Abstract
Enantiornithines are the most speciose avian clade in the Mesozoic, with a fossil record that nearly spans the Cretaceous; however, with less than half of known taxa preserving skull material, our understanding of their cranial morphology remains incomplete. Here we present a comprehensive overview of the current knowledge of enantiornithine skull anatomy and discuss the range of morphologies known for each of the main cranial elements. The typical enantiornithine skull retains numerous ancestral features such as the absence of fusion among bones, the presence of a postorbital bone, a primitive quadrate with a single headed otic process, an unforked dentary, and teeth. The postorbital in at least one taxon is unreduced, suggesting the existence of a complete infratemporal fenestra and thus an unmodified diapsid skull as in confuciusornithids. The rostrum is well known and shows considerable variation, typical of theropods; however, in terms of rostral proportions, enantiornithines are extremely limited within the modern avian spectrum. Although Late Cretaceous skull material is extremely fragmentary, when compared to Early Cretaceous material it reveals a trend towards more specialized morphologies in younger taxa. The foramen magnum in all taxa points caudally, indicating that the ‘flexed’ type skull morphology may not have evolved in this group. Enantiornithine teeth show considerable diversity in numbers, size, morphology and placement, ranging from taxa with large teeth found throughout the jaws to taxa with small, rostrally restricted teeth, to the fully edentulous. Despite limited preservation of skull material, a number of trophic specializations can be deduced from the range of preserved morphologies, further hinting at the morphological and ecological diversity of the Cretaceous Enantiornithes.
Keywords:
Introduction
Enantiornithes is a diverse group of Cretaceous birds known from deposits worldwide (CitationChiappe & Walker 2002). With over 50 species named in the past three decades, enantiornithines are the most speciose clade of Mesozoic birds. These small to medium sized birds are phylogenetically intermediate between basal pygostylians and modern birds, forming the sister group to Ornithuromorpha, which together make the clade Ornithothoraces (CitationChiappe 2002; CitationChiappe & Walker 2002). While their placement in the avian tree is well established, it is based primarily on postcranial synapomorphies rather than features of the skull (CitationChiappe 2002). A comparative study of the cranial morphology of enantiornithines has the potential to provide additional synapomorphies that can diagnose the entire group, as well as subclades, on the basis of skull morphologies, and therefore illuminate aspects of cranial evolution in basal birds. Furthermore, from the known morphology of basal bird crania, it can be inferred that the most recent ornithothoracine common ancestor retained a primitive toothed skull with an overall lack of bone fusion, and that the development of a toothless beak and an enlarged premaxilla in several avian lineages including Enantiornithes is the result of convergent evolution (CitationChiappe et al. 1999; CitationZhou & Zhang 2006a). Thus studies of enantiornithine skull morphology can reveal significant information about the extent, the timing and the sequence of the evolution of modern morphologies (and their correlated ecologies) in a much older lineage, distantly related to modern birds.
For the first half of the clade's history, only very limited enantiornithine skull material had been uncovered (CitationElzanowski 1974, 1977; CitationWalker 1981; CitationChiappe 1991) and very little information could be inferred for the clade. Subsequently, dozens of nearly complete skeletons from the Early Cretaceous Jehol Group of northern China were discovered; these have provided unprecedented information elucidating the anatomical and taxonomic diversity and the evolution of Enantiornithes (; CitationZhang & Zhou 2000; CitationZhang et al. 2000; CitationZhou & Zhang 2006a; CitationZhou et al. 2008). Even though almost all these largely complete specimens from the Jehol Group retain the skull, the cranial anatomy of the clade has remained largely unknown, due to the poor preservation typical of cranial bones, primarily preserved in 2D with the bones often torn between two slabs, crushed and/or disarticulated (CitationSereno & Rao 1992; CitationZhou et al. 1992; CitationHou 1997).
Figure 1 Photographs of well preserved enantiornithine skulls: A, Rapaxavis pani, DNHM D2522; B, Longipteryx sp., DNHM D2889; C, Pengornis houi, IVPP V15336; D, Shenqiornis mengi, DNHM D2950; E, Longipteryx chaoyangensis, IVPP V12552; F, Cathayornis yandica, IVPP V9769; G, juvenile Euenantiornithes indet, GMV 2158. All scale bars = 1 cm.
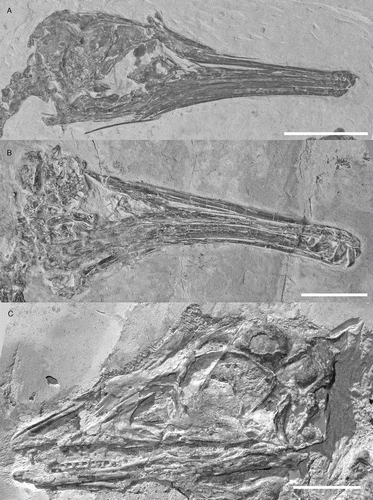
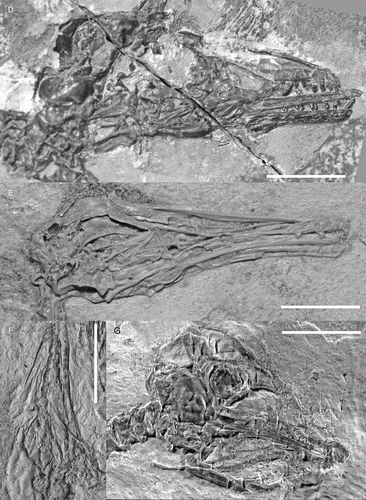
Figure 2 Camera lucida drawings of select enantiornithine skulls: A, Rapaxavis pani, DNHM D2522; B, Longirostravis hani, IVPP V11309; C, Longipteryx chaoyangensis, IVPP V12325; D, Eoenantiornis buhleri, IVPP 11537; E, Longipteryx sp., DNHM D2889; F, Longipteryx chaoyangensis, IVPP V12552; G, Shenqiornis mengi, DNHM D2951; H, Pengornis houi, IVPP 15336; I, Alethoalaornis agitornis, LPM 00009; J, Cathayornis yandica, IVPP V9769; K, Dapingfangornis sentisorhinus, LPM 00039; L, M, Gobipteryx minuta, IGM-100/1011, right and left lateral views.
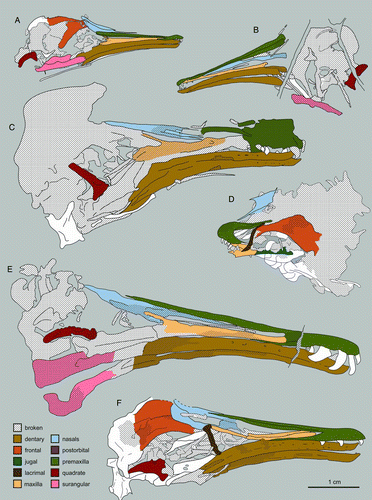
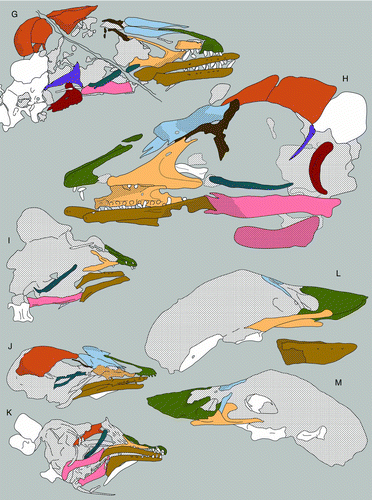
Reconstructions of the enantiornithine skull are few, which can be attributed to preservational limitations. The only major attempts to construct the enantiornithine skull are those of CitationMartin & Zhou (1997) and CitationSanz et al. (1997). The reconstruction of CitationMartin & Zhou (1997) based on Cathayornis yandica (IVPP V9769, IVPP V10896 and IVPP V10916—the latter now the holotype of Eocathayornis walkeri CitationZhou, 2002) lacks a postorbital and possesses unsubstantiated lacrimal and premaxilla morphology. The second reconstruction, that of the Montsec hatchling (LP 4450), is more accurate. However, both reconstructions are based on single taxa, and little has been done to find common morphological ground between all members of the clade.
The recent discovery of exceptionally preserved specimens, such as Eoenantiornis buhleri, Longipteryx chaoyangensis, Pengornis houi and new specimens of Gobipteryx minuta, provides new insights into how the enantiornithine skull was constructed and the degree of variation among taxa (CitationHou et al. 1999; CitationZhang et al. 2000; CitationZhou et al. 2008; CitationChiappe et al. 2001). Together with data extracted from the nearly 30 skulls used in this study, including every published taxon that preserves cranial material, we can begin to reveal the anatomy of the enantiornithine skull. Common morphologies emerge and a better understanding of enantiornithine skull morphology offers an interesting case study in diversity and evolution. By focusing on an aspect of the skeleton other than morphologies directly related to flight, we may be able to understand evolutionary pressures acting on early avifaunas that are less influenced by aerodynamic constraints.
Material
Approximately 60 species of enantiornithines have been named (including several potential synonyms); of these, 25 preserve skull material (). An additional four unnamed juveniles (CitationSanz et al. 1997; CitationChiappe et al. 2007) preserve crania and will be discussed in this review. This study relies almost entirely on Asian material and this should be recognized as a major bias of any enantiornithine study; more than 90% of the specimens come from the Jehol Group, which records a time interval spanning approximately 11 Ma (c. 131–120 Ma) and representing a single geographical area (CitationHe et al. 2006; CitationZhou 2006; CitationYang et al. 2007).
Table 1 A list of known enantiornithine specimens that preserve skull material; grey indicates unpublished material.
The best skull material belongs to Gobipteryx, specimen IGM-100/1011 from the Campanian Barun Goyot and Djadokhta formations (, M; CitationChiappe et al. 2001). Though only preserving the rostral half of the skull, IGM-100/1011 boasts three-dimensional preservation and provides the most reliable information on the enantiornithine palate. Additional Gobipteryx skulls (ZPAL-MgR-I/12, ZPAL-MgR-I/32, PIN 4492; CitationElzanowski 1976, 1977; CitationKurochkin 1996) are known but the material is either compromised (diagenetically recrystallized), crushed, or largely incomplete. The Jehol Group has produced six specimens with well-preserved skulls: IVPP V11537—the holotype of Pengornis houi (Figs , ; CitationZhou et al. 2008), IVPP V15336—the holotype of Eoenantiornis buhleri (; CitationHou et al. 1999; CitationZhou et al. 2005), DNHM D2522 –the holotype of Rapaxavis pani (, ; Morchhauser et al. 2009), DNHM D2950/1—the holotype of Shenqiornis mengi (, ; CitationWang et al. 2010), LPM 00039—the holotype of Dapingfangornis sentisorhinus (; CitationLi et al. 2006—unfortunately, this specimen is poorly prepared and glue obscures most features), and two referred specimens of Longipteryx chaoyangensis, IVPP V12552 (, ; CitationZhang et al. 2000; CitationChiappe et al. 2007) and DNHM D2889 (, ). Other largely complete though poorly preserved skulls include: IVPP V12325—the holotype of L. chaoyangensis (; CitationZhang et al. 2000), IVPP V11309—the holotype of Longirostravis hani (; CitationHou et al. 2004), NIGP-CAS 130722—the holotype of Hebeiornis fengningensis (CitationXu et al. 1999; CitationZhang et al. 2004), IVPP V11665—the holotype of Protopteryx fengningensis (CitationZhang & Zhou 2000), IVPP V9769—the holotype of Cathayornis yandica (, ; CitationZhou et al. 1992), DNHM D1878—the holotype of Shanweiniao cooperorum (CitationO’Connor et al. 2009), and LPM 00009, LPM 00038 and LPM B00017 (; published as LPM 00040)—three specimens of Alethoalaornis agitornis (CitationLi et al. 2007). Juvenile Euenantiornithes indet. (GMV 2158, GMV 2159—, and NIGPAS 130723/GMV 2156) were also included in this study (CitationChiappe et al. 2007). This study relies primarily on the morphology from these specimens but includes details discerned from two-dozen other enantiornithine fossils (), including several that are undescribed (DNHM D2836, DNHM D2510/1, DNHM D2567/8, DNHM D2952/3, DNHM D2884–1/2, DNHM D2130, DNHM D2566). The holotype of Dalingheornis liwei (CNU VB2005001; CitationZhang et al. 2006) is in a private collection (Z.-H. Zhang pers. comm.) and the skull of the holotype of Paraprotopteryx gracilis (STM V001) does not belong to the main slab (CitationZheng et al. 2007; Z.-H. Zhang pers. comm.). Thus these taxa are listed in as published with skull material but not included in this morphological review. Most birds from the Jehol Group (with the exception of Cathayornis, Longipteryx, Yanornis, and Alethoalaornis) are known from a single specimen; unless specified, if the generic name is used, it refers to the holotype specimen.
