Abstract
Objective
The purpose of this study is to test the hypothesis that race and supplementation affect the concentration and correlation of various folate species in maternal and umbilical cord blood.
Methods
This is a single-center, prospective, cross-sectional cohort of cord blood samples obtained from 40 uncomplicated term pregnancies as a pilot study, following a protocol approved by the Institutional Review Board. High performance liquid chromatography mass spectrometry quantitated the following concentrations in extracted plasma samples: 5-methyltetrahydrofolate (5MTHF), 5,10-methenyl-tetrahydrofolate (5,10-MeTHF), tetrahydrofolate (THF), and unmetabolized folic acid.
Results
Folate concentrations in the umbilical cord plasma were consistently higher than maternal samples for 5MTHF (p < .001), 5,10-MeTHF (p < .001), and THF (p < .001); cord blood folic acid levels, however, were lower than maternal samples (p < .03). While 5MTHF was the most prevalent folate, ratios comparing cord blood to maternal blood folates suggests a fourfold preponderance of THF in cord blood folate signature, a trend unchanged by supplementation. Prenatal supplementation increased the concentrations of 5MTHF, for both maternal (p < .01) and cord blood samples (p < .005). In comparison to the other two racial groups, African American 5MTHF concentration demonstrated a lower total folate concentration in both maternal samples and cord blood samples, in addition to a relatively blunted response to supplementation. A significantly positive correlation between maternal and cord blood 5MTHF concentration was noted in all three racial groups. Supplementation resulted in a positive correlation between maternal and cord blood 5MTHF concentrations (r = 0.85, p < .0001).
Conclusions
5MTHF is the most prevalent folate in both cord and maternal plasma, and race and supplementation primarily affect variations in maternal and fetal 5MTHF concentrations and their correlation with each other. However, the greater concentration of THF in cord blood relative to maternal blood offers preliminary insight into the importance of how folate metabolism differs in the specific context of fetal development and physiology, with greater emphasis on DNA synthesis and stability. Furthermore, supplementation appeared to not have as great an impact on African American maternal or cord blood folates, suggesting a variable benefit of current repletion strategies to certain subsets of the population. Future studies that further elucidate these differences and their impact on birth outcomes may help inform supplementation protocols that are more personalized, with greater efficacy in promoting positive perinatal outcomes.
Keywords:
Introduction
Folic acid, a synthetic folate, functions as a highly effective nutritional prophylaxis against congenital anomalies including cardiac malformations [Citation1], cleft lip and palate [Citation2], and neural tube defects [Citation3]. The CDC currently recommends a dose of at least 0.4 mg/day for all women capable of pregnancy, and most prenatal vitamin formulations contain at least 400 μg of folic acid [Citation4]. Additionally, in 1998, the US Food and Drug Administration mandated supplementation of 140 µg of folic acid for every 100 g of cereal and whole grain, and since the initiation of this program, the CDC observed a 26% reduction of neural tube defects in pregnancy [Citation5,Citation6].
Interestingly, the 26% reduction of neural tube defects in the USA falls below the projected 50% decline the CDC had anticipated with mandatory folate fortification in the grain supply [Citation7]. One reason for this disparity may be nutritional lapses among women of childbearing potential. In addition to a reported 16% decline in plasma total folate among reproductive females due to pervasive changes in dietary habits [Citation8], cultural and racial disparities exist in both dietary consumption of folate and folic acid and the use of prenatal vitamins [Citation9–11].
Studies also suggest a differential racial response to grain fortification and supplements, and current repletion strategies do not account for these intrinsic differences in maternal folate metabolism. A 2002 CDC study found that African American women had a lower folate reserve than both Caucasian and Latina women, a discrepancy that persisted even after implementation of grain fortification [Citation8].
