Abstract
Purpose: To assess the prevalence of an abnormal number of ribs in a cohort of fetuses and neonates with trisomy 21 and compare this with a subgroup of fetuses without anomalies.
Materials and methods: Radiographs of 67 deceased fetuses, neonates, and infants that were diagnosed with trisomy 21 were reviewed. Terminations of pregnancy were included. The control group was composed of 107 deceased fetuses, neonates, and infants without known chromosomal abnormalities, structural malformations, infections or placental pathology. Cases in which the number of thoracic ribs or presence of cervical ribs could not be reliably assessed were excluded. The literature concerning vertebral patterning in trisomy 21 cases and healthy subjects was reviewed.
Results: Absent or rudimentary 12th thoracic ribs were found in 26/54 (48.1%) cases with trisomy 21 and cervical ribs were present in 27/47 (57.4%) cases. This prevalence was significantly higher compared to controls (28/100, 28.0%, Χ2(1) = 6.252, p = .012 and 28/97, 28.9%, Χ2(1) = 10.955, p < .001, respectively).
Conclusions: Rudimentary or absent 12th thoracic ribs and cervical ribs are significantly more prevalent in deceased fetuses and infants with trisomy 21.
Introduction
The mammalian vertebral column is separated into cervical, thoracic, lumbar, sacral, and coccygeal regions. The vertebrae in these different regions exhibit specific characteristics, allowing each segment of the spine to perform slightly different functions and movements. The thoracic vertebrae are the only rib-bearing vertebrae.
Throughout evolution, the pattern of the vertebral column in mammals has remained remarkably constant. Particularly in the cervical region, deviation from the standard number of seven vertebrae is rarely seen, regardless of the length of the neck [Citation1,Citation2]. Several researchers hypothesized that the stability of the cervical vertebral number is the result of developmental constraints or a stabilizing selection against changes in this number [Citation1,Citation3,Citation4]. The fact that selection against variations in caudally located parts of the vertebral column is less prominent and thoracolumbar and lumbosacral shifts are more common in the general population, could be a reflection of the craniocaudal direction in which the vertebral column is formed [Citation3,Citation5]. Formation of the cervical region of the vertebral column starts earlier in embryogenesis than the formation of the thoracic and lumbar regions. It has been suggested that the interactivity between the developing organ systems and the patterning of the embryonic axes is higher during early organogenesis than during later stages [Citation5,Citation6]. This can lead to a higher vulnerability to disruptions in embryonic development during the early stage in which the cervical vertebral column is formed, compared with the stages in which the thoracic and lumbar regions of the vertebral column are formed [Citation7]. A shift at the cervicothoracic junction, that occurs in the presence of a cervical rib, could therefore be regarded as a marker for disrupted embryonic development. This hypothesis is further supported by the higher prevalence of cervical ribs in individuals with adverse outcomes. Several studies showed a high frequency of (rudimentary) cervical ribs in specific study populations [Citation8], such as stillbirths and deceased neonates with and without structural anomalies [Citation4], children suffering from malignancies [Citation9] and extinct mammoths [Citation10].
A high prevalence of vertebral patterning anomalies has also been reported in cases with chromosomal anomalies [Citation8,Citation11–15]. However, the included study populations were relatively small and in only one of these studies the number of thoracic ribs was compared with a control group [Citation12].
The aim of this study was to assess the prevalence of an abnormal number of ribs and vertebrae in a cohort of deceased fetuses and neonates with trisomy 21 and compare this with a subgroup of deceased fetuses without anomalies, from the same cohort. The literature was reviewed in order to retrieve more information concerning the underlying cause for differences in vertebral patterning between trisomy 21 cases and healthy subjects.
Materials and methods
Between 1990 and 2010 all deceased fetuses and infants presented for autopsy at the VU Medical Center were routinely radiographed, both anteroposteriorly and laterally (23 mA, 70–90 kV, 4–12 s, Agfa Morsel, Belgium, Gevaert D7DW Structurix films). Deceased infants, spontaneous miscarriages, stillbirths, and induced abortions for medical reasons of at least 13 weeks of gestation were included. At least two observers (F.G., C.B.) independently reviewed the 1.389 radiographs, without prior knowledge of the presence of structural or chromosomal abnormalities.