Figure 3 Interpretive drawings of select juvenile enantiornithine skulls: A, GMV 2159; B, C, LP 4450 slab and counterslab. Anatomical abbreviations: cmf, caudal mandibular fenestra; den, dentary; frn, frontal; jug, jugal; la, lacrimal; max, maxilla; nas, nasal; oc, occipital condyle; pmx, premaxilla; po, postorbital; qd, quadrate; rmf, rostral mandibular fenestra; sq, squamosal; sur, surangular.
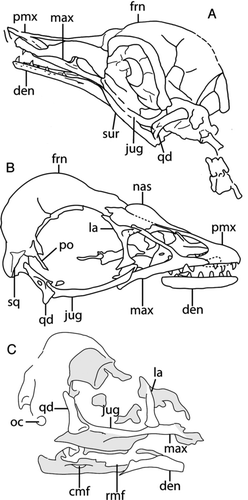
Outside Asia, the published record of enantiornithine skull material is limited to a nearly complete juvenile specimen from the Lower Cretaceous La Pedrera de Rubies Lithographic Limestones Formation, Lleida, Spain (, C; LP 4450—CitationSanz et al. 1997), and a partial braincase belonging to the holotype of Neuquenornis volans from the Upper Cretaceous (Campanian) Río Colorado Formation of Neuquén, Argentina (; MUCPv-142—CitationChiappe & Calvo 1994). A partial mandible from the Upper Cretaceous (Maastrichtian) Lecho Formation, El Brete, Argentina (PVL 4698) has been suggested to be enantiornithine based on the absence of any other avian material from the locality (CitationChiappe & Walker 2002). Currently there are no synapomorphies that support the placement of this specimen within the group, thus it will not be included in this review. The entire Late Cretaceous record of enantiornithine skull material is limited to the Neuquenornis and Gobipteryx material.
Despite the serious limitations of material in terms of temporal and geographical distribution, even the few known specimens record a large range of morphologies and specializations, and some major evolutionary patterns can be discerned.
Institutional abbreviations
CNU: Capital Normal University, Beijing, China; DNHM: Dalian Natural History Museum, Dalian, Liaoning China; GMV: Geological Museum of China, Beijing, China; IGM: Geological Institute, Mongolian Academy of Sciences, Ulaan Bataar, Mongolia; IVPP: Institute of Vertebrate Paleontology and Paleoanthropology, Beijing, China; LP: Institut d’Estudis Ilerdencs, Lleida, Spain; LPM: Liaoning Paleontological Museum, Shenyang, Liaoning, China; MUCPv: Museo de Ciencias Naturales, Universidad Nacional del Comahue, Neuquén, Argentina; NIGP-CAS: Nanjing Institute of Geology and Paleontology, Chinese Academy of Sciences, Nanjing, Jiangsu, China; PIN: Paleontological Institute, Moscow, Russia; PVL: Paleontología de Vertebrados, Fundación-Instituto Miguel Lillo, Universidad Nacional de Tucúman, Tucúman, Argentina; STM: Shandong Tianyu Museum, Tianyu, Shandong, China; ZPAL-MgR: Zoological Institute of Paleobiology, Polish Academy of Sciences, Warsaw, Poland.
Morphology
Anatomical nomenclature mainly follows CitationBaumel & Witmer (1993); certain structures not cited therein follow CitationHoward (1929). While the Latin terminology used by CitationBaumel & Witmer (1993) is retained for muscles and ligaments, osteological structures are described using English equivalents of the Latin terms.
Cranium
The rostrum, orbit and braincase in all enantiornithine taxa appear aligned in the same plane in lateral view (, ). All known foramina magna are directed caudally, indicating that these birds had ‘extended’ type skull morphology (; CitationMarinelli 1928; CitationVan der Klaauw 1948). Well-preserved skulls indicate enantiornithines were typically mesorostrine (sensu CitationBusbey 1995) with the rostrum contributing to around 50% of the total skull length. Longipterygid enantiornithines (e.g. Longirostravis, Longipteryx and others), though not truly longirostrine (sensu CitationBusbey 1995), represent a significant departure from other enantiornithines and an excursion into new morphospace, with the average rostrum being 65% of the skull length ().
Premaxilla
This element varies considerably throughout the clade in degree of fusion, relative lengths of the nasal and maxillary processes, degree of facial contribution, morphology of the articulations with the nasal and maxilla, and dentition. The premaxillae range from completely free from one another (unfused) (e.g. Pengornis; , ) to rostrally fused (nasal processes remain unfused) (e.g. Eoenantiornis, Longipteryx, Gobipteryx), and in some cases (e.g. IVPP V10897—the holotype of Cuspirostrisornis) these bones even appear fused along their entire length.
The prenarial portion of the premaxilla ranges from low, with the dorsal and ventral margins nearly parallel (e.g. Boluochia, Longipteryx), to high, with the dorsal margin strongly angled caudodorsally forming a skull with a more robust rostrum (e.g. Shenqiornis). The prenarial region is usually short and thus small (e.g. Longirostravis, Eoenantiornis), but in some taxa it is long (e.g. Longipteryx) and massive (e.g. Gobipteryx). The premaxilla typically bears four teeth, as in most non-avian theropods and other basal birds (e.g. Archaeopteryx, Sapeornis). Teeth are absent in Gobipteryx, thus far the only known edentulous enantiornithine (CitationElzanowski 1976, 1977). The nasal (frontal) processes of the premaxilla are shorter than those of modern birds, typically extending only to the level of the caudal margin of the nares (e.g. Shenqiornis) and not reaching the frontals. Although the morphology is not limited to this group (present also in Gobipteryx), the longipterygids all possess elongate nasal processes that extend nearly to the level of the caudal margin of the antorbital fenestra (e.g. Rapaxavis, Longirostravis, Shanweiniao).
The articulation of the premaxillae with the nasals is typically wedge-like, extending for approximately 50% of the length of the nasal (e.g. Shenqiornis, C. yandica, Rapaxavis).
The premaxilla is often perforated with small nutrient foramina along the facial margin (e.g. Longirostravis, Pengornis, Eoenantiornis, Longipteryx). In the presumably beaked Gobipteryx, the premaxilla bears a pair of dorsal grooves in which the nutrient foramina are located (CitationElzanowski 1977; CitationChiappe et al. 2001). This distribution differs from foramina associated with sensory organs in modern birds, which have been suggested for the beaked bird Confuciusornis (CitationZinoviev 2009), in which the foramina have a greater distribution and are more densely packed (CitationPiersma et al. 1998). The maxillary process of the premaxilla is typically short so that the facial contribution of this bone is restricted rostrally in most taxa (e.g. Pengornis, Eoenantiornis, Gobipteryx). However, the three-dimensionally preserved skulls of Gobipteryx (CitationChiappe et al. 2001) show that the maxillary process of this taxon extends for approximately half the length of the rostrum, but a portion of this is laterally obscured by the overlapping premaxillary process of the maxilla. While the condition in Gobipteryx suggests that the visible portion of the maxillary process of taxa preserved in two dimensions such as Eoenantiornis may extend farther caudally, the disarticulated premaxilla of IVPP V10897 (i.e. Cuspirostrisornis) indicates that, in at least some taxa, the maxillary process of this bone was short. In Longipteryx, a long and tapering maxillary process fully exposed laterally shows that the premaxilla of this taxon also contributed to nearly half the facial margin (IVPP V12552). Unsurprisingly, the taxa with a more extensive contribution of the premaxilla to the facial margin (e.g. Longipteryx and Gobipteryx) also possess a larger prenarial region. Very little is known about the details of the premaxilla–maxilla contact. In Gobipteryx it is complex; the maxillary process of the premaxilla tightly fits into a dorsal groove defined by the premaxillary process of the maxilla (CitationChiappe et al. 2001).
Nasal
The nasal varies in relative length, width, and morphology of the maxillary process. The nasals are typically elongate bones that contact each other; however, the long nasal processes of the premaxilla of Longipteryx may have separated both nasals for most of their length (, E, F). The premaxillary (internarial) process of the nasal is sharply tapered. The maxillary process of the nasal is not present in all taxa. The maxillary process, when present, is typically short—shorter than the premaxillary process—and also sharply tapered (e.g. Eoenantiornis, C. yandica). The maxillary process is longer than the premaxillary process in at least Gobipteryx (, M). In Longipteryx, the maxillary process is reduced so that it does not extend ventrally beyond the caudodorsal margin of the nares, while in other taxa (e.g. Shenqiornis, Rapaxavis, Shanweiniao), the process is completely absent ().
The mediolateral width of the nasal corpus varies from very narrow (e.g. Shenqiornis, Longipteryx, Rapaxavis) to broad (e.g. Eoenantiornis, C. yandica). Taphonomic biases notwithstanding, estimated nasal width-to-length ratios vary from 0.04 in taxa such as Rapaxavis (), to 0.22 in C. yandica (IVPP V9769; ). Caudally, the nasal articulates with the lacrimal and the frontal. In Pengornis and LP 4450, the articulation with the lacrimal extends over the caudal half of the lateral margin of the nasal. While the shape of the articulation with the frontal remains unclear, the caudal margin of the nasal is typically rounded (e.g. Longipteryx, C. yandica, Shenqiornis).
Maxilla
The maxilla is not as well known as the premaxilla. It varies mainly in dentition, relative lengths of the premaxillary, jugal, and nasal processes, and fenestration. The typical maxilla contributes to a majority of the facial margin, has premaxillary and jugal processes (the jugal process here refers to the ramus of the maxilla that extends from the rostral margin of the antorbital fossa to the articulation with the jugal) of subequal length, and bears teeth (e.g. Shenqiornis, Gobipteryx, Hebeiornis, Pengornis). Gobipteryx and the longipterygids represent the only known examples in which the maxilla lacks teeth. When present, the number of teeth varies from 5–6 to 11. The longipterygid Rapaxavis further departs from the typical morphology, possessing a premaxillary process three times longer than the jugal process (). Conversely, in some taxa (e.g. LP 4450) the premaxillary process is shorter than the jugal process (), although there are no known taxa in which the latter is considerably longer than the former.