Furthermore, if little is known regarding folic acid metabolism among reproductive women, even less is known regarding the mechanism of fetal utilization of maternal folates. Maternal folate stores and folic acid supplementation probably fulfill an increased demand for one-carbon metabolism during pregnancy, which is critical in fetal DNA synthesis and maintenance, epigenetic programing via methylation, cell proliferation, and amino acid synthesis. However, very few studies have examined the impact that supplementation and maternal habits have on fetal folate stores, and only one study has analyzed the impact of folic acid consumption on various folate species in cord blood [Citation12]. Moreover, while the maternal–cord blood correlations of folic acid [Citation13] and vitamin B12 (a cofactor in the folate cycle) [Citation14] have been described, no study, to our knowledge, has described the maternal-cord blood correlation of multiple folate species, including the most physiologically available and plentiful folate, 5-methyltetrahydrofolate (5MTHF), and none have examined the impact of race on these correlations and cord blood samples.
Therefore, this study tests the hypothesis that race and supplementation affect the concentration and correlation of various folate species in maternal and umbilical cord blood samples, utilizing ultraperformance liquid chromatography (UPLC)–tandem mass spectrometry.
Materials and methods
Patient population
This is a single-center, cross-sectional analysis of patients with normal, singleton pregnancies. Forty maternal-cord blood sample pairs were collected at delivery to look at various aspects of folate metabolism.
In all cases, the patients, who were between 16 and 40 years of age, were recruited in an outpatient setting during routine prenatal care visits, using a protocol that was approved by the affiliated Institutional Review Board of St. Luke’s Hospital. Informed consent was obtained, using good clinical and ethical practice; if women were less than 18 years of age, both the patient and their designated guardian provided consent.
In the clinic, an interviewer-administered questionnaire was utilized to obtain information regarding race, medical history, body mass index (BMI), maternal habits including smoking and prenatal vitamin use. The type of prenatal vitamin used by this cohort was a generic form easily obtained over the counter. The pregnancy was followed via chart review to search for gestation-related complications such as preterm birth, preeclampsia, and diabetes. Exclusion criteria included longstanding history of anemia, seizures, prior pregnancy affected by neural tube defect, and history of gastric bypass surgery or current intestinal malabsorption.
During delivery, the patient had a non-fasting venous blood sample collected, using EDTA tubes, as well as a mixed arterial-venous umbilical cord sample, prior to placental separation.
Preparation of samples
After the blood was collected, it was immediately shielded from light using foil and placed on ice. Within 12 h of collection, the samples were then centrifuged at 2000 rpm for 10 min in a desktop centrifuge at 4 °C. The plasma supernatant was then recovered in aliquots and stored in amber vials in an −80 °C freezer for future analysis.
Quantification of plasma folates
Folate analysis in whole blood and plasma was conducted per previously published procedure using UPLC tandem mass spectrometry [Citation15]. The following folate species were measured: 5MTHF, 5,10-methenyl- tetrahydrofolate (5,10-MeTHF), tetrahydrofolate (THF), and unmetabolized folic acid.
Folate extraction was performed on 50 ml aliquots of plasma, to which 25 ml of internal standard solution containing 100 nM of the isotope-labeled internal standards were added and vortexed for 10 s. Subsequently, 20 ml of 10% (w/v) trichloroacetic acid was added, vortexed, and centrifuged to precipitate and discard proteins. Samples were then immediately neutralized with 200 ml of extraction buffer. Aliquots of the supernatant were transferred to autosampler vials with a 250 ml inserts for analysis of content.
The plasma folate assays were validated using standards. The recovery of the different folate species was estimated by spiking (in triplicate) the [13C5]-labeled standards in the pooled matrix at 10, 50, and 100 nM for plasma and comparing the analyte response (AUC) of the extracted spiked biological sample to an aqueous reference control prepared at a similar concentration. Precision and accuracy were tested by a standard addition experiment, adding known concentrations of the analytes to a pooled matrix 0, 5, 25, and 50 nM for plasma. During this experiment, the [13C5]-labeled internal standards were spiked in at 50 nM for plasma. Intrarun precision was determined by measuring five replicates of each concentration in one day. Inter-run precision was assessed by the measurement of five replicates of each concentration for a three-day period.
The linear range and lower limits of quantification (LLOQ) and limits of detection (LOD) were assessed by adding the [13C5]-labeled analytes at concentrations of 0.1–100 nM to plasma. The LLOQ was defined as the lowest concentration that yielded peaks with signal-to-noise (S/N) ratios of 10, and the LOD was defined as the lowest concentration that yielded peaks with S/N ratios of 3.
