of method. Exp Dermatol. 2014;23(2):135–37. doi:10.1111/exd.12303.
- Vernier PT, Sun Y, Marcu L, Salemi S, Craft CM, Gundersen MA. Calcium bursts induced by nanosecond electric pulses. Biochem Biophys Res Commun. 2003;310(2):286–95. doi:10.1016/j.bbrc.2003.08.140.
- White JA, Blackmore PF, Schoenbach KH, Beebe SJ. Stimulation of capacitative calcium entry in HL-60 cells by nanosecond pulsed electric fields. J Biol Chem. 2004;279(22):22964–72. doi:10.1074/jbc.M311135200.
- Pakhomova ON, Khorokhorina VA, Bowman AM, Rodaite-Riseviciene R, Saulis G, Xiao S, Pakhomov AG. Oxidative effects of nanosecond pulsed electric field exposure in cells and cell-free media. Arch Biochem Biophys. 2012;527:55–64. doi:10.1016/j.abb.2012.08.004.
- Nuccitelli R, Lui K, Kreis M, Athos B, Nuccitelli P. Nanosecond pulsed electric field stimulation of reactive oxygen species in human pancreatic cancer cells is Ca2+-dependent. Biochem Biophys Res Commun. 2013;435(4):580–85. doi:10.1016/j.bbrc.2013.05.014.
- Nuccitelli R, Chen X, Pakhomov AG, Baldwin WH, Sheikh S, Pomicter JL, Ren W, Osgood C, Swanson RJ, Kolb JF, et al. A new pulsed electric field therapy for melanoma disrupts the tumor’s blood supply and causes complete remission without recurrence. Int J Cancer. 2009;125(2):438–45. doi:10.1002/ijc.24345.