Abstract
Objective
Intra-amniotic inflammation (IAI), associated with either microbe (infection) or danger signals (sterile), plays a major role in the pathophysiology of preterm labor and delivery. Coiled-Coil-Helix-Coiled-Coil-Helix Domain Containing 2 (CHCHD2) [also known as Mitochondrial Nuclear Retrograde Regulator 1 (MNRR1)], a mitochondrial protein involved in oxidative phosphorylation and cell survival, is capable of sensing tissue hypoxia and inflammatory signaling. The ability to maintain an appropriate energy balance at the cellular level while adapting to environmental stress is essential for the survival of an organism. Mitochondrial dysfunction has been observed in acute systemic inflammatory conditions, such as sepsis, and is proposed to be involved in sepsis-induced multi-organ failure. The purpose of this study was to determine the amniotic fluid concentrations of CHCHD2/MNRR1 in pregnant women, women at term in labor, and those in preterm labor (PTL) with and without IAI.
Methods
This cross-sectional study comprised patients allocated to the following groups: (1) mid-trimester (n = 16); (2) term in labor (n = 37); (3) term not in labor (n = 22); (4) PTL without IAI who delivered at term (n = 25); (5) PTL without IAI who delivered preterm (n = 47); and (6) PTL with IAI who delivered preterm (n = 53). Diagnosis of IAI (amniotic fluid interleukin-6 concentration ≥2.6 ng/mL) included cases associated with microbial invasion of the amniotic cavity and those of sterile nature (absence of detectable bacteria, using culture and molecular microbiology techniques). Amniotic fluid and maternal plasma CHCHD2/MNRR1 concentrations were determined with a validated and sensitive immunoassay.
Results
(1) CHCHD2/MNRR1 was detectable in all amniotic fluid samples and women at term without labor had a higher amniotic fluid CHCHD2/MNRR1 concentration than those in the mid-trimester (p = 0.003); (2) the amniotic fluid concentration of CHCHD2/MNRR1 in women at term in labor was higher than that in women at term without labor (p = 0.01); (3) women with PTL and IAI had a higher amniotic fluid CHCHD2/MNRR1 concentration than those without IAI, either with preterm (p < 0.001) or term delivery (p = 0.01); (4) women with microbial-associated IAI had a higher amniotic fluid CHCHD2/MNRR1 concentration than those with sterile IAI (p < 0.001); (5) among women with PTL and IAI, the amniotic fluid concentration of CHCHD2/MNRR1 correlated with that of interleukin-6 (Spearman’s Rho = 0.7; p < 0.001); and (6) no correlation was observed between amniotic fluid and maternal plasma CHCHD2/MNRR1 concentrations among women with PTL.
Conclusion
CHCHD2/MNRR1 is a physiological constituent of human amniotic fluid in normal pregnancy, and the amniotic concentration of this mitochondrial protein increases during pregnancy, labor at term, and preterm labor with intra-amniotic infection. Hence, CHCHD2/MNRR1 may be released into the amniotic cavity by dysfunctional mitochondria during microbial-associated IAI.
Introduction
Preterm birth, a leading cause of neonatal morbidity and mortality [Citation1–5], represents a significant economic burden on society, as approximately $26.2 billion is spent in the United States annually on the care of premature infants [Citation6]. Intra-amniotic inflammation (IAI) associated with microbial invasion of the amniotic cavity has been causally linked to spontaneous preterm delivery [Citation7–12]. Furthermore, a subset of pregnant women diagnosed with IAI does not have identifiable microorganisms in the amniotic fluid [Citation13–17], and this condition, also known as sterile IAI, is more common than microbial-associated IAI among patients who delivered preterm [Citation15,Citation17]. Understanding the pathophysiology of these inflammatory conditions is key to improving preventive strategies and patient management.
Coiled-Coil-Helix-Coiled-Coil-Helix Domain-Containing Protein 2 (CHCHD2), also known as Mitochondrial Nuclear Retrograde Regulator 1 (MNRR1), is a recently characterized bi-organellar protein encoded by the homonymous gene [Citation18,Citation19], localized primarily to the mitochondria and, to a lesser extent, in the cell nucleus [Citation20]. Within mitochondria, this protein is involved in oxidative phosphorylation and adenosine triphosphate (ATP) production [Citation21–23], whereas, in the nucleus, it is a transcription factor for genes implicated in the cellular response to tissue hypoxia [Citation20,Citation22]. Moreover, CHCHD2/MNRR1 is required for optimal induction of cellular stress-responsive signaling pathways, such as the mitochondrial unfolded protein response [Citation24], and it can inhibit apoptosis through Bax activation [Citation25,Citation26]. Therefore, this bi-organellar regulator of the mitochondria and the nucleus is important for cellular function, stress response, and survival.
The ability to maintain an appropriate energy balance at the cellular level while adapting to environmental stress is essential for the survival of an organism [Citation27–29]. Mitochondrial dysfunction has been observed in acute systemic inflammatory conditions, such as sepsis [Citation30–33], and is proposed to be involved in sepsis-induced multi-organ failure [Citation31,Citation34,Citation35]. Reduction of the cellular CHCHD2/MNRR1 protein, as part of mitochondrial dysfunction, has recently been shown to play a role in the amplification of inflammatory cytokines in a murine model of lipopolysaccharide (LPS)-induced systemic inflammation leading to preterm birth [Citation36]. However, whether this protein is involved in the physiological or pathological inflammatory processes of human pregnancy has never been examined.
The aim of this study was to determine whether CHCHD2/MNRR1 can be detected in the amniotic fluid of normal pregnant women and whether the concentration of CHCHD2/MNRR1 changes during pregnancy, parturition, or with IAI. The IAI group included cases associated with microbes and those of sterile nature. We also determined maternal plasma concentrations of CHCHD2/MNRR1 in women with preterm labor to evaluate whether there is a correlation between the concentrations of maternal plasma and of amniotic fluid in this protein.
Materials and methods
Study design and population
A cross-sectional study was conducted by searching our clinical database and bank of biological samples to identify 200 women allocated to the following groups: (1) women with an uncomplicated pregnancy who underwent amniocentesis in the mid-trimester for genetic indications and delivered at term (n = 16); (2) women with an uncomplicated pregnancy at term with (n = 37) and without spontaneous labor (n = 22); (3) women with a pregnancy complicated by an episode of spontaneous preterm labor (PTL) with intact membranes who were further classified as (a) PTL without IAI who delivered at term (n = 25); (b) PTL without IAI who delivered preterm (n = 47); and (c) PTL with IAI who delivered preterm (n = 53) ().
Amniocentesis was performed for the following indications: genetic indication, assessment of fetal lung maturity, or evaluation of microbial invasion of the amniotic cavity. The group of women with PTL and IAI included cases of either microbial-associated or sterile IAI. Patients with a multiple pregnancy, preeclampsia, maternal medical disease, fetal death, and fetal congenital or chromosomal abnormalities were excluded from the study. Each patient provided written informed consent upon enrollment and prior to the collection of samples. The Institutional Review Boards of Wayne State University and the Eunice Kennedy Shriver National Institute of Child Health and Human Development (NICHD) approved the collection of samples and the use of biological specimens and clinical data for research purposes.
Clinical definitions
Spontaneous PTL was defined by the presence of at least two regular uterine contractions every 10 min with documented cervical changes between 20 and 36 6/7 weeks of gestation and by the requirement of hospitalization [Citation17,Citation37]. Amniocentesis was performed to assess the presence of microbial invasion of the amniotic cavity. Preterm delivery was defined as birth occurring prior to the 37th week of gestation. The management of PTL in our hospital at the time of admission included corticosteroid (betamethasone or dexamethasone) administration between 24 and 34 weeks of gestation. Betamethasone was given intramuscularly in two doses (12 mg), 24 h apart, and dexamethasone was administered intramuscularly in 4 doses (6 mg), 12 h apart.