The nasal process (ramus) is typically thin and caudally directed, separating the nares from the antorbital fenestra. The rostral portion of the nasal process of Pengornis forms a fenestrated recessed bony wall that medially lines the rostral third of the antorbital fossa (, ). The recessed nasal process and accessory antorbital fenestra, less clearly preserved in DNHM D2889 (, ) and suggested to be present in C. yandica (CitationMartin & Zhou 1997), may have a broader distribution but preservational biases in two-dimensional specimens make this difficult to determine (including the presence of this condition in C. yandica). The nasal process of the maxilla is reduced in Gobipteryx, only contributing to the caudoventral margin of the nares, and thus clearly lacked the above-mentioned recessed bony wall (, M). In Pengornis, the caudodorsal end of its well developed nasal process forms a fork (unforked in LP 4450), presumably for the articulation with the lacrimal, in which the ventral ramus projects considerably beyond the dorsal ramus (). Whether the nasal process of the maxilla articulated with the lacrimal in other taxa is not clear due to the poor preservation of the latter bone in most specimens, yet the elongate nasal process of some taxa (e.g. Shenqiornis, LP 4450, Longipteryx) suggests that this condition may have had a wider distribution.
Figure 4 Close-up of the skull of Eoenantiornis buhleri IVPP showing the two alternate interpretations of the specimen: A, the S-shaped bone fragment is inferred to be part of the maxilla as in CitationZhou et al. (2005); B, the S-shaped bone as the lacrimal. See for anatomical abbreviations.
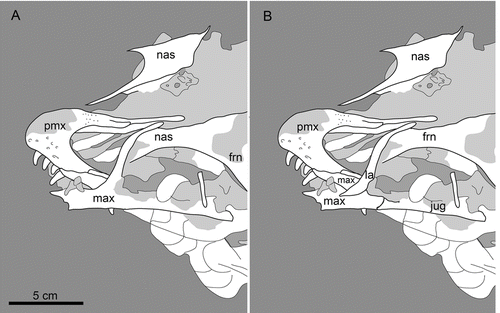
Lacrimal
The morphology of this bone is definitively clear in only two specimens: Pengornis (IVPP V15336) and LP 4450 (). In these specimens, the lacrimal is T-shaped as in most non-avian maniraptorans (e.g. Velociraptor, Jinfengopteryx, Tsaagan). The dorsal rami are tapered and angled so that the rostral ramus is rostroventrally directed and the caudal ramus is oriented caudodorsally. In Pengornis, the dorsal margin is strongly concave between the two rami defining a small foramen between the lacrimal and the nasal (). The dorsal margin of the nasal also appears slightly concave in LP 4450; however, the nasal is broken along the lacrimal contact, making it impossible to determine if a foramen was present. In LP 4450, the descending (ventral) ramus of the lacrimal appears to form a mediolaterally broad sheet descending perpendicularly to the long axis of the skull; the distal end, not preserved in Pengornis, expands into a broad articulation with the jugal. Eoenantiornis may exhibit a unique lacrimal morphology, depending on the reinterpretation of the structure identified as the nasal process of the maxilla by Zhou et al. (Citation2005; ). In this alternative view, the caudal half of the skull is interpreted as pushed forward, covering most of the left maxilla so that only the caudal end of this bone is visible in lateral view (). The nasal process of the maxilla of CitationZhou et al. (2005) is thus regarded as the lacrimal (). If this is the case, the lacrimal of Eoenantiornis is a thin, rostroventrally angled S-shaped bone with an expanded ventral ramus and a caudally tapering dorsal ramus, a design significantly different from the T-shaped bone described above.
Jugal
In most taxa the jugal appears to be a simple rod-like element lacking a postorbital process (e.g. C. yandica, Pengornis, Rapaxavis). This bone is best preserved in LP 4450 (). In this specimen, the rostral end articulates with both the maxilla and lacrimal, contributing to the caudoventral corner of the antorbital fenestra. The caudal end is forked, with the dorsal ramus longer and more robust than the ventral ramus, a condition similar to that in Archaeopteryx. This morphology also appears to be present in Shenqiornis (CitationWang et al. 2010).
Postorbital
This element—absent among modern birds—is retained within Enantiornithes but is only definitively preserved in three specimens: LP 4450, Pengornis and Shenqiornis. In LP 4450 and Shenqiornis it exhibits a typical T-shaped design but in Pengornis the caudodorsal (squamosal) ramus is either absent or broken. The postorbital of these specimens shows considerable variation in size and morphology. In Shenqiornis it is large and broad, with a straight and tapered jugal process that possibly enclosed a complete infratemporal fenestra (, ). In contrast, in Pengornis and LP 4450 the bone is much smaller, with a rostrally deflected, splint-like jugal process that fails to reach the jugal (, ). In LP 4450 and Shenqiornis the dorsal margin of the postorbital is concave, suggesting it contributed to the margin of a supratemporal fenestra. The rostrodorsal (frontal) ramus is larger than the caudodorsal ramus in LP 4450; in Shenqiornis the disarticulated postorbital cannot be oriented unequivocally, however one ramus is much more robust than the other, and has a more dorsal position.
Squamosal
The only known squamosal is preserved in LP 4450 (). It is a small triradiate bone that, unlike modern birds, is unincorporated into the braincase. In lateral view it has a broad descending process and a small caudally directed process. The third process articulated with the dorsocaudal ramus of the postorbital and contributed to the caudal margin of the supratemporal fenestra.
Quadrate
The quadrate is preserved in several taxa (e.g. Rapaxavis, Pengornis, LP 4450, Shenqiornis, Eocathayornis, Protopteryx, Gobipteryx), but unfortunately few morphological details are known (, ). The mandibular process appears bicondylar in all specimens, with the medial condyle larger than the lateral condyle. As in other basal birds and non-avian theropods, the orbital ramus is broad (e.g. Gobipteryx), lacking the narrow and tapered morphology of ornithurines. The caudal surface is excavated. Some quadrates are pierced by a foramen, which may be an indication that the bone was pneumatic; the ‘pneumatopore’ is interpreted as piercing the bone caudally in Shenqiornis and Pengornis.
Frontal
Completely unfused in most taxa, the frontals are rostrocaudally elongate, narrow rostrally and largely expanded caudally (e.g. C. yandica, Shenqiornis, Pengornis, DNHM D2511; ). They form a majority of the dorsal margin of the orbit; in Pengornis, this portion of the lateral margin of the frontal forms a slight laterally projecting surface. Best evidenced in Neuquenornis, the frontals contact on the midline forming a longitudinal suture; the caudal margin of each frontal is rounded, forming a caudally convex suture with the parietals.
Parietal
These bones are unfused to each other and the rest of the braincase (e.g. Dapingfangornis, Pengornis, Eocathayornis; ). They are vaulted and oval in shape. The parietals are smaller and rostrocaudally shorter than the frontals. In Neuquenornis, the medial portion of both parietals contributes to a dome-like structure located between the interfrontal suture and the central occiput. The parietals of Neuquenornis also form a gentle nuchal crest along their margin with the occiput.
Figure 5 Basicrania: A, the Early Cretaceous Shenqiornis mengi, DNHM D2951; B, the Late Cretaceous Neuquenornis volans, MUCPv 142. Abbreviations: boc, basioccipital; bt, basal tubera; cerebral prominence; eoc, exoccipital; fm, foramen magnum; fov, foramen for the external occipital vein; nh, canals for the hypglossus nerve; oc, occipital condyle; ocoe, ostium canalis ophthalmici externi; pop, paraoccipital process; soc, supraoccipital.
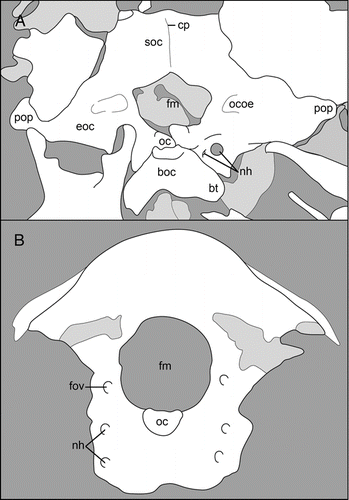
Supraoccipital
This bone is completely fused to the exoccipital bones in all known specimens (e.g. Neuquenornis, Hebeiornis and Shenqiornis), thus the precise lateral margin of this bone is unclear. The midline is vaulted, forming a cerebral eminence that is most prominent in Shenqiornis (). In this taxon the dorsal margin of the supraoccipital is horizontal, forming right angles with the lateral margins. The supraoccipital forms the dorsal margin of the foramen magnum. In Shenqiornis this forms a ventrally obtuse angle that defines the dorsal margin of a pentagonal foramen magnum, a feature currently an autapomorphy of this taxon (; CitationWang et al. 2010).
Exoccipital
The exoccipitals may (e.g. Neuquenornis) or may not (e.g. Hebeiornis, Shenqiornis) be fused to the basiocciptal. In Shenqiornis, the exoccipitals form the lateral and complete ventral margin of the foramen magnum; the medial margins slope medioventrally, giving the foramen magnum a pentagonal shape (). The exoccipitals bear mediolaterally long excavations on either side of the foramen that shallow laterally, and based on location, may possibly be homologous to the external opening of the ophthalmic canal (ostium canalis ophthalmici externi) of modern birds (CitationBaumel & Witmer 1993). In the same location, Neuquenornis possesses a foramen interpreted as for the external occipital vein (; CitationChiappe & Calvo 1994). In Shenqiornis, the exoccipitals extend laterally, forming long and slightly tapering paraoccipital processes.
The dorsal half of the occipital condyle is formed by the exoccipitals in Shenqiornis; they contact on the midline but remain completely unfused, each forming approximately a quarter of the condyle (; CitationWang et al. 2010). In Hebeiornis, the basioccipital accounts for a majority of the occipital condyle; the exoccipitals each bear a small flange that contributes to a small portion of the margin of the foramen magnum and possibly the occipital condyle (CitationZhang et al. 2004).
Ventral and lateral to the occipital condyle in Neuquenornis there is a vertical depression that contains two foramina, one located above the other (; CitationChiappe & Calvo 1994). Between the basioccipital and right exoccipital in Shenqiornis lies a bone fragment with two foramina; this bone is interpreted as a piece of the exoccipital, and defines a concave margin between the paraoccipital processes and the basioccipital (). The larger of the two foramina is circular; the second, much smaller, elliptical foramen is located just medial to the former. The openings in both taxa are interpreted as the canals for the hypoglossus nerve; their position in the exoccipital is consistent with that of modern birds. A similar location for the hypoglossus nerve is reported in Gobipteryx (ZPAL-MgR-I/33; CitationElzanowski 1981).
Basioccipital
This bone varies in degree of fusion with other occipital bones and degree of contribution to the occipital condyle. The basioccipital is unfused to the exoccipitals in Hebeiornis and Shenqiornis, but fully fused in Neuquenornis. The dorsal-most portion of this bone contributes to the occipital condyle in all known taxa (e.g. Hebeiornis, Shenqiornis), as in modern birds (CitationZusi 1993). Ventral to the occipital condyle, the basioccipital expands giving this bone an overall trapezoidal appearance (e.g. Hebeiornis, Shenqiornis). The basioccipital forms ventrolaterally projecting basal tubera which define a strongly concave ventral margin (e.g. Shenqiornis; ).
Foramen magnum and occipital condyle
The foramen magnum () is mediolaterally wider than it is dorsoventrally tall (e.g. Hebeiornis, Shenqiornis). Its shape ranges from round (e.g. Neuquenornis) to pentagonal (e.g. Shenqiornis). The foramen magnum is much larger than the occipital condyle (e.g. Neuquenornis, Hebeiornis, Shenqiornis) but the exact proportion varies widely from three (i.e. Shenqiornis) to eight times the size of the latter (i.e. Neuquenornis). Although the exact proportion cannot be measured, the Early Cretaceous holotype of Hebeiornis displays a relatively small foramen magnum compared to Late Cretaceous enantiornithines and modern birds.