Radiographs were assessed as previously described by Ten Broek et al. [Citation4], entailing the following: the visibility of the phalanges was taken as a measure of reliable visualization of the ossification centers. The number of vertebrae and ribs were counted. If a rib on the most cranial or most caudal thoracic vertebra had a length of less than half of the rib of the adjacent thoracic vertebra, it was considered rudimentary. Enlarged transverse processes were considered rudimentary cervical ribs if the length of the transverse process of the seventh cervical vertebra was more than the transverse process of the first thoracic vertebra [Citation5,Citation16,Citation17]. Karyotype was analyzed using fluorescent in situ hybridization (FISH). Information concerning the presence and type of additional structural anomalies was retrieved from standard autopsy reports, stored in a national pathological archive (PALGA). When pathological examination of organ systems was not possible due to maceration, it was categorized as nonavailable.
The control group consisted of deceased fetuses and neonates without known chromosomal abnormalities, structural malformations, infections or placental pathology. Chromosomal analysis was not routinely performed in the control group. The presence of cervical ribs and the number of thoracic ribs in cases with trisomy 21 and controls were compared.
Data were analyzed using SPSS statistics (IBM Corp released 2013. IBM SPSS statistics for windows, version 21.0. Armonk, NY: IBM Corp). Pearson Chi squared test was applied for the assessment of significance of the difference in the prevalence of an abnormal rib number between the two groups. A p-value < .05 was considered statistically significant.
Results
Of the 1470 radiographs, 104 cases with a gestational age from 14 to 19 weeks were excluded due to insufficient ossification. Another 233 cases were excluded because the cervicothoracic region could not be assessed. This was mainly caused by overprojection of the mandibula on the cervical spine, when the head of the fetus was not correctly positioned, or by inadequate radiographical techniques. This resulted in the assessment of a total of 1133 radiographs. 623 (55.0%) of these cases were male, 486 females (42.9%), and in 24 (2.1%) the sex was unknown. Karyotype was available in 162 of the 1133 (14.3%) cases.
In total, 67 of the 1470 (4.6%) cases had been diagnosed with trisomy 21, of which 37 (55.2%) were male and 30 (44.8%) female; 64 fetuses with a gestational age ranging from 13 + 5 weeks to 38 + 5 weeks and three neonates. In 13 of the 67 cases, the number of thoracic vertebrae and ribs could not be reliably assessed, leaving 54 radiographs available for analysis of the number of thoracic ribs. In approximately half of these cases with trisomy 21, the 12th thoracic rib was absent or rudimentary (26/54, 48.1%), which occurred bilaterally in the majority (N = 25, 96.2%).
In 20 of the 67 cases, the number of cervical vertebrae and ribs could not be reliably determined, which resulted in a number of 47 radiographs, available for analysis of the presence of cervical ribs. Cervical ribs were seen in 27 of the 47 cases (57.4%). Five (18.5%) of these were unilateral and 22 (81.5%) bilateral. Fifteen cases (31.9%) had both rudimentary cervical ribs and absent or rudimentary 12th thoracic ribs. A radiograph of a fetus with both rudimentary cervical ribs and absent 12th thoracic ribs is shown in .
For comparison, a subgroup of 107 (107/1.470, 7.3%) deceased fetuses, neonates and infants without structural or known chromosomal anomalies was selected. The gestational age of the included fetuses ranged from 13 + 6–41 + 6 weeks. The majority of this group consisted of unexplained stillbirths. Other possible causes of immature delivery and death were cervical insufficiency or premature rupture of membranes. In case of termination of pregnancy, only inductions of labor following intrauterine fetal death or terminations of pregnancy for maternal indications were included.
Seven cases were excluded because reliable assessment of the thoracic vertebrae and ribs was not possible and an additional three cases were excluded because the cervical region could not be reliably assessed. Within this group, 28 of the 100 (28.0%) cases had rudimentary or absent 12th thoracic rib(s), which was often bilaterally (N = 25, 89.3%). Cervical ribs were present in 28 of the 97 (28.9%) cases without anomalies, of which most were bilateral (N = 24, 85.7%). Sixteen cases (16.5%) had both cervical rib(s) and absent or rudimentary 12th thoracic rib(s). These results are shown in .
Figure 2. The percentage of absent or rudimentary 12th thoracic ribs and cervical ribs in fetuses with trisomy 21 compared with controls.