Women at term (≥37 weeks of gestation) without labor underwent amniocentesis to assess fetal lung maturity prior to a delivery by cesarean section. Women at term in labor were those who presented in labor with uncertain gestational age and who underwent amniocentesis for the assessment of fetal lung maturity and of microbial invasion of the amniotic cavity. Retrospectively, these patients were considered to be at term given the following characteristics: (1) spontaneous labor; (2) delivery within 48 h of amniocentesis; (3) analysis of amniotic fluid consistent with fetal lung maturity; (4) birthweight ≥2500 grams; (5) absence of respiratory distress syndrome or other complications of prematurity; and (6) physical examination by a pediatrician consistent with that of a term neonate. IAI was defined by an amniotic fluid interleukin (IL)-6 concentration ≥ 2.6 ng/mL [Citation38]. Women with IAI were classified as having either microbial-associated or sterile IAI according to the presence or absence of microbial invasion of the amniotic cavity. The presence of micro-organisms was assessed by conventional cultures for bacteria and by the polymerase chain reaction technique coupled with electrospray ionization mass spectrometry ([PCR/ESI-MS]; Ibis® Technology, Athogen, Carlsbad, CA, USA) in amniotic fluid samples, as previously described [Citation17,Citation39]. Sterile IAI was defined by an IAI without evidence of microbial invasion of the amniotic cavity by culture and by PCR/ESI-MS. Amniotic fluid samples of women at term were assessed by conventional cultures for bacteria and without IL-6 results. Only women at term without evidence of microbial invasion of the amniotic cavity were included in this study.
Amniotic fluid and maternal peripheral blood collection
Amniotic fluid samples were obtained by transabdominal amniocentesis under ultrasonographic guidance and cultured for the presence of microorganisms (aerobic and anaerobic bacteria and genital Mycoplasmas). A white blood cell count [Citation40], a glucose concentration [Citation41,Citation42], and a Gram stain [Citation43] were also performed. The results of these tests were used to guide clinical management. Amniotic fluid samples not required for clinical assessment were centrifuged at 1300 g for 10 min at 4 °C, and the supernatant was aliquoted and stored at −80 °C until analysis.
Maternal blood samples, obtained by venipuncture in patients with an episode of preterm labor, were collected in tubes containing ethylenediaminetetraacetic acid. Samples were centrifuged shortly after collection and stored at −80 °C until analysis. Only samples of maternal blood collected within 48 h of amniocentesis were considered for analysis.
Determination of human CHCHD2/MNRR1 concentrations in amniotic fluid and maternal plasma
Concentrations of amniotic fluid CHCHD2/MNRR1 and maternal plasma CHCHD2/MNRR1 were determined by using a commercially available immunoassay (Human CHCHD2 ELISA Kit, Abbexa LTD, Cambridge, UK). Briefly, 100 μL of maternal plasma, amniotic fluid, or calibrator were dispensed into separate wells of the assay plates and incubated for 90 min at 37 °C. After the removal of the remaining sample and calibrator, the plates were washed twice with 1X wash buffer and 100 μL of detection reagent. A working solution was added to each well. Plates were then incubated for 60 min at 37 °C. Next, the plates were washed three times with 1X wash buffer; 100 μL of detection reagent B working solution were added to each well; and plates were then incubated for 30 min at 37 °C. Subsequently, the plates were washed five times with 1X wash buffer, and 90 μL of TMB substrate were added to each well. Plates were then mixed thoroughly and incubated for 20 min at 37 °C. Finally, 50 μL of stop solution were added to each well to halt the reaction. The SpectraMax iD5 (Molecular Devices, San Jose, CA, USA) was utilized to read the plates, and CHCHD2/MNRR1 concentrations were calculated with SoftMax Pro 7 (Molecular Devices). The inter- and intra-assay coefficients of variation were 8.3% and 7.1%, respectively, with an assay sensitivity of 12.3 pg/mL.
Statistical Analysis
The Kolmogorov-Smirnov test and visual plot inspection were used to assess the normality of data distribution. Demographic categorical data were summarized as proportions, whereas continuous variables were summarized as medians and interquartile ranges (IQR). Differences in proportions were assessed with a Chi-square or a Fisher’s exact test, while continuous data were compared between two groups with the Mann–Whitney U-test or an independent t-test. Data were compared among groups by using the Kruskal–Wallis test with post-hoc Mann–Whitney U-tests or Analysis of Variance (ANOVA) with post-hoc t-tests, depending on data distribution. Generalized linear models were utilized to assess differences in biomarkers while adjusting for potential confounders (i.e. gestational age at amniocentesis). Data were log (base 2) transformed prior to analysis. A receiver operating characteristic (ROC) curve was generated among all patients with PTL to examine the diagnostic performance of CHCHD2/MNRR1 for the identification of patients with microbial-associated IAI. Statistical analyses were performed with R statistical language version 4.1.2 and IBM SPSS version 19.0 (IBM Corporation., Armonk, NY, USA).
Results
Concentration of CHCHD2/MNRR1 in the amniotic fluid of women with normal pregnancy
Clinical characteristics of women in the mid-trimester and term-no labor groups are presented in . The median gestational age (IQR) at amniocentesis was 16.1 weeks (16.0–16.9) and 38.7 weeks (38.0–40.0), respectively. CHCHD2/MNRR1 was detectable in all samples. Women at term not in labor showed a higher amniotic fluid CHCHD2/MNRR1 concentration than women in the mid-trimester group [5055 pg/mL (3780–6101) vs 3473 pg/mL (2786-4124); p = 0.003, 1.4-fold change (FC)] as shown in .
Figure 2. Concentration of CHCHD2/MNRR1 (pg/mL) in the amniotic fluid of pregnant women in the mid-trimester, at term without labor and at term with labor. Data are reported as medians and interquartile ranges. The analysis was performed after log2 transformation of the data.
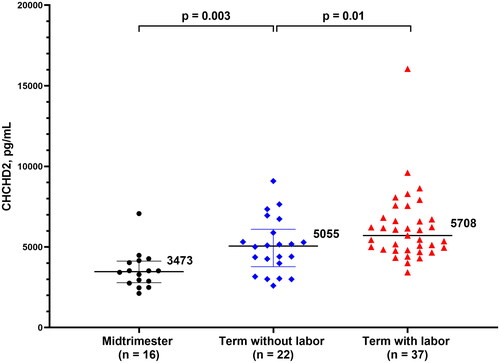
Table 1. Clinical characteristics of women in the mid-trimester of pregnancy and at term with and without labor.
Concentration of CHCHD2/MNRR1 in the amniotic fluid of women at term with and without labor
There were no significant differences in maternal age, gestational age at amniocentesis and at delivery, duration of sample storage, birthweight, and frequency of nulliparity between the two groups (shown in ). CHCHD2/MNRR1 concentration in the amniotic fluid of women at term in labor was significantly higher than that of women at term not in labor [5708 pg/mL (4822–6764) vs 5055 pg/mL (3780–6101); p = 0.01, FC = 1.23] as shown in .
