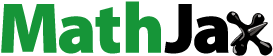
Abstract
Extant istiophorids are open ocean apex predators that are extensively studied due to their ecological importance and high values for fisheries. Nevertheless, little is known about their evolution because of a fragmentary fossil record and extremely difficult taxonomy of fossil species. Here, we present a new phylogenetic hypothesis covering fossil and living istiophorids. Our results demonstrate that istiophorid richness is larger than previously assumed, comprising eight genera with 20 species. The phylogenetic analysis shows that istiophorids are grouped into four clades: the Istiophorus clade, which includes the sailfish; the Machairostra clade, which comprises Makaira spp., including two new species from the late Miocene (†Makaira colonense sp. nov. and †Makaira fierstini sp. nov.); the Gracilorostra clade, which comprise all remaining istiophorids with exception of spearfishes and includes two new genera and one new species (†Morgula donosochagrense gen. et sp. nov. and †Spathochoira calvertense gen. et. comb. nov.); and the Tetrapturomorpha clade is composed of the spearfishes and the extinct †Prototetrapturus courcelli gen. et. comb. nov. The family Istiophoridae shows an evolutionary trend toward reduction of the premaxillary thickness and increasing the extension of narial cavities. This reduction is related to an increase of adipose tissues in the rostrum base probably driven by the presence of the oleofera gland, an organ involved in feeding, healing, endothermy and hydrophobic functions. Our phylogeny shows a direct relationship between the rostral and cranial shape explained by body size and feeding behaviour. The larger istiophorids have lateral apophysis and the larger spines of the vertebral column. The spearfishes represent the smaller species of the family, with the extant Tetrapturus spp. first appearing in the late Pliocene. The clade Tetrapturomorpha shows an extreme size reduction over time when compared with species of their sister clade Gracilorostra, demonstrating an evolutionary trend towards size reduction.
http://zoobank.org/urn:lsid:zoobank.org:pub:D3D3B15B-36FA-42EB-98AD-FAF369D989EB
Introduction
Istiophorid billfishes (Istiophoridae) are a small group of teleostean fishes with about 9–10 species of apex predators inhabiting tropical to subtropical open oceanic environments (Gregory & Conrad Citation1937; Nakamura Citation1983; Fierstine & Voigt Citation1996; Nelson et al. Citation2016). These are large and fast swimmers characterized by elongated rostra formed by fused premaxillae, prenasals and nasals (Gregory & Conrad Citation1937; Nakamura Citation1983; Fierstine & Voigt Citation1996; Nelson et al. Citation2016). Most studies focusing on extant istiophorids centred primarily on phylogenetics and population genetics (Graves & McDowell Citation1995, Citation2012, Citation2015; Hoolihan et al. Citation2004; Collette et al. Citation2006; Little et al. Citation2010; Hanner et al. Citation2011; Bernard et al. Citation2013; Williams et al. Citation2018), and only a few compared the morphology of extant istiophorids using structures other than the rostrum (Gregory & Conrad Citation1937; Nakamura Citation1955, Citation1983, Citation1985; Morrow & Posner Citation1957; Fierstine & Walters Citation1968; Fierstine & Voigt Citation1996). This imbalance between palaeontological and biological studies has resulted in an ambiguous taxonomy and systematic assignment of fossil species and a deficient understanding regarding the origin and evolutionary history of extant billfishes and their structures (Fierstine Citation1978, Citation1990, Citation2006; Schultz Citation1987; Fierstine & Voigt Citation1996; Carnevale et al. Citation2002; Santini & Sorenson Citation2013; Graves & McDowell Citation2015; Williams et al. Citation2018). Among istiophorids, rostral features that may be taxonomically diagnostic have not yet been fully established, with the species-level resolution for fossil taxa thus remaining obscure (Fierstine Citation1978, Citation1990, Citation2006; Schultz Citation1987; Fierstine & Voigt Citation1996; Carnevale et al. Citation2002) while the origin of the rostrum from a deep-time perspective is still unclear (Habegger et al. Citation2015, Citation2017, Citation2020).
The fossil record of istiophorids extends back to the early Miocene (Bianucci et al. Citation2018). Nonetheless, most fossil specimens were recovered during the late nineteenth and early twentieth centuries, when the stratigraphical certainty about the corresponding localities still was limited (Rütimeyer Citation1857; Van Beneden Citation1871; Berry Citation1917; Lériche Citation1926; Arambourg Citation1927). The combination of uncertainty with regards to the geological provenance of certain fossil specimens, poorly constrained fossil sites with little information about palaeoenvironments, and uncertainty in the relationships between living and fossil billfishes have contributed to a poorly understood evolutionary history for billfishes. Here we present the first unifying systematic scheme of extinct and extant istiophorids based on a thorough revision of the fossil record of billfishes. We examine the variation of rostral traits within and across species of istiophorids, and discuss the phylogenetic relationships between fossil and extant istiophorid billfishes.
Geological setting
Three new specimens are presented herein: MUPAN–STRI 31291, MUPAN–STRI 31293 and MUPAN–STRI 39292, which were recovered from near-coastal sediments of the Miocene Chagres Formation, NNE of Panama City along the Caribbean coast (). The Chagres Formation is a ∼ 250 m sequence that overlies the Gatun Formation (Coates Citation1999) and spans the late Miocene, c. 8.3 to 5.6 Ma (Collins et al. Citation1996; Coates Citation1999; Hendy Citation2013). The Chagres Formation is divided into three members: Toro, Rio Indio and Chagres Sandstone (). The specimens described here were collected in the upper Chagres Sandstone member from two sites near the town of Piña, 9°16′53.4ʺN, 80°2′40.9ʺW and 9°17′24.89ʺN, 80°2′25.39ʺW (). Both sites are at stratigraphical metres 361 to 367 of the stratigraphical section #57 of Coates (Citation1999) that are dated as ranging from 6.4 to 5.8 Ma (Collins et al. Citation1996). The Chagres Sandstone consists of grey volcanic, quartzose, silty sandstones, with lithic and feldspar grains, and pervasive bioturbation of arthropod burrows (Collins et al. Citation1996). This member is highly fossiliferous including invertebrates, benthic foraminifera (Collins et al. Citation1996), chondrichthyan teeth (Carrillo-Briceño et al. Citation2015), fish otoliths (De Gracia et al. Citation2012; Schwarzhans & Aguilera Citation2013), fish bones (Fierstine Citation1978) and cetaceans (Pyenson et al. Citation2015; Vigil & Laurito Citation2014; Velez-Juarbe et al. Citation2015)
Figure 1. A–C, location map; stratigraphy and geological setting of the type locality (Piña, Chagres district, Costa Abajo, Colon Panama) after Collins et al. (Citation1996).
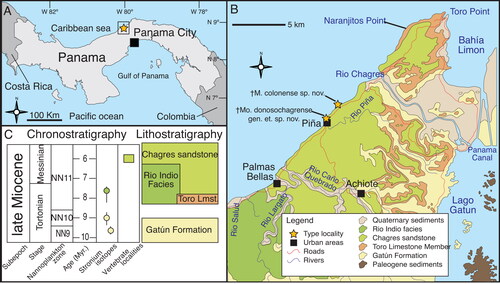
Material and methods
Institutional abbreviations
CTPA, Center of Tropical Paleontology and Archaeology of STRI, Panama City, Panama; IRSNB, Institut Royal des Sciences Naturelles de Belgique, Brussels, Belgium; LACM, Natural History Museum of Los Angeles Country, Los Angeles, CA, USA; MAUL, Museo dell’Ambiente, Università del Salento, Lecce, Italy; MHNW, Natural History Museum of Vienna, Austria; MNHN, Museum National d’Histoire Naturelle, Paris, France; MUPAN, Museo de Paleontología de Panamá, Universidad de Panamá, Panama City, Panama; OCPC, Orange County Paleontology Collection, Santa Ana, CA, USA; STRI, Smithsonian Tropical Research Institute, Panama City, Panama; UF, Florida Museum of Natural History, Gainesville, FL, USA; USNM, Smithsonian National Museum of Natural History, Washington, DC, USA.
Material
The herein newly described specimens from the Chagres Formation (MUPAN–STRI 31293 and MUPAN–STRI 39292) were collected in October 2011 and July 2014, respectively, by the Smithsonian Tropical Research Institute (STRI) on the beach of the town of Piña, Colon, Panama (). Specimen MUPAN–STRI 31291 is included here and identified as Istiophoridae sp. 1, and its detailed morphological description is prepared for an additional publication.
Fossils were prepared mechanically with carbon fibre needles, air scribe chisels and rotatory flexible shafts tools, and subsequently chemically stabilized with epoxy, paraloid PB72 and paleobond PB40. We employed a combination of the osteological terms of Gregory (Citation1933), Gregory & Conrad (Citation1937), Jollie (Citation1986), Schultz (Citation1987), Davie (Citation1990) and Rojo (Citation2017), and the common names used by Pepperell (Citation2010) to refer to extant species. Linear measures of bones were made to the nearest 0.5 mm. The taxonomical scheme for fossil billfishes follows that of Nelson et al. (Citation2016).
Reviewed fossil material. †Istiophorus solidus (holotype): IRSNB P643 rostrum; †Istiophorus calvertensis (holotype): USNM 9344 nearly complete rostrum; †Makaira belgica (holotype): IRSNB P117 distal rostrum fragment; †Makaira courcelli (holotype): MNHNP 250 nearly complete rostrum; †Makaira panamensis (holotype): USNM 18710 nearly complete skull and rostrum; †Makaira purdyi (holotype): USNM 481933 nearly complete rostrum; †Makaira nigricans: OCPC 31001 skull with rostrum; Makaira cf. M. nigricans: USNM 358534, MAUL 917/1 rostrum with partially preserved skull; MAUL 57/1 complete rostrum; MAUL 60/1 nearly complete rostrum; †Thalattorhynchus austriacus (holotype): NHMW 1986/109.
Extant taxa and specimens used for comparative purposes. Xiphias gladius (swordfish): MNHN 698 entire skeleton; MHMW uncatalogued mounted skeleton; USNM 110042 entire skeleton; Istiophorus platypterus (sailfish): STRI archaeology collection 1628 entire skeleton, MNHN A-9463 skull, MNHN A-9464 skull, MNHN 6960 complete skeleton; Makaira nigricans (blue marlin): USNM 196019 entire skeleton, LACM 46023-1 vertebral column, MNHN 1892-1050 rostrum; Istiompax indica (black marlin): LACM 25509 entire skeleton, UF 210017 pectoral girdle, MNHN 1884.29 pectoral fin, MNHN A-5514 rostrum fragment, MNHN 2003-0420 complete dry specimen; Kajikia albida (white marlin): USNM 270766 skeleton, USNM 3605507 axial skeleton; Kajikia audax (striped marlin): USNM 372777 entire skeleton, MNHN 6821 entire skeleton, LACM 25500 skull; Tetrapturus pfluegeri (long bill spearfish): LACM 25462 entire skeleton, UF-208789 vertebral column and pectoral fin girdle; Tetrapturus belone: MNHN A-7504 entire skeleton, NHMW 93902 skull, NHMW uncatalogued mounted skeleton; Tetrapturus angustirostris (short bill spearfish): LACM 25499 entire skeleton. Additional material for extant species that includes complete rostrums and crania from LACM are listed in Supplemental file 1.
Analyses
Morphological measurements. Measurements are based on the methodology developed by Fierstine & Voigt (Citation1996) and Fierstine (Citation1998, Citation2001) to describe and classify fossil billfishes using rostral characters (, ). The istiophorid rostrum length (L) is measured as the distance between the distal tip and the orbital margin of the lateral ethmoid bone (). Two defined sections along the rostral length are used: at 0.5 L representing the middle of the rostral length () and at 0.25 L representing a quarter of the rostral length (). Characters studied in each region were depth (D1, D2) and width (W1, W2) of the rostrum (); height (H1, H2) and width (N1, N2) of the left internal canal (); distance of the internal canal from the dorsal surface (DD1, DD2) and distance between canals (IC1, IC2) (). Characters without region reference are the distance of the prenasal bone (P) from the distal tip of the rostrum, length from the distal tip to where the fused premaxillaries divide (VSPM) in two separate bones, and distribution of the denticles on the ventral surface of the bill (DVS) (Fierstine & Voigt Citation1996; Fierstine Citation1998, Citation2001) ().
Figure 2. Generalized istiophorid rostrum (modified from Fierstine & Voigt Citation1996): A, left lateral view; B, dorsal view; C, ventral view. Abbreviations for characters: DVS, distribution of denticles on the ventral surface; DZ, distribution of denticles on the dorsal surface of the rostrum; i pr, internal process of maxilla; L, bill length; m pr, maxillary process; NC, narial cavity; ORB, orbital region; P, position of prenasal bone from the distal tip of the rostrum; tr, triangular region of maxilla; VA, ventral aperture; VSPM, length from the tip where fused premaxillaries divide into two separate bones. The abbreviations of bone names are listed in the methodology, illustrations have been modified from Fierstine & Voigt (1996).
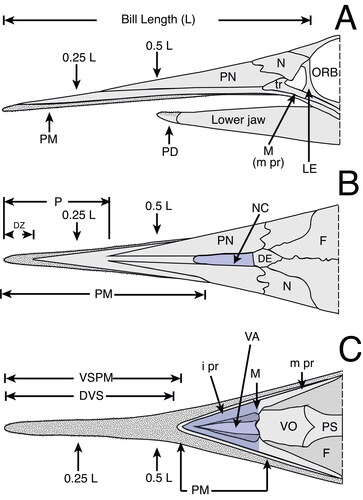
Figure 3. A, B, characters studied with emphasis on two regions of the rostrum: 0.5 L, half of the distance between the distal tip and the orbital margin of the lateral ethmoid bone and 0.25 L, one-fourth the distance between the distal tip and the orbital margin of the lateral ethmoid bone; A, cross-section view at 0.5 L; B, cross-section view at 0.25 L; C, left dentary on medial view of the interdentary joint. Character abbreviations: depth (D1, D2) and width (W1, W2) of the rostrum, height (H1, H2) and width (N1, N2) of the left internal canal (as seen in cross-section), intercanal distance (IC1, IC2) and distance of the internal canal from dorsal surface (DD1, DD2). Depth from the anterior-most denticles perpendicular to the ventral margin of the dentary (not to the distal margin, which often has projection) (DAD), and length of the intermediary joint from the mandibular foramen to the anterior-most denticles (DJL). Mandibular foramen = mf. Modified from Fierstine & Voigt (Citation1996) and Fierstine (Citation2001).