Separation was conducted on an Acquity UPLC BEH C18 column (2.1 100 mm; Waters, Milford, MA, USA) packed with 1.7-mm particles. The column was protected by an Acquity UPLC BEH C18 VanGuard precolumn (2.1 5 mm; Waters, Milford, MA, USA) packed with 1.7 mm particles. Mobile phase A consisted of 1% acetic acid in H2O, and mobile phase B was a blend of 25% ACN, 74% MeOH, and 1% acetic acid. The folates were eluted utilizing a gradient elution program followed by a step gradient to return to initial conditions.
Mass spectrometry was then performed using a Xevo TQS “triple” quadrupole mass spectrometer (Waters, Manchester, UK) equipped with an electrospray ionization (ESI) source.
Statistics
This is a pilot study. As no previous data exist quantifying folate in normal pregnancy among different racial groups, no sample size calculation was performed. The data analysis was performed using SAS software (SAS Inc., Cary, NC, USA). For both maternal and cord plasma, mean concentrations with standard deviation were calculated for each of the different folates. As the data collected followed a normal distribution, differences between values were determined using Student’s t test, with a significance defined as a p value < .5. To study the relationship between maternal and cord plasma values, bivariate linear regression was performed, with calculated Pearson’s coefficients, to characterize maternal-cord blood folate correlations.
Results
Subject characteristics
Among the 40 mother-cord blood pairs analyzed in this study, mean maternal age was 23.5 ± 5 years, with a BMI of 31 ± 9.3 and 60% with an obese prepregnancy BMI, and 35.7% were multiparous. Eighteen of the women were African American, while 12 were Latina and 10 were Caucasian. Three subjects (7.5%) had a positive history of smoking. The majority of patients (57.5%) took prenatal vitamins during pregnancy at the time of prenatal intake. Among the different races, 45% of African American, 66.7% of Latina, and 60% of Caucasian women used supplementation.
Gestational age at the time of collection at delivery was 39 ± 1.8 weeks. Three patients developed gestational diabetes, and three patients developed preeclampsia. There were no preterm births. Mean birthweight was 3221 ± 385 g. Three women were smokers.
Maternal and fetal folate quantification
Cord blood concentrations were significantly higher than their corresponding maternal levels in all folate species, with the exception of folic acid (); cord blood plasma folic acid levels were consistently lower than maternal plasma levels. Furthermore, 5MTHF was, by far, the most prevalent folate species, in both maternal (39.12 nmol/L, 89% of total folate) and cord blood plasma samples (59.5 nmol/L, 94% of total folate). This trend continued with and without supplementation () and within each racial group (). That said, when looking at cord blood/maternal ratios of each plasma folate, THF concentration in cord blood was four to fivefold higher than maternal blood, a significant trend not seen with other folates (). This trend was not affected by supplementation.
Table 1. Concentrationsa of maternal and cord blood plasma folate isoforms.
Table 2. The effect of supplementation on concentrationsTable Footnotea of maternal and cord blood folate isoforms.
Table 3. Racial differences in maternal and cord blood folatesTable Footnotea.
Supplementation appeared to primarily affect 5MTHF concentrations, with higher levels noted with prenatal vitamin use in both maternal (45.1 versus 31.1 nmol/L, p < .001) and cord blood samples (67.3 nmol/L versus 49.1/L nmol, p < .01), while not similarly affecting the concentrations of other folate species (). Interestingly, folic acid concentrations were noted in both maternal and cord blood of pregnancies where the mothers reported no prenatal vitamin use, which was probably indicative of folic acid consumption via grain fortification.
African American pregnant mothers had a lower concentration of 5MTHF (), in comparison to Caucasian women (30.1 versus 53.4 nmol/L, p < .002). African American women also had a lower concentration of 5MTHF than Latina women, though the difference was not significant (30.1 versus 40.8 nmol/L, p < .07). A similar trend was seen with cord blood. African American cord blood 5MTHF concentration (46.0 nmol/L) was much lower in comparison with both Caucasian (71.8 nmol/L, p < .001) and Latina samples (69.6 nmol/L, p < .01). This racial difference was not seen with the other folate species, for both maternal and cord blood samples.