Concentration of CHCHD2/MNRR1 in the amniotic fluid of women with preterm labor, classified by gestational age at delivery and the presence of intra-amniotic inflammation
displays the clinical characteristics of women with preterm labor according to the time of delivery and the presence of IAI. Women in the preterm labor and IAI group had the lowest median gestational age at amniocentesis among the three preterm labor groups (shown in ). After adjustment for gestational age at amniocentesis, women with IAI and preterm delivery showed a significantly higher amniotic fluid CHCHD2/MNRR1 concentration than those with preterm labor without IAI who delivered preterm or at term [5535 pg/mL (4703–8730) vs 5285 pg/mL (4402–5855), p < 0.001, FC = 1.31; and 5535 pg/mL (4703–8730) vs 5595 pg/mL (4893–6631), p = 0.012, FC = 1.23, respectively]. No significant difference in amniotic fluid CHCHD2/MNRR1 concentration was observed between women with preterm labor without IAI who delivered preterm and those who delivered at term (p = 0.4) as shown in . The clinical characteristics of women with sterile (n = 37) and microbial-associated (n = 16) IAI are displayed in . Women with microbial-associated IAI had a higher amniotic fluid CHCHD2/MNRR1 concentration than those with sterile IAI [9829 pg/mL (7749–11818) vs 5296 pg/mL (4583–6236), p < 0.001] as shown in . However, no significant difference was found in the amniotic fluid CHCHD2/MNRR1 concentrations between women with sterile IAI and those without IAI who delivered either preterm or term after adjustment for gestational age at amniocentesis (p > 0.05). There was a significant correlation between IL-6 and CHCHD2/MNRR1 concentrations in the amniotic fluid of women with preterm labor (n = 125, Spearman’s Rho =0.3; p < 0.001). The strength of this correlation was higher among women with IAI (n = 53, Spearman’s Rho = 0.7; p < 0.001) as shown in Supplementary Figure 1. An elevated amniotic fluid CHCHD2/MNRR1 concentration ≥7632 pg/mL identified women with microbial-associated IAI with a sensitivity of 81% and a specificity of 90% (area under the ROC curve 0.90; 95% confidence interval, 0.80–0.98; p < 0.001) as shown in Supplementary Figure 2.
Figure 3. Concentration of CHCHD2/MNRR1 (pg/mL) in the amniotic fluid of pregnant women in preterm labor classified by gestational age at delivery and the presence of intra-amniotic inflammation. Data are reported as medians and interquartile ranges. The analysis was performed after log2 transformation of the data.
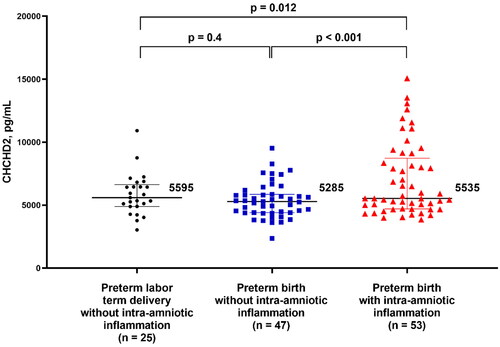
Figure 4. Concentration of CHCHD2/MNRR1 (pg/mL) in the amniotic fluid of pregnant women in preterm labor with intra-amniotic inflammation classified by the presence or absence of microbial invasion of the amniotic cavity. Data are reported as medians and interquartile ranges. The analysis was performed after log2 transformation of the data.
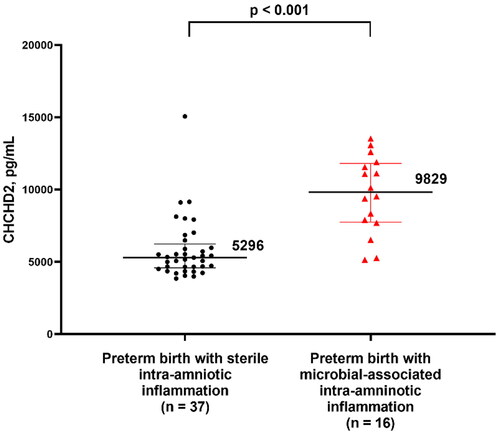
Table 2. Clinical characteristics of women with preterm labor according to the gestational age at delivery (preterm and term) and to the presence of intra-amniotic inflammation.
Table 3. Clinical characteristics of women with preterm birth and intra-amniotic inflammation in the presence or absence of microbial invasion of the amniotic cavity.
Concentration of CHCHD2/MNRR1 in the maternal plasma of women with preterm labor
Among women with preterm labor, CHCHD2/MNRR1 concentration was also evaluated in the maternal plasma of those whose blood sample was collected within 48 h of amniocentesis (shown in Supplementary Table 1). There were no significant differences in maternal plasma CHCHD2/MNRR1 concentrations among the three subgroups (p = 0.3) as shown in Supplementary Figure 3. Moreover, no correlation was observed between the concentrations of CHCHD2/MNRR1 in maternal plasma and in amniotic fluid (n = 98, Spearman’s Rho =0.1; p = 0.2).
Discussion
Principal findings of the study
The current study demonstrates that (1) CHCHD2/MNRR1 is a physiological constituent of human amniotic fluid in normal pregnancy; (2) amniotic fluid concentration of CHCHD2/MNRR1 increases as pregnancy progresses; (3) spontaneous labor at term is a physiological inflammatory state associated with an increase in amniotic fluid CHCHD2/MNRR1 concentration; (4) among women with PTL, those with IAI show a significantly higher concentration of CHCHD2/MNRR1 than those without this condition, and this difference is mainly attributable to women with microbial invasion of the amniotic cavity; (5) a positive correlation exists between CHCHD2/MNRR1 and IL-6 concentrations in the amniotic fluid of women with PTL; and (6) there is no significant correlation between amniotic fluid and maternal plasma CHCHD2/MNRR1 concentrations in women with preterm labor.
What is CHCHD2/MNRR1?
CHCHD2/MNRR1, a recently characterized bi-organellar protein encoded by the homonymous gene [Citation18,Citation19], is a member of the coiled-coil-helix-coiled-coil-helix (CHCH) domain-containing protein family, a group of evolutionarily conserved proteins [Citation44,Citation45] found in the mitochondrial intermembrane space and now recognized as cellular factors regulating respiration, redox equilibrium, lipid homeostasis, and membrane dynamics, among others. Furthermore, a growing body of evidence suggests the involvement of several of these protein family members in human disease [Citation46].
The CHCHD2/MNRR1 gene was first reported in a study designed to identify new genes that affect oxidative phosphorylation [Citation18,Citation19]. Suppression of CHCHD2/MNRR1 cell expression led to a 50% reduction in cellular oxygen consumption and a 2-fold increase in reactive oxygen species (ROS) levels [Citation22]. As a transcription regulator in the nucleus, CHCHD2/MNRR1 acts by binding and activating a so-called oxygen-responsive element (ORE), a conserved 13-bp DNA sequence identified in the promoter regions of COX4I2 and of CHCHD2/MNRR1 itself. An in vitro study showed that ORE-stimulated transcription is maximally induced by CHCHD2/MNRR1 under moderate tissue hypoxia (4% oxygen), suggesting that, under moderate hypoxia, it promotes a transcriptional program that achieves its normal homeostatic state, similar to the function of hypoxia-inducible factors under more severe hypoxic conditions [Citation22]. The ORE has recently been found in other genes apart from COX4I2 and CHCHD2/MNRR1, some of which are implicated in functions such as cell migration and adhesion, e.g. Mucosal Vascular Addressin Cell Adhesion Molecule 1 (MADCAM1) and cadherin4 [23]. Furthermore, CHCHD2/MNRR1 has a survival value, as this protein is capable of inhibiting apoptosis by binding to the anti-apoptotic protein Bcl-xL and by preventing the accumulation of the pro-apoptotic protein Bax in the mitochondria [Citation25]. Lastly, CHCHD2/MNRR1 is required for optimal induction of cellular stress-responsive signaling pathways, such as the mitochondrial unfolded protein response [Citation24]. The pleiotropic role of CHCHD2/MNRR1 in physiological and pathological inflammatory processes makes this protein a candidate for studies investigating normal pregnancy and its complications.