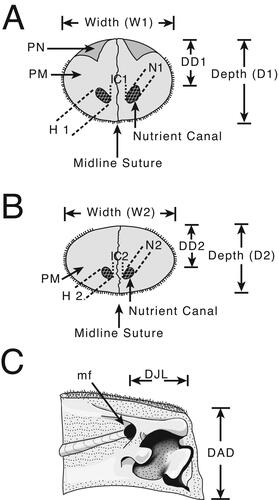
We implemented new variables for quantifying the cross-section areas for the rostrum and internal canals: variables AR1 and AR2 measure the cross-section area of the rostrum at 0.5 L and 0.25 L (; Supplemental material 1) and were estimated by using width (W) and depth (D) as the major (1/2W) and minor (1/2D) semi-axes of an ellipse. Variables CA1 and CA2 measure the cross-section area of the left internal canals at 0.5 L and at 0.25 L (). The internal canal area (CA1 and CA2) was estimated by using width (N) and height (H) as the major (1/2W) and minor (1/2D) semiaxes of an ellipse (). Discrete variables include the morphological study of the distal tip shape and the position, lateral distribution and rotation of the left internal canal (Supplemental material Figs S1, S2). These characters are described with a new terminology applied here to the descriptions of fossil species and are coded for the phylogenetic analysis where specified. For the dentary two measures are taken from the interdentary joint for specimen MUPAN–STRI 31293: depth from the anterior-most denticles to the ventral margin (DAD); and length of the interdentary joint from the mandibular foramen to the anterior-most denticles (DJL) () (Fierstine Citation2001).
Table 3. Relationship between height of left nutrient canal at 0.5 L (H1) with the CA1/AR1 ratio (canal area / cross-section area at 0.5 L). Using H1 as size estimator, small nutrient canals range between 1.0 and 3.9 mm and big canals range between 4.0 and 8.5 mm. The ratio CA1/AR1 removes the size effect and small canals have values CA1/AR1 of 0.002–0.019 and big canals of 0.02–0.07. In the score column ‘b’ is big and ‘s’ is small for the H1 and CA1/AR1 variables. For †Makaira purdyi variables at 0.25 L were used given the special morphology of this species. Ranges were defined after studying fossils and modern specimens together (Table S1).
Rostral reconstruction. The istiophorid rostrum is triangular () and it is easy to estimate the missing portions by extrapolating this shape in incomplete specimens (Fierstine Citation1999a). To estimate the missing length and the prenasals extension of incomplete specimens, we used straight linear projections from the outer margins of the preserved parts of the rostral bones. The point where the two linear projections intersect is assumed to represent the anterior-most extension of the rostrum and thus provides an estimate for the percentage of the missing portion. The estimated distance is added to the observed length (Fierstine Citation1999a). The estimation of the missing distance is possible only when partially preserved rostrums include both the 0.5 L and the 0.25 L regions (). The cross-section appearance can be reconstructed from CT scans or photographs if the preserved portion includes at least one prenasal, premaxilla and one internal canal (). If a cross-section of a rostrum in a CT image is disarticulated and the specimen is not deformed, the internal appearance can be reconstructed by placing the bones in their correct position using any drawing software, e.g. Adobe Illustrator ().
Ratio analysis. We applied the ratios method developed by Fierstine (Citation1998, Citation2001) for billfish classification. This method uses the measures of individual bones (characters section of methods; ; Supplemental material 1) converted into ratios () to identify fossil billfishes. Ratios are calculated by dividing the shorter measurement by the longer measurement (). This method takes ratios as variables instead of individual measurements to eliminate size variation (Fierstine Citation1998, Citation2001). The calculated ratios from fossil specimens are compared with ratios of extant istiophorid species () and a score sheet () is prepared. The score sheet summarizes the number of ratios that are within the range for each extant species by individual bones (). Then, fossil taxa needing further taxonomical assessment should be identifiable as known species if most of the scores are within the range of a particular species unless its overall score overlaps two or more genera, its overall score overlaps with two or more species within the same genus, or some of its scores fall outside the observed range of recent species (Fierstine Citation1998, Citation2001). In the first two cases, the unknown fossil would be identified only to genus or family level, respectively. In the third case, the unknown specimen would potentially be identified as a known fossil species, a variant of a recent or fossil taxon, or a new species, depending on how it differs from the fossil or recent species (Fierstine Citation1999a). The ratios analysis is compared to the morphometric analysis in order to improve our understanding of shape variation in billfish rostra. The range of the observed values for the ratios in our sample across different species () was used to establish limits for the phylogenetic characters as specified in the Supplemental material 1.
Table 1. Mean (x), observed range, and number of specimens examined (n) for nine rostral and one dentary ratios of eight Istiophoridae billfish compared with three fossil species. Abbreviations of the ratio are explained in the methodology and in the legends of , and . Values presented here for marlins were obtained after data imputation. The variable DD1/D1 for K. audax and all data from spearfishes (Tetrapturus ssp.) were taken from Fierstine (Citation2001). ‘NA’ non-available data, ‘*’ indicates out of range values if compared with modern species.
Table 2. Score sheet of nine rostral ratios of seven modern billfish species compared with three fossil species. The first number is the number of ratios on the range and the second number is the total of analysed ratios of each region. The total score indicates the number of ratios of fossil species on the rage for each modern species. For †Mo. donosochagrense gen et. sp. nov. one ratio of dentaries is analysed.
Morphometric analysis. We used a morphological dataset comprising 105 extant individuals from five marlin species (Supplemental material 1). As the original dataset includes missing values, we assumed those are missing at random (MAR). MAR means that the probability of a missing observation is related to the values for the observed variables (Rubin Citation1976; Dray & Josse Citation2015). Such a relationship is demonstrated by applying a multiple correlation using all variables (Supplemental material Fig. S3). In order to reduce the noise introduced by missing data in the morphological analysis, we applied the Multiple Imputation with Regularized Version of Interactive PCA Algorithm (MIPCA) for MAR data to obtain the missing values (Josse & Husson Citation2012, Citation2016). MIPCA is a recently developed method that does not alter the original values and overcomes problems such as over-fitting. Additionally, it reduces uncertainty in the predicted values (Josse et al. Citation2011; Josse & Husson Citation2012, Citation2016). MIPCA is available in the package missMDA (Josse et al. Citation2011; Josse & Husson Citation2012, Citation2016) for R version 4.0.2 (R Core Team Citation2013) and its application consists of three steps: (1) calculating the number of dimensions (ncp) that minimizes the mean square error prediction; (2) performing the MIPCA with the estimated ncp number (to obtain the missing values); and (3) performing the selected multivariate analysis with the new dataset (Josse & Husson Citation2016).
To calculate ncp (step 1) each species was treated as separate data subset to prevent biases by mixing data with different distributions (different species). The percentage of missing values were 10.5% for Istiophorus platypterus, 10.4% for Makaira mazara, 20.7% for Istiompax indica, 21.9% for Kajikia audax and 32% for Kajikia albida. The implemented model to generate the ncp number (step 1) was to insert and predict values with the PCA algorithm = 0.05%, number of simulations = 100, minimal ncp = 0, maximum ncp = 6 and cross validation technique = k-fold method (see Josse & Husson [Citation2016] for a detailed explanation of the model parameters). For K. audax, after detecting an elbow with the ncp number, a value of 2 was assigned to this parameter (J. Josse, pers. comm). When the ncp value for each modern species was obtained, we performed the MIPCA method (step 2) to obtain the missing values in each data subset. The parameters for the performed MIPCA model were: 200 imputed datasets using the regularized version of the PCA algorithm and Bayesian approach as variance estimator across calculated parameters (see Josse & Husson Citation2016). The same process was performed for each species and the resulting five data subsets were combined into one data frame (Supplemental material 1). For K. audax, variable DD1 was completely missed and its values were obtained from its close relative K. albida by repeating the MIPCA process with combined data for these two species. This approach did not change the final result and prevented loss of information for variable DD1 in other species during the final analysis. With the complete dataset, the four new variables that measure areas were calculated (AR1, CA1, AR2 and CA2) and included into the dataset (Supplemental material 1).
We assembled the analysed dataset using all specimens with MIPCA treatment, eight fossil specimens previously described, and three unpublished specimens (Supplemental material 1). Fossil individuals were not analysed with MIPCA. This dataset was analysed using PCA (step 3). For this purpose, only 13 variables were used because fossil specimens lack information on DVS, P and VSPM, whereas the variables CA2, N2, IC1 and IC2 display little variability across the entire clade. Considering the first two components of the PCA as a morphospace, Euclidean distances among all specimens were calculated and considered an appropriate indicator of their morphologic differences. Distances among specimens belonging to the same (extant) species were summarized using non-parametric probability density functions (Silverman Citation1986). Thus, this function represents the morphological variability within modern species. Then, the similarity between a fossil specimen with an extant species was evaluated calculating the distances between the fossil individual to each specimen belonging to a same (extant) species. This process resulted in a distance range that can be associated and compared with the summarized probabilities of the density functions of extant species. When the distance ranges from a fossil fell within the range of the summarized probabilities of an extant species, the assignment of the extinct specimen to this species is considered probable. However, if a non-measurable attribute (e.g. atypical morphology) is present in the fossil specimen, the comparison was considered unviable. All statistical analyses were conducted with R (R Core Team Citation2013) and all code employed for the analyses is available in the R script (Supplemental material 2).
Phylogenetic analysis. Relationships between extinct and extant species of Istiophoridae were examined using constrained and unconstrained phylogenetic analyses based on osteological data preserved in the fossil taxa. Forty-three characters (Supplemental material Table S2) were scored for 10 fossil and 11 living species of Istiophoridae. The matrix (Supplemental material 3) was created by combining 17 characters of the morphological matrix of Nakamura (Citation1983) and four characters of the matrix of Fierstine & Monsch (Citation2002) using Mesquite (Maddison Citation2017). Additionally, 22 new characters were defined and included in the data matrix (Supplemental material Table S2). Of these, nine characters are based on bibliographical revisions (Berry Citation1917; Fierstine Citation1978, Citation2006; Nakamura Citation1985; Schultz Citation1987; Collette et al. Citation2006), and 13 are new and identified by direct observations and by using the results of the PCA morphospace (). The morphological characters are supported by anatomical descriptions, ratios, measures and bibliographical information, and they are specified in each case (, Supplemental material Table S2). Two living species, Xiphias gladius and Acanthocybium solandri, were included as outgroup taxa for character polarization (Supplemental material Table S2).
The morphological dataset was analysed using parsimony and maximum likelihood approaches (, Supplemental material Figs S7, S8), as implemented in Paup v.40b10 (Swofford & Sullivan Citation2003) and iqtree v.1 (Nguyen et al. Citation2015), respectively. Thirty-eight characters were parsimony informative, and all characters were treated unordered and equally weighted. An unconstrained parsimony analysis based on a heuristic search with 100 replicates and tree branch swapping yielded 516 trees of 81 steps (CI = 0.7037, RI = 0.8481, RC = 0.5968, HI = 0.2962) that are summarized in a strict consensus tree (). Branch support was obtained from 1000 bootstrap replicates of full heuristic searches and decay indices were estimated from trees suboptimal by four steps (). For the maximum likelihood analysis of the morphological dataset we employed the Mk model, which is a simple model of discrete morphological character evolution that assumes equally probable transitions among all states of a given character (Lewis Citation2001), and an ascertainment bias correction (+ASC). Branch support was obtained from 1000 bootstrap replicates (Supplemental material Fig. S8).
The tree topologies recovered highlight a consistent discrepancy from recent phylogenies of Istiophoridae, indicating high levels of morphological convergence across lineages. As a means to overcome the confounding effect of homoplasy (Sanderson & Hufford Citation1996) on the relationships between living and fossil taxa, a topological constraint was enforced on parsimony and maximum likelihood analyses (, Supplemental material Figs S9). The constraint topology was based on the analysis of Williams et al. (Citation2018) of the relationships among Istiophorids based on the whole mitochondrial genome (see accession codes in Supplemental material Table S1), which recovers Makaira and Istiophorus forming a clade that is sister to all other Istiophoridae. For the constrained parsimony analysis, we used a heuristic search with 100 replicates and tree branch swapping. Only trees consistent with the topological constraint were kept, which resulted in 1851 trees of 107 steps that are summarized in Supplemental material Figure S9. The constrained likelihood analysis used the Mk model with an ascertainment bias correction, and branch support was estimated from 1000 fast bootstrap replicates ().
Body size and weight. We applied the equation of Prager et al. (Citation1995) to calculate the lower jaw fork length for M. nigricans with undetermined sex using length–length measures, as shown in Equationequation (1)(1)
(1) . We modified Equationequation (1)
(1)
(1) assuming that the total length (TL) equals the lower jaw fork length (LJFL) plus the VSPM distance (). Our modification therefore allows the body size to be estimated using fossil rostra that preserve the ventral aperture or have a measured value for the VSPM variable (). We used the constants (α and β) for M. nigricans with undetermined sex (see Prager et al. Citation1995 for the constant values), because its rostral morphology fits better with most of the studied fossils and to standardize the results. After introducing the VSPM variable in Equationequation (1)
(1)
(1) we solve the variable LJFL, which results in Equationequation (2)
(2)
(2) that also includes a correction factor (∁). We included the correction factor because the VSPM values of fossils are biased caused by the preservational state and due to the VSPM variable being an equivalent measure, but not an exact distance that complements the TL. We fitted the correction factor by using the observed TL = 266 cm of a nearly complete istiophorid skeleton (MUPPAN–STRI 31291) to establish its LJFL using Equationequation (1)
(1)
(1) . Subsequently, the observed LJFL (TL – VSPM) was divided by the calculated LJFL (of 105.02 cm) to produce the correction factor ∁ = 2.23. The calculated LJFL of each fossil specimen is used to obtain the TL by adding the VSPM distance. The body weight then was estimated using the estimated LJFL as predictor with the notation Ŵ = alb (see Prager et al. Citation1995 for additional details).