Palatal bones
The only information available on the enantiornithine palate comes from Gobipteryx (CitationElzanowski 1995; CitationChiappe et al. 2001). In the palate, the premaxilla does not contact the rostral end of the fused vomers, which are instead contacted by the maxilla. This results in a horseshoe-shaped rostral opening that connects the palatal vault and oral cavity. Just caudal to this opening lies the choana, below the caudal margin of the nares, rostral to its position in modern birds. The fused vomers form a slender rod-like element forming the palatal midline; rostrally it contacts the maxilla and the palatine, a paddle-shaped bone. The pterygoids are robust, forked rostrally and do not contact the vomers (CitationChiappe et al. 2001). An Archaeopteryx-like ectopterygoid has been suggested for Gobipteryx by CitationElzanowski (1995). A possible ectethmoid has also been identified in this taxon (CitationChiappe et al. 2001).
Cranial fenestrae
The external nares are typically elliptical and longer than the antorbital fenestra, and the orbit is the largest cranial opening, as in modern birds. The antorbital fossa is filled by a large fenestra in most taxa (e.g. Shenqiornis, Rapaxavis, Alethoalaornis). The external nares and antorbital fenestra are not separated by a large space, so the caudal margin of the external nares usually approaches and sometimes overlaps the rostral margin of the antorbital fenestra (, ). Maxillary fenestrae homologous to those of non-avian theropods appear to be absent, although the homology of an accessory fenestra in the maxilla of Pengornis remains uncertain. An enclosed supratemporal fenestra is known in some taxa (e.g. Shenqiornis, LP 4450, Pengornis). Likewise, a fully separated infratemporal fenestra may have existed in at least one taxon (i.e. Shenqiornis), although this fenestra appears to be partially connected to the orbit in most (e.g. Pengornis, LP 4450). Additional pneumatic foramina are known to occur (e.g. Pengornis).
External nares
The nares vary in the bones that form them, position and shape. The nares are typically holorhinal, rostrocaudally elongate and elliptical (e.g. Eoenantiornis, C. yandica). The opening is bounded rostrally, rostrodorsally and typically rostroventrally by the premaxilla; in some longipterygids it appears that the majority of the ventral margin of the nares is formed by the maxilla. The nasals form the dorsal margin of the nares and, depending on the development of their maxillary process, contribute to the caudodorsal and caudal margins (e.g. Eoenantiornis, Gobipteryx, C. yandica, DNHM D2889). The maxilla forms the caudoventral margin and contributes to the caudal and caudodorsal (e.g. Shenqiornis, Rapaxavis) margins of the nares in varying degrees depending on the morphology of the maxilla-nasal contact. In most taxa, the nares are contained within the rostral third of the skull. An exception is represented by the longipterygids in which the nares are retracted and appear to be schizorhinal (–C, E, F). The latter condition is also known outside the longipterygids (e.g. Shenqiornis; ). The proportions between length and height vary among taxa. Typically, the nares are elliptical—the length is several times the height—but in the retracted nares of the Late Cretaceous Gobipteryx these two dimensions are subequal (, M). The shape of the longipterygid nares is difficult to ascertain due to poor preservation but it appears that these openings are very narrow and slit-like (–C, E, F).
Antorbital fossa
In most taxa, the antorbital fossa appears to be entirely excavated by a large antorbital fenestra (e.g. Shenqiornis, LP 4450); however, in at least two specimens (e.g. Pengornis, DNHM D2889) the fossa is rostrally lined by a recessed medial wall of the maxilla—in Pengornis, the antorbital fenestra is approximately two-thirds the size of the antorbital fossa (). The antorbital fossa and fenestra are typically triangular with subequal length and height. The relative contribution of the maxilla, nasal and lacrimal to the antorbital fossa is unclear for most taxa. In Pengornis and Shenqiornis, the maxilla forms the rostral margin and rostral half of the dorsal margin; the lacrimal forms the remaining half of the dorsal margin, thus excluding the nasal from participating in the fossa. However, in Gobipteryx, the maxillary contribution is limited to a small rostroventral corner of the fossa, while most of the rostral and possibly dorsal margins are formed by the nasal (the lacrimal contribution remains unknown; , M). The jugal contributes to the caudoventral corner of the antorbital fossa in LP 4450 (). In Pengornis, the maxilla is pierced by a single accessory foramen (). The foramen is small and elliptical, located in the rostrodorsal corner of the fossa; its size and placement suggest it may not be homologous to either of the accessory fenestrae (maxillary and promaxillary) of Archaeopteryx and non-avian theropods, which are more ventrally located (CitationElzanowski 2002; CitationNorell & Makovicky 2004).
Temporal fenestrae
The preservation of a T-shaped postorbital suggests the presence of a fully demarcated supratemporal fenestra formed by the frontal, parietal, postorbital and squamosal (e.g. Pengornis, Shenqiornis, LP 4450). The elongate jugal process of the postorbital of at least one specimen (i.e. Shenqiornis; ) further suggests a completely enclosed infratemporal fenestra, and thus a fully diapsid skull, may have been present in at least one taxon. However, the reduced postorbital of other specimens indicates that in most taxa the infratemporal fenestra converged with the orbit (e.g. Pengornis, LP 4450). The elongate postorbital bone in Shenqiornis is disarticulated, making it difficult to determine with absolute certainty if this bone fully separated the infratemporal fenestra from the orbit, especially given the absence of a postorbital process on the jugal or a preserved quadratojugal (, ). The jugal process of the postorbital in this specimen is very long (more than three times the length of the proximal rami), suggesting the postorbital would have been capable of forming a completely delimited infratemporal fenestra. Based on the position of the postorbital in articulated specimens, if the postorbital were to contact a bone ventrally in Shenqiornis, it likely be the medial or lateral surface of the jugal, a very different articulation from the long overlapping contact between the postorbital and postorbital process of the jugal in non-avian theropods and some other basal birds (e.g. Confuciusornis; CitationChiappe et al. 1999).
Additional fenestrae
In Pengornis the nasal and lacrimal form a small fenestra (, ). This feature, not preserved in any other enantiornithine, is inferred to be pneumatic based on the extensive pneumaticity that characterizes the nasolacrimal region of birds and non-avian theropods (CitationWitmer 1997). Pengornis also possesses an additional small rostrocaudally elongate oval foramen that pierces the nasal at the level of the rostral margin of the antorbital fenestra.
Mandible
There is a general lack of fusion between the dentary and postdentary bones, and the dentaries are typically not fused into a mandibular symphysis; the only specimen with fusion between mandibular bones is from the Late Cretaceous (e.g. Gobipteryx ZPAL-MgR-I/12; CitationElzanowski 1977). The degree of mandibular fenestration is unclear, but all known adult mandibles are imperforate.
Dentary
The dentary varies in morphology and dentition. This bone is most commonly unfused, toothed, and straight with an unforked ventrally sloping caudal articulation with the surangular (e.g. Longipteryx, C. yandica, Alethoalaornis, Shenqiornis). Caudally, the ventral margin of the dentary often expands ventrally at the level of the lacrimal while the dorsal margin remains straight (e.g. Hebeiornis, Shenqiornis, Eocathayornis). In the longipterygids Longirostravis, Rapaxavis and Longipteryx, the dentary is overall slightly concave ventrally (CitationHou et al. 2004; CitationMorschhauser et al. 2009; CitationO’Connor et al. 2009). Gobipteryx reportedly differs from Early Cretaceous enantiornithines in that the mandibular bones are nearly completely fused (dentaries strongly ankylosed in IGM -100/1011; CitationChiappe et al. 2001) and the caudal articulation of the dentary is forked, as in more advanced birds (CitationElzanowski 1977). These specimens however, reportedly have undergone some diagenetic recrystallization, which may have obscured sutures and other interosseous connections (CitationElzanowski 1977).
Dentary teeth range from absent (i.e. Gobipteryx) to rostrally restricted (e.g. Longipterygidae) to present throughout (e.g. Pengornis, C. yandica, Shenqiornis). The number of teeth ranges from low in the longipterygids to 6–7 in the typical enantiornithine (e.g. Hebeiornis, Shenqiornis), with an estimated 13 teeth marking the known upper limit (i.e. Pengornis; CitationZhou et al. 2008).
Surangular
Usually unfused to the dentary, this bone is typically shorter than the dentary, robust and straight (e.g. Rapaxavis, Hebeiornis, DNHM D2510/1). The articulation with the dentary is long and slopes rostrodorsal—ventrocaudally so that the rostral third of the surangular tapers to a blunt tip rostrodorsally (e.g. Dapingfangornis, Longipteryx, Protopteryx). No coronoid bone is known but Gobipteryx ZPAL-MgR-I/12 and Rapaxavis possess a rounded coronoid process on the dorsal margin of the surangular ().
Angular
Often difficult to differentiate from possible hyoid bones (e.g. Rapaxavis, Longirostravis), the angular appears to be a thin, straight and rod-like element that forms the ventrolateral margin of the postdentary portion of the mandible (e.g. Shanweiniao, DNHM D2567/8). The angular is slightly curved so that it is convex ventrally in some taxa (e.g. Hebeiornis, Longipteryx). The rostral end is wider than the caudal end in lateral view (e.g. Hebeiornis, Longipteryx, DNHM D2567/8).
Splenial
This bone is usually not visible but in a few specimens it is unfused and disarticulated into view (e.g. Hebeiornis, Dapingfangornis). In Hebeiornis the bone is more than half the length of the dentary. The ventral margin of this bone is slightly concave ventrally. The dorsal margin defines the apex of an obtuse triangle; the rostral ramus is twice the length of the caudal ramus and tapers more sharply. In Archaeopteryx the rostral and caudal rami are closer to subequal in length, although the rostral ramus is still longer (CitationElzanowski 2002).
Prearticular and articular
Preserved in Hebeiornis, the prearticular is rostrally lancet-shaped (rostral ramus is tapered sharply) as in Archaeopteryx (CitationElzanowski 2002), differing from the rounded margin in neornithines (CitationZhang et al. 2004). The articular is preserved in LP 4450 but no information can be discerned.
Mandibular fenestrae
Completely perforated rostral and caudal mandibular fenestrae are only known in LP 4450, although since the specimen is a juvenile it is difficult to ascertain to what degree this is the result of incomplete ossification (CitationSanz et al. 1997). In this specimen, the fenestrae are elongate ovals (). The rostral fenestra retains the ancestral condition in which the ventral margin is formed by the angular, as in non-avian dinosaurs (as opposed to the dentary of modern birds; CitationSanz et al. 1997). The caudal mandibular fenestra in LP 4450, which is largely enclosed by the surangular with a rostroventral contribution from the angular, is inferred to be homologous to the surangular foramen of non-avian theropods (CitationSanz et al. 1997; CitationWeishampel et al. 2004). In Gobipteryx (ZPAL-MgR I/12), a small, depressed area on the rostral-most surangular is reported to correspond to the rostral mandibular fenestra while the caudal fenestra is reduced to a small foramen (CitationElzanowski 1977); the extent to which the alleged rostral fenestra perforates the mandible is unclear. GMV-2158 also possesses a single fully perforate caudal (surangular) fenestra (CitationChiappe et al. 2007); the mandibular fenestra of this juvenile is relatively smaller than that of LP 4450 and round. Given that the only two specimens with fully perforate mandibular fenestrae are juveniles (CitationChiappe et al. 2007; CitationSanz et al. 1997), the absence of mandibular fenestrae in specimens of adult enantiornithines could suggest their presence in these specimens reflects their ontogenetic stage. However, similar structures are absent in other juvenile specimens GMV-2159 and GMV 2156/NIGP-130723 (CitationChiappe et al. 2007).