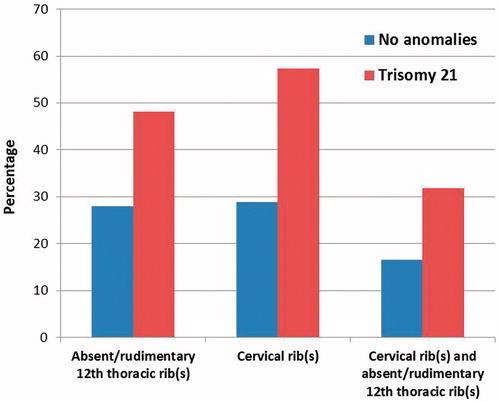
The differences in the prevalence of both rudimentary or absent 12th thoracic ribs and cervical ribs between the cases with trisomy 21 and the cases without anomalies were statistically significant (Χ2(1) = 6.252, p = .012 and Χ2(1) = 10.955, p < .001, respectively).
Structural anomalies were found in 33 (49.3%) of the cases with trisomy 21. Cardiovascular anomalies were the most frequent (17/33, 51.5%). In 23 cases (34.3%) no structural anomalies were reported and in another 11 cases (16.4%) no structural anomalies were confirmed, but one or more organ systems could not be adequately investigated during autopsy. No statistically significant differences in the presence of cervical ribs between cases with trisomy 21 with and without structural anomalies were found (Χ2(1) = 0.264, p = .607). The same applies to the presence of rudimentary or absent 12th thoracic ribs (Χ2(1) = 1.042, p = .307).
Discussion
The study demonstrates that in deceased fetuses, neonates and infants with trisomy 21, an abnormal vertebral pattern is very common and significantly more frequent than in the deceased fetuses, neonates and infants without structural or known chromosomal anomalies. In the latter group, the prevalence of cervical ribs and rudimentary or absent 12th thoracic ribs was relatively high compared to the general population, which can be explained by the fact that the control group consisted of deceased fetuses and neonates instead of healthy individuals.
In the general population or in patients without anomalies, a low prevalence of absent or rudimentary 12th thoracic ribs (0.4–6.6%) and cervical ribs (0.2–6.2%) is reported [Citation5,Citation8,Citation9,Citation17–34]. Bots et al. [Citation17] found a higher prevalence of agenesis of the 12th thoracic ribs (12.6%) and cervical ribs (37.7%) in fetuses with a gestational age ranging between 10 and 21 weeks that were electively aborted, but medical data concerning these pregnancies and the mothers were missing. In their study, alizarin red staining for calcium was used, while most other studies assessed the vertebral pattern using radiographs, which might have led to the detection of smaller osseous elements than on radiographs. This is supported by the detection of cervical ribs at a considerably earlier age in fetuses that had been stained with alizarin red compared to the detection on fetal radiographs (youngest estimated age of 10 versus 14 weeks) [Citation17]. The main findings of the studies addressing the presence of an abnormal rib number in cases with trisomy 21 are presented in .
Table 1. The reported prevalence of cervical ribs and absent 12th thoracic ribs in cases with trisomy 21, assessed on radiographs.
In these studies, the prevalence of absent 12th thoracic ribs ranged between 18.9 and 37.5%. Possible explanations for the lower prevalence of absent 12th thoracic ribs in some of these studies compared with the present study (48.1%), might be under-reporting of rudimentary 12th ribs, which in this study was classified under the same heading as agenesis of the 12th thoracic ribs.
The prevalence of cervical ribs in cases with trisomy 21 ranged from 12.5 to 57.1% in previously reported studies [Citation14,Citation15,Citation35]. The prevalence of cervical ribs in this study population was only slightly higher (57.4%) and not very different from the study of Castori et al. [Citation15], who included the largest study population.
Phenotypic differences between the included study populations could perhaps be partly responsible for the reported variety in prevalence of an abnormal number of thoracic ribs and cervical ribs. The severity of additional structural anomalies in trisomy 21 cases varies between individuals and it seems plausible that the study population of Willich et al. [Citation36], who included live born patients up to the age of 17, rather than deceased fetuses, had a milder phenotype and therefore less severe disruptions in vertebral patterning than the fetuses and neonates included in the present study.
The prevalence of an abnormal vertebral pattern was not higher in trisomy 21 cases with additional structural anomalies, compared to those without additional anomalies. In the light of the hypothesized negative effect of dysregulation of the anteroposterior axis on other developmental processes, a higher prevalence of an abnormal vertebral pattern would be expected in cases with additional structural anomalies. The small number of included cases might explain the inability to detect a significant difference.