Concentration of CHCHD2/MNRR1 in the amniotic fluid increases as gestation progresses
CHCHD2/MNRR1 was detectable in all amniotic fluid samples obtained from normal pregnant women examined in the current study, indicating that this protein represents a physiological constituent of the amniotic cavity. In addition, amniotic fluid CHCHD2/MNRR1 concentration was higher in women at term than in mid-trimester, suggesting that its concentration increases as gestation progresses. Throughout pregnancy, amniotic fluid is in direct contact with fetal organs (including mucosal surfaces of the gastrointestinal and respiratory tracts) and the chorioamniotic membranes [Citation47–49] where water and solute exchanges take place [Citation50–52]. Different cells could serve as potential sources for amniotic fluid components. Indeed, CHCHD2/MNRR1 is expressed in several cell types such as neurons [Citation53,Citation54], hepatocytes [Citation55], and lung cells [Citation56]. Additionally, previous analyses of the transcriptome of different reproductive tissues found CHCHD2/MNRR1 gene expression in the chorioamniotic membranes [Citation57], myometrium [Citation58], and cervix of normal pregnant women [Citation59] as well as in the umbilical cord blood of preterm neonates [Citation60]. Changes in the protein and metabolite components of human amniotic fluid throughout gestation were reported in several studies [Citation61–63]. A recent proteomic study of amniotic fluid from normal pregnant women showed that, among the 320 proteins that significantly changed in abundance in term pregnancy compared to mid-gestation, about 48% increased in abundance with advancing gestational age and were implicated in antimicrobial, developmental, and inflammatory functions [Citation63]. Furthermore, Gene Ontology analysis of such upregulated proteins identified terms related to immune effector processes involved in defense against invading microbes [Citation63]. However, the significance of the increased amniotic fluid CHCHD2/MNRR1 concentration as pregnancy progresses as well as the source and mechanisms of CHCHD2/MNRR1 release in the amniotic fluid remains to be determined.
Women with intra-amniotic inflammation have a higher amniotic fluid CHCHD2/MNRR1 concentration than those without intra-amniotic inflammation
Among the processes implicated in the activation of the common pathway of preterm labor [Citation64], inflammation of the amniotic cavity, or IAI, is the most well established [Citation64–68]. Indeed, microbial-associated IAI [Citation7,Citation68–71] and sterile IAI [Citation38] are associated with early preterm delivery, adverse neonatal outcome, and acute inflammatory lesions of the placenta [Citation15,Citation71–88]. Our findings, 1) that the concentration of amniotic fluid CHCHD2/MNRR1 was significantly higher in women with preterm labor with IAI than in women without IAI, and 2) that amniotic fluid CHCHD2/MNRR1 concentration correlated with amniotic fluid IL-6 concentration, led us to hypothesize that CHCHD2/MNRR1 is a part of host response against intra-amniotic infection. Among women with IAI, those with microbial-associated IAI showed a higher amniotic fluid CHCHD2/MNRR1 concentration than those with sterile IAI, which is consistent with the previous observation of significantly higher IL-6 concentrations in the amniotic fluid of the former group compared to the latter [Citation17,Citation37,Citation89]. However, only pregnant women with microbial-associated IAI, but not those with sterile IAI, had a significantly higher amniotic fluid CHCHD2/MNRR1 concentration than women without IAI who delivered term or preterm, after adjustment for gestational age at amniocentesis. Thus, the difference in amniotic fluid CHCHD2/MNRR1 concentrations between women in PTL with and without IAI is mainly attributable to the group of women with proven intra-amniotic infection.
Lastly, we found that an amniotic fluid CHCHD2 concentration ≥7632 pg/ml can identify women with microbial-associated IAI among all women who presented with PTL, with a sensitivity of 81% and a specificity of 90%. This finding might be useful for targeted antimicrobial therapy.
The effect of microbial-associated IAI on CHCHD2/MNRR1 release during pregnancy was recently examined with the use of trophoblast cell cultures exposed to LPS and a murine model of preterm birth induced by the intra-peritoneal administration of LPS. LPS exposure resulted in reduced levels of CHCHD2/MNRR1 in trophoblast cells due to the stabilization of YME1L1, a protease implicated in post-translational degradation of CHCHD2/MNRR1 [Citation36]. Additionally, decreased levels of CHCHD2/MNRR1 were associated with increased mitochondrial ROS production and with the transcription of TNF-⍺ that was independent of the Toll-Like Receptor 4 signaling pathway [Citation36]. Our finding that the amniotic fluid CHCHD2/MNRR1 concentration was increased in women with IAI seems to contradict the observed decrease of CHCHD2/MNRR1 in trophoblast cells. However, the reduced level of CHCHD2/MNRR1 in trophoblast cells could be due, in part, to the heightened extracellular release of this protein; indeed, murine macrophages treated with LPS release CHCHD2/MNRR1 in the supernatant (Purandare N, Grossman LI, Aras S, et al. unpublished data). Thus, invading bacteria may induce the extracellular release of CHCHD2/MNRR1 from either amniotic fluid leukocytes [Citation90–94] or from the chorioamniotic membranes of women with IAI. Alternatively, this intracellular bi-organellar protein could be indirectly released into the amniotic cavity as a result of cell death caused by IAI, especially if infection is present. Future investigations of the chorioamniotic membranes, which are in direct contact with amniotic fluid, may help to examine these hypotheses.
Plasma concentration of CHCHD2/MNRR1 in maternal blood of women with preterm labor
We found no correlation between concentrations of amniotic fluid CHCHD2/MNRR1 and maternal plasma CHCHD2/MNRR1 in women with preterm labor. This observation is consistent with the asymptomatic or the subclinical nature of IAI in the majority of women [Citation67, Citation95–98], with only a small proportion developing systemic signs and symptoms of maternal infection (i.e. clinical chorioamnionitis) [Citation99,Citation100]. Thus, the increased concentration of CHCHD2/MNRR1 in the amniotic cavity may reflect the localized nature of the intra-amniotic inflammatory response. Amniotic fluid represents a unique source of information about processes localized to the uterus. However, techniques for amniotic fluid collection are invasive whether performed transabdominally or transcervically by needle amniotomy as recently described [Citation101]. Devices have been designed for non-invasive transcervical collection of amniotic fluid in women with ruptured membranes [Citation102]; however, noninvasive techniques are not available to retrieve amniotic fluid from women with intact membranes. We evaluated CHCHD2 in maternal plasma, but the results were disappointing. Future studies of this mitochondrial protein in the vaginal fluid as a noninvasive biomarker of intra-amniotic infection/inflammation may be possible.
Labor at term is associated with a higher concentration of CHCHD2/MNRR1 in amniotic fluid
The activation of the common pathway of spontaneous labor at term [Citation65,Citation103,Citation104] is a coordinated physiological inflammatory process [Citation79,Citation105–111]. Multiple studies have shown that an increase in cellular and soluble inflammatory mediators occurs in the uterine tissues [Citation112–120], decidua [Citation107,Citation114,Citation115,Citation121–125], chorioamniotic membranes [Citation114,Citation115,Citation121,Citation122,Citation126], cervix [Citation59,Citation105,Citation113,Citation116,Citation121,Citation127], and amniotic fluid [Citation128–134] during normal parturition at term. Furthermore, several cytokines, including TNF-α [Citation132,Citation133], are higher in the amniotic fluid of women at term in labor compared to those not in labor [Citation128–133]. The localized inflammatory state may be responsible for the increased release of mitochondrial protein CHCHD2/MNRR1 from the gestational tissues or immune cells that are in direct contact with the amniotic fluid of women at term in labor [Citation135]. The changes in amniotic CHCHD2/MNRR1 concentration in women at term in labor may be due to enhanced protein release rather than to increased gene expression in the myometrium [Citation58] and chorioamniotic membranes [Citation136], as transcriptomic analysis did not show differences in CHCHD2 expression in those tissues from women at term in labor compared to those not in labor. Elevated amniotic fluid CHCHD2/MNRR1 concentration may therefore reflect the physiological mild and localized inflammatory state that characterizes spontaneous labor at term. However, it is difficult to reconcile why the amniotic fluid CHCHD2/MNRR1 concentration was higher in women with spontaneous labor at term but not in those with PTL and sterile IAI, compared to controls without IAI.
Strengths and limitations
This is the first study to report on the amniotic fluid concentration of CHCHD2/MNRR1 in pregnant women with an uncomplicated pregnancy, in women at term in labor, and in those with preterm labor with or without IAI. In women with PTL, the diagnosis of microbial invasion of the amniotic cavity was made by real-time PCR/ESI-MS. We also examined whether there was a correlation between CHCHD2/MNRR1 concentration in the amniotic fluid and in the maternal plasma of women with preterm labor. However, the cross-sectional nature of this study does not allow evaluation of the temporal relationship between the changes in CHCHD2/MNRR1 concentration and the clinical conditions examined.