Discussion
Enantiornithines display a unique mosaic of primitive and derived characters, reflecting their intermediate phylogenetic position between the basal-pygostylians and modern birds, and the scale of their Cretaceous evolutionary radiation (an alternative phylogenetic proposal for Enantiornithines—sharing a most common recent ancestor with Archaeopteryx [Martin Citation1983, Citation2004]—has been shown to be less parsimonious by phylogenetic analyses over the last two decades [e.g. CitationChiappe 1995a, 2002; CitationClarke and Norell 2002; CitationO’Connor et al. 2009]). However, the pattern of character loss and/or plesiomorphic retention in certain areas of the skeleton is intriguing. Within the skull, the lack of derived characters relative to more basal birds such as Archaeopteryx is most notable. Instead, in many details the skull bears strong similarity to this basal taxon; enantiornithines retain teeth, a postorbital bone, a quadrate with a single headed otic process, bicondylar mandibular process, and a broad orbital process, a complete osseous bar separating the orbit and antorbital fenestra, a small premaxilla, a squamosal unincorporated into the braincase, long paraoccipital processes, unfused skull bones, and the apparent absence of kinetic features. All these features are modified in modern birds. The absence of teeth and presence of a caudally forked dentary—features otherwise common to modern birds and present in the basal ornithuromorph Apsaravis ukhaana (CitationClarke & Norell 2002) and in the basal pygostylian Confuciusornis sanctus (CitationChiappe et al. 1999)—are only known in a single Late Cretaceous taxon (i.e. Gobipteryx), suggesting an overall trend towards more advanced morphologies in at least one lineage.
The absence of anatomically modern features in the skull of the Early Cretaceous enantiornithines is not unique to this one avian lineage, but plesiomorphic for basal birds. Sapeornithids and confuciusornithids, basal pygostylians also known from the Jehol Biota, share the presence of a postorbital, a squamosal unincorporated into the braincase, absence of element fusion, and an unforked dentary (forked in Confuciusornis); both these groups, however, have either reduced (e.g. Sapeornis) or lost (e.g. confuciusornithids) their dentition. Even the skulls of some sympatric Jehol basal ornithuromorphs (e.g. Yanornis martini, Hongshanornis longicresta; Zhou & Zhang Citation2001, Citation2005) are fairly primitive and highly unfused, having small premaxillae, teeth, and unforked dentaries, though no ornithuromorph is known to retain a postorbital bone and the skulls of ornithothoracine taxa are considerably less massive than those of basal taxa such as Jeholornis, Sapeornis and Confuciusornis. The absence of derived features in the skulls of early birds with advanced flight features, such as an alula, strut-like coracoid, and reduced manual digits, emphasizes that the refinement of powered flight dominated early avian evolution (CitationChiappe 1995b). Despite the conservative nature of enantiornithine skull morphology relative to more basal taxa and the absence of detailed morphologies preserved among a large number of specimens, variation within the group can be discussed at the level of skull shape and fenestration. Enantiornithine dentition and trophic specialization are also diverse relative to other sympatric avian clades and are discussed below.
Fusion
The extent of fusion between the bones of the skull varies widely between known enantiornithines; however, the degree to which this is an ontogenetic artefact is largely unknown. Given the absence of good growth series within the clade, it cannot be determined if the absence of fusion between two elements is due to incomplete growth, or represents a true feature of the species. This is further complicated by our poor understanding of enantiornithine developmental strategy and ontogeny (CitationChinsamy & Elzanowski 2001; CitationCambra-Moo et al. 2006). The complete fusion of the basicranium (parietal and frontal fusion incomplete) in Neuquenornis may either represent the derived state, or the incomplete fusion observed in the basicrania of the holotype specimens of Shenqiornis and Hebeiornis may not be a true morphological state but the result of incomplete growth at the time of death, despite the adult appearance of the specimens based on their size, ossification of periosteal surfaces, and skull proportions.
Despite this, there is no doubt that the few Late Cretaceous skull specimens available show greater degrees of premaxillary, basicranial and mandibular fusion compared to Early Cretaceous specimens, given that the only definitively known fused premaxillae, basicranium and mandible are all known from the Late Cretaceous.
Fenestration
The main cranial fenestrae in enantiornithines are very similar to those of Archaeopteryx, with large external nares and orbits as in modern birds, rather than the small external nares (relative to the antorbital fenestra) of most non-avian theropods (CitationCurrie & Chen, 2001; CitationNorell & Makovicky 2004; Gölich & Chiappe 2006). The antorbital fossa of Archaeopteryx, Dalianraptor and closely related non-avian theropods typically possesses two small foramina (promaxillary and maxillary fenestrae) in addition to the larger antorbital fenestra (CitationElzanowski 2002; CitationNorell & Makovicky 2004; CitationGao & Liu 2005). This condition is unknown among enantiornithines, although a single additional foramen within the antorbital fossa is known to occur in Pengornis (). Basal pygostylians Sapeornis and Confuciusornis also possessed an accessory maxillary foramen, but this feature is unknown in birds more advanced than enantiornithines. In Confuciusornis, the round shape of the foramen and its position suggest it may be the maxillary fenestra of non-avian theropods, although this homology is uncertain (CitationWitmer 1997; CitationChiappe et al. 1999). Preservation in Sapeornis is unclear and it cannot be determined if one or two accessory foramen are present, nor can their possible homology be determined. The rostrodorsal position of the accessory foramen in Pengornis suggests that, unlike Confuciusornis, this feature may not be homologous to the accessory maxillary fenestrae in the antorbital fossa of Archaeopteryx and non-avian theropods. Although only identifiable in a single exceptionally well preserved specimen, the ‘accessory foramen’ present in Pengornis indicates that, like in modern birds (CitationWitmer 1997; CitationWitmer & Ridgely 2008), enantiornithines had evolved additional pneumatic features. Given the poor preservation of the maxilla in most specimens and the thinness of the recessed bone that lines the antorbital fossa, this feature may have a wider distribution within the clade.
Modern birds, having lost their postorbital bone, no longer possess fully isolated supra- and infratemporal fenestrae. Basal birds like Confuciusornis that possess a diapsid skull are considered to have secondarily derived the primitive condition (CitationChiappe et al. 1999), an inference supported by the presence of a reduced postorbital in Archaeopteryx that does not contact the jugal as in non-avian maniraptoran dinosaurs (CitationElzanowski 2002). While the postorbital is a bone rarely preserved, in enantiornithines it also appears to be typically reduced (e.g. Pengornis, LP 4450). One specimen (DNHM D2950/1, the holotype of Shenqiornis) preserves a large T-shaped bone interpreted as the postorbital based on its morphology; however, the caudal half of the skull, including the presumed postorbital, is disarticulated and our interpretation is not unequivocal (, ). The large size of the presumed postorbital of Shenqiornis suggests not only that a supratemporal fenestra may have been present in this enantiornithine taxon (also inferred for Pengornis and LP 4450), but that this bone may also have completely enclosed an infratemporal fenestra. However, the jugal morphology in this specimen suggests that if a fully delimited infratemporal fenestra was present, the bone interpreted as a postorbital would have contacted the medial or lateral surface of the jugal bar, a different configuration from the long articulation of the postorbital and postorbital process of the jugal in non-avian theropods and confuciusornithids. This supports the hypothesis that the condition in Shenqiornis is also secondarily derived. Alternatively, the presence of a postorbital with an elongate jugal ramus in Sapeornithidae, Confuciusornithidae and one enantiornithine (i.e. Shenqiornis), as well as in non-avian theropods (CitationOstrom 1969; CitationClarke et al. 2002; CitationXu et al. 2002), may suggest that a large postorbital is the plesiomorphic avian condition with the postorbital in Archaeopteryx being reduced during the evolution of this lineage. As the phylogenetic position of the known pre-pygostylian taxa (e.g. Jeholornis, Rahonavis, Zhongornis) becomes better understood, we may be able to differentiate between these two hypotheses.
Skull geometry
The great diversity of skull morphologies of modern birds has been subdivided into two general geometric types: extended and flexed (CitationMarinelli 1928; CitationVan der Klaauw 1948). While skull geometry is associated with the position of the brain, rotated in flexed skulls, it can be determined osteologically from the position of the foramen magnum. In extended crania the skull units (i.e. rostrum, orbit, braincase) are aligned in lateral view and the foramen magnum is directed caudally; in flexed skulls, the units are decoupled, not limited to the same plane in lateral view, and the foramen magnum is ventrally located (CitationMarugán-Lobón & Buscalioni 2006). The avian skull is incredibly diverse in form and proportion; however, a number of morphologies are limited to the flexed type of skull, leading to the hypotheses that this specific design is derived within Aves and that the evolutionary decoupling of the skull units within the group facilitated the evolution of a wider range of morphologies (CitationMarugán-Lobón & Buscalioni 2006). The rotation of the brain characteristic of many modern lineages probably occurred after the ornithothoracine split, within the lineage moving towards modern birds. Currently there is no evidence that enantiornithines or any other basal bird developed a flexed cranial geometry; all three preserved enantiornithine basicrania—belonging to taxa of substantially different size, morphology and geological age (i.e. Hebeiornis, Neuquenornis, Shenqiornis)—possess caudally directed foramina magna (). Taxa in which the skull is well preserved in lateral view all show the skull units aligned in a single plane (e.g. Longipteryx, Shenqiornis, Protopteryx, Pengornis; –), suggesting that during the evolution of Enantiornithes the brain remained un-rotated. This conclusion is equivocal, based on the limited information available, but even without the rotation of the brain, the range of morphological variation observed in modern birds was not impossible for enantiornithines to achieve. However, if enantiornithines did not develop flexed type skulls, the development of morphologies comparable to those of modern flexed type skulls would probably have involved developmental pathways different from those in living birds.
Figure 6 Ternary diagram showing the relative proportions of skull units among Enantiornithes, relative to Neornithes. Abbreviations: B, braincase; O, orbit; R, rostrum.
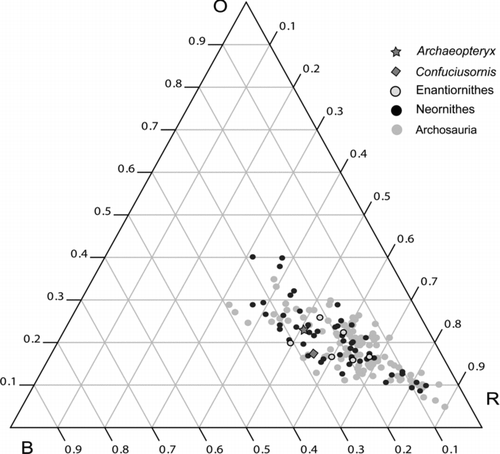
Several enantiornithines were measured and added to the archosaur skull geometry morphoset of CitationMarugán-Lobón & Buscalioni (2003) in order to determine how the clade compares to neornithines (modern birds) and other archosaurs (). The range of enantiornithine cranial morphospace is based on a sample size of only five taxa (several of which are longipterygids, thus the sample size is biased towards longer rostra). Given the preservation of fossil specimens (disarticulated, compressed, damaged and/or incomplete), it is impossible to measure as accurately as with modern specimens. Thus the measurements used () are considered best estimates, even in the most exceptionally preserved specimens such as the holotype of Pengornis. Consequently, it is premature to make detailed inferences about variation and proportions and these results will have to be explored further when a larger number of well-preserved specimens are available. Nevertheless, all five enantiornithines fall approximately within the known range of avian mesorostrine morphospace—the only slight outlier is the poorly preserved Protopteryx (IVPP V11665). Archaeopteryx (measurements taken from the juvenile Eichstatt specimen) and Confuciusornis (GMV 2132) were plotted in order to determine whether basal bird skull geometry is conservative; the two taxa plot distinct from each other and enantiornithines. Archaeopteryx and Confuciusornis differ in that the latter has a smaller orbit, but both taxa fall within the range of extant mesorostrine birds (this difference is exaggerated due to the juvenile status of the Eichstatt Archaeopteryx, resulting in a proportionately larger orbit in this specimen). Enantiornithines form a cluster in the centre of the archosaur spectrum, and vary in proportions of all skull units, not just the rostrum. The braincase and orbit show the most variation; however, these dimensions are much more difficult to measure than the rostral length and this variation is considered an artefact of poor preservation.