The pathophysiology of the skeletal anomalies and other (phenotypic) anomalies found in patients with trisomy 21 remains unknown. Garcia-Ramírez et al. [Citation37] examined cartilage from different bone structures in 29 human fetuses with trisomy 21 and 13 controls. The microscopic appearance of the costochondral junctions differed significantly and showed hypertrophic disorganization and a reduction in proliferative and resting zones in trisomy 21 cases. They suggested that the increased number of hypertrophic cells might be the result of failure of progression to bone formation. Perhaps the absence of the 12th thoracic ribs could be related to this mechanism, which however does not explain the prevalence of cervical ribs. In addition, the simultaneously occurring absence of 12th thoracic ribs and presence of cervical ribs in approximately half of the cases makes it even more likely that the underlying process responsible for the anteroposterior patterning is disturbed.
In mammals, the anteroposterior patterning is regulated by Hox genes. These 39 genes are an evolutionary conserved family of genes that play a key role in the development of anteroposterior axis, but also in other physiologic and pathologic processes, such as oncogenesis [Citation38,Citation39]. Several experiments on chicks and mice have shown that Hox-gene mutations can lead to homeotic transformations [Citation40]. In homozygous Hoxa4, Hoxa5, and Hoxb8-mutant mice, cervical ribs had developed on the seventh cervical vertebra [Citation41–43]. Although the human Hox genes are not located on chromosome 21, it has been proposed that overexpression of genes from the trisomic chromosome lead to a generalized transcriptional misregulation [Citation44]. Research on gene expression in humans and mice with trisomy 21 not only showed a significant different expression pattern of genes located on chromosome 21, but also of genes mapped to other chromosomes [Citation45–47]. Furthermore, microarray analysis of mandibular precursors in Down syndrome mice showed that six Hox genes were amongst the most dysregulated genes [Citation48]. Therefore, dysregulation of Hox gene expression in cases with trisomy 21 may lead to disruptions in vertebral patterning. So far, this hypothesis has not been confirmed and should be further investigated in future studies.
The fetal vertebral column and the number of thoracic ribs can be assessed using 2- and 3-dimensional ultrasonography [Citation21,Citation49]. The value of prenatal 3-dimensional ultrasonography for the assessment of cervical ribs has not been investigated. Although an abnormal number of ribs can be found in healthy individuals, the presence of an abnormal number of thoracic ribs in a fetus should alert the sonographer that other structural or chromosomal anomalies may be present. Prospective studies are required for the evaluation of the association between an abnormal number of ribs found on prenatal ultrasound and other structural or chromosomal anomalies.
Limitations of this study were the absence of a healthy control group and the limited availability of karyotyping in fetuses without structural malformations. Therefore, it cannot be assumed that all subjects from the control group had a normal karyotype. It seems unlikely though, that fetuses with trisomy 21 or other severe chromosomal aberrations were included in this group, because pathological examination was performed in all cases and even mild anomalies, including dysmorphic features, were registered. Within our study population, this subgroup seemed the most suitable subpopulation that was available for comparison. As the differences in prevalence of rudimentary or absent 12th thoracic ribs and presence of cervical ribs between these two groups were already statistically significant, these will most likely become even more evident when compared with healthy subjects.
Strengths of this study were the availability of pathologic reports of all cases, the assessment of the radiographs by two different observers and the inclusion of a control group without structural and known chromosomal anomalies. The included number of cases was also relatively large, compared with previously reported studies.
Based on the study results and the available literature, it can be concluded that the prevalence of an abnormal vertebral pattern is significantly higher in fetuses and neonates with trisomy 21 compared with fetuses without anomalies and compared with the general population. Further elucidation of the underlying pathophysiological mechanism, which could be due to dysregulation of Hox gene expression, is needed.
Acknowledgements
We thank Ron Otsen and Jaap van Veldhuisen for the high-quality radiographs, Irma Varela-Lasheras and Jessica Bots for digitizing and Alexander Bakker for analyzing the radiographs. Patient data and radiographs were used according to the guidelines of the Medical Ethics Committee of the VU University Medical Center and patient anonymity was strictly maintained. Parental written informed consent was obtained for patients and data were handled in a coded and completely anonymous fashion, according to Dutch national ethical guidelines (Code for Proper Secondary Use of Human Data, Dutch Federation of Medical Scientific Societies; http://www.federa.org/codes-conduct).