(1)
(1)
(2)
(2)
Systematic palaeontology
Subclass Neopterygii Regan, Citation1923
Infraclass Teleostei Müller, Citation1845
Cohort Euteleosteomorpha Greenwood et al., Citation1966
Subcohort Neoteleostei Rosen, Citation1973
Infracohort Eurypterygia Rosen, Citation1973
Section Ctenosquamata Rosen, Citation1973
Subsection Acanthomorphata Betancur-R. et al., Citation2013
Division Acanthopterygii Johnson & Patterson, Citation1993
Subdivision Percomorphaceae Betancur-R. et al., Citation2013
Series Carangaria Betancur-R. et al., Citation2013
Order Istiophoriformes Betancur-R. et al., Citation2013
Family Istiophoridae Robins & de Sylva, Citation1960
Clade Gracilorostra nov.
Genus †Morgula gen. nov.
Type species. †Morgula donosochagrense gen. et sp. nov.
Diagnosis. As for the species by monotypy ().
Figure 5. A–C, G–I, †Morgula donosochagrense gen. et sp. nov., holotype (MUPAN–STRI31293); A, rostrum dorsal view; B, rostrum left lateral view; C, rostrum ventral view; G–I, lower jaw showing articulate dentaries; G, ventral view; H, dorsal view; I, disarticulated right partial dentary in lateral view showing the interdentary joint and the mandibular foramen. D–F, †Makaira fierstini sp. nov., holotype, USNM 358534; D, rostrum dorsal view; E, rostrum ventral view; F, detail of the right lateral view of the rostrum at its proximal end. Missing parts of the bone are indicated by dash-lines. Abbreviations: mf, mandibular foramen; N, nasal; PM, premaxilla; PN, prenasal. Scale bars: A–F = 10 cm, G–I = 5 cm.
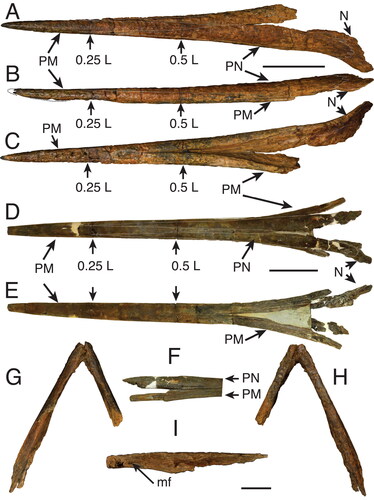
Derivation of name. Latinization of the Sindarin elvish noun ‘morgul’, that means black magic, dark sorcery and refers to the ‘Morgul blade’, a legendary dagger (similar in shape to the fossil) from the novel ‘Lord of the Rings’ by J. R. R. Tolkien.
Occurrence. Late Miocene (6.4–5.8 Ma), Chagres Sandstone member, Chagres Formation, Colon, Panama.
†Morgula donosochagrense sp. nov.
()
Diagnosis. The new species is characterized by the following combination of morphological traits (autapomorphic characters are indicated by an asterisk): slender rostrum along its entire length combined with a circular cross-section at 0.25 L (); premaxillae divided into two separate bones for about 60% of the total rostral length*; the internal canals circular () in ventral position (); rostrum elongated triangular in dorsal view*; nasals angularly extended laterally ()*.
Figure 6. A, B, †Morgula donosochagrense gen et sp. nov. (MUPAN–STRI31293); A, cross-section at 0.5 L; B, cross-section at 0.25 L. C, D, †Makaira colonense sp. nov. (MUPAN–STRI39292); C, cross-section at 0.5 L; D, cross-section at 0.25 L. Abbreviation: PN, prenasal. Scale bars = 1 cm.
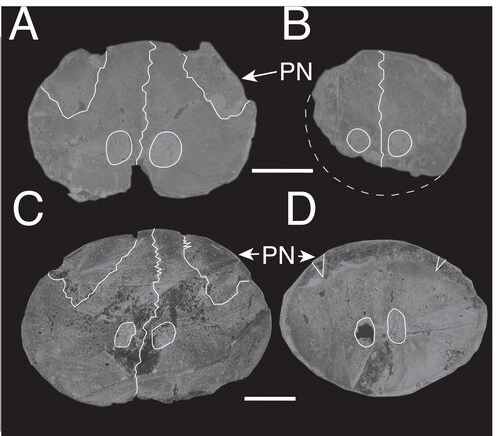
Derivation of name. The species name ‘donosochagrense’ is coined from a combination of the words ‘Donoso’ and ‘Chagres’, the names of the two districts in which the outcrops of the Chagres Formation are located including parts of the natural reserve of Donoso.
Holotype. MUPAN–STRI31293, a nearly complete rostrum () with associated lower jaw lacking the predentary ().
Occurrence. The specimen was collected in 2011 from a sea cliff exposure on the beach of the town of Piña (), about 20 metres west of 9°9′16ʺN; 80°2′71ʺW. The fossiliferous unit is ∼5 m above a massive bioturbated layer that is exposed at tide level.
Remarks. The specimen was found in a weathered block above the tide zone. The surface of the fossil consequently is worn () and alveoli for denticles were not recognized. The specimen includes an associated lower jaw () that was found in the same block during preparation.
The rostrum of †Morgula donosochagrense gen. et. sp. nov. is nearly complete and consists of prenasals, premaxillaries and a complete left nasal (). The posterior edge of the left nasal is in the same transverse plane as the lateral ethmoid (), which allows a comparison with other taxa (Fierstine Citation1999a). The overall preserved length of the rostrum is 611 mm and only c. 13 mm of the distal tip are missing. The estimated total bill length therefore is 624 mm (Supplemental material 1). The rostrum’s cross-section is oval at 0.5 L (307 mm from the tip) but circular at 0.25 L (155 mm from the tip []; see the out-range ratio D2/W2 in ). The distal tip has a slender outline (, Supplemental material S1B), is acute and dorsoventrally compressed. The premaxillaries start to separate at 248 mm (VSPM) measured from the distal tip (). The VSPM distance represents approximately 30% of the total rostrum length and coincides with the point where the ventral canal starts to be visible in cross-section at 0.5 L (). The ventral aperture (L – VSPM) represents 60% of the rostral length (). In dorsal view, the nasals are angularly oriented posterolaterally (). The internal canals are large, ventrally located, centrally aligned, and with a circular cross-section (; ). The rounded internal canals that are ventrally placed are autapomorphies of †Morgula donosochagrense gen. et sp. nov. (), as is the triangular shape of the rostrum and the nasal bones that are angularly extended laterally in dorsal view (, ). Other istiophorids have oval internal canals in a central or dorsal position (see for examples and Supplemental material Fig. S2 for definitions).
Figure 7. A–L, comparison among selected fossils and extant marlin species with emphasis on the rostral morphology and its cross-sections at 0.5 and 0.25 L; A, reconstruction of the rostrum of †Prototetrapturus courcelli gen. et. comb. nov. (MNHN P250); B, reconstruction of the rostrum of †Spathochoira calvertense gen. et. comb. nov. (USNM 9344); C, †Morgula donosochagrense gen. et sp. nov. (MUPAN–STRI31293); D, †Makaira colonense sp. nov. (MUPAN–STRI31292); E, reconstruction of the rostrum of Makaira panamense (USNM 181710); F, reconstruction of the rostrum of †Makaira belgica (MAUL 917/1) from the late Miocene of Italy; G, †Makaira fierstini sp. nov. (USNM 358534); H, Makaira nigricans (USNM 196019); I, Istiompax indica (LACM 25509); J, Kajikia albida (USNM 360507); K, Kajikia audax (USNM 372777); L, Istiophorus platypterus (MNHN A-9463). The white circle indicates the point where fused prenasals start to divide in two separate bones, if this point is more distal, the narial cavity is longer and rostrum is more open. Arrows indicates where premaxillaries protrude from prenasals in Makaira ssp., the outer projection of the prenasals is represented by an angle symbol. Cross-sections at 0.5 and 0.25 L: blue is oval shape, light blue is oval shape compressed laterally (borders could be more angular instead of smooth and prenasals are embedded into premaxillaries; see ), green is round, yellow is oval highly depressed (almost plane), red represents other shapes (see ). Pink water mark indicates the reconstructed sections. Different rostrums are labelled according the observed or inferred feeding strategy. Thrusting refers to rostrums used mostly for direct impacts and specific refers to rostrums used in multidirectional, lateral or complex feeding strategies. Scale bars = 10 cm.
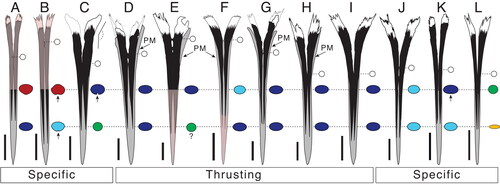
Lower jaw. The associated lower jaw is represented by partial right and left dentaries (). In the articulation of the dentaries, two variables (DAD and DJL) were studied and converted into a ratio (). The ratio DAD/DJL for †Morgula donosochagrense gen. et. sp. nov. is 0.52 () and this value represents a shape that is more rectangular than quadratic distally where both dentaries join (). The general appearance of the dentaries is slender with a height at the interdentary joint of 21 mm (DAD distance) and of 38 mm at the level of the articular socket for the head of the quadrate. The right dentary is nearly complete with its proximal part missing ventrally for half of its length (), while its dorsal surface is completely preserved (). The left dentary is partially preserved but broken in its middle part at the location of the articular joint (). In dorsal view, the dentary is covered by denticles from its most distal part to about the level of the articular socket (). There is no further evidence of denticles from this point to the most proximal end. The surface that is covered with denticles presents circular structures of 3–4 mm that are possibly alveoli for larger teeth (). There are one and three of these structures visible on the right and left dentary, respectively ().
Genus †Spathochoira gen. nov.
Type species. †Spathochoira calvertense comb. nov. (Berry, Citation1917).
Diagnosis. As for the species by monotypy.
Derivation of name. The taxon name is formed by the combination of the Latin word ‘spatha’ meaning ‘sword’ and the Greek word ‘choiros’ meaning ‘pig’, because of the characteristic cross-section at 0.5 L (), which superficially resembles the nose of a pig in anterior view.
Occurrence. Late Miocene, Eastover Formation, Virginia, USA.
†Spathochoira calvertense (Berry, Citation1917) comb. nov.
()
Figure 8. A–D, Istiophoridae holotypes represented by fragmented rostrums in dorsal, right lateral and ventral view; A, †Spathochoira calvertense gen et. comb. nov. (USNM 9344), holotype; B, Makaira belgica (IRSNB P1117), holotype; C, †Prototetrapturus courcelli gen et. comb. nov. (MNHN P250), holotype; D, Makaira purdyi (USNM 481933), holotype. Dashed black lines show the positions where the sections at 0.5 L and 0.25 L have been studied. White dashed lines indicates the prenasal sutures. Scale bar = 5 cm.
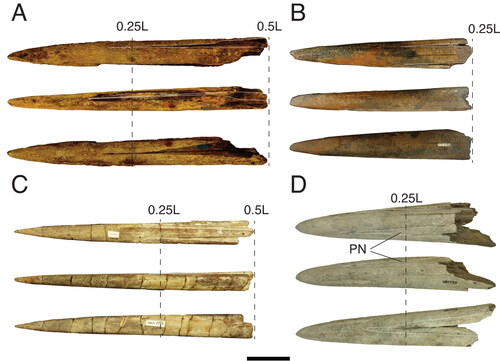
Figure 9. A–E, Cross-sections of fossil istiophorids. †Prototetrapturus courcelli gen. et. comb. nov. (MNHN P250): A, cross-section at the proximal end of the rostrum; B, cross-section at 92 mm measured from its proximal end; C, cross-section at 139 mm measured from its proximal end; D, cross-section at 200 mm measured from its proximal end; E, cross-section at 260 mm measured from its proximal end, F, †Makaira purdyi (USNM 481933), cross-section at 0.25 L distance. G, †Makaira panamense (USNM 481933), cross-section approximately at 0.5 L distance (prenasal sutures are not shown due to the poor preservation state). H, I, N, †Makaira belgica, MAUL 917/1; H, cross-section at 0.5 L and I, 0.25 L distance; IRSNB P117; N, cross-section at 0.25 L distance (prenasal sutures are not shown due to the poor preservation). J, K, †Spathochoira calvertense gen et. comb. nov. (USNM 9344) at J, 0.5 L and K, 0.25 L; L, M, extant I. platypterus at L, 0.5 L and M, 0.25 L; O, †Xiphiorhynchus solidus (IRSNB P643), cross-section at the proximal end of the rostrum. Dashed lines show reconstruction of missing sections. The black arrow in I, shows the dorsal placement of the internal canals. In J, K, the arrows show the ventral external canal in cross-section at 0.5 L and 0.25 L distance. Scale bars = 1 cm.
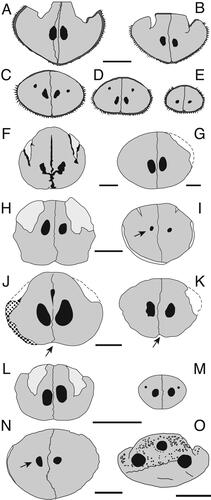
1917 †Istiophorus calvertensis Berry: 461, figs 1, 2.