The rostrum is the most variable skull unit within Theropoda, and the same holds true for avian as well as non-avian taxa (CitationMarugán-Lobón & Buscalioni 2003). Such a wide range of rostral morphological variation has been explained by greater functional constraints on the orbit and the postorbit (to house and protect the eye and brain) as opposed to those acting on the rostrum, which has a greater freedom to respond to selective pressures and to evolve a wide range of morphologies associated with specialized trophic function (CitationMarugán-Lobón & Buscalioni 2003). Following the terminology for rostral proportions set forth by CitationBusbey (1995) and maintained by CitationMarugán-Lobón & Buscalioni (2003), the archosaur skull is broken into three categories according to rostral proportions: brevirostrine (rostrum 30–50% of total skull length), mesorostrine (50–70%) and longirostrine (70–90%).
Archaeopteryx, Confuciusornis and the enantiornithines fall in the mesorostrine range (), suggesting that this condition is basal within Aves, as in all Archosauria (CitationMarugán-Lobón & Buscalioni 2003). Though few skulls are preserved well enough to be measured accurately, observations and measurements indicate that enantiornithines typically possess skulls that are approximately 50% rostrum. The longipterygid clade represents an enantiornithine incursion into new morphospace, with rostral proportions approaching and exceeding 60% skull length. Currently, there are no enantiornithine or other Mesozoic avians unequivocally known to have possessed a brevirostrine or true longirostrine skull, suggesting that brevirostrality and longirostrality are derived conditions within Aves.
Dental variation
A majority of enantiornithine taxa, for which cranial material is known, possess teeth (, , ), except the Late Cretaceous Gobipteryx minuta (, M; CitationElzanowski 1974). Taxa vary considerably in the morphology of their teeth, their size, distribution, number, and where they are located. With the exception of LP 4450 (CitationSanz et al. 1997), all specimens used in this discussion are from the Early Cretaceous Jehol Group. Even within this single geological unit, a diversity of dental morphologies can be recognized. Teeth are typically similar to those of Archaeopteryx (CitationElzanowski 2002): simple, conical, slightly constricted at the base, recurved, and without serrations (e.g. Hebeiornis, C. yandica, Eocathayornis, LP 4450). The degree of caudal curvature is relatively low, with the tip of the tooth located just caudal to the midpoint of the alveolus (e.g. C. yandica, Pengornis, GMV 2158, Shenqiornis). In taxa in which teeth are more strongly recurved, the tip reaches the caudal margin of the tooth socket (i.e. Eoenantiornis; , B), and in extreme taxa, projects beyond it (i.e. Longipteryx; –L).
Figure 7 Enantiornithine teeth, photographs and interpretative drawings: A, B, Eoenantiornis buhleri, IVPP 11537; C, D, Shenqiornis mengi, DNHM D2951; E, F, Pengornis houi, IVPP 15336; G, H, Longirostravis hani, IVPP V11309; I, J, Longipteryx chaoyangensis, IVPP V12552; K, L, Longipteryx sp., DNHM D2889.
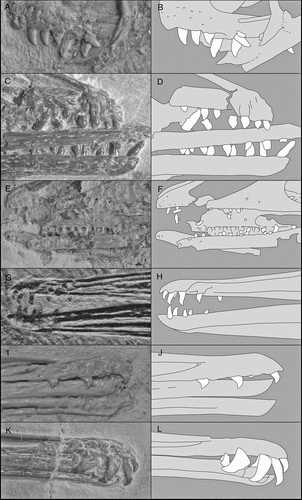
Teeth range in size from very small, reduced peg-like teeth (e.g. Longirostravis, , H) to the large, robust teeth of Shenqiornis and Longipteryx (–L). Tooth size relative to body size also varies considerably; the teeth of Longirostravis (c. 0.45–0.5 mm; , H) are nearly the same size as those of Pengornis (c. 0.55–0.65 mm; , F), despite the fact that the latter specimen is several times the size of the former. The teeth of Shenqiornis are among the largest and most robust currently recognized within enantiornithines; fat with circular cross sections (, D), they taper rapidly into a slightly caudally deflected tip. The teeth of Shenqiornis represent one extreme within the clade; another extreme is exemplified by the numerous low crowned teeth of Pengornis (, F). It has been suggested that the teeth of this specimen bear wear facets and evidence for primitive-style tooth replacement, with teeth replaced throughout the organism's life span (CitationZhou et al. 2008). This detail is difficult to verify and could equally represent heterodonty or differential preservation. Currently, despite the large number of known toothed taxa, there are no known enantiornithines that clearly preserve a resorption pit or newly erupting replacement teeth, therefore evidence for dental replacement remains elusive.
Trophic specialization
The diversity of recognized dental patterns, such as in the longipterygids, which possess rostrally-restricted dentition, suggests that a great deal of trophic specialization evolved within Enantiornithes. Stomach contents are known from two specimens, the holotypes of Eoalualvis and Enantiophoenix (CitationSanz et al. 1996; CitationDalla Vecchia & Chiappe 2002; CitationCau & Arduini 2008). Eoalualvis preserves the remains of arthropods associated with the visceral region, while Enantiophoenix is associated with bits of amber scattered among the skeletal elements, and is interpreted as a sap-eater (CitationDalla Vecchia & Chiappe 2002). Neither preserves any skull material, thus morphologies of the rostrum and teeth associated with such diets cannot be determined. No enantiornithine is known to preserve gastroliths, which have been found in a number of sympatric ornithuromorphs (pers. obs.; CitationZhou et al. 2004; CitationZhou & Zhang 2006b).
Without stomach contents, dietary inferences for fossil taxa are weak; however, the following interpretations are based on tooth morphology and observations of related taxa. The teeth of Archaeopteryx are interpreted as too weak for crushing hard foods, and the taxon is interpreted as a general insectivore, limited to small arthropods with softer cuticles (CitationElzanowski 2002). The tooth morphology in a number of enantiornithines is largely consistent with Archaeopteryx, but a number of taxa depart from this morphology, thus indicating specialization for a unique niche. Longipteryx, Pengornis and Shenqiornis represent the most extreme deviations from the typical tooth morphology within the clade (). The teeth of Shenqiornis are relatively the largest and most robust known within Enantiornithes (, D); this taxon is interpreted as having a duraphageous diet, utilizing food items unavailable to other birds of a similar size. Longipterygids all possess rostrally restricted dentition, and most taxa bear very small, reduced teeth (, H); Longirostravis has been suggested to be a mud-prober, using its delicate rostrum to retrieve worms and other soft-bodied organisms from the littoral substrate (CitationHou et al. 2004). The teeth of Longipteryx are large, recurved, and slightly laterally compressed, features associated with increased predatory capability (–L). The rostral restriction of the teeth and their morphology indicates that Longipteryx may have been piscivorous. The numerous small teeth of Pengornis are blunt, brachydont, and may bear wear facets (, F; CitationZhou et al. 2008); low-crowned teeth, however, are inconsistent with a diet of abrasive food items. The morphology of the teeth of Pengornis is more consistent with a diet of soft-shelled arthropods, which were abundant in the Jehol lake system (CitationChang et al. 2003).
Cranial kinesis
The absence of a complete jugal-postorbital bar in some enantiornithine taxa indicates that cranial kinesis was at least possible. Without jugal and postorbital contact, the jugal is free to rotate forward and elevate the skull as in modern birds (CitationZusi 1984; CitationBühler 1987; CitationBock 2002). Osteologically, kinesis can be determined by identifying thinned regions of bone, representing bending zones, or true mobile hinges such as a frontal-nasal hinge, where the movement occurs. All modern birds possess some form of cranial kinesis and are able to move their upper jaw with respect to the lower jaw to some degree (CitationZusi 1984). Among modern taxa there are many forms of kinesis (amphikinesis, prokinesis and rhynchokinesis) which reflect where the bending occurs within the rostrum (CitationZusi 1984). These different types of kinesis are associated with different specialized trophic functions; for example, rhynchokinesis (rostrally located bending zone) is common among (although not limited to) the longirostrine shore birds (e.g. Scolopacidae, sandpipers and their relatives) and aids the removal of food items from the substrate as well as the capture of planktonic prey (CitationZusi 1984; CitationEstrella & Masero 2007).
It has been observed within Neornithes that all schizorhinal skulls are rhynchokinetic, though not all rhynchokinetic skulls are schizorhinal (CitationZusi 1984). Longirostravis, a purported mud-prober (CitationHou et al. 2004), has schizorhinal nostrils, as does the closely related Rapaxavis; together with the absence of a preserved postorbital in these specimens, it is possible that the skulls of these taxa may have been capable of rhynchokinesis (, B). If so, given that rhynchokinesis is generally considered to be derived among modern birds, such a condition must have evolved independently from that in neornithines. Unfortunately, the incomplete and 2D preservation of these taxa and most other enantiornithine specimens makes it impossible to determine if bending zones were present. No cranial hinges have been reported or observed in any enantiornithine. The nature of the postorbital-jugal contact in taxa such as Shenqiornis is currently unknown but future discoveries may prove that some enantiornithines may have had limited (if any) kinetic potential in the skull, as in the confuciusornithids (CitationChiappe et al. 1999).
Evolutionary trends
The Late Cretaceous record of enantiornithine skull material is extremely limited compared to that of the Early Cretaceous; as a result, any inferences made regarding trends in enantiornithine evolution through time are inherently weak. Despite this, the obvious trends should be noted. An increase in the degree of fusion between skull bones is apparent, evidenced by the highly fused premaxillae of Gobipteryx, although greater information on the ontogeny of enantiornithines may affect this hypothesis. The complete loss of teeth within Enantiornithes is only known in a Late Cretaceous taxon (i.e. Gobipteryx), but occurred much earlier in other lineages of Mesozoic birds (e.g. Confuciusornithidae, Ornithuromorpha; CitationHou et al. 1995; CitationZhou & Zhang 2006a). The foramen magnum of the Late Cretaceous Neuquenornis is proportionately much larger than the occipital condyle relative to Early Cretaceous enantiornithines (), a derived feature of modern birds that also independently arose in Late Cretaceous non-avian theropods such as alvarezsaurids (CitationChiappe et al. 2002). The premaxilla is enlarged in Gobipteryx relative to Early Cretaceous taxa, and the maxilla is reduced, both morphologies characteristic of modern birds (, M; CitationZusi 1993). An enlarged premaxilla is also present in the highly modified skull of confuciusornithids (CitationChiappe et al. 1999), although absent in basal ornithuromorphs indicating convergence between these three clades. The increased fusion, loss of teeth, greater ratio of foramen magnum to occipital condyle, and greater premaxilla/maxilla ratio can all be considered derived features, consistent with morphologies present in modern birds. Thus despite the extremely fragmentary information available for Late Cretaceous taxa, a weak, general trend from more basal to derived features is observed during enantiornithine evolution—a trend that given the phylogenetic position of Enantiornithes is interpreted as having developed in parallel to Ornithuromorpha. This trend is also observed in the postcrania, with an increase in size and degree of fusion in compound bones such as the tarsometatarsus in the Late Cretaceous (CitationChiappe & Walker 2002). The well-sampled Jehol avifauna, however, indicates that by the Early Cretaceous, enantiornithines were already incredibly diverse in form and function.