Disclosure statement
The authors report no conflicts of interest.
References
- Galis F. Why do almost all mammals have seven cervical vertebrae? Developmental constraints, Hox genes, and cancer. J Exp Zool. 1999;285:19–26.
- Hautier L, Weisbecker V, Sánchez-Villagra MR, et al. Skeletal development in sloths and the evolution of mammalian vertebral patterning. Proc Natl Acad Sci USA. 2010;107:18903–18908.
- Narita Y, Kuratani S. Evolution of the vertebral formulae in mammals: a perspective on developmental constraints. J Exp Zool B Mol Dev Evol. 2005;304:91–106.
- Ten Broek CM, Bakker AJ, Varela-Lasheras I, et al. Evo-Devo of the human vertebral column: on homeotic transformations, pathologies and prenatal selection. Evol Biol. 2012;39:456–471.
- Galis F, Van Dooren TJM, Feuth JD, et al. Extreme selection in humans against homeotic transformations of cervical vertebrae. Evolution. 2006;60:2643–2654.
- Galis F, Metz JA. Testing the vulnerability of the phylotypic stage: on modularity and evolutionary conservation. J Exp Zool. 2001;291:195–204.
- Galis F, Metz JAJ. Evolutionary novelties: the making and breaking of pleiotropic constraints. Integr Comp Biol. 2007;47:409–419.
- Schut PC, Cohen-Overbeek TE, Galis F, et al. Adverse fetal and neonatal outcome and an abnormal vertebral pattern: a systematic review. Obstet Gynecol Surv. 2016;71:741–750.
- Merks JHM, Smets AM, Van Rijn RR, et al. Prevalence of RIB anomalies in normal Caucasian children and childhood cancer patients. Eur J Med Genet. 2005;48:113–129.
- Reumer JW, Ten Broek CM, Galis F. Extraordinary incidence of cervical ribs indicates vulnerable condition in Late Pleistocene mammoths. PeerJ. 2014;2:e318.
- Beber BA. Absence of a rib in Down’s syndrome. Lancet. 1965;2:289.
- Edwards DK, Berry CC, Hilton SW. Trisomy 21 in newborn infants: chest radiographic diagnosis. Radiology. 1988;167:317–318.
- Keeling JW, Kjaer I. Cervical ribs: useful marker of monosomy X in fetal hydrops. Pediatr Dev Pathol. 1999;2:119–123.
- Furtado LV, Thaker HM, Erickson LK, et al. Cervical ribs are more prevalent in stillborn fetuses than in live-born infants and are strongly associated with fetal aneuploidy. Pediatr Dev Pathol. 2011;14:431–437.
- Castori M, Servadei F, Laino L, et al. Axial skeletogenesis in human autosomal aneuploidies: a radiographic study of 145 second trimester fetuses. Am J Med Genet A. 2016;170:676–687.
- Bagnall KM, Harris PF, Jones PRM. A radiographic study of variations of the human fetal spine. Anat Rec. 1984;208:265–270.
- Bots J, Wijnaendts LCD, Delen S, et al. Analysis of cervical ribs in a series of human fetuses. J Anat. 2011;219:403–409.
- Zierhut HA, Murati MA, Holm T, et al. Association of rib anomalies and childhood cancers. Cancer Res. 2011;105:1392–1395.
- Nakajima A, Usui A, Hosokai Y, et al. The prevalence of morphological changes in the thoracolumbar spine on whole-spine computed tomographic images. Insights Imaging. 2014;5:77–83.
- Schumacher R, Mai A, Gutjahr P. Association of rib anomalies and malignancy in childhood. Eur J Pediatr. 1992;151:432–434.
- Hershkovitz R. Prenatal diagnosis of isolated abnormal number of ribs. Ultrasound Obstet Gynecol. 2008;32:506–509.
- Southam AH, Bythell WJ. Cervical ribs in children. Br Med J. 1924;2:844–845.
- Etter M. Osseous abnormalities of the thoracic cage seen in forty thousand consecutive chest photoroentgenograms. Am J Roentgenol. 1944;51:359–363.
- Sycamore L. Common congenital anomalies of the bony thorax. Am J Roentgenol. 1944;51:593–599.
- Singh HK. Incidence of congenital rib anomalies. Indian J Chest Dis. 1973;15:157–164.