Of note, three outliers were noted in the maternal blood samples, with a much higher concentration of folic acid; the outliers did not significantly affect the observed maternal-fetal difference in folic acid concentrations. The outliers belonged to one Caucasian woman (25.9 nmol/L) and one African American woman (37 nmol/L), who took prenatal vitamins, and one Latina woman (43.1 nmol/L) who did not take prenatal vitamins; despite their high levels of folic acid, the corresponding cord blood values (0.4 nmol/L, 0.6, and 0.5 nmol/L, respectively) did not deviate significantly from the mean (0.4 nmol/L).
Furthermore, racial differences existed not only in 5MTHF concentrations but also in response to supplementation. Prenatal vitamin use increased the 5MTHF folate concentrations of Latina and Caucasian cord plasmas while African American cord plasma levels changed less (), demonstrating a comparatively blunted response to supplementation.
Figure 1. The effect of supplementation on plasma folates by race. This figure compares the differential response to prenatal vitamin use between African American samples (supplemented, n = 9; unsupplemented, n = 9) and combined Caucasian and Latina samples (supplemented, n = 14; unsupplemented, n = 8) on plasma 5MTHF concentrations, for both maternal and cord blood. *The difference seen with supplementation was significant for Caucasian and Latina women in both maternal (p < .02) and cord blood (p < .03), while it was not significant for African American blood samples (maternal, p < .93; cord blood, p < .3).
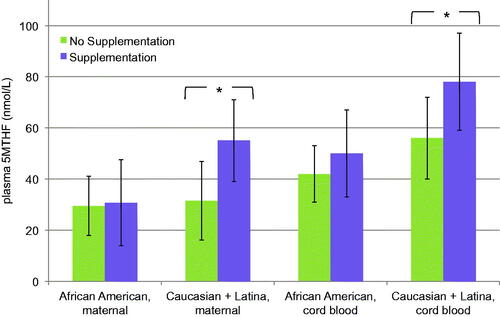
Maternal-fetal correlation and supplementation
The data demonstrated a positive correlation between maternal and cord blood plasma 5MTHF (r = 0.75, p < .001; ); however, this correlation was far weaker when only unsupplemented mothers were included (r = 0.46, p = .07), and far stronger when only supplemented mothers were included (r = 0.85, p < .0001). Therefore, the correlation existed far more predictably with supplementation, with overall higher concentrations of both cord and maternal blood samples.
Figure 2. Correlation between maternal and cord blood plasma 5MTHF. This figure presents graphs that correlate the maternal plasma 5MTHF (x-axis) concentrations (nmol/L) to umbilical cord plasma 5MTHF concentrations (y-axis); graphs b and c look at the same correlation without and with maternal prenatal vitamin supplementation, respectively. Bivariate linear regression analysis with r values representing Pearson’s correlation coefficients. p Values were calculated using Student’s t test.
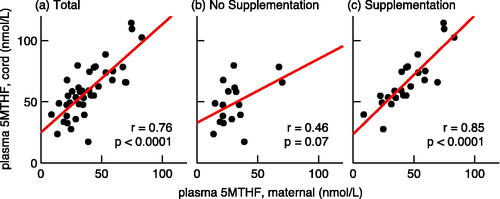
Moreover, the correlation of concentrations observed in the unsupplemented population appeared scattered, with some patients demonstrating high cord blood values despite low corresponding maternal values. Though the linear regression demonstrated a weak correlation, it was driven by two outliers, where the maternal and cord blood concentrations of 5MTHF are both high (one Latina and one Caucasian patient, both with normal pregnancies and birth outcomes).
This positive correlation with supplementation, again, only appeared significant for 5MTHF and not for the other folate species (); similarly, in all three racial groups, a significant positive correlation was observed only with 5MTHF: African Americans (r = 0.52, p < .02), Caucasians (r = 0.66, p < .04), and Latina women (r = 0.88, p < .002).