1987 †Pseudohistiophorus calvertensis (Berry), Schultz: 120, tab. 2.
1990 †Makaira calvertensis (Berry); Fierstine: 15, tab. 2.
Diagnosis. This taxon is characterized by the following combination of morphological traits (an asterisk indicates autapomorphic traits): a sub-cylindrical and anteriorly gradually tapering rostrum with extremely large internal canals and that are oval in cross-section in the most distal part; in having a concave ventral margin in the anterior two-thirds forming a pronounced ventral canal along the whole length of rostrum resulting in a cross-sectional shape reminiscent of a pig nose at 0.5 L*; prenasals that terminate close to distal tip*.
Holotype. USNM 9344, a 310 mm long distal rostral fragment.
Occurrence. Late Miocene, Eastover Formation, Tar Bay, James River, Virginia, USA (see also Fierstine Citation1998, Citation2001, Citation2006).
Remarks. †Spathochoira calvertensis gen. nov. is a 310 mm long distal fragment of a rostrum that is dorsally covered by poorly preserved denticles at 57.3 mm from its distal tip (Fierstine Citation1998, Citation2001). The rostrum is sub-cylindrical in cross-section, tapering gradually until the cross-section becomes oval in outline towards the most distal part (Berry Citation1917) (). The distal tip is slender and resembles that of Istiompax indica (). There are two grooves on the dorsal surface that represent the sutures for prenasals (). These grooves decrease gradually distally until they disappear completely near the distal tip (P distance) (Berry Citation1917). The ventral surface of the rostrum is concave in its anterior two thirds and there is a pronounced median suture that forms a distinct canal identifiable along the whole length of the rostrum (Berry Citation1917) (). The rostrum is broken proximally and the transversal fracture represents the 0.5 L distance () (Fierstine Citation2001). A pair of large internal canals is visible (), which are located at 11.0 mm from the dorsal surface of the rostrum (DD1 distance) (). The internal canals are very large based on their proportion relative to the rostral depth (D1) (). The position of the canals () is medial and they have a central arrangement following our terminology (Supplemental material Fig. S2). The canals are levogyres (Supplemental material Fig. S2I) rotated about 40°, they are wider inferiorly and separated from each other by 1.0 mm (Berry Citation1917). In the midline suture, c. 7.0 mm dorsomedial to the canals, a central oblanceolate foramen with a 1.0 mm diameter is present () (Berry Citation1917; Fierstine Citation2001). The cross-section at 0.25 L for this specimen was studied with the help of CT scans and estimated to be 134 mm from the distal tip (Fierstine Citation1998, Citation2001) (). The CT results show that the rostrum is oval, the ventral convexity is visible, and the internal canals are ovals and proportionately large (). The internal canals at this position are in a medial position, centrally arranged and are 5.72 mm height (H2), 3.29 mm wide (N2) and are separated by 2.0 mm bone (Fierstine Citation1998, Citation2001) (, Supplemental material 1).
Table 4. Estimated body size (LJFL and TL) in fossil istiophorids using the VSPM distance as an allometric predictor. Species range suggests which extant species or genera fit better with the fossil specimen according the morphology and by the size ranges established by Nakamura (1983). Abbreviations: LJFL, lower jaw fork length; TL, total length; VSPM, length from the distal tip to the point where the fussed premaxillaries start to divide in two separate bones.
Diagnosis. †Makaira fierstini sp. nov. is characterized by the following autapomorphy (autapomorphic characters indicated by an asterisk): in lateral view, lateral planes are present at the level of the triangular process of the premaxillaries that extend up to half of its total height*. Makaira fierstini sp. nov. can also be differentiated by the following combination of characters: the rostrum is straight along its entire length; prenasals never contact each other in dorsal view; denticles cover the entire lateral and ventral surfaces; large internal canals present.
Derivation of name. Named in honour of the late Harry Fierstine (1932–2021) in recognition of his contributions to our understanding of the fossil record of marlins and billfishes.
Holotype. USNM 358534, a nearly complete rostrum, 794 mm long.
Occurrence. Late Miocene (12.0–9.2 Ma), lower Gatun Formation (Collins et al. Citation1996; Hendy Citation2013). Sabanitas, Colón, Panama at 43°38′19.39ʺN + 675 m, 116°14′28.86ʺW + 1600 m, (Fierstine Citation1999a). The type locality is not accessible anymore, as it is currently a housing development.
Remarks. The rostrum of †Makaira fierstini sp. nov. (USNM 358534) is almost complete. The premaxillaries and prenasals are well preserved, whereas the nasals are poorly preserved (). The estimated total length is 794 mm, of which 45 mm of the distal tip, however, are missing (Fierstine Citation1999a). The rostrum is slender and has an oval outline in cross-section at 0.5 L and 0.25 L distances (). In dorsal view, the prenasals never contact each other or the premaxillaries (). In lateral view, the rostrum is completely straight with no detectable distal curvature (Fierstine Citation1999a). The premaxillaries are laterally planar at their proximal ends and occupy half of the total lateral height (). The rostral morphology indicate that the distal tip is slender and pointed (Supplemental material Fig. S1B). In ventral view, the premaxillaries begin to be separated at 483 mm from their distal tips (VSPM) (). The nasals are only partially preserved (). The internal canals are in a medial position, centrally aligned and have oval cross-sections (Fierstine Citation1999a). †Makaira fierstini sp. nov. has proportionally large internal canals (). The statistical analysis () indicates that the rostrum morphology of †M. fierstini sp. nov. is close in morphology to M. nigricans and Is. indica (), but it differs from them in its extremely long rostrum, shorter narial aperture, the possession of large internal canals () and by having planar premaxillaries that occupy half of the lateral height in its proximal part ().
Figure 10. A, PCA morphospace for MAR obtained values from 110 modern marlin individuals distributed in five extant species and 11 fossil specimens. B, probability density function for five modern marlin species and its probabilistic associations with fossil specimens based on the PCA analysis. The PCA explains 90.9% of total variation in the first two axes. In B, the numbers correspond with those in the PCA analysis for species names. Grey lines are the 95% confidence intervals for extant species and the lines represent the distances of each fossil individual. For the three individuals identified as Istiophoridae sp. 1 the correspondence between the numbers in parenthesis and the collection number is: MAUL 57/1 (3), MUPPAN– STRI 31291 (6) and OCPC 31001 (7).
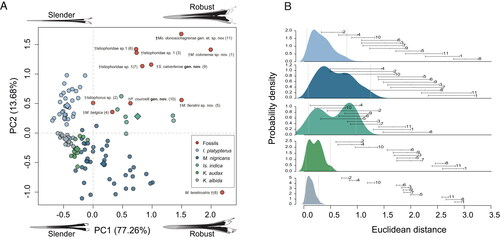
†Makaira colonense sp. nov.
(, Supplemental material Fig. S5B)
Figure 11. A–C, †Makaira colonense sp. nov. (MUPAN–STRI 39292), rostrum; A, dorsal view; B, right lateral view; C, ventral view. D–G, †Makaira panamense (USNMN 18710), holotype; D, skull in dorsal view; E, skull ventral view; F, left lateral skull view; G, occipital region. Scale bars: A–C, F = 10 cm; D, E = 20 cm; G = 4 cm.
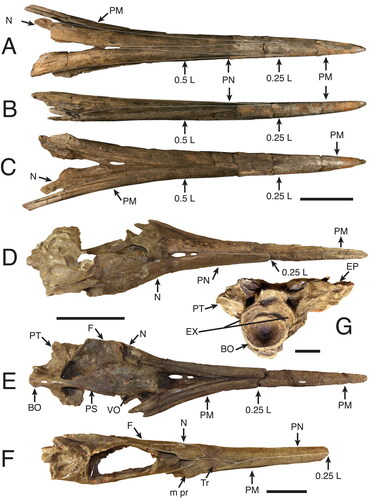
Diagnosis. An extinct species of Makaira characterized by the following combination of morphological traits (autapomorphic characters indicated by an asterisk): osteosclerotic rostrum and narial cavities enclosed by premaxillaries, prenasals and dermethmoid*; prenasals in dorsal view well separated and the narial cavity is divided into two portions by extensions of the prenasals; large internal canals; from the most proximal end to about 0.5 L distance, the premaxillaries are convex and projected outward along the lateral surface; in lateral view, the premaxillaries occupy about ¾ of the total height at the contact point between prenasals, premaxillary and maxilla.
Derivation of name. The species name ‘colonense’ refers to the Colon province of Panama and is used due to the importance of this province for Panamanian palaeontology.
Holotype. MUPAN–STRI39292, a large nearly complete rostrum.
Occurrence. Late Miocene (6.4–5.8 Ma), Chagres Sandstone Member, Chagres Formation. Bioturbated horizon on Piña beach () at 9°17′24.89ʺN, 80°2′25.39ʺW, near the town of Piña in the Chagres District, Colón Province, Panama.
Remarks. The rostrum of †Makaira colonense sp. nov. (MUPAN–STRI39292) is nearly complete and consists of complete prenasals and premaxillaries with partially preserved nasals (). The estimated length is 672 mm. In dorsal view, the premaxillaries extend over the prenasals () in the proximal part of the rostrum. The narial cavity is divided into two parts separated by extensions of the prenasals (Supplemental material Fig. S5B). In dorsal view, premaxillaries overcomes forming the outline () instead the prenasals (–L). In lateral view from the most proximal point to about 0.5 L distance, the premaxillaries are extremely thickened and extended laterally, forming a convex projection (). This smooth and rounded projection gives its distinctive shape to the rostrum (). The rostrum is osteosclerotic and stout, with an oval outline in cross-section at 0.5 L and 0.25 L distances (). The distal tip has a globular outline, is wide and curved slightly upwards in lateral view (). In ventral view, the premaxillaries begin to be separate at 367 mm from their distal tips (VSPM) (). This distance represents approximately 54% of the total length of the rostrum. The nasals are poorly preserved. The internal canals are large and have an oval outline, located in a medial position and are centrally aligned (; ). Both statistical analyses () place the rostrum of †M. colonense sp. nov. outside the group formed by all extant species and only can be confused with †M. panamense. However, in †M. colonense sp. nov. the prenasals conversely never contact each dorsally (, S5B) and the premaxillaries form the posterior enclosure of the narial cavity (Supplemental material Fig. S5B).
†Makaira belgica (Lériche, Citation1926)
()
1926 †Brachyrhynchus belgicus Lériche: 443, figs 210, 211.
1978 †Istiophorus belgicus (Lériche) in Fierstine: 8.
1990 †Makaira belgicus (Lériche) in Fierstine: 15, table 2.
Diagnosis. This species is characterized by dorsally positioned internal canals in the rostrum, which represents an autapomorphic trait for this species.
Holotype. IRSNB P1117, holotype is a 200 mm-long distal rostral fragment (Lériche Citation1926). Middle Miocene, Anvers, Belgium (Lériche Citation1926; Schultz Citation1987).
New referred material. MAUL 917/1, is a 500 mm-long, nearly complete rostrum with associated left lower jaw and poorly preserved and deformed skull from the late Miocene of Pietra Leccese Formation, Apulia, Italy (Carnevale et al. Citation2002).
Occurrence. Middle Miocene (Burdigalian) to late Miocene (Tortonian).
Remarks. †Makaira belgica represents the earliest record of the genus Makaira. The holotype is represented by a 200 mm distal rostral fragment (), which is robust and oval in cross-section (). At 0.25 L, it has a pair of small and circular internal canals, which are arranged dorsally and parallel along the midline (). In the new specimen MAUL 917/1 referred to this species, the prenasals and maxillae are well preserved but the nasals are only partially preserved. In the holotype the nasals are only represented by grooves () (Lériche Citation1926; Schultz Citation1987). Alveoli and denticles are visible across the completely ventral surface in both specimens () (Carnevale et al. Citation2002).
The studied ratios of †M. belgica at the 0.5 L section fall within the rage of recent and fossil M. nigricans, but differ in its dorsally aligned internal canals (, ) (Lériche Citation1926; Schultz Citation1987). This feature represents the only autapomorphy for this species (). The holotype (IRSNB P1117) of †M. belgica is represented by a poorly preserved distal rostral fragment and we assign a second specimen, MAUL 917/1, to this taxon, because both specimens have dorsally arranged internal canals (), representing an autapomorphic trait for this species. Specimen MAUL 917/1 has internal canals in a dorsal position at 0.25 L (; see also Carnevale et al. Citation2002), which is consistent with what is seen in the holotype of †M. belgica (). Specimen MAUL 917/1 additionally has distinctive ratios for D2/VSPM and W2/VSPM. These out-of-range values are interpreted as short VSPM that covers less than 50% of the rostrum length.
†Makaira panamense Fierstine, Citation1978
1978 †Makaira panamensis Fierstine: 2, figs 1, 2, 4c.
Emended diagnosis. An extinct species of Makaira characterized by the following combination of traits (autapomorphic characters indicated by an asterisk): triangular basioccipital process*; massive prenasals that in dorsal view are strongly fused at the 0.5 L region forming a solid structure*; narial cavities divided in two voids and distinctively enclosed only by prenasals*.
Holotype. USNM 18710, a large neurocranium with a poorly preserved rostrum with an estimated length of 953 mm (G).
Occurrence. Only known from the late Miocene (6.4–5.8 Ma). Chagres Sandstone Member, Chagres Formation, Colon, Panama. The precise site of collection is unknown.
Remarks. The holotype is a large partial neurocranium with a poorly preserved rostrum, with an estimated length of 953 mm (). Only the left anterior half of the skull roof and one fourth of the distal rostrum are missing (). The specimen is slightly dorsoventrally compressed and twisted (). The neurocranium is wide, squared and depressed (). The orbit is elongated () and the dorsal wall of the myodome is wider than high in the sidewalls. The vomer and the parasphenoid also are wide. The vomer has a small anterior projection and the angle of the parasphenoid is acute (). The basioccipital process is triangular, rather than elongated (), displays low paired ridges on the dorsal surface of the supraoccipital and the dorsal surfaces of the exoccipital form together a board posterior projection. For additional, more detailed descriptions of the skull see also Fierstine (Citation1978).