Conclusions
The enantiornithine skull can be described as plesiomorphically unfused, retaining a small premaxilla, a postorbital bone, a squamosal not incorporated to the braincase, teeth, and a primitive quadrate with a single headed otic process and broad orbital process. The premaxilla, maxilla and nasal show considerable morphological variation, which results in a diversity of skull shapes, most notably the elongate skull of Longipterygidae; schizorhinal nostrils are also known to have evolved within this particular clade. Despite the current diversity of published skull material and numerous undescribed specimens, some areas of the enantiornithine skull remain unclear. The lacrimal, jugal and postorbital bones are poorly preserved in most specimens and the articulation between and degree of morphological variation within them cannot be determined from currently available specimens. The palatal complex and basicranium are preserved in only one or two specimens, thus variation between groups in the morphology of these regions is also incompletely known. Based on this morphological review, however, we offer updated reconstructions of the enantiornithine skull ().
Fossils from the Late Cretaceous are rare, but comparison with older specimens indicates a general trend towards more advanced morphologies (e.g. enlarged premaxilla, absence of teeth, increased fusion between elements) in stratigraphically younger taxa. Currently there is no evidence that this clade evolved the flexed type skull with ventrally oriented foramen magnum associated with unique skull shapes in many modern birds, or truly brevirostrine or longirostrine proportions. In parallel with groups of more advanced birds and some non-avian theropods, the enantiornithine skull developed pneumatic features absent in primitive birds, as evidenced by Pengornis.
The available skull material reveals abundant evidence of trophic specialization; a diversity of dental morphologies and patterns are recognized. The elongated rostrum of longipterygids is interpreted as trophic specialization for aquatic environments, and intraclade diversity suggests adaptation to a wide range of ecological niches. No specimen preserves a skull that can be clearly identified as possessing cranial kinesis; however, the reduced postorbital in most specimens, where preserved, suggests that this feature may have been present as it is in all modern birds.
In reviewing the enantiornithine skull, it is clear that the high level of evolutionary experimentation characterizing the transition from non-avian theropods to modern birds (CitationChiappe & Dyke 2007) also involved many skull morphologies. For example, nearly every basal bird between Archaeopteryx and Ornithothoraces has reduced dentition to some degree, yet only one Late Cretaceous enantiornithine is known to have possessed a beak. Instead a number of enantiornithines show teeth larger and more robust than those of Archaeopteryx. Likewise, enantiornithines show a number of skull morphologies more primitive than those present in more basal birds, such as the absence of a caudally forked dentary (a condition present in the more basal Confuciusornithidae). Overall, the enantiornithine skull is characterized by a large amount of morphological diversity but very few major apomorphies, with the skull remaining morphologically more comparable to non-avian theropods than to modern birds. This contrasts with the enantiornithine post-cranial skeleton, which shows numerous advancements over more primitive birds, e.g. a rostrocaudally elongate sternum and narrow furcula. This suggests that during the early evolution of Aves, while morphological experimentation did extend to the skull, this region lagged behind the post-cranial skeleton in terms of apomorphic change, with even basal ornithuromorphs retaining a skull that remains fairly conservative and similar to Archaeopteryx in morphology.
Acknowledgements
We are grateful to Stephanie Abramowicz for producing several of the illustrations for this paper. We thank Jesus Marugán-Lobón for his assistance with measuring and plotting the skull units and use of his dataset, Zhang Zihui for data provided through personal communication, and two reviewers for their useful comments on this manuscript. We also thank Zhou Zhonghe and Zhang Fucheng of the Institute of Vertebrate Paleontology and Paleoanthropology, and Gao Chunling of the Dalian Natural History Museum for access to specimens. Special thanks to Judy and Ron Perlstein and Lynn and Carl Cooper for additional funding and support. We thank David Bottjer of the University of Southern California for advising this project.
References
- Baumel , J. J. and Witmer , L. M. 1993 . “ Osteologia ” . In Handbook of Avian Anatomy: Nomina Anatomica Avium, Second Edition , Edited by: Baumel , J. J. , King , A. S. , Breazile , J. E. , Evans , H. E. and Vanden Berge , J. C. 45 – 132 . Cambridge : Nuttall Ornithological Club .
- Bock , W. J. 2002 . “ The evolution of avian cranial kinesis ” . In Proceedings of the 5th Symposium of the Society of Avian Paleontology and Evolution, Beijing, 1–4 June 2000 , Edited by: Zhou , Z-H. and Zhang , F. 191 – 201 . Beijing : Science Press .
- Busbey , A. B. 1995 . “ The structural consequences of skull flattening in crocodilians ” . In Functional morphology in vertebrate paleontology , Edited by: Thomason , J. 173 – 193 . Cambridge, Massachusetts : Cambridge University Press .
- Bühler , P. 1987 . “ On the mobility of the upper jaw and the segments of the braincase in the Mesozoic birds ” . In L’Evolution des Oiseaux d’apres le Temoignage des Fossiles , Edited by: Mourer-Chauviré , C. 41 – 48 . Lyon : Département des Sciences de la Terre, Université Claude-Bernard .
- Cambra-Moo , O. , Buscalioni , A. D. , Cubo , J. , Castanet , J. , Loth , M.-M. , de Margerie , E. and de Ricqlès , A. 2006 . Histological observations of enantiornithine bone (Saurischia, Aves) from the Lower Cretaceous of Las Hoyas (Spain) . Comptes Rendus Palevol , 5 ( 3 ) : 685 – 691 .
- Cau , A. and Arduini , P. 2008 . Enantiophoenix electrophyla gen. et sp. nov. (Aves, Enantiornithes) from the Upper Cretaceous (Cenomanian) of Lebanon and its phylogenetic relationships . Atti della Società Italiana di Scienze Naturali e del Museo Civico di Storia Naturale di Milano , 149 ( 2 ) : 293 – 324 .
- Chang , M.-M. , Chen , P.-J. , Wang , Y.-Q. , Wang , Y. and Miao , D.-S. 2003 . The Jehol Fossils: The Emergence of Feathered Dinosaurs, Beaked Birds and Flowering Plants , 209 Shanghai : Shanghai Scientific & Technical Publishers .
- Chiappe , L. M. 1991 . Cretaceous avian remains from Patagonia shed new light on the early radiation of birds . Alcheringa , 15 : 333 – 338 .
- Chiappe , L. M. 1995a . The phylogenetic position of the Cretaceous birds of Argentina: Enantiornithes and Patagopteryx deferrariisi . Courier Forschungsinstitut Senckenberg , 181 : 55 – 63 .
- Chiappe , L. M. 1995b . The first 85 million years of avian evolution . Nature , 378 : 349 – 355 .
- Chiappe , L. M. 2002 . “ Basal bird phylogeny: problems and solutions ” . In Mesozoic Birds: Above the Heads of Dinosaurs , Edited by: Chiappe , L. M. and Witmer , L. M. 448 – 472 . Berkeley : University of California Press .
- Chiappe , L. M. and Calvo , J. O. 1994 . Neuquenornis volans, a new Late Cretaceous bird (Enantiornithes: Avisauridae) from Patagonia, Argentina . Journal of Vertebrate Paleontology , 14 ( 2 ) : 230 – 246 .
- Chiappe , L. M. and Dyke , G. J. 2007 . “ The beginnings of birds: recent discoveries, ongoing arguments, and new directions ” . In Major Transitions in Vertebrate Evolution , Edited by: Sues , H.-D. and Anderson , J. S. 303 – 336 . Bloomington : Indiana University Press .
- Chiappe , L. M. and Walker , C. A. 2002 . “ Skeletal morphology and systematics of the Cretaceous Euenantiornithes (Ornithothoraces: Enantiornithes) ” . In Mesozoic Birds: Above the Heads of Dinosaurs. , Edited by: Chiappe , L. M. and Witmer , L. M. 240 – 267 . Berkeley : University of California Press .
- Chiappe , L. M. , Ji , S. , Ji , Q. and Norell , M. A. 1999 . Anatomy and systematics of the Confuciusornithidae (Theropoda: Aves) from the Late Mesozoic of northeastern China . Bulletin of the American Museum of Natural History , 242 : 1 – 89 .
- Chiappe , L. M. , Norell , M. and Clark , J. 2001 . A new skull of Gobipteryx minuta (Aves: Enantiornithes) from the Cretaceous of the Gobi Desert . American Museum Novitates , 3346 : 1 – 15 .
- Chiappe , L. M. , Norell , M. A. and Clark , J. M. 2002 . “ The Cretaceous, short-armed Alvarezsauridae ” . In Mesozoic Birds: Above the Heads of Dinosaurs , Edited by: Chiappe , L. M. and Witmer , L. M. 87 – 120 . Berkeley : University of California Press .
- Chiappe , L. M. , Ji , S. and Ji , Q. 2007 . Juvenile birds from the Early Cretaceous of China: implications for enantiornithine ontogeny . American Museum Novitates , 3594 : 1 – 46 .
- Clark , J. M. , Norell , M. A. and Rowe , T. 2002 . Cranial anatomy of Citipati osmolskae (Theropoda, Oviraptorosauria), and a reinterpretation of the holotype of Oviraptor philoceratops . American Museum Novitates , 3364 : 1 – 24 .
- Clarke , J. A. and Norell , M. A. 2002 . The morphology and phylogenetic position of Apsaravis ukhaana from the Late Cretaceous of Mongolia . American Museum Novitates , 3387 : 1 – 46 .
- Chinsamy , A. and Elzanowski , A. 2001 . Evolution of growth pattern in birds . Nature , 412 : 402 – 403 .
- Currie , P. J. and Chen , P-J. 2001 . Anatomy of Sinosauropteryx prima from Liaoning, northeastern China . Canadian Journal of Earth Sciences , 38 : 1705 – 1727 .
- Dalla Vecchia , F. M. and Chiappe , L. M. 2002 . First avian skeleton from the Mesozoic of northern Gondwana . Journal of Vertebrate Paleontology , 22 ( 4 ) : 856 – 860 .
- Elzanowski , A. 1974 . Preliminary note on the palaeognathous bird from the Upper Cretaceous of Mongolia . Palaeontologica Polonica , 30 : 103 – 109 .
- Elzanowski , A. 1976 . Paleognathous bird from the Cretaceous of central Asia . Nature , 264 : 51 – 53 .
- Elzanowski , A. 1977 . Skulls of Gobipteryx (Aves) from the Upper Cretaceous of Mongolia . Palaeontologica Polonica , 37 : 153 – 165 .
- Elzanowski , A. 1981 . Embryonic bird skeletons from the Late Cretaceous of Mongolia . Palaeontologica Polonica , 42 : 147 – 179 .
- Elzanowski , A. 1995 . “ Cretaceous birds and avian phylogeny ” . In Courier Forschungsinstitut Senckenberg 37 – 53 . 181
- Elzanowski , A. 2002 . “ Archaeopterygidae (Upper Jurassic of Germany) ” . In Mesozoic Birds: Above the Heads of Dinosaurs , Edited by: Chiappe , L. M. and Witmer , L. M. 129 – 159 . Berkeley : University of California Press .
- Estrella , S. M. and Masero , J. A. 2007 . The use of distal rhynchokinesis by birds feeding in water . Journal of Experimental Biology , 210 ( 21 ) : 3757 – 3762 .
- Gao , C.-L. and Liu , J.-Y. 2005 . A new avian taxon from Lower Cretaceous Jiufotang Formation of western Liaoning . Global Geology , 24 ( 4 ) : 313 – 318 .
- Göhlich , U. B. and Chiappe , L. M. 2006 . A new carnivorous dinosaur from the Late Jurassic Solnhofen archipelago . Nature , 440 : 329 – 332 .