Table 4. Correlation between maternal and cord blood folatesTable Footnotea.
Discussion
As far as we know, our study may be the first to characterize racial trends in cord blood folate status and maternal-cord blood correlations. Our study demonstrates that, regardless of race, the most plentiful physiologic folate in both cord and maternal blood is 5MTHF, which is consistent with findings from previous studies [Citation12,Citation16,Citation17]. Furthermore, statistically significant positive correlations between maternal and cord blood 5MTHF were observed in all three racial groups, suggesting that maternal 5MTHF levels are consistently predictive of fetal folate status. These two findings together imply that 5MTHF plays a critical role in both maternal and fetal folate-mediated processes during pregnancy. 5MTHF feeds into the S-adenosyl methionine cycle that supplies methyl donors for DNA methylation, thereby affecting genetic expression.
Cord blood, not surprisingly, had higher levels of 5MTHF, THF, and 5,10-MeTHF than maternal samples, a finding that corroborates previous studies [Citation18–22]. This is likely due to placental transport and preferential depletion of maternal stores in favor of the fetus.
While 5MTHF appears generally the most plentiful folate, cord blood/maternal blood ratios suggest a significant preponderance of THF within the fetal circulation, relative to maternal blood. This is not surprising given the conversion of 5MTHF back to THF drives the synthesis of methyl groups that drive DNA expression. Also, THF, after conversion by dihydrofolate reductase (DHFR) from dihydrofolate, plays a critical role as a pivotal entryway into the folate cycle, especially with respect to DNA and protein synthesis and stability. Diminished THF, in relation to dysfunctional DHFR enzyme activity, has been shown to increase the risk of uracil misincorporation into DNA, increasing mutations and apoptosis [Citation23]; therefore, robust fetal stores may be protective against potential developmental disruption. The increased THF concentrations in fetal blood also implies potential preferential transfer from maternal blood or possible increased DHFR activity; however, DHFR activity, while implicated in the pathogenesis of conotruncal heart anomalies and neural tube defects in animal models [Citation24,Citation25], has not been well described in human fetal tissues, and it would be particularly interesting to study how dietary exposure and race informs genetic expression and enzyme activity within the folate cycle.
With regard to racial differences, consistent with the aforementioned 2002 CDC study [Citation8], this study found African American mothers had a lower folate status and a less dramatic response to supplementation, in comparison to the other racial groups, and this trend similarly affects cord blood concentrations. 5MTHF drives most of these differences seen with supplementation and race.
This finding may support a recent postulation that 5MTHF may be a better repletion strategy than folic acid among reproductive females [Citation26,Citation27]. Folic acid must be deglutamated and reduced prior to eventual conversion to 5MTHF and entry into the folate cycle. However, by bypassing these additional steps, 5MTHF may offer a more direct form of supplementation that more readily rectifies these racial differences in a way that positively affects the fetus.
Given this trend among African Americans, questions arise regarding how this disparity influences their pregnancy outcomes. Despite their lower folate concentrations, which is associated typically with an increased risk of neural tube defects, African Americans have the lowest incidence of neural tube defects among the three races studied [Citation23]. This paradox suggests the possibility of a genetically mediated efficiency in folate metabolism in regards to first-trimester organogenesis. However, other studies imply that this lower folate status in African Americans may play a role in their increased prevalence of late pregnancy complications, such as preterm birth [Citation28–31] and preeclampsia [Citation32,Citation33]. It remains unclear how lower folate concentrations may improve outcomes in early pregnancy but contribute to complications seen later in gestation; perhaps a subtlety in folate metabolism that has yet to be elucidated may explain this phenomenon. One possibility may align with a current theory proposing that darker melanin prevents UV-mediated folate degradation in the circulation at the dermal layer [Citation34]; given African American women with darker melanin are less susceptible to folate oxidation, they may need lower levels of folates to promote proper embryo development and pregnancy health compared to lighter-skinned mothers.