The rostrum of †Makaira panamense (USNM 18710) is broken at c. 0.5 L distance (). The distal-most part of the rostrum (0.25 L) is weathered, very poorly preserved and the distal tip is missing so that it is not possible to observe sutures (). The estimated length is 953 mm based on the preserved portion () (Fierstine Citation1978). In dorsal view, the premaxillae overlap the prenasals at their proximal end and the narial cavity is enclosed only by the prenasals (, Supplemental material Fig. S5A). The rostrum is massive and stout, and has an oval outline in cross-section at 0.5 L (). The internal canals are relatively large (), located medially, centrally aligned and have an oval outline (). At the proximal end of the rostrum, the prenasals are massive, contact each other along the midline (Supplemental material Fig. S5A), and are strongly fused forming a solid structure (, Supplemental material Fig. S5A). Makaira panamense was not analysed in the PCA because all features at 0.25 L are lacking because of the incomplete distal section of the rostrum.
†Makaira purdyi Fierstine, Citation1999b
()
1999a †Makaira purdyi Fierstine: 76, figs 1–4, table 1–4.
Emended diagnosis. †Makaira purdyi is differentiated by the following combination of morphological traits (autapomorphic characters indicated by an asterisk): rostrum higher than wide in cross-section*; denticles covering the dorsal surface of the rostrum including the prenasals*; rostrum short; prenasals terminate close to distal tip.
Holotype. USNM 481933, a partial rostrum without nasals and a preserved total length of 480 mm.
Occurrence. Early to late Pliocene (∼4.8–3.1 Ma), Yorktown Formation (Snyder et al. Citation1983). Lee Creek Mine, located near Aurora, Beaufort Country, eastern North Carolina, USA (Fierstine Citation1999b).
Remarks. The only fossil of this species is an unusually short (480 mm) and stout bill () that is oval in cross-section with a globular distal tip (). The most diagnostic characteristic is the presence of prenasals that terminate nearly at the most distal tip (). The rostrum is massive and differs morphologically from any extant or fossil istiophorid rostrum by having short and strongly fused premaxillae with denticles covering at least half of its dorsal surface (). The internal canals are large, oval and levogyres, ventrally positioned and centrally arranged at 0.25 L distance (; ). For a more complete description of †Makaira purdyi see Fierstine (Citation1999b).
†Makaira teretirostris Rütimeyer, Citation1857
()
Figure 12. A, †Makaira teretirostris (Van Beneden, Citation1871); original diagram (Van Beneden Citation1871) with detailed cross-sections highlighted that show a cylindrical shape. B, C, Tetrapturus sp. from the late Pliocene of Orciano Formation; B, rostrum in dorsal view; C, rostrum in ventral view. Scale bars A = 10 cm, B = 5 cm.
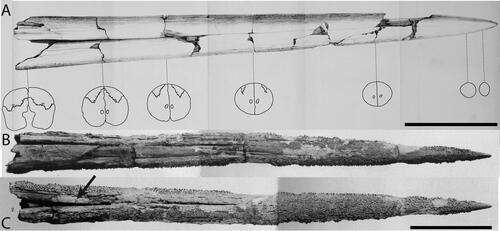
1857 †Encheiziphius teretirostris Rütimeyer: 561, pl. i.
1871 †Brachyrhynchus teretirostris Rütimeyer; Van Beneden: 495, pl. i − ii, figs 1, 2.
Emended diagnosis. This species is characterized by the following single autapomorphic character: rostrum cylindrical (or round in cross–section) with equal height and width at 0.5 L, giving it a characteristic conical aspect.
Holotype. Specimen is currently missing, and the exact point of collection is unknown; it is represented by a 520 mm nearly complete rostrum (Van Beneden Citation1871, pl. 1).
Occurrence. Pliocene sandstones, Montpellier, France (Roman Citation1922). Early Pliocene Zanclean, Sables à Gryphaea virleti Formation (Bianucci et al. Citation2008).
Remarks. The description here is based on a natural scale drawing of a nearly complete rostrum in ventral view accompanied by seven schematic drawings of cross-sections () provided by Van Beneden (Citation1871). In cross-section, the rostrum is nearly circular at 0.5 L and gradually tapers towards its distal end (). The distal tip is almost circular basally, becomes oval in the middle part and ends in a rounded point (). The distal tip has a globular shape, while the transverse outlines at 0.5 L and 0.25 L indicate a cylindrical rostrum in cross-section (; Supplemental file 1). The rostrum has two continuous, oval, small internal canals that are centrally aligned (). The H1 variable suggests large, whereas the CA1/AR1 ratio suggest small canals (). In ventral view, there is a pronounced canal that is visible from the most proximal part of the rostrum to about half the distance of the specimen (). The measurements of †M. teretirostris, which were published by Van Beneden based on a cast (L = 522 mm, W1 = 52 mm), differ from those of the natural size illustration (). The measurements taken from the original illustration are the same as those published by Rütimeyer (Citation1857): L = 530 mm, W1 = 58 mm, D1 = 50 at the proximal end. By converting these measurements in ratios, five out of the six ratios of †M. teretirostris are in the range of the extant M. nigricans (see supplemental file 1 for unconverted values). The ratio D1/W1 is the only value that differs from those of M. nigricans as shown in the PCA, where †M. teretirostris falls outside of extant species representing an outlier () and seemingly is unrelated to any extant species (). The ratio D1/W1 = 0.88 indicates that the rostrum is rounder than oval at its base and represents the biggest value observed for these ratios in all studied specimens (Supplemental material 1).
Clade Tetrapturomorpha nov.
Genus †Prototetrapturus nov.
Type species. †Prototetrapturus courcelli (Arambourg, Citation1927) comb. nov.
Diagnosis. As for the species by monotypy.
Derivation of name. The genus name is a combination of the Greek word ‘protos’ meaning ‘first’ and the generic name Tetrapturus, thus meaning ‘the first Tetrapturus’, referring to its relationship to spearfishes.
Occurrence. Late Miocene (Messinian) of Algeria (6.4–5.8 Ma).
†Prototetrapturus courcelli (Arambourg, Citation1927) comb. nov.
1927 †Xiphiorhynchus courcelli Arambourg: 173, pl. 36/6, fig. 40.
1978 †Istiophorus courcelli (Arambourg); Fierstine: 8.
1987 †Makaira courcelli (Arambourg); Schultz: 120, 140, 148, 172, tabs. 2, 3, figs 6A, 7C, 8A–E, S1, S4.
Diagnosis. †Prototetrapturus courcelli comb. nov. is differentiated from all other istiophorids in the following combination of morphological traits (an asterisk indicates autapomorphic traits): very slender rostrum with acute distal tip; an oval to angularly obtuse cross-section*; two additional, small and dorsolaterally positioned internal canals in dorsal position and laterally distributed*; denticles on the lateral sides that are distinctly large, conical and pointed* (Supplemental material Fig. S4).
Holotype. MNHNP 250 (), a nearly complete rostrum without nasals.
Occurrence. Late Miocene (Messinian), pre-evaporitic period of the Tripoli Unit, locality of ‘Les Planteurs’, Algeria (Landini & Menesini Citation1984; Carnevale et al. Citation2002; Gaudant Citation2008).
Remarks. The preserved rostrum is 295 mm long, rather slender, with a generally elliptical cross-section (wider than tall) (). In cross-section, two continuous and large internal internal canals are visible (; ). In lateral view, the rostrum is straight dorsally, but slightly curved upwards ventrally (). It is covered partly by conical denticles that are restricted to the ventral and lateral surfaces of the specimen. The denticles located on the lateral sides are the largest, while those located on the ventral surface are smaller (Supplemental material Fig. S4).
The cross-sections of the rostrum change along its length and each section is described here in the same sequence as in Arambourg (Citation1927). The first section is represented by the fracture at the proximal end of the rostrum and is equivalent to the 0.5 L distance (). At this distance, the rostrum is oval in cross-section but with an angularly obtuse ventral surface. The shape for the second section () is similar to the first section. The obtuse ventral angle forms a convex surface along the ventral surface of the rostrum that decreases gradually as it advances distally up to approximately 139 mm (if measured from the proximal end) (). At this distance, the third section is located and is equivalent to that at 0.25 L (). Here the rostrum is oval and two smaller canals located dorsolateral to the centre of the bone are visible (). These small canals are visible for about 62 mm from this point extending dorsally before they gradually disappear distally. The fourth section is located at 200 mm (). It is oval but with a planar ventral margin and the second pair of internal canals are visible (). From this point (at 200 mm) to the distal tip, the ventral planar surface extends for about 46 mm, until the rostrum acquires an oval shape anew in cross-section. The last section is located at 260 mm (). Here the lateral sides (when observed in cross-section) are compressed and the distal tip acquires a slender and very pointed shape ().
Material. Rostrum N°1, which Barbolani di Montauto (Citation1910) figured on pl. 1, figs 1, 2.
Occurrence. Late Pliocene of Orciano Formation, Pisa, Italy.
Remarks. Unfortunately, the single specimen was not directly available for this study and the description provided here is based on the published figures by Barbolani di Montauto (Citation1910). The specimen is a distal, nearly complete and 300 mm long rostrum fragment with weathered dorsal surface (). Only the premaxillae are preserved, the prenasals are completely missing and represented only by the distal grooves (). The proximal portion of 100–150 mm is missing. The preserved portion is very slender and oval in cross-section at 0.5 L and 0.25 L (). The distal tip is slender and pointed with denticles preserved for about 50 mm of its length. The rostrum is completely covered with denticles laterally and ventrally () (Barbolani di Montauto Citation1910; Schultz Citation1987; Fierstine Citation1990).
1987 †Thalattorhynchus austriacus Schultz: 151–152, abb. 3, 4, 5/78, pl. 1, figs 1, 11, fig. 17.
1998 †Thalattorhynchus austriacus Schultz: 128, pl. 58, fig. 6 (Schultz Citation1998).
2013 †Thalattorhynchus austriacus Schultz: 373/2, pl. 70, fig. 4a–d (Schultz Citation2013).
Material. NHMW 1986/109, a distal section of rostrum of 79.30 mm long.
Occurrence. Middle Miocene (∼16.3–12.8 Ma), Badenian Paratethys stage, Marzer Kogel, SE Mattersburg, Burgenland, Austria.
Remarks. The specimen is a slender distal rostral fragment of 79.3 mm length that represents the 0.5 L region (Supplemental material Fig. S6A). The distal tip is pointed and preserves the distal end of the grooves that articulate with the prenasals in dorsal view (Supplemental material Fig. S6A). In lateral view, the rostrum is straight with a slightly upward curvature (Supplemental material Fig. S6A). The distal fragment is entirely covered by denticles ventrally along its entirely preserved length and in lateral view the zone covered by denticles is extended about ¾ of the total height (Supplemental material Fig. S6A). The denticles, which are housed in alveoli, are conical and range from 0.79 to 1.19 mm in height (Supplemental material Fig. S6A, B). Those placed on the lateral edges are larger than those positioned in the middle section (Supplemental material Fig. S6A).
In cross-section the rostrum is oval (W2 = 17.51 mm, D2 = 13.72 mm) and the suture of the premaxillae is visible but secondarily filled with gypsum (Supplemental material Fig. S6B). It has only one internal canal that is circular in shape and placed ventrally at about 1.75 mm of the diameter (Supplemental material Fig. S6B). This specimen was described as Thalattorhynchus austriacus representing a xiphiorhynchid billfish based on having a single longitudinal canal as autapomorphy (Schultz Citation1987). However, the preserved rostrum has a morphology that corresponds to members of the family Istiophoridae possessing slender rostra (Supplemental material Fig. S6A, B). The evidence shows that some extant istiophorid specimens may lack one internal canal in the rostrum as an anomalous condition and with the preserved section, it is not possible to detect additional autapomorphies to correctly assign this specimen to any genus or species (Fierstine Citation1990; Fierstine & Voigt Citation1996). However, we nevertheless include this specimen here to highlight the importance of this specimen because demostrates the existence of slender rostra istiophorids during the middle Miocene.
Discussion
Rostral morphology
The istiophorid rostrum is a polygonal structure with a triangular shape in dorsal, lateral and ventral views (). Most of the studied rostral variables (e.g. length) scale linearly, allowing estimation of the lengths of missing portions (Supplemental material Fig. S3). The triangular shape and the scaling of measurements facilitates the reconstruction of incomplete specimens using diagonal linear projections from the preserved parts () (Fierstine & Voigt Citation1996; Fierstine Citation1998, Citation1999a, Citation2001). As demonstrated by Fierstine & Voigt (Citation1996), the application of ratios helps us to better understand interspecific shape changes at 0.5 L and 0.25 L of the rostrum (; ). The ratios are also used to establish the multivariate PCA analysis applied to the rostral variables and to visualize how these changes occur by grouping individuals with similar shapes (). As result, the PCA analysis produced a morphospace () that was used to create density functions (). These density functions were effective in showing the intraspecific variation of rostral shape in extant species and discriminating extinct forms from the extant species (). Our PCA morphospace demonstrates that the istiophorid rostrum has a strong phylogenetic signal by grouping the individuals of extant marlin species () and support our fossil descriptions and the phylogenetic traits based on rostra (Supplemental material Table S3; ).