Conclusions
We report herein for the first time on the presence of the mitochondrial protein, CHCHD2/MNRR1, in the amniotic fluid of human pregnancy. The amniotic fluid concentration of this protein increases as gestation progresses, in women at term in labor, and in the presence of IAI. It is possible that CHCHD2/MNRR1 represents a mitochondrial protein released by dysfunctional mitochondria during microbial-associated IAI. Further studies are needed to better understand the mechanisms of CHCHD2/MNRR1 release and the clinical significance of this protein in the context of obstetrical disease.
Author contributions
Conceptualization, methodology, validation: Mariachiara Bosco, Tinnakorn Chaiworapongsa, Roberto Romero, Lawrence Grossman, and Siddhesh Aras.
Data curation, writing, original draft preparation: Mariachiara Bosco, Tinnakorn Chaiworapongsa, Nardhy Gomez-Lopez, and Adi Tarca.
Visualization, writing, review, and editing: Manaphat Suksai, Eunjung Jung, Arun Meyyazhagan, Malek Al Qasem, Marcia Arenas-Hernandez, Francesca Gotsch, Dahiana Gallo, Lawrence Grossman, Siddhesh Aras, Piya Chaemsaithong, and Roberto Romero.
Formal analysis: Mariachiara Bosco, Tinnakorn Chaiworapongsa, Adi Tarca.
Resources: Tinnakorn Chaiworapongsa, Roberto Romero, Nardhy Gomez-Lopez, Marcia Arenas-Hernandez.
Supervision: Roberto Romera, Tinnakorn Chaiworapongsa, Adi Tarca, Nardhy Gomez-Lopez, and Massimo Franchi.
Each author approved the final version of the manuscript prior to its submission to the Journal.
Ethical statement
This research complies with the guidelines for human studies and was conducted ethically in accordance with the World Medical Association Declaration of Helsinki. The study protocols (OH97-CH-N067, OH99-CH-N055, OH98-CH-N001, and OH99-CH-N056) were reviewed and approved by the Institutional Review Board of the Eunice Kennedy Shriver National Institute of Child Health and Human Development, National Institutes of Health, United States Department of Health and Human Services (NICHD/NIH/DHHS) and by the Human Investigation Committee of Wayne State University (IRB Nos. 110605MP2F, 082403MP2F(5R), 075299M1E(R)(RCR), and 103108MP2F RCR).
Patient consent
Written informed consent was obtained from the study participants prior to the collection of maternal amniotic fluid and plasma samples.
Supplemental Material
Download MS Word (15.6 KB)Supplemental Material
Download MS Word (15.6 KB)Supplemental Material
Download Zip (573.8 KB)Acknowledgements
The authors thank Maureen McGerty, M.A., (Wayne State University) for her critical reading of the manuscript and editorial support.
Disclosure statement
The authors report no potential conflicts of interest. The funders had no role in the design of the study, in the collection, analyses, or interpretation of data, in the writing of the manuscript, or in the decision to publish the results. Dr. Romero has contributed to this work as part of his official duties as an employee of the United States Federal Government.
Data availability Statement
All data generated or analyzed during this study are included in this article. Further inquiries can be directed to the corresponding author at [email protected] (Dr. Romero).
Additional information
Funding
References
- Liu L, Oza S, Hogan D, et al. Global, regional, and national causes of under-5 mortality in 2000-15: an updated systematic analysis with implications for the sustainable development goals. Lancet. 2016; 388(10063):3027–3035.
- Brown HK, Speechley KN, Macnab J, et al. Neonatal morbidity associated with late preterm and early term birth: the roles of gestational age and biological determinants of preterm birth. Int J Epidemiol. 2014;43(3):802–814.
- Chawanpaiboon S, Vogel JP, Moller AB, et al. Global, regional, and national estimates of levels of preterm birth in 2014: a systematic review and modelling analysis. Lancet Glob Health. 2019;7(1):e37–e46.
- Manuck TA, Sheng X, Yoder BA, et al. Correlation between initial neonatal and early childhood outcomes following preterm birth. Am J Obstet Gynecol. 2014;210(5):426.e1-9.
- Blencowe H, Cousens S, Oestergaard MZ, et al. National, regional, and worldwide estimates of preterm birth rates in the year 2010 with time trends since 1990 for selected countries: a systematic analysis and implications. Lancet. 2012;379(9832):2162–2172.
- Institute of Medicine Committee on Understanding Premature B, Assuring Healthy O. The national academies collection: reports funded by national institutes of health. In: Behrman RE, Butler AS, editors. Preterm birth: causes, consequences, and prevention. Washington (DC): National Academies; 2007.
- Romero R, Gomez R, Chaiworapongsa T, et al. The role of infection in preterm labour and delivery. Paediatr Perinat Epidemiol. 2001;15 Suppl 2:41–56.
- Bobitt JR, Ledger WJ. Unrecognized amnionitis and prematurity: a preliminary report. J Reprod Med. 1977;19(1):8–12.
- Miller JM, Jr., Pupkin MJ, Hill GB. Bacterial colonization of amniotic fluid from intact fetal membranes. Am J Obstet Gynecol. 1980;136(6):796–804.
- Bobitt JR, Hayslip CC, Damato JD. Amniotic fluid infection as determined by transabdominal amniocentesis in patients with intact membranes in premature labor. Am J Obstet Gynecol. 1981;140(8):947–952.
- Wallace RL, Herrick CN. Amniocentesis in the evaluation of premature labor. Obstet Gynecol. 1981;57(4):483–486.
- Wahbeh CJ, Hill GB, Eden RD, et al. Intra-amniotic bacterial colonization in premature labor. Am J Obstet Gynecol. 1984;148(6):739–743.
- DiGiulio DB, Romero R, Amogan HP, et al. Microbial prevalence, diversity and abundance in amniotic fluid during preterm labor: a molecular and culture-based investigation. PLOS One. 2008;3(8):e3056.
- DiGiulio DB, Romero R, Kusanovic JP, et al. Prevalence and diversity of microbes in the amniotic fluid, the fetal inflammatory response, and pregnancy outcome in women with preterm pre-labor rupture of membranes. Am J Reprod Immunol. 2010;64(1):38–57.
- Romero R, Miranda J, Chaemsaithong P, et al. Sterile and microbial-associated intra-amniotic inflammation in preterm prelabor rupture of membranes. J Matern -Fetal Neonatal Med. . 2015; Aug28(12):1394–1409.
- Romero R, Miranda J, Chaiworapongsa T, et al. Sterile intra-amniotic inflammation in asymptomatic patients with a sonographic short cervix: prevalence and clinical significance. J Matern -Fetal Neonatal Med. 2015;28(11):1343–1359.
- Romero R, Miranda J, Chaiworapongsa T, et al. Prevalence and clinical significance of sterile intra-amniotic inflammation in patients with preterm labor and intact membranes. Am J Reprod Immunol. 2014;72(5):458–474.
- Baughman JM, Nilsson R, Gohil VM, et al. A computational screen for regulators of oxidative phosphorylation implicates SLIRP in mitochondrial RNA homeostasis. PLoS Genet. 2009;5(8):e1000590.
- Nayak RR, Kearns M, Spielman RS, et al. Coexpression network based on natural variation in human gene expression reveals gene interactions and functions. Genome Res. 2009;19(11):1953–1962.
- Aras S, Pak O, Sommer N, et al. Oxygen-dependent expression of cytochrome c oxidase subunit 4-2 gene expression is mediated by transcription factors RBPJ, CXXC5 and CHCHD2. Nucleic Acids Res. 2013;41(4):2255–2266.