In terms of supplementation, prenatal vitamin use increased the folate 5MTHF concentrations in cord blood; supplementation also made the maternal concentrations of 5MTHF more predictive of folate concentrations. However, some maternal and cord blood concentrations remained low despite supplementation. Conversely, in the unsupplemented group, as the distribution was more scattered, some maternal samples with low folate concentrations interestingly translated into higher cord blood levels than the correlation with supplementation would otherwise predict. In both cases, this study suggests that potential intrinsic physiologic processes may regulate folate transfer to the fetus, independent of dietary and supplementation habits.
The three outliers in maternal folic acid concentrations require further consideration. The persistence of high levels of unmetabolized folic acid in maternal blood suggests a surplus and the possibility that a subset of the population may not efficiently process this increased supply [Citation35]. However, despite the high levels observed in maternal plasma, corresponding cord blood values did not vary from the mean as dramatically. This implies that despite aberrant maternal folic acid metabolism, the transfer of folic acid to the fetus remains highly regulated.
This study’s main strength is that it prospectively recruited patients, with a varied representation of racial groups and supplementation habits. As mentioned, it is the only study which seeks to look at racial differences in cord blood folates, and the only study to characterize the maternal–cord blood correlations of multiple folate species. The concurrent measurement of multiple different folate species, as opposed to only analyzing total folate concentrations, allowed for greater specificity in delineating differences in folate status.
While plasma folate is indicative of bioavailability, one of the weaknesses of the study is the use of non-fasting maternal plasma samples, which are vulnerable to short term and inconsistent dietary habits. In the future, the inclusion of erythrocyte folate profiles may be beneficial in examining long-term status that is less susceptible to diet. Also, prenatal vitamin use was determined via self-report, and consistent use was not verified at multiple points in the pregnancy. Performing a validated dietary questionnaire, alongside blood collection, may help explain how eating habits inform the differences seen in folate concentrations.
Furthermore, vitamin B12 levels were not measured alongside the folate concentrations; vitamin B12 deficiency is associated with impairment of methionine synthase which can result in the accumulation of 5MTHF. It is unlikely to affect supplemented mothers, as most formulations have 6 μg of vitamin B12; with unsupplemented mothers, however, it may falsely elevate 5MTHF levels, diminishing differences seen within the cohort [Citation36]. Nonetheless, vitamin B12 deficiency clinically manifests as megaloblastic anemia, which was part of the exclusion criteria for the study, and it is unlikely any of the mothers in this study were affected.
Additionally, a high proportion of the cohort was obese, which may be associated with altered pharmacokinetics of folic acid and may have affected the trends seen among the mothers in this cohort [Citation37,Citation38]. However, controversy exists whether changes seen in folate metabolism among obese women has to do with total body weight or lean body mass [Citation39] and whether observed differences warrant additional supplementation with folic acid and/or vitamin B12 [Citation40]. Also, an initial assessment of our data found no correlation between BMI and folate concentrations, with no significant differences seen between mean 5MTHF concentrations between obese (BMI ≥ 30) and nonobese mothers (p = .23). Nevertheless, a future study may consider a larger cohort that can better elucidate previously observed trends with regard to folate signature among obese mothers and delineate whether similar racial differences exist within subcategories of obese and non-obese women.
Finally, this study is limited by sample size. A larger study population may help better characterize the racial differences, alongside pregnancies affected by birth outcomes potentially affected by folate deficiency, such as congenital heart defects, neural tube defects, preterm birth, and preeclampsia.
Eventually, this study emphasizes the need for further investigation in elucidating racial differences, exploring fetal folate utilization and optimizing perinatal outcomes through personalized folate repletion strategies in the future.
Disclosure statement
No potential conflict of interest was reported by the authors.
References
- Ionescu-Ittu R, Marelli AJ, Mackie AS, et al. Prevalence of severe congenital heart disease after folic acid fortification of grain products: time trend analysis in Quebec, Canada. BMJ. 2009;338(2):b1673.
- Yazdy MM, Honein MA, Xing J. Reduction in orofacial clefts following folic acid fortification of the U.S. grain supply. Birth Defect Res A. 2007;79(1):16–23.
- Czeizel AE, Dudás I. Prevention of the first occurrence of neural-tube defects by periconceptional vitamin supplementation. N Engl J Med. 1992;327(26):1832–1835.