Figure 4. Maximum likelihood constrained topology based on Williams et al. (Citation2018) for istiophorid billfishes. Colours indicate different clades that are correlated with different types of rostra and behaviours based on extant species. The definition for the rostra shape is detailed in the character matrix (Supplemental material Table S2). The skull figures in ventral view show the morphology of the neurocranium, vomer and parasphenoid in different clades. The colours for skull bones are explained in . The rostrum and drawings on the button show how each type of rostra correlated with a different feeding behaviours based on observations in extant species. The silhouettes of the three main types of rostra described are compared with drawings of different types of swords for better understanding of the rostral morphology. Green is extremely thin rostra similar to a fencing sword; blue illustrates robust rostrums compared with a ‘gladius hispaniensis’ or ‘Mainz-type swords’, as shown in Bishop (Citation2016, pp. 18–21); red shows slender rostrums compared with a Viking sword (see Oakeshott [Citation1960] for examples of this type of sword) and orange indicates the same rostrum type as red. Colours also show the convergence of different rostral, vomer and parasphenoid shapes in our topology. The Tetrapturus clade (orange) includes species with slender rostra similar to the Gracilorosta clade (red) but no information about the feeding behaviour in spearfishes is available.
![Figure 4. Maximum likelihood constrained topology based on Williams et al. (Citation2018) for istiophorid billfishes. Colours indicate different clades that are correlated with different types of rostra and behaviours based on extant species. The definition for the rostra shape is detailed in the character matrix (Supplemental material Table S2). The skull figures in ventral view show the morphology of the neurocranium, vomer and parasphenoid in different clades. The colours for skull bones are explained in Figure 13. The rostrum and drawings on the button show how each type of rostra correlated with a different feeding behaviours based on observations in extant species. The silhouettes of the three main types of rostra described are compared with drawings of different types of swords for better understanding of the rostral morphology. Green is extremely thin rostra similar to a fencing sword; blue illustrates robust rostrums compared with a ‘gladius hispaniensis’ or ‘Mainz-type swords’, as shown in Bishop (Citation2016, pp. 18–21); red shows slender rostrums compared with a Viking sword (see Oakeshott [Citation1960] for examples of this type of sword) and orange indicates the same rostrum type as red. Colours also show the convergence of different rostral, vomer and parasphenoid shapes in our topology. The Tetrapturus clade (orange) includes species with slender rostra similar to the Gracilorosta clade (red) but no information about the feeding behaviour in spearfishes is available.](/cms/asset/4358cf31-edc8-47bb-89be-949339a1dc23/tjsp_a_2091959_f0004_c.jpg)
The rostral shape variation between different istiophorid species is determined by the general rostrum outline, elongation and thickening of the prenasals and premaxillae () and the length of the ventral aperture (or the VSPM–L distance; ). The internal shape variation within different species is observed by studying the position and size of internal canals and in the area and shape occupied by the premaxillae and nasals in cross-section (, Supplemental material Fig. S2). Between the extant species, M. nigricans and Is. indica have rostra with more intraspecific variation while in Istiophorus and Kajikia spp. the shape is less variable (). The greater variability is associated to the ontogenic changes in the rostral shape of the blue and black marlins during growth (Collette & Graves Citation2019). The typical Makaira rostrum has thickened premaxillaries that cover more surface area of the rostrum than in any other taxon (). The thickened premaxillaries give the Makaira spp. rostra a robust and massive appearance that is similar to the outline of a gladius sword (‘gladius hispaniensis’ or ‘Mainz-type sword’ as shown in Bishop Citation2016, pp. 18–21) (e.g. ). The extant Istiompax, Istiophorus, Kajikia spp. and Tetrapturus ssp. and the extinct †Morgula gen. nov., †Prototetrapturus gen. nov. and †Spathochoira gen. nov. have reduced premaxillae and instead, the prenasals are covering more area giving them a more slender appearance, which is similar to the outline of a Viking sword (, ) (see Oakeshott Citation1960 for examples of sword shapes). In Istiophorus, the bill is extremely thin and is the longest observed (Citation1983, Citation1985), with a circular cross-section at 0.5 L and uniquely depressed cross-section at 0.25 L that extends to the distal tip whereby it resembles a fencing sword (, , ). Kajikia spp. have reduced premaxillae and the prenasals are embedded within the premaxillae up to c. 0.25 L, or even take up the entire rostrum length, which is unique to this taxon (). A similar pattern of cross-section is also observed in †M. belgica at 0.5 L (). The taxon †Morgula donosochagrense gen. et sp. nov. displays a distinctive rostrum with a very triangular outline (in dorsal view), nasals that angularly extend laterally and has the longest observed ventral aperture () that extends up to half of the rostral length (). †Prototetrapturus courcelli gen. et. comb. nov. has a long and slim rostrum with variable shapes in cross-section and relatively larger denticles as observed in the Austrian specimen NHMW 1986/109 (, Supplemental material Figs S4, S6). †Spathochoira calvertense gen. et. comb. nov. has a long ventral canal that extends up to 0.5 L (). These combinations of traits identified and described for †Morgula gen. nov., †Prototetrapturus gen. nov. and †Spathochoira gen. nov. (, ) are not present in any other extinct or extant species ().
The rostral traits that allow species-level differentiation among istiophorids are the length of the rostrum, the cross-section shapes at 0.5 L and 0.25 L regions (), the ventral aperture (), the shape of the distal tip (Supplemental material Fig. S1), presence and extension of the external ventral canal (), the distribution of denticles (Schultz Citation1987), lateral height of premaxillae (), contact of prenasals in the most anterior portion of the rostrum (, Supplemental material Fig. S5), lateral extension of nasals (), number of narial cavities and bones that enclose it (Supplemental material Fig. S5), and position, size and shape of the internal canals (, Supplemental material Fig. S2). PCA and density functions analyses () demonstrate that variables proposed in the ratios method express shape correctly and that complete or partial rostrums allow species-level identification if preserved parts include the 0.5 and 0.25 L regions (; see also Fierstine & Voigt Citation1996). Various authors (e.g. Barbolani di Montauto Citation1910; Berry Citation1917; Lériche Citation1926; Arambourg Citation1927; Schultz Citation1987; Fierstine & Voigt Citation1996; Fierstine Citation1998, Citation1999b, Citation2001) previously established the taxonomical importance of the istiophorid rostrum, but this structure was only rarely analysed in extant species (Nakamura Citation1983; Fierstine & Voigt Citation1996; Habegger et al. Citation2015, Citation2020). The reason why the rostrum in extant taxa has received less attention seems derived from the difficulty to identify and visualize such traits (Carnevale et al. Citation2002).
After having defined the traits related to rostral variation (), we identified new taxa, therefore increasing species richness of istiophorids to eight genera and about 15 species (; ). Of these, 10 species are extinct (†Mo. donosochagrense gen. et sp. nov., †Prototetrapturus courcelli gen. et. comb. nov., †Spathochoira calvertense gen et. comb. nov., †M. fierstini sp. nov., †M. colonense sp. nov., †M. belgica, †M. purdyi, †M. panamense, †M. teretirostris and †Istiophoridae sp. 1). The five other species are living in modern oceans (M. nigricans, Is. indica, I. platypterus, K. albida and Tetrapturus sp.), but their fossil record extends back to the late Pliocene (Supplemental material Table S5). The rest of the extant istiophorid species have no known fossil record at the moment. The specimen IRSNB P643, previously identified as ‘Istiophorus’ solidus, shows unambiguously that it is not a member of Istiophoridae, because it has three internal canals conversely to two, which is a persistent and reliable trait in istiophorids (). The specimen IRSNB P643 has a pair of canals positioned in a lateromedial position (), while the third canal is slightly smaller and placed centrally in a dorsal position (). Based on this feature, we conclude that specimen IRSNB P643 does not meet the definition of Istiophoridae, but its association with xiphiorhynchids is still unclear.
Phylogenetic relationships
The phylogenetic relationships between fossil and living istiophorids were reconstructed based on morphological traits, using maximum parsimony (Supplemental material Fig. S7) and maximum likelihood approaches (Supplemental material Fig. S8). Thirty-eight morphological characters were found to be parsimony-informative. Our MP analysis recovered 516 trees of 81 steps (CI = 0.7037, RI = 0.8481, RC = 0.5968, HI = 0.2962) that were summarized into a strict consensus (Supplemental material Fig. S7).
Both MP and ML analyses retrieved similar topologies in which Istiompax is sister to Makaira spp. (Supplemental material Figs S7, S8) and together form a clade sister to all other istiophorids (Supplemental material Figs S7, S8). These results contrast with recent phylogenetic analyses of extant istiophorids using mitochondrial and nuclear genes, in which Istiompax is sister to Kajikia (Supplemental material Fig. S7). The discrepancies observed between the morphology-based analyses (Supplemental material Figs S7, S8) and previously published phylogenies (Santini & Sorenson Citation2013; Williams et al. Citation2018) for extant istiophorids are caused by the high morphological similarities between Istiompax indica and Makaira spp., and between Istiophorus spp. and Kajikia ssp. (Nakamura Citation1983; Davie Citation1990). The morphological convergences observed among extant lineages of Istiophoridae indicates that the morphology by itself is insufficient to solve the systematic position of Istiompax and Makaira within the family (Supplemental material Figs S7, S8) (Nakamura Citation1983, Citation1985).
In order to overcome these problems, a topological constraint was enforced in subsequent MP and ML analyses (constrained MP and ML; , Supplemental material Fig. S9). The constraint was taken from the results of Williams et al. (Citation2018), based on phylogenetic analyses using the whole mitochondrial genome. This topology groups Istiophorus and Makaira into a clade, which is sister to Istiompax, Kajikia and Tetrapturus. The constrained MP analysis did not resolve all relationships between extant and fossil taxa (Supplemental material Fig. S9), but the constrained ML topology overcomes these problems by correcting the position of Istiophorus and Istiompax (). The strict consensus tree in the constrained MP summarizes 1851 trees of 107 steps, and places all fossil taxa as a polytomy basal to extant istiophorids (Supplemental material Fig. S9). We consider that the lack of resolution in the constrained MP analysis is related to convergences in cranial and vertebral features and the fragmentary nature of the fossil specimens (Supplemental material Fig. S9).
The constrained ML analysis recovered †Makaira teretirostris, †M. colonense, †M. panamense, †M. fierstini, †M. belgica and †M. purdyi nested within extant Makaira. The remaining fossil taxa described, †Morgula gen. nov. and †Spathochoira gen. nov., were recovered as sister group to †Prototetrapturus gen. nov. + Tetrapturus spp. (). In our results, fossil species are arranged among extant species in agreement with their cranial and vertebral morphologies and we recognize four main evolutionary lineages within Istiophoridae: (1) the Istiophorus clade, a lineage that includes the sailfish; (2) Machairostra, a new clade including all species more closely related to M. nigricans than I. platypterus or Kajikia spp.; (3) Gracilorostra, a new clade, which includes all species more closely related to Kajikia than to Makaira spp. or Tetrapturus spp. (); and (4) Tetrapturomorpha, a new clade including all species more closely related to Tetrapturus spp. than to Kajikia spp.
Members of Istiophorus are characterized by high dorsal and long pelvic fins, and very slender rostrum (Nakamura Citation1983, Citation1985). These features are present in stem billfishes of the families †Hemingwayidae, †Paleorhynchidae and †Blochidae (Fierstine & Monsch Citation2002; Fierstine Citation2006), and are also expressed in M. nigricans during ontogeny (Nakamura Citation1985). The presence of a high dorsal fin in stem billfish families and at one point during the ontogeny of extant M. nigricans suggests that the morphology of Istiophorus includes plesiomorphic traits (Nakamura Citation1983, Citation1985; Fierstine & Monsch Citation2002; Fierstine Citation2006). We therefore interpret Istiophorus as a lineage retaining plesiomorphic features, the origin of which dates back to the Paleocene as observed in extinct billfish families (Fierstine Citation2006).
Machairostra includes all extant and extinct species of Makaira and the clade is supported by morphological traits as robust rostrums with globular distal tip and premaxillaries that overcome the prenasal outline in dorsal view (). The fossil record of Makaira extends back to the middle Miocene of Anvers in Belgium, and the middle Miocene of southern California, with a probable occurrence during the early Miocene of Pisco in Peru (Lériche Citation1926; Fierstine & Applegate Citation1968; Louwye et al. Citation2010; di Celma et al. Citation2018; Bianucci et al. Citation2018; Parham et al. Citation2022). The branch lengths between living species in our topology reflect the little morphological differentiation and limited set of traits used to compare with fossil taxa (). We consider the relationships within Machairostra unsolved until more complete fossils and a larger set of characters are included in the phylogeny (). The relationships between fossil species of Makaira reflect patterns in morphological evolution. †Makaira fierstini sp. nov. and †M. belgica are similar in external appearance but differ significantly in that the internal canals are located dorsally in †M. belgica (). The rostral length of †M. fierstini sp. nov. is longer and its premaxillaries extend up to the half of the height in its anterior section (). The sister grouping of †M. colonense sp. nov. and †M. panamense is supported by having two narial cavities (Supplemental material Fig. S5). Both the species differ in the fused prenasals in †M. panamense (Supplemental material Fig. S5A), which are not fused in †M. colonense sp. nov. (). The narial cavity () in †M. panamense is enclosed only by the prenasals (Supplemental material Fig. S5A), while it is enclosed by dermothmoid + premaxillaries + prenasals bones in †M. colonense sp. nov. (Supplemental material Fig. S5B).
Gracilorostra is characterized by species with a slender rostrum (). The extinct taxa placed within this clade, namely †Morgula donosochagrense gen. nov., †Spathochoira calvertense gen. et. comb. nov. and †Prototetrapturus gen. nov., occur in the late Miocene, whereas the fossil record of the extant Kajikia extends back only into the early and late Pliocene () (Fierstine Citation2001). Our topology places the extant Kajikia spp. in a polytomy and as sister to the clade comprising extinct taxa (†Morgula gen. nov. + †Spathochoira gen. nov.) + (Tetrapturomorpha) (). Rostra with triangular outlines as in †Morgula gen. nov. and the slender rostrum of †Spathochoira gen. nov. (, , ) are predictors of a cranial shape similar to that of Kajikia (), and robust rostra predicts wide and squared craniums as observed in Istiompax or Makaira () (Gregory & Conrad Citation1937; Nakamura Citation1983; Davie Citation1990; Habegger et al. Citation2015). The placement of late Miocene taxa within Gracilorostra and Tetrapturomorpha is evidence that the divergence between Kajikia spp. and Tetrapturus spp. occurred earlier during the late Miocene, but the slender rostral morphology was established at least from the middle Miocene onwards, as the specimen from Austria shows (). The earliest fossil record of Tetrapturus comes from the Pliocene of Italy (Barbolani di Montauto Citation1910) and its sister relationship with †Prototetrapturus gen. nov. from the late Miocene of Algeria supports an origin of spearfishes in the Mediterranean Sea (Arambourg Citation1927; Carnevale et al. Citation2002; Gaudant Citation2008).