- He , H.-Y. , Wang , X.-L. , Jin , F. , Zhou , Z.-H. , Wang , F. , Yang , L.-K. , Ding , X. , Boven , A. and Zhu , R.-X. 2006 . The 40Ar/39Ar dating of the early Jehol Biota from Fengning, Hebei Province, northern China . Geochemistry, Geophysics, Geosystems , 7 ( 4 ) : 1 – 8 .
- Hou , L. 1997 . Mesozoic Birds of China , 221 Taiwan : Feng-huang-ku Bird Park .
- Hou , L. , Zhonghe , Z. , Martin , L. D. and Feduccia , A. 1995 . A beaked bird from the Jurassic of China . Nature , 377 : 616 – 618 .
- Hou , L. , Martin , L. D. , Zhou , Z. and Feduccia , A. 1999 . Archaeopteryx to opposite birds—missing link from the Mesozoic of China . Vertebrata PalAsiatica , 37 ( 2 ) : 88 – 95 .
- Hou , L. , Chiappe , L. M. , Zhang , F. and Chuong , C.-M. 2004 . New Early Cretaceous fossil from China documents a novel trophic specialization for Mesozoic birds . Naturwissenschaften , 91 : 22 – 25 .
- Howard , H. 1929 . The avifauna of Emeryville shellmound . University of California Publications in Zoology , 32 ( 2 ) : 301 – 394 .
- Kurochkin , E. N. 1996 . A new enantiornithid of the Mongolian Late Cretaceous, and a general appraisal of the Infraclass Enantiornithes (Aves) . Russian Academy of Sciences, Palaeontological Institute, Special Issue , : 50
- Li , L. , Duan , Y. , Hu , D. , Wang , L. , Cheng , S. and Hou , L. 2006 . New eoentantiornithid bird from the Early Cretaceous Jiufotang Formation of western Liaoning, China . Acta Geologica Sinica (English edition) , 80 ( 1 ) : 38 – 41 .
- Li , L. , Hu , D.-Y. , Duan , Y. , Gong , E.-P. and Hou , L.-H. 2007 . Alethoalaornithidae fam. nov.: a new family of enantiornithine bird from the Lower Cretaceous of western Liaoning . Acta Palaeontologica Sinica , 46 : 365 – 372 .
- Marinelli , W. 1928 . Über den Schädel der Schnepfe . Paleobiologica , 1 : 135 – 160 .
- Martin , L. D. 1983 . “ The origin and early radiation of birds ” . In Perspectives in Ornithology: essays presented for the Centennial of the American Ornithological Union , Edited by: Brush , A. H. and Clark , G. A. Jr. 291 – 338 . Cambridge : Cambridge University Press .
- Martin , L. D. 2004 . A basal archosaurian origin for birds . Acta Zoologica Sinica , 50 : 978 – 990 .
- Martin , L. D. and Zhou , Z. 1997 . Archaeopteryx-like skull in enantiornithine bird . Nature , 389 : 556
- Marugán-Lobón , J. and Buscalioni , A. D. 2003 . Disparity and geometry of the skull in Archosauria (Reptilia: Diapsida) . Biological Journal of the Linnean Society , 80 : 67 – 88 .
- Marugán-Lobón , J. and Buscalioni , A. D. 2006 . Avian skull morphological evolution: exploring exo- and endocranial covariation with two-block partial least squares . Zoology , 109 : 217 – 230 .
- Morschhauser , E. , Varricchio , D. J. , Gao , C.-H. , Liu , J.-Y. , Wang , X.-R. , Cheng , X.-D. and Meng , Q.-J. 2009 . Anatomy of the Early Cretaceous bird Rapaxavis pani, a new species from Liaoning Province, China . Journal of Vertebrate Paleontology , 29 : 545 – 554 .
- Norell , M. A. and Makovicky , P. J. 2004 . Dromaeosauridae , Edited by: Weishampel , D. B. , Dodson , P. and Osmólska , H. 196 – 209 . Berkeley : University of California Press .
- O’Connor , J. K. , Wang , X.-R. , Chiappe , L. M. , Gao , C.-H. , Meng , Q.-J. , Cheng , X.-D. and Liu , J.-Y. 2009 . Phylogenetic support for a specialized clade of Cretaceous enantiornithine birds with information from a new species . Journal of Vertebrate Paleontology , 29 : 188 – 204 .
- Ostrom , J. H. 1969 . Osteology of Deinonychus antirrhopus, an unusual theropod from the Lower Cretaceous of Montana . Bulletin of the Peabody Museum of Natural History , 30 : 1 – 165 .
- Piersma , T. , van Aelst , R. , Kurk , K. , Berkhoudt , H. and Maas , L. R. M. 1998 . A new pressure sensory mechanism for prey detection in birds: the use of principles of seabed dynamics? . Proceedings of the Royal Society of London, Series B , 265 : 1777 – 1783 .
- Sanz , J. L. , Chiappe , L. M. , Pérez-Moreno , B. P. , Buscalioni , A. D. , Moratalla , J. J. , Ortega , F. and Poyato-Ariza , F. J. 1996 . An Early Cretaceous bird from Spain and its implications for the evolution of avian flight . Nature , 382 : 442 – 445 .
- Sanz , J. L. , Chiappe , L. M. , Pérez-Moreno , B. , Moratalla , J. J. , Hernández-Carrasquilla , F. , Buscalioni , A. D. , Ortega , F. , Poyato-Ariza , F. J. , Rasskin-Gutman , D. and Martinez-Delclòs , X. 1997 . A nestling bird from the Lower Cretaceous of Spain: implications for avian skull and neck evolution . Science , 276 : 1543 – 1546 .
- Sereno , P. C. and Rao , C. 1992 . Early evolution of avian flight and perching: new evidence from the Lower Cretaceous of China . Science , 255 : 845 – 848 .
- Van Der Klaauw , C. J. 1948 . Size and position of the functional components of the skull. A contribution to the knowledge of the architecture of the skull, based on data in the literature . Archives Néerlandaises de Zoologie , 9 : 1 – 558 .
- Walker , C. A. 1981 . New subclass of birds from the Cretaceous of South America . Nature , 292 : 51 – 53 .
- Wang , X.-R. , O’Connor , J. K. , Zhao , B. , Chiappe , L. M. , Gao , C.-H. and Cheng , X.-D. 2010 . A new species of Enantiornithes (Aves: Ornithothoraces) based on a well preserved specimen from the Qiaotou Formation of Northern Hebei, China . Acta Geologica Sinica , 84 : 247 – 256 .
- Weishampel , D. B. , Dodson , P. and Osmolska , H. 2004 . The Dinosauria , 880 Berkeley : University of California Press .
- Witmer , L. M. 1997 . The evolution of the antorbital cavity of archosaurs: a study in soft-tissue reconstruction in the fossil record with an analysis of the function of pneumaticity . Society of Vertebrate Paleontology Memoir , 3 : 1 – 73 .
- Witmer , L. M. and Ridgely , R. C. 2008 . The paranasal air sinuses of predatory and armored dinosaurs (Archosauria: Theropoda and Ankylosauria) and their contribution to cephalic structure . The Anatomical Record , 291 : 1362 – 1388 .
- Xu , G.-L. , Yang , Y.-S. and Deng , S.-Y. 1999 . First discovery of Mesozoic bird fossils in Hebei Province and its significance . Regional Geology of China , 1 : 444 – 448 .
- Xu , X. , Cheng , Y.-N. , Wang , X.-L. and Chang , C.-H. 2002 . An unusual oviraptorosaurian dinosaur from China . Nature , 419 : 291 – 293 .
- Yang , W. , Li , S. and Jiang , B. 2007 . New evidence for Cretaceous age of the feathered dinosaurs of Liaoning: zircon U-Pb SHRIMP dating of the Yixian Formation in Sihetun, northeast China . Cretaceous Research , 28 : 177 – 182 .
- Zhang , F. and Zhou , Z. 2000 . A primitive enantiornithine bird and the origin of feathers . Science , 290 : 1955 – 1960 .
- Zhang , F. , Zhou , Z. , Hou , L. and Gu , G. 2000 . Early diversification of birds: evidence from a new opposite bird . Kexue Tongbao , 45 : 2650 – 2657 .
- Zhang , F. , Ericson , P. G. and Zhou , Z. 2004 . Description of a new enantiornithine bird from the Early Cretaceous of Hebei, northern China . Canadian Journal of Earth Sciences , 41 : 1097 – 1107 .
- Zhang , Z. , Hou , L. , Hasegawa , Y. , O’Connor , J. , Martin , L. D. and Chiappe , L. M. 2006 . The first Mesozoic heterodactyl bird from China . Acta Geologica Sinica (English edition) , 80 : 631 – 635 .
- Zheng , X. , Zhang , Z. and Hou , L. 2007 . A new enantiornitine bird with four long rectrices from the Early Cretaceous of northern Hebei, China . Acta Geologica Sinica (English edition) , 81 : 703 – 708 .
- Zhou , Z. 2002 . A new and primitive enantiornithine bird from the Early Cretaceous of China . Journal of Vertebrate Paleontology , 22 : 49 – 57 .
- Zhou , Z. 2006 . Evolutionary radiation of the Jehol Biota: chronological and ecological perspectives . Geological Journal , 41 : 377 – 393 .
- Zhou , Z. and Zhang , F. 2001 . Two new ornithurine birds from the Early Cretaceous of western Liaoning, China . Chinese Science Bulletin , 46 : 1258 – 1264 .
- Zhou , Z. and Zhang , F. 2005 . Discovery of an ornithurine bird and its implication for Early Cretaceous avian radiation . Proceedings of the National Academy of Sciences , 102 : 18998 – 19002 .
- Zhou , Z.-H. and Zhang , F.-C. 2006a . Mesozoic birds of China—a synoptic review . Vertebrata PalAsiatica , 44 : 74 – 98 .
- Zhou , Z.-H. and Zhang , F.-C. 2006b . A beaked basal ornithurine bird (Aves, Ornithurae) from the Lower Cretaceous of China . Zoologica Scripta , 35 : 363 – 373 .
- Zhou , Z. , Jin , F. and Zhang , J. 1992 . Preliminary report on a Mesozoic bird from Liaoning, China . Chinese Science Bulletin , 37 : 1365 – 1368 .
- Zhou , Z. , Clarke , J. , Zhang , F. and Wings , O. 2004 . Gastroliths in Yanornis: an indication of the earliest radical diet-switching and gizzard plasticity in the lineage leading to living birds . Naturwissenschaften , 91 : 571 – 574 .
- Zhou , Z. , Chiappe , L. M. and Zhang , F. 2005 . Anatomy of the Early Cretaceous bird Eoenantiornis buhleri (Aves: Enantiornithes) from China . Canadian Journal of Earth Sciences , 42 : 1331 – 1338 .
- Zhou , Z. , Clarke , J. and Zhang , F. 2008 . Insight into diversity, body size and morphological evolution from the largest Early Cretaceous enantiornithine bird . Journal of Anatomy , 212 : 565 – 577 .
- Zinoviev , A. V. 2009 . An attempt to reconstruct the lifestyle of confuciusornithids (Aves, Confuciusornithifomes) . Paleontological Journal , 43 : 444 – 452 .
- Zusi , R. L. 1984 . A functional and evolutionary analysis of rhynchokinesis in birds . Smithsonian Contributions to Zoology , 395 : 1 – 40 .
- Zusi , R. L. 1993 . “ Patterns of diversity in the Avian skull ” . In The Skull: patterns of structural and systematic diversity , Edited by: Hanken , J. and Hall , B. K. 391 – 437 . Chicago : University of Chicago Press .