- Centers for Disease Control (CDC). Use of folic acid for prevention of spina bifida and other neural tube defects – 1983–1991. MMWR Morb Mortal Wkly Rep. 1991;40(30):513–516.
- Food and Drug Administration. Food standards: amendment of standards of identity for enriched grain products to require addition of folic acid. Fed Regist. 1996;61:8781–8797.
- Ishikawa T, Obara T, Nishigori H, et al. Update on the prevalence and determinants of folic acid use in Japan evaluated with 91,538 pregnant women: the Japan Environment and Children’s Study. J Matern Fetal Neonatal Med. 2018;31:1–10.
- Erickson JD. Folic acid and prevention of spina bifida and anencephaly. 10 years after the U.S. Public Health Service recommendation. MMWR Recomm Rep. 2002;51(RR-13):1–3.
- Centers for Disease Control and Prevention (CDC). Folate status in women of childbearing age, by race/ethnicity – United States, 1999–2000. MMWR Morb Mortal Wkly Rep. 2002;51(36):808–810.
- Bestwick JP, Huttly WJ, Morris JK, et al. Prevention of neural tube defects: a cross-sectional study of the uptake of folic acid supplementation in nearly half a million women. PLOS One. 2014;9(2):e89354.
- Yang Q, Cogswell ME, Hamner HC, et al. Folic acid source, usual intake, and folate and vitamin B-12 status in US adults: National Health and Nutrition Examination Survey (NHANES) 2003–2006. Am J Clin Nutr. 2010;91(1):64–72.
- Simpson JL, Bailey LB, Pietrzik K, et al. Micronutrients and women of reproductive potential: required dietary intake and consequences of dietary deficiency or excess. Part I – folate, vitamin B12, vitamin B6. J Matern Fetal Neonatal Med. 2010;23(12):1323–1343.
- Obeid R, Kasoha M, Kirsch SH, et al. Concentrations of unmetabolized folic acid and primary folate forms in pregnant women at delivery and in umbilical cord blood. Am J Clin Nutr. 2010;92(6):1416–1422.
- Jacquemyn Y, Ajaji M, Karepouan N, et al. Vitamin B12 and folic acid status of term pregnant women and newborns in the Antwerp region, Belgium. Clin Exp Obstet Gynecol. 2014;41(2):141–143.
- Weber D, Stuetz W, Bernhard W, et al. 5-Methyltetrahydrofolate and thiamine diphosphate in cord-blood erythrocytes of preterm versus term newborns. Eur J Clin Nutr. 2013;67(10):1029–1035.
- Haandel L, Becker ML, Williams TD, et al. Comprehensive quantitative measurement of folate polyglutamates in human erythrocytes by ion pairing ultra-performance liquid chromatography/tandem mass spectrometry. Rapid Commun Mass Spectrom. 2012;26(14):1617–1630.
- Prasodjo A, Pfeiffer CM, Fazili Z, et al. Serum cotinine and whole blood folate concentrations in pregnancy. Ann Epidemiol. 2014;24(7):498–503.e1.
- Bodnar LM, Himes KP, Venkataramanan R, et al. Maternal serum folate species in early pregnancy and risk of preterm birth. Am J Clin Nutr. 2010;92(4):864–871.
- Dunstan JA, West C, McCarthy S, et al. The relationship between maternal folate status in pregnancy, cord blood folate levels, and allergic outcomes in early childhood. Allergy. 2012;67(1):50–57.
- Stark KD, Pawlosky RJ, Sokol RJ, et al. Maternal smoking is associated with decreased 5-methyltetrahydrofolate in cord plasma. Am J Clin Nutr. 2007;85(3):796–802.
- Molloy AM, Mills JL, Cox C, et al. Choline and homocysteine interrelations in umbilical cord and maternal plasma at delivery. Am J Clin Nutr. 2005;82(4):836–842.
- Guerra-Shinohara EM, Paiva AA, Rondo PH, et al. Relationship between total homocysteine and folate levels in pregnant women and their newborn babies according to maternal serum levels of vitamin B12. BJOG. 2002;109(7):784–791.