Figure 13. A–H, skull shape variation in fossil and extant istiophorids with emphasis in the basicranium; A, Istiompax indica; B, †Makaira panamense; C, Makaira nigricans; D, Istiophorus platypterus; E, Kajikia audax; F, Kajikia albida; G, Tetrapturus pfluegieri; H, Tetrapturus angustirostris. Colours shows the changes of shape in the vomer and parasphenoid. Wide vomer is associated with wide parasphenoid; obovate vomer is related to moderately wide vomer and narrow vomer is associated with narrow presphenoid. See Supplemental material Table S3 for the definition of these shapes.
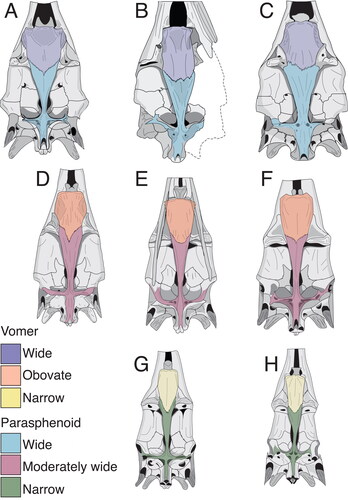
†Prototetrapturus gen. nov. is characterized by a highly modified rostrum with a slim distal tip and a cross-section outline that varies from obtuse to oval and pointed, and that is not comparable with that of any other species (−E). The phylogenetic relationships and the morphology show that †Prototetrapturus gen. nov. (–E) is unlikely to have wider cranial bones as observed in Makaira ssp., Istiompax indica or Kajikia ssp. (–C). Consequently, †Prototetrapturus courcelli gen. et. comb. nov. was a spearfish given its smaller size, which did not exceed the 200 cm in total body length (Nakamura Citation1983, Citation1985). Extant Tetrapturus spp. are monophyletic and their phylogenetic relationships are well supported (). If we consider the spearfish morphology, Tetrapturomorpha is supported by a rectangular cranium, a narrow (and rectangular) vomer, rectangular spines in the caudal vertebra and a reduced body size, representing synapomorphies (, , Supplemental material Figs. S7–S9). The genus Tetrapturus includes two short-billed species: T. belone and T. angustirostris with rostral lengths 14–20% of the total body length, showing a reduction trend of body size and rostrum length (Robins & de Sylva Citation1960, Citation1963; Nakamura Citation1983; Santini & Sorenson Citation2013). For example, fossil and extant species within Gracilorostra have body lengths between 200 and 300 cm, whereas species in Tetrapturomorpha do not exceed 200 cm (). However, the reduction in rostrum length is not exclusive to spearfishes, but evolved independently in †Makaira purdyi during the early Pliocene (, ).
Istiompax, which is sister to Gracilorostra, is characterized by having a massive (rather than slender) rostrum () with wide cranial bones (). However, the robust rostrum of Istiompax seems to be produced by its large size (). As Istiompax is placed in a polytomy we include it in neither Machairostra nor Gracilorostra until more morphological information of this taxon can be included in a phylogenetic analysis ().
Evolutionary implications of the rostrum
Rostral shape. Changes in rostral morphology that occurred throughout the evolution of istiophorid billfishes include variations in the rostral shape as seen in cross-section, changes in the length of the rostrum and the reduction of the premaxillae that resulted in an increase of the area covered by prenasals (, , ). In general, the morphological bauplan of the rostrum in istiophorids did not change much over time (), but specific modifications representing homoplasies and which include specific changes of individual bones resulted in significant rostral variations (, ). Extant istiophorids have the proportionally longest and open narial cavity (), while extinct species (such as †M. fierstini sp. nov., †M. belgica, †M. colonense sp. nov. and †M. panamense in the Machairostra clade) have smaller and more enclosed narial cavities (, , , Supplemental material Fig. S5). The Machairostra clade includes the late Miocene †M. colonense sp. nov., which is the species with the most massive and widest rostrum of the family (–C), which is formed by the thickening of the premaxillae, the maxillaries and the prenasals (, , ). Conversely, extant species in all clades display a reduction of premaxillaries and maxillaries with simultaneous elongation of the narial aperture if compared with theirs closed extinct relatives ().
Extinct species in the Machairostra clade have a shorter narial cavity and relatively smaller ventral cavity () than extant species (). However, this difference does not affect their large sizes (). Instead, these differences indicate that the accumulation of soft tissues (hyaline cartilage, adipose tissue and size of the oleofera gland) at the base of the rostrum increased, while the amount of bone progressively decreased throughout the evolutionary history of istiophorids (, ; Videler et al. Citation2016; Habegger et al. Citation2020). The reduction of the amount of bone in combination with the elongation of the narial aperture in M. nigricans and Is. indica highlights the evolution of a different strategy for maintaining high volumes in robust rostra of larger species (). We interpret that the reduction of the premaxillary thickness and the increase of tissue/hyaline cartilage accumulation at the rostrum base seen in M. nigricans and species assigned to the Gracilorostra clade build a lighter rostrum, enhance the ability to better absorb impacts and improves the ability to manoeuvre in multiple directions during prey capture in larger species () (see also Habegger et al. Citation2015; Videler et al. Citation2016).
The ventral cavity of the rostrum is filled by the accumulation of large amounts of adipose tissue created by the oleofera gland in extant istiophorids (Videler et al. Citation2016; Dhellemmes et al. Citation2020). The oleofera gland has the function of creating a hydrophobic layer in the head and rostrum, transporting fatty acids with healing function along the rostrum, enhancing the manoeuvrability in species with slender rostra and contributing to maintaining the internal temperature by its association with the heat producing organ (Carey Citation1982; Block Citation1986, Citation1990; Davie Citation1990; Domenici et al. Citation2014; Marras et al. Citation2015; Herbert-Read et al. Citation2016; Videler et al. Citation2016; Kurvers et al. Citation2017; Dhellemmes et al. Citation2020; Hansen et al. Citation2020). The oleofera gland is associated with capillaries that transport oil to the ‘oil excreting pores’ located in the skin of the forehead and the rostrum (Nakamura Citation1983; Videler et al. Citation2016; Dhellemmes et al. Citation2020). In living species the excreted oil produces a hydrophobic layer that reduces the streamwise friction drag and increases swimming efficiency (Videler et al. Citation2016). Our phylogeny placed extant species with larger area covered by these pores in the clade Gracilorostra and the pores should be analysed in extinct species to establish if the pattern is consistent () (Dhellemmes et al. Citation2020). Due to the importance of the oleofera gland and its multiple associated functions, additional studies of this organ and their associated structures in different extant species, including spearfishes, are necessary for providing a theoretical base to analyse the fossil record.
Rostral shape and skull association. Our phylogenetic hypothesis shows that the rostral shape is directly associated with the roof of the skull, the shapes of the vomer and parasphenoid (). Wide craniums, shield-like shaped vomers and wide and robust parasphenoids appear always combined with robust and thick bills (, ). This combination of traits is restricted to members of the Machairostra clade and Is. indica (, , ). Moderately wide craniums (as described in ) are linked with obovate vomers and moderately wide parasphenoids, and are associated to the extremely long and slender rostra in the Istiophorus clade and in members of the Gracilorostra clade (). The most extreme changes are expressed in members of the Tetrapturomorpha clade where species with different shapes of slender craniums are associated with narrow vomers, narrow parasphenoids and slender rostrums (; Gregory & Conrad Citation1937; Nakamura Citation1983). In other vertebrates such as cetaceans and ichthyosaurs, species with robust rostra are assumed to be feeding generalists, whereas species with slender rostra are more specialized () (Fischer et al. Citation2016; McCurry et al. Citation2017a, Citationb; McCurry & Pyenson Citation2019). Extant billfishes are also known to be generalists and opportunistic feeders (Collette & Graves Citation2019) and the morphologic changes in the cranial shape in different clades is associated with body size and feeding strategy ().
The phylogenetic association between the type of rostrum, skull shapes and body size () is explained with the prey size selection and the specific feeding behaviour of different species () (Talbot & Penrith Citation1962; Van der Elst & Roxbourg Citation1981; Nakamura Citation1983, Citation1985; Shimose et al. Citation2007; Domenici et al. Citation2014; Habegger et al. Citation2015, Citation2020; Marras et al. Citation2015; Fischer et al. Citation2016; Kurvers et al. Citation2017; Hansen et al. Citation2020). As a general pattern in fishes, variations in cranial and jaw shapes are related to the high diversity of feeding modes (Schwenk Citation2000; Westneat Citation2004; Schwenk & Rubega Citation2005; Gidmark et al. Citation2019) and the species-specific rostral elongation seen in istiophorids is not an exception to this rule (, ). In general, changes in rostrum, skull and jaw morphologies are linked to different feeding modes () (Habegger et al. Citation2015, Citation2017, Citation2020; McCurry & Pyenson Citation2019). Despite this pattern is expected, it provides a good base to understand the evolution and palaeobiology of istiophorids ().
Rostral shape and feeding behaviour. When different feeding strategies based on observed and inferred behaviours (, S6) are mapped on our phylogeny, a major pattern where rostrum, skull shape and body size can be explained by predatory behaviour emerges (). Our morphometric analysis demonstrates that the istiophorid rostrum is a species-specific structure () and thus supports the association between morphology and feeding strategy () (Habegger et al. Citation2015, Citation2020). In extant species, robust and stiff rostra () resist high loads in dorsoventral and lateral directions (Habegger et al. Citation2015). Stomach contents of the blue and black marlins show that this type of rostra is used to perform direct strong and fast lunges to strike and impale large prey items (Talbot & Penrith Citation1962; Van der Elst & Roxbourg Citation1981; Shimose et al. Citation2007; Domenici et al. Citation2014; Habegger et al. Citation2015, Citation2020; Marras et al. Citation2015; Fischer et al. Citation2016; Kurvers et al. Citation2017; Hansen et al. Citation2020). In fossil species of the Machairostra clade, the abrasion of the distal tip is identical in all directions when preserved, making possible to stablish relationships with the patterns observed in extant counterparts ().
Extant species with slender rostra () are always involved in complex predatory behaviours where direct strikes to prey items are rare (Nakamura Citation1983; Frazier et al. Citation1994; Júnior et al. Citation2004; Shimose et al. Citation2007; Domenici et al. Citation2014; Habegger et al. Citation2015, Citation2020; Marras et al. Citation2015; Hansen et al. Citation2020). In sailfishes, the lateral abrasions on the distal tip of the rostrum are produced by an active use during specialized lateral slashes that inflict serious damage to the prey (Domenici et al. Citation2014; Marras et al. Citation2015; Kurvers et al. Citation2017). In Kajikia spp., the rostrum is used for high-speed dashes, breaking schools apart and contacting prey incidentally, and tapping at isolated prey from diverse directions (Hansen et al. Citation2020). These predatory behaviours produce different abrasion patterns of the denticles at the distal tip of the rostrum (Domenici et al. 2015; Marras et al. Citation2015; Kurvers et al. 2016; Hansen et al. Citation2020) that is also observed their fossils relatives with slender rostra (Supplemental material Fig. S6). Additionally, our fossil evidence also shows that the lateralization is a specialization that evolved at least during the middle Miocene, as it is observed in a fossil specimen from Austria (Supplemental material Fig. S6).
Caudal skeleton and gigantism
Time-calibrated molecular phylogenies of istiophorid billfishes show that the large size and weight in istiophorids is a product of convergent increases in two different lineages followed by a dramatic decrease in the spearfish clade (Santini & Sorenson Citation2013). Independent of whether the phylogeny is constrained or not, our results show that this evolutionary pattern is consistent when the body size, morphology and extinct species are analysed together (, Supplemental material Table S2; ). Our results show that different clades include species with delimited size ranges (). The larger body sizes are linked with square and massive craniums, the increase of one caudal vertebra, the presence of the lateral apophysis and larger spines in the vertebral column () (Nakamura Citation1983, Citation1985; Davie Citation1990). With the exception of the black marlin, all larger species are nested in the Machairostra clade, in which maximum adult body size ranges from 300 up to 400 cm () (Nakamura Citation1983, Citation1985; Davie Citation1990). Both the Istiophorus and Gracilorostra clades are restricted to species with maximum body sizes ranging from 200 up to 300 cm (; ) (Nakamura Citation1983, Citation1985). The most extreme size reduction is observed in the Tetrapturomorpha clade, which includes species with body sizes not larger than 200 cm (Nakamura Citation1983, Citation1985).
Our topology shows that the vertebral formula 12 + 12 and the lack of the lateral apophyses evolved convergently as depicted in the Istiophorus and the Gracilorsotrae + Tetrapturomiorpha clades (). The lack of the lateral apophysis in members of the Gracilorostra clade is accompanied with a gradual body size reduction that started during the late Miocene with †Prototetrapturus courcelli comb. nov. (; ) (Nakamura Citation1983, Citation1985; Davie Citation1990). The 13 caudal vertebrae in Machairostra together with the lateral apophysis and the larger square spines are innovations that contribute to producing larger body sizes in istiophorids (). (Fierstine & Walters Citation1968; Davie Citation1990; Hebrank et al. Citation1990). For example, the lateral apophysis connects the posterior oblique tendons that attach the epaxial and hypaxial musculature (Davie Citation1990; Hebrank et al. Citation1990) and the plate-like spines provide extra skeletal attachment areas for additional muscle fibres (Fierstine & Walters Citation1968; Davie Citation1990; Hebrank et al. Citation1990). The muscles attached to these structures are involved in propulsion of the caudal fin and constitute about the 57–65% of the total body weight in larger extant species (Davie Citation1990). The gradual body size reduction in istiophorids is an evolutionary pattern associated with the lack of lateral apophyses but also with the reduction of the neural spine area and size (; ) (Nakamura Citation1983, Citation1985; Davie Citation1990).