- Kimonis VE, Mehta SG, DiGiovanna JJ, et al. Radiological features in 82 patients with nevoid basal cell carcinoma (NBCC or Gorlin) syndrome. Genet Med. 2004;6:495–502.
- Gulekon IN, Turgut HB. The prevalence of cervical rib in Anatolian population. Gazi Med J. 1999;10:149–152.
- Erken E, Ozer HTE, Gulek B, et al. The association between cervical rib and sacralization. Spine (Phila Pa 1976). 2002;27:1659–1664.
- Loder RT, Huffman G, Toney E, et al. Abnormal rib number in childhood malignancy: implications for the scoliosis surgeon. Spine (Phila Pa 1976). 2007;32:904–910.
- Brewin J, Hill M, Ellis H. The prevalence of cervical ribs in a London population. Clin Anat. 2009;22:331–336.
- Bokhari RF, Al-Sayyad MJ, Baeesa SS. Prevalence of cervical ribs and elongated transverse processes in Saudi Arabia. Saudi Med J. 2012;33:66–69.
- Venkatesan V, Prabhu KP, Ram Kumar B, et al. Incidence of cervical rib in Chennai population. World J Med Sci. 2014;10:250–253.
- Walden MJ, Adin ME, Visagan R, et al. Cervical ribs: identification on MRI and clinical relevance. Clin Imaging. 2013;37:938–941.
- Viertel VG, Intrapiromkul J, Maluf F, et al. Cervical ribs: a common variant overlooked in CT imaging. Am J Neuroradiol. 2012;33:2191–2194.
- Keeling JW, Hansen BF, Kjaer I. Pattern of malformations in the axial skeleton in human trisomy 21 fetuses. Am J Med Genet. 1997;68:466–471.
- Willich E, Fuhr U, Kroll W. Skeletal changes in Down’s syndrome. A correlation between radiological and cytogenetic findings. Fortschr Geb Rontgenstr Nuklearmed. 1977;127:135–142.
- Garcia-Ramírez M, Toran N, Carrascosa A, et al. Down’s syndrome: altered chondrogenesis in fetal rib. Pediatr Res. 1998;44:93–98.
- Mallo M, Alonso CR. The regulation of Hox gene expression during animal development. Development. 2013;140:3951–3963.
- Quinonez SC, Innis JW. Human HOX gene disorders. Mol Genet Metab. 2014;111:4–15.
- Wellik DM. Hox genes and vertebrate axial pattern. Curr Top Dev Biol. 2009;88:257–278.
- Horan GSB, Ramírez-Solis R, Featherstone MS, et al. Compound mutants for the paralogous hoxa-4, hoxb-4, and hoxd-4 genes show more complete homeotic transformations and a dose-dependent increase in the number of vertebrae transformed. Genes Dev. 1995;9:1667–1677.
- Jeannotte L, Lemieux M, Charron J, et al. Specification of axial identity in the mouse: role of the Hoxa-5 (Hox1.3) gene. Genes Dev. 1993;7:2085–2096.
- Charité J, De Graaff W, Deschamps J. Specification of multiple vertebral identities by ectopically expressed Hoxb-8. Dev Dyn. 1995;204:13–21.
- FitzPatrick DR, Ramsay J, McGill NI, et al. Transcriptome analysis of human autosomal trisomy. Hum Mol Genet. 2002;11:3249–3256.
- Rozovski U, Jonish-Grossman A, Bar-Shira A, et al. Genome-wide expression analysis of cultured trophoblast with trisomy 21 karyotype. Hum Reprod. 2007;22:2538–2545.
- Laffaire J, Rivals I, Dauphinot L, et al. Gene expression signature of cerebellar hypoplasia in a mouse model of Down syndrome during postnatal development. BMC Genomics. 2009;10:138.
- Sommer CA, Pavarino-Bertelli EC, Goloni-Bertollo EM, et al. Identification of dysregulated genes in lymphocytes from children with Down syndrome. Genome. 2008;51:19–29.
- Billingsley CN, Allen JR, Baumann DD, et al. Non-trisomic homeobox gene expression during craniofacial development in the Ts65Dn mouse model of Down syndrome. Am J Med Genet A. 2013;161A:1866–1874.
- Gindes L, Benoit B, Pretorius DH, et al. Abnormal number of fetal ribs on 3-dimensional ultrasonography: associated anomalies and outcomes in 75 fetuses. J Ultrasound Med. 2008;27:1263–1271.