- Aras S, Arrabi H, Purandare N, et al. Abl2 kinase phosphorylates Bi-organellar regulator MNRR1 in mitochondria, stimulating respiration. Biochim Biophys Acta Mol Cell Res. 2017;1864(2):440–448.
- Aras S, Bai M, Lee I, et al. MNRR1 (formerly CHCHD2) is a bi-organellar regulator of mitochondrial metabolism. Mitochondrion. 2015;20:43–51.
- Grossman LI, Purandare N, Arshad R, et al. MNRR1, a biorganellar regulator of mitochondria. Oxid Med Cell Longevity. 2017;2017:6739236.
- Aras S, Purandare N, Gladyck S, et al. Mitochondrial nuclear retrograde regulator 1 (MNRR1) rescues the cellular phenotype of MELAS by inducing homeostatic mechanisms. Proc Natl Acad Sci USA. 2020;117(50):32056–32065.
- Liu Y, Clegg HV, Leslie PL, et al. CHCHD2 inhibits apoptosis by interacting with bcl-x L to regulate bax activation. Cell Death Differ. 2015;22(6):1035–1046.
- Liu Y, Zhang Y. CHCHD2 connects mitochondrial metabolism to apoptosis. Molecular & Cellular Oncology. 2015;2(4):e1004964.
- Zhang DW, Shao J, Lin J, et al. RIP3, an energy metabolism regulator that switches TNF-induced cell death from apoptosis to necrosis. Science. 2009;17(325(5938):332–336.
- Jang JY, Blum A, Liu J, et al. The role of mitochondria in aging. J Clin Invest. 2018;128(9):3662–3670.
- Sun N, Youle RJ, Finkel T. The mitochondrial basis of aging. Mol Cell. 2016;61(5):654–666.
- Lee I, Hüttemann M. Energy crisis: the role of oxidative phosphorylation in acute inflammation and sepsis. Biochim Biophys Acta. 2014;1842(9):1579–1586.
- Brealey D, Brand M, Hargreaves I, et al. Association between mitochondrial dysfunction and severity and outcome of septic shock. Lancet. 2002;360(9328):219–223.
- Takasu O, Gaut JP, Watanabe E, et al. Mechanisms of cardiac and renal dysfunction in patients dying of sepsis. Am J Respir Crit Care Med. 2013;187(5):509–517.
- Crouser ED. Mitochondrial dysfunction in septic shock and multiple organ dysfunction syndrome. Mitochondrion. 2004;4(5-6):729–741.
- Zou R, Tao J, Qiu J, et al. DNA-PKcs promotes sepsis-induced multiple organ failure by triggering mitochondrial dysfunction. J Adv Res. 2022;41:39–48.
- Fink MP. Cytopathic hypoxia. Mitochondrial dysfunction as mechanism contributing to organ dysfunction in sepsis. Critical Care Clinics. 2001;17(1):219–237.
- Purandare N, Kunji Y, Xi Y, et al. Lipopolysaccharide induces placental mitochondrial dysfunction in murine and human systems by reducing MNRR1 levels via a TLR4-independent pathway. iScience. 2022;25(11):105342.
- Romero R, Grivel JC, Tarca AL, et al. Evidence of perturbations of the cytokine network in preterm labor. Am J Obstet Gynecol. 2015;213(6):836.e1–836.e18.
- Yoon BH, Romero R, Moon JB, et al. Clinical significance of intra-amniotic inflammation in patients with preterm labor and intact membranes. Am J Obstet Gynecol. 2001;185(5):1130–1136.
- Romero R, Miranda J, Chaiworapongsa T, et al. A novel molecular microbiologic technique for the rapid diagnosis of microbial invasion of the amniotic cavity and intra-amniotic infection in preterm labor with intact membranes. Am J Reprod Immunol. 2014;71(4):330–358.
- Romero R, Quintero R, Nores J, et al. Amniotic fluid white blood cell count: a rapid and simple test to diagnose microbial invasion of the amniotic cavity and predict preterm delivery. Am J Obstet Gynecol. 1991;165(4 Pt 1):821–830.
- Romero R, Yoon BH, Mazor M, et al. A comparative study of the diagnostic performance of amniotic fluid glucose, white blood cell count, interleukin-6, and gram stain in the detection of microbial invasion in patients with preterm premature rupture of membranes. Am J Obstet Gynecol. 1993;169(4):839–851.
- Romero R, Yoon BH, Mazor M, et al. The diagnostic and prognostic value of amniotic fluid white blood cell count, glucose, interleukin-6, and gram stain in patients with preterm labor and intact membranes. Am J Obstet Gynecol. 1993;169(4):805–816.
- Romero R, Emamian M, Quintero R, et al. The value and limitations of the gram stain examination in the diagnosis of intraamniotic infection. Am J Obstet Gynecol. 1988;159(1):114–119.
- Longen S, Bien M, Bihlmaier K, et al. Systematic analysis of the twin cx(9)c protein family. J Mol Biol. 2009;393(2):356–368.
- Cavallaro G. Genome-wide analysis of eukaryotic twin CX9C proteins. Mol Biosyst. 2010;6(12):2459–2470.
- Modjtahedi N, Tokatlidis K, Dessen P, et al. Mitochondrial proteins containing coiled-coil-helix-coiled-coil-helix (CHCH) domains in health and disease. Trends Biochem Sci. 2016;41(3):245–260.
- Underwood MA, Gilbert WM, Sherman MP. Amniotic fluid: not just fetal urine anymore. J Perinatol. 2005;25(5):341–348.
- Larrabee PB, Johnson KL, Lai C, et al. Global gene expression analysis of the living human fetus using cell-free messenger RNA in amniotic fluid. Jama. 2005;293(7):836–842.
- Zwemer LM, Bianchi DW. The amniotic fluid transcriptome as a guide to understanding fetal disease. Cold Spring Harb Perspect Med. 2015;5(4):a123101.
- Ross MG, Brace RA. National institute of child health and development conference summary: amniotic fluid biology–basic and clinical aspects. J Matern Fetal Med. 2001;10(1):2–19.
- Cho CK, Shan SJ, Winsor EJ, et al. Proteomics analysis of human amniotic fluid. Mol Cell Proteomics. 2007;6(8):1406–1415.
- Hui L, Bianchi DW. Cell-free fetal nucleic acids in amniotic fluid. Hum Reprod Update. 2011;17(3):362–371.
- Funayama M, Ohe K, Amo T, et al. CHCHD2 mutations in autosomal dominant late-onset parkinson’s disease: a genome-wide linkage and sequencing study. Lancet Neurol. 2015;14(3):274–282.
- Feyeux M, Bourgois-Rocha F, Redfern A, et al. Early transcriptional changes linked to naturally occurring Huntington’s disease mutations in neural derivatives of human embryonic stem cells. Hum Mol Genet. 2012;21(17):3883–3895.
- Song R, Yang B, Gao X, et al. Cyclic adenosine monophosphate response element-binding protein transcriptionally regulates CHCHD2 associated with the molecular pathogenesis of hepatocellular carcinoma. Mol Med Rep. 2015;11(6):4053–4062.
- Wei Y, Vellanki RN, Coyaud É, et al. CHCHD2 is coamplified with EGFR in NSCLC and regulates mitochondrial function and cell migration. Mol Cancer Res. 2015;13(7):1119–1129.
- Nhan-Chang CL, Romero R, Tarca AL, et al. Characterization of the transcriptome of chorioamniotic membranes at the site of rupture in spontaneous labor at term. Am J Obstet Gynecol. 2010; 202(5):462.e1-41–462.41.
- Mittal P, Romero R, Tarca AL, et al. Characterization of the myometrial transcriptome and biological pathways of spontaneous human labor at term [research support, N.I.H., extramural]. Journal of Perinatal Medicine. 2010; 38(6):617–643.
- Hassan SS, Romero R, Haddad R, et al. The transcriptome of the uterine cervix before and after spontaneous term parturition [research support. Am J Obstet Gynecol. 2006;195(3):778–786.