- Carter MF, Powell TL, Li C, et al. Fetal serum folate concentrations and placental folate transport in obese women. Am J Obstet Gynecol. 2011;205(1):83.e17–83.e25.
- Baek EJ, Nam HW. Changes in enzyme activity and expression of DHFR of Toxoplasma gondii by antifolates. Korean J Parasitol. 1998;36(3):191–198.
- Prasoona KR, Sunitha T, Srinadh B, et al. Maternal association and influence of DHFR 19 bp deletion variant predisposes foetus to anencephaly susceptibility: a family-based triad study. Biomarkers. 2018;23(7):640–646.
- Sun S, Gui Y, Jiang Q, et al. Dihydrofolate reductase is required for the development of heart and outflow tract in zebrafish. Acta Biochim Biophys Sin (Shanghai). 2011;43(12):957–969.
- Lamers Y, Prinz-Langenohl R, Moser R, et al. Supplementation with [6S]-5-methyltetrahydrofolate or folic acid equally reduces plasma total homocysteine concentrations in healthy women. Am J Clin Nutr. 2004;79(3):473–478.
- Obeid R, Holzgreve W, Pietrzik K. Is 5-methyltetrahydrofolate an alternative to folic acid for the prevention of neural tube defects? J Perinat Med. 2013;41(5):469–483.
- Williams LJ, Rasmussen SA, Flores A, et al. Decline in the prevalence of spina bifida and anencephaly by race/ethnicity: 1995–2002. Pediatrics. 2005;116(3):580–586.
- Dhobale M, Chavan P, Kulkarni A, et al. Reduced folate, increased vitamin B(12) and homocysteine concentrations in women delivering preterm. Ann Nutr Metab. 2012;61(1):7–14.
- Olapeju B, Saifuddin A, Wang G, et al. Maternal postpartum plasma folate status and preterm birth in a high-risk US population. Public Health Nutr. 2019;22(7):1281–1291.
- Papadopoulou E, Stratakis N, Roumeliotaki T, et al. The effect of high doses of folic acid and iron supplementation in early-to-mid pregnancy on prematurity and fetal growth retardation: the mother–child cohort study in Crete, Greece (rhea study). Eur J Nutr. 2013;52(1):327–336.
- Kim MW, Ahn KH, Ryu KJ, et al. Preventive effects of folic acid supplementation on adverse maternal and fetal outcomes. PLoS ONE. 2014;9(5):e97273.
- Wen SW, Chen XK, Rodger M, et al. Folic acid supplementation in early second trimester and the risk of preeclampsia. Am J Obstet Gynecol. 2008;198(1):45.e1–45.e7.
- Jones P, Lucock M, Veysey M, et al. The vitamin D-folate hypothesis as an evolutionary model for skin pigmentation. Nutrients. 2018;10(5):554.
- Sweeney MR, McPartlin J, Weir DG, et al. Evidence of unmetabolised folic acid in cord blood of newborn and serum of 4-day-old infants. Br J Nutr. 2005;94(5):727–730.
- Krikke GG, Grooten IJ, Vrijkotte TG, et al. Vitamin B12 and folate status in early pregnancy and cardiometabolic risk factors in the offspring at age 5–6 years: findings from the ABCD multi-ethnic birth cohort. BJOG. 2016;123(3):384–392.
- Da Silva VR, Hausman DB, Kauwell GP, et al. Obesity affects short-term folate pharmacokinetics in women of childbearing age. Int J Obes. 2013;37(12):1608–1610.
- Stern SJ, Matok I, Kapur B, et al. A comparison of folic acid pharmacokinetics in obese and nonobese women of childbearing age. Ther Drug Monit. 2011;33(3):336–340.
- O’Malley EG, Reynolds CME, Cawley S, et al. Folate and vitamin B12 levels in early pregnancy and maternal obesity. Eur J Obstet Gynecol Reprod Biol. 2018;231:80–84.
- Tinker SC, Hamner HC, Berry RJ, et al. Does obesity modify the association of supplemental folic acid with folate status among nonpregnant women of childbearing age in the United States? Birth Defects Res A Clin Mol Teratol. 2012;94(10):749–755.