- Madsen-Bouterse SA, Romero R, Tarca AL, et al. The transcriptome of the fetal inflammatory response syndrome. Am J Reprod Immunol (New York, NY: 1989). 2010;63(1):73–92.
- Michaels JE, Dasari S, Pereira L, et al. Comprehensive proteomic analysis of the human amniotic fluid proteome: gestational age-dependent changes. J Proteome Res. 2007;6(4):1277–1285.
- Queloz PA, Crettaz D, Thadikkaran L, et al. Proteomic analyses of amniotic fluid: potential applications in health and diseases. J Chromatogr B Analyt Technol Biomed Life Sci. 2007;1850(1-2):336–342.
- Bhatti G, Romero R, Gomez-Lopez N, et al. The amniotic fluid proteome changes with gestational age in normal pregnancy: a cross-sectional study. Sci Rep. 2022;12(1):601.
- Romero R, Dey SK, Fisher SJ. Preterm labor: one syndrome, many causes. Science (New York, NY.). 2014; 15345(6198):760–765.
- Romero R, Espinoza J, Kusanovic JP, et al. The preterm parturition syndrome. Bjog. 2006; Dec113 Suppl 3(Suppl 3):17–42.
- Romero R, Espinoza J, Gonçalves LF, et al. The role of inflammation and infection in preterm birth. Semin Reprod Med. 2007; 25(1):21–39.
- Goldenberg RL, Culhane JF, Iams JD, et al. Epidemiology and causes of preterm birth. Lancet. 2008;5371(9606):75–84.
- Gomez-Lopez N, Galaz J, Miller D, et al. The immunobiology of preterm labor and birth: intra-amniotic inflammation or breakdown of maternal-fetal homeostasis. Reproduction. 2022;20164(2):R11–R45.
- Goncalves LF, Chaiworapongsa T, Romero R. Intrauterine infection and prematurity. Mental Retardation Dev Disabil Res Rev. 2002;8(1):3–13.
- y MJ, Duffy L, Axthelm MK, et al. Ureaplasma parvum or Mycoplasma hominis as sole pathogens cause chorioamnionitis, preterm delivery, and fetal pneumonia in rhesus macaques. Reprod Sci. 2009;16(1):56–70.
- Bastek JA, Gomez LM, Elovitz MA. The role of inflammation and infection in preterm birth [review]. Clinics Perinatol. 2011;38(3):385–406.
- Morales WJ, Washington SR, 3rd, Lazar AJ. The effect of chorioamnionitis on perinatal outcome in preterm gestation. J Perinatol. 1987;(2):105–110.
- Sperling RS, Newton E, Gibbs RS. Intraamniotic infection in low-birth-weight infants. J Infect Dis. 1988;157(1):113–117.
- Romero R, Sirtori M, Oyarzun E, et al. Infection and labor. V. Prevalence, microbiology, and clinical significance of intraamniotic infection in women with preterm labor and intact membranes. Am J Obstet Gynecol. 1989;161(3):817–824.
- Yoon BH, JK, Romero et al. Amniotic fluid inflammatory cytokines (interleukin-6, interleukin-1beta, and tumor necrosis factor-alpha), neonatal brain white matter lesions, and cerebral palsy. Am J Obstet Gynecol. 1997;177(1):19–26.
- Yoon BH, Chang JW, Romero R. Isolation of Ureaplasma urealyticum from the amniotic cavity and adverse outcome in preterm labor. Obstet Gynecol. 1998;92(1):77–82.
- Hitti J, Tarczy-Hornoch P, Murphy J, et al. Amniotic fluid infection, cytokines, and adverse outcome among infants at 34 weeks’ gestation or less. Obstet Gynecol. 2001;98(6):1080–1088.
- Kirchner L, Helmer H, Heinze G, et al. Amnionitis with Ureaplasma urealyticum or other microbes leads to increased morbidity and prolonged hospitalization in very low birth weight infants. Eur J Obstet Gynecol Reprod Biol. 2007;134(1):44–50.
- Romero R, Gotsch F, Pineles B, et al. Inflammation in pregnancy: its roles in reproductive physiology, obstetrical complications, and fetal injury. Nutr Rev. 2007;65(12 Pt 2):S194–S202.
- Korzeniewski SJ, Romero R, Cortez J, et al. A “multi-hit” model of neonatal white matter injury: cumulative contributions of chronic placental inflammation, acute fetal inflammation and postnatal inflammatory events. J Perinatal Med. 2014;42(6):731–743.
- Oh KJ, Park JY, Lee J, et al. The combined exposure to intra-amniotic inflammation and neonatal respiratory distress syndrome increases the risk of intraventricular hemorrhage in preterm neonates. J Perinat Med. 2018;2646(1):9–20.
- Al-Haddad BJS, Oler E, Armistead B, et al. The fetal origins of mental illness. Am J Obstet Gynecol. 2019;221(6):549–562.
- Venkatesh KK, Leviton A, Hecht JL, et al. Histologic chorioamnionitis and risk of neurodevelopmental impairment at age 10 years among extremely preterm infants born before 28 weeks of gestation. Am J Obstet Gynecol. 2020;223(5):745.e1–745.e10.
- Köstlin-Gille N, Härtel C, Haug C, et al. Epidemiology of early and late onset neonatal sepsis in very low birthweight infants: data from the German neonatal network. Pediatr Infect Dis J. 2021;140(3):255–259.
- Plazyo O, Romero R, Unkel R, et al. HMGB1 induces an inflammatory response in the chorioamniotic membranes that is partially mediated by the inflammasome. Biol Reprod. 2016;95(6):130.
- Gomez-Lopez N, Romero R, Plazyo O, et al. Preterm labor in the absence of acute histologic chorioamnionitis is characterized by cellular senescence of the chorioamniotic membranes. Am J Obstet Gynecol. 2017;217(5):592.e1–592.e17.
- Gomez-Lopez N, Romero R, Panaitescu B, et al. Inflammasome activation during spontaneous preterm labor with intra-amniotic infection or sterile intra-amniotic inflammation. Am J Reprod Immunol.. 2018;80(5):e13049.
- Gomez-Lopez N, Romero R, Garcia-Flores V, et al. Inhibition of the NLRP3 inflammasome can prevent sterile intra-amniotic inflammation, preterm labor/birth, and adverse neonatal outcomes†. Biol Reprod. 2019;1100(5):1306–1318.
- Motomura K, Romero R, Galaz J, et al. RNA sequencing reveals distinct immune responses in the chorioamniotic membranes of women with preterm labor and microbial or sterile intra-amniotic inflammation. Infect Immun. 2021;89(5):e00819–e00820.
- Gomez-Lopez N, Romero R, Galaz J, et al. Cellular immune responses in amniotic fluid of women with preterm labor and intra-amniotic infection or intra-amniotic inflammation. Am J Reprod Immunol. 2019;82(5):e13171.
- Gomez-Lopez N, Romero R, Xu Y, et al. The immunophenotype of amniotic fluid leukocytes in normal and complicated pregnancies. Am J Reprod Immunol. 2018;79(4):e12827.
- tinez-Varea A, Romero R, Xu Y, et al. Clinical chorioamnionitis at term VII: the amniotic fluid cellular immune response. J Perinat Med. 2017;2645(5):523–538.
- Galaz J, Romero R, Xu Y, et al. Cellular immune responses in amniotic fluid of women with preterm clinical chorioamnionitis. Inflamm Res 2020;69(2):203–216.
- Gomez-Lopez N, Romero R, Varrey A, et al. RNA sequencing reveals diverse functions of amniotic fluid neutrophils and monocytes/macrophages in Intra-Amniotic infection. J Innate Immun. 2021;13(2):63–82.
- Gravett MG, Hummel D, Eschenbach DA, et al. Preterm labor associated with subclinical amniotic fluid infection and with bacterial vaginosis. Obstet Gynecol. 1986;67(2):229–237.
- Gibbs RS, Romero R, Hillier SL, et al. A review of premature birth and subclinical infection. Am J Obstet Gynecol. 1992;166(5):1515–1528.
- Romero R, Espinoza J, Gonçalves LF, et al. Inflammation in preterm and term labour and delivery. Seminars Fetal Neonatal Medicine. 2006;11(5):317–326.
- Romero R. Prevention of spontaneous preterm birth: the role of sonographic cervical length in identifying patients who may benefit from progesterone treatment. Ultrasound Obstetr Gynecol 2007;30(5):675–686.
- Gibbs RS, Blanco JD, Clair S, et al. Quantitative bacteriology of amniotic fluid from women with clinical intraamniotic infection at term. J Infect Dis. 1982;145(1):1–8.
- Gibbs RS, Duff P. Progress in pathogenesis and management of clinical intraamniotic infection [review. Am J Obstet Gynecol. 1991;164(5 Pt 1):1317–1326.
- Kusanovic JP, g E, Romero R, et al. Characterization of amniotic fluid sludge in preterm and term gestations. J Matern -Fetal Neonatal Med. 2022;27:1–10.
- Lee SM, Romero R, Park JS, et al. A transcervical amniotic fluid collector: a new medical device for the assessment of amniotic fluid in patients with ruptured membranes. J Perinat Med. 2015;43(4):381–389.
- Norwitz ER, Robinson JN, Challis JR. The control of labor. N Engl J Med. 1999;26341(9):660–666.
- Smith R. Parturition. N Engl J Med. 2007;356(3):271–283.
- Liggins G. Cervical ripening as an inflammatory reaction. In: Ellwood D, Anderson A, editors. The cervix in pregnancy and labor: clinical and biochemical investigation. Edinburgh: Churchill Livingstone; 1981. p. 1–9.
- Norman JE, Bollapragada S, Yuan M, et al. Inflammatory pathways in the mechanism of parturition. BMC Pregnancy Childbirth. 2007;7(Suppl 1):S7.
- Gomez-Lopez N, Guilbert LJ, Olson DM. Invasion of the leukocytes into the fetal-maternal interface during pregnancy. J Leukoc Biol. 2010;88(4):625–633.
- Norwitz ER, Bonney EA, Snegovskikh VV, et al. Molecular regulation of parturition: the role of the decidual clock. Cold Spring Harb Perspect Med. 2015;5(11):a023143.
- Kyathanahalli C, Snedden M, Hirsch E. Is human labor at term an inflammatory condition?†. Biol Reprod. 2023;108(1):23–40.
- Romero R, Xu Y, Plazyo O, et al. A role for the inflammasome in spontaneous labor at term. Am J Reprod Immunol. 2018;79(6):e12440.
- Gomez-Lopez N, Motomura K, Miller D, et al. Inflammasomes: their role in normal and complicated pregnancies. J Immunol. 2019; 1203(11):2757–2769.
- Thomson AJ, Telfer JF, Young A, et al. Leukocytes infiltrate the myometrium during human parturition: further evidence that labour is an inflammatory process. Hum Reprod. 1999;14(1):229–236.
- Mackler AM, Iezza G, Akin MR, et al. Macrophage trafficking in the uterus and cervix precedes parturition in the mouse. Biol Reprod. 1999;61(4):879–883.
- Young A, Thomson AJ, Ledingham M, et al. Immunolocalization of proinflammatory cytokines in myometrium, cervix, and fetal membranes during human parturition at term. Biol Reprod. 2002;66(2):445–449.
- Osman I, Young A, Ledingham MA, et al. Leukocyte density and pro-inflammatory cytokine expression in human fetal membranes, decidua, cervix and myometrium before and during labour at term. Mol Hum Reprod. 2003;9(1):41–45.
- Yellon SM, Mackler AM, Kirby MA. The role of leukocyte traffic and activation in parturition. J Soc Gynecol Investig. 2003;10(6):323–338.
- Shynlova O, Tsui P, Dorogin A, et al. Monocyte chemoattractant protein-1 (CCL-2) integrates mechanical and endocrine signals that mediate term and preterm labor. J Immunol. 2008;181(2):1470–1479.
- Shynlova O, Lee YH, Srikhajon K, et al. Physiologic uterine inflammation and labor onset: integration of endocrine and mechanical signals. Reprod Sci. 2013;20(2):154–167.
- Pique-Regi R, Romero R, Garcia-Flores V, et al. A single-cell atlas of the myometrium in human parturition. JCI Insight. 2022;7(5):e153921.
- Stanfield Z, Lai PF, Lei K, et al. Myometrial transcriptional signatures of human parturition. Front Genet. 2019;10:185.
- Fidel PL, Jr., Romero R, Ramirez M, et al. Interleukin-1 receptor antagonist (IL-1ra) production by human amnion, chorion, and decidua. Am J Reprod Immunol. 1994;32(1):1–7.
- Keelan JA, vin KW, Sato TA, et al. Cytokine abundance in placental tissues: evidence of inflammatory activation in gestational membranes with term and preterm parturition. Am J Obstet Gynecol. 1999;181(6):1530–1536.
- Gomez-Lopez N, Estrada-Gutierrez G, Jimenez-Zamudio L, et al. Fetal membranes exhibit selective leukocyte chemotaxic activity during human labor. J Reprod Immunol. 2009;80(1-2):122–131.
- Gomez-Lopez N, Vega-Sanchez R, Castillo-Castrejon M, et al. Evidence for a role for the adaptive immune response in human term parturition. Am J Reprod Immunol. 2013;69(3):212–230.
- Hamilton SA, Tower CL, Jones RL. Identification of chemokines associated with the recruitment of decidual leukocytes in human labour: potential novel targets for preterm labour. PLoS One. 2013;8(2):e56946.
- Sacks G, Sargent I, Redman C. An innate view of human pregnancy. Immunol Today. 1999;20(3):114–118.
- Timmons BC, Fairhurst AM, Mahendroo MS. Temporal changes in myeloid cells in the cervix during pregnancy and parturition. J Immunol. 2009; 1182(5):2700–2707.
- Romero R, Avila C, Santhanam U, et al. Amniotic fluid interleukin 6 in preterm labor. Association with infection. J Clin Invest. 1990 ;85(5):1392–1400.
- Santhanam U, Avila C, Romero R, et al. Cytokines in normal and abnormal parturition: elevated amniotic fluid interleukin-6 levels in women with premature rupture of membranes associated with intrauterine infection. Cytokine. 1991;3(2):155–163.
- Saito S, Kasahara T, Kato Y, et al. Elevation of amniotic fluid interleukin 6 (IL-6), IL-8 and granulocyte colony stimulating factor (G-CSF) in term and preterm parturition. Cytokine. 1993;5(1):81–88.
- Romero R, Mazor M, Brandt F, et al. Interleukin-1 alpha and interleukin-1 beta in preterm and term human parturition. Am J Reprod Immunol. 1992;27(3-4):117–123.
- Romero R, Mazor M, ulveda W, et al. Tumor necrosis factor in preterm and term labor. Am J Obstet Gynecol. 1992;166(5):1576–1587.
- Hayashi M, Zhu K, Sagesaka T, et al. Amniotic fluid levels of tumor necrosis factor-alpha and soluble tumor necrosis factor receptor 1 before and after the onset of labor in normal pregnancies. Horm Metab Res. 2008;40(4):251–256.
- Houben ML, Nikkels PG, van Bleek GM, et al. The association between intrauterine inflammation and spontaneous vaginal delivery at term: a cross-sectional study. PLoS One. 2009;104(8):e6572.
- Maeda A, Fadeel B. Mitochondria released by cells undergoing TNF-α-induced necroptosis act as danger signals. Cell Death Dis. 2014;5(7):e1312.
- Haddad R, Tromp G, Kuivaniemi H, et al. Human spontaneous labor without histologic chorioamnionitis is characterized by an acute inflammation gene expression signature. Am J Obstet Gynecol. 2006;195(2):394.e1-24–394.24.