The current evidence suggests that diversification of istiophorids started during the middle to late Miocene, with most phylogenetic splits occurring since the Pliocene (c. 5 Ma). However, the phylogenetic evidence presented here demonstrates that the late Miocene includes a diverse fauna of istiophorid billfishes (). Nevertheless, our current evidence does not allow us to understand the evolutionary and possible environmental factors involved in the diversification of billfishes, but giant marlins over 400 cm exist at least since the late Miocene alongside smaller sized taxa () (Fierstine Citation2006). The giant marlins show that the inclusion of one additional precaudal vertebra to the caudal region and the presence of the lateral apophyses are important evolutionary innovations occurring in larger species (Nakamura Citation1983, Citation1985; Davie Citation1990). Our hypothesis based on morphological traits shows that homoplasies in body size, rostral morphology, skull shape and caudal vertebral count are crucial to understand the evolution of billfishes () (Sanderson & Hufford Citation1996). The giant size seen in various species evolved independently during the Miocene and again in the Pliocene with the black marlin () (Santini & Sorenson Citation2013; Williams et al. Citation2018). Thus, smaller sized members with slender rostra appear to represent the plesiomorphic condition within billfishes (Fierstine & Monsch Citation2002). However, analyses of early Miocene billfishes may contribute to our knowledge and could explain the scenario in which these patterns evolved.
Conclusions
We present here the first phylogenetic analysis of fossil and extant istiophorids based on morphological characters. According to our results, the rostral morphology in istiophorids is highly variable and it represents a species-specific structure. Our work shows that the family Istiophoridae is composed of 20 species of which 10 are extinct and the remaining species are inhabitants of modern oceans. In general, extinct istiophorids have rostra with massive bones, while extant species show a reduction of several skeletal elements, which is compensated by an increase of adipose tissue and hyaline cartilage.
The istiophorid rostrum is a structure generally assumed to perform various functions such as improving their hydrodynamic features, defence and prey capture. However, our results suggest that the main rostral function is predominantly associated with feeding.
Fossil and extant species are arranged in four well-defined clades, where any clade includes specific fossil or extant species. The phylogenetic relationships display association patterns between rostral and cranial morphologies as well as number and shape of caudal vertebrae. The state changes in these morphological traits are linked to differences in the maximum body size. A progressive size reduction occurred throughout different clades as expressed in Gracilorostra and Tetrapturomorpha. Our work shows that species richness and complexity of istiophorids was larger than previously assumed and that most of the observed traits in living species have a long evolutionary history. Despite all our advances presented here, several questions about the evolutionary history of istiophorids remain unsolved. For instance, we still lack a complete understanding of the underlying processes that drove the morphological evolution in istiophorids and whether they evolved rapidly or progressively. Additional early and middle Miocene fossils are necessary in order to solve these and other open questions, and also to establish the origin of istiophorids.
Associate Editor: Martin Brazeau
Supplemental Material 3
Download (2.4 KB)Supplemental Material 2
Download R Objects File (7.2 KB)Supplemental Material 1
Download Comma-Separated Values File (18.4 KB)Supplemental Material - Tables S1 and S2
Download MS Word (22.4 KB)Supplemental Material - Figs. S1-S6
Download MS Word (9.5 MB)Acknowledgements
This project was made possible by various grants to CD: SENACYT APY-NI10-016A grant (National Secretary of Science and Technology of Panama), Ricardo Perez S.A, Smithsonian Tropical Research Institute – Tupper Paleontological Fund, International Travel Grant for Vertebrate Palaeontology of the University of Florida, Travel Program of the Charles University in Prague and the Doctoral and Postdoctoral Scholarships Program from SENACYT BIP-2018-004. TP is grateful for financial support by the Institute of Geology of the Czech Academy of Sciences (RVO67985831). We thank the Dirección de Recursos Minerales of Panama for collecting permits. Thanks also go to Jeffrey Clayton, Jeffrey Williams and Michael Brett-Surman (from the Smithsonian National Museum of Natural History, Washington, DC, USA) for help with osteological and palaeontological collections. Thanks to Phillipe Béarez from the Muséum national d’Histoire naturelle of France and Richard Cooke from STRI for his help with osteological material of I. platypterus. Also to Annelise Folie (Royal Belgian Institute of Natural Sciences, Brussel, Belgium) for access to collections; to Gustavo Ballen and Irvy Quitmyer for their support by providing the specimens FLMNH-EA 210017 and 208089 from collections of the Florida Museum of Natural History, Gainesville, USA for this study; to the CTPA – STRI palaeontological crew (STRI – Panama) and Abraham Osorio for their support during fieldwork. And to Jorge Aleman (STRI – Panama) for preparing photographs of all new described specimens and Angel Aguirre (STRI – Panama) for his valuable help by obtaining rare literature. We extend our gratitude to W. Gelnaw (OC Parks) for providing photos of OCPC 31001. Special thanks go to Félix Rodriguez (STRI, Panama) for his support during the early stages of this project, to Eduardo Villalobos for discussions and to the reviewers who improved our manuscript with their comments and suggestions. We dedicate this work to the late Harry Fierstine (1932–2021), who generously shared unpublished data as well his experience and knowledge about istiophorid morphology during the earliest years of the academic formation of CD, which made this study possible. He will be missed.
Supplemental material
Supplemental material for this article can be accessed here: https://doi.org/10.1080/14772019.2022.2091959.
References
- Arambourg, C. 1927. Les poissons fossiles d’Oran. Matériaux pour la Carte géologiques de l’Algérie, Série 1, Paléontologie, 6, 1–298.
- Barbolani di Montauto, G. 1910. L’Histiophorus herschelii (Gray) nel terziario superiore. Palaeontographia Italica, 16, 1–22.
- Bernard, A. M., Shivji, M. S., Domingues, R. R., Hazin, F. H. V., de Amorim, A. F., Domingo, A., Arocha, F., Prince, E. D., Hoolihan, J. P. & Hilsdorf, A. W. S. 2013. Broad geographic distribution of roundscale spearfish (Tetrapturus georgii) (Teleostei, Istiophoridae) in the Atlantic revealed by DNA analysis: implications for white marlin and roundscale spearfish management. Fisheries Research, 139, 93–97. doi:10.1016/j.fishres.2012.10.009
- Berry, E. W. 1917. A sail fish from the Virginia Miocene. American Journal of Science, 43, 461–464.
- Betancur-R., R., Broughton, R. E., Wiley, E. O., Carpenter, K., López, J. A., Li, C., Holcroft, N. I., Arcila, D., Sanciangco, M., Cureton II, J. C., Zhang, F., Buser, T., Campbell, M. A., Ballesteros, J. A., Roa-Varon, A., Willis, S., Borden, W. C., Rowley, T., Reneau, P. C., Hough, D. J., Lu, G., Grande, T., Arratia, G. & Ortí, G. 2013. The tree of life and a new classification of bony fishes. PLoS Currents, 5. doi:10.1371/currents.tol.53ba26640df0ccaee75bb165c8c26288
- Bianucci, G., Carone, G., Domning, D. P., Landini, W., Rook, L. & Sorbi, S. 2008. Peri-Messinian dwarfing in Mediterranean Metaxytherium (Mammalia: Sirenia): evidence of habitat degradation related to the Messinian salinity crisis. Garyounis Scientific Bulletin, 5, 145–157.
- Bianucci, G., Collareta, A., Bosio, G., Landini, W., Gariboldi, K., Gioncada, A., Lambert, O., Malinverno, E., de Muizon, C., Varas-Malca, R., Villa, I. M., Coletti, G., Urbina, M. & di Celma, C. 2018. Taphonomy and palaeoecology of the lower Miocene marine vertebrate assemblage of Ullujaya (Chilcatay Formation, East Pisco Basin, southern Peru). Palaeogeography, Palaeoclimatology, Palaeoecology, 511, 256–279. doi:10.1016/j.palaeo.2018.08.013
- Bishop, M. C. 2016. The gladius: the roman short sword. Osprey Publishing Ltd, Oxford, 82 pp.
- Block, B. A. 1986. Structure of the brain and eye heater tissue in marlins, sailfish, and spearfishes. Journal of Morphology, 190, 169–189. doi:10.1002/jmor.1051900203
- Block, B. A. 1990. Physiology and ecology of brain and eye heaters in billfishes. Pp. 123–136 in R. H. Stroud (ed.) Proceedings of the 2nd international billfish symposium. Kailuakona, Hawaii.
- Carey, F. G. 1982. A brain heater in the swordfish. Science, 216, 1327–1329. doi:10.1126/science.7079766
- Carnevale, G., Sorbini, C., Landini, W. & Varola, A. 2002. Makaira cf. M. nigricans Lacepede, 1802 (Teleostei: Perciformes: Istiophoridae) from the Pietra Leccese, late Miocene, Apulia, southern Italy. Palaeontographia Italica, 88, 63–75.
- Carrillo-Briceño, J. D., De Gracia, C., Pimiento, C., Aguilera, O. A., Kindlimann, R., Santamarina, P. & Jaramillo, C. 2015. A new late Miocene chondrichthyan assemblage from the Chagres Formation, Panama. Journal of South American Earth Sciences, 60, 56–70. doi:10.1016/j.jsames.2015.02.001
- Coates, A. G. 1999. Lithostratigraphy of the Neogene strata of the Caribbean coast from Limon, Costa Rica, to Colon, Panama. Bulletins of American Paleontology, 113, 17–37.
- Collette, B. & Graves, J. 2019. Tunas and billfishes of the world. Johns Hopkins University Press, Baltimore, Maryland, 352 pp.
- Collette, B. B., McDowell, J. R. & Graves, J. E. 2006. Phylogeny of recent billfishes (Xiphioidei). Bulletin of Marine Science, 79, 455–468.
- Collins, L. S., Coates, A. G., Berggren, W. A., Aubry, M.-P. & Zhang, J. 1996. The late Miocene Panama isthmian strait. Geology, 24, 687–690.
- Davie, P. S. 1990. Pacific marlins: anatomy and physiology. Department of Physiology and Anatomy, Massey University, Palmerston North, New Zealand, 88 pp.
- De Gracia, C., O’Dea, A., Rodríguez, F. & D’Croz, L. 2012. Respuesta ambiental en el Pacífico frente a la subducción de la dorsal asísmica de Cocos (Panamá y Costa Rica). Revista de Biología Tropical, 60, 893–908. doi:10.15517/rbt.v60i2.4025
- Dhellemmes, F., Hansen, M. J., Bouet, S. D., Videler, J. J., Domenici, P., Steffensen, J. F., Hildebrandt, T., Fritsch, G., Bach, P. & Sabarros, P. S. 2020. Oil gland and oil pores in billfishes: in search of a function. Journal of Experimental Biology, 223, 224956. doi:10.1242/jeb.224956
- di Celma, C., Malinverno, E., Collareta, A., Bosio, G., Gariboldi, K., Lambert, O., Landini, W., Pierantoni, P. P., Gioncada, A. & Villa, I. M. 2018. Facies analysis, stratigraphy and marine vertebrate assemblage of the lower Miocene Chilcatay Formation at Ullujaya (Pisco basin, Peru). Journal of Maps, 14, 257–268. doi:10.1080/17445647.2018.1456490
- Domenici, P., Wilson, A. D. M., Kurvers, R. H. J. M., Marras, S., Herbert-Read, J. E., Steffensen, J. F., Krause, S., Viblanc, P. E., Couillaud, P. & Krause, J. 2014. How sailfish use their bills to capture schooling prey. Proceedings of the Royal Society B, 281, 20140444. doi:10.1098/rspb.2014.0444
- Dray, S. & Josse, J. 2015. Principal component analysis with missing values: a comparative survey of methods. Plant Ecology, 216, 657–667.
- Fierstine, H. L. 1978. A new marlin, Makaira panamensis, from the late Miocene of Panama. Copeia, 1978, 1–11. doi:10.2307/1443812
- Fierstine, H. L. 1990. A paleontological review of three billfish families (Istiophoridae, Xiphiidae, and Xiphiorhynchidae). Pp. 11–19 in S. Richard (ed.) Proceedings of the second international billfish symposium. Kailua-Kona, Hawaii.
- Fierstine, H. L. 1998. Makaira sp., cf. M. nigricans Lacépède, 1802 (Teleostei: Perciformes: Istiophoridae) from the Eastover Formation, late Miocene, Virginia, and a reexamination of †Istiophorus calvertensis Berry, 1917. Journal of Vertebrate Paleontology, 18, 30–42. doi:10.1080/02724634.1998.10011032
- Fierstine, H. L. 1999a. Makaira sp., cf. M. nigricans Lacépède, 1802 (Teleostei: Perciformes: Istiophoridae) from the late Miocene, Panama, and its probable use of the Panama seaway. Journal of Vertebrate Paleontology, 19, 430–437. doi:10.1080/02724634.1999.10011156
- Fierstine, H. L. 1999b. A new shortbilled marlin of the genus Makaira, from the Yorktown Formation, (early Pliocene), Eastern North Carolina at Lee Creek Mine, USA. Tertiary Research, 19, 71–77.
- Fierstine, H. L. 2001. Analysis and new records of billfish (Teleostei: Perciformes: Istiophoridae) from the Yorktown Formation, early Pliocene of eastern North Carolina at Lee Creek Mine. Smithsonian Contributions to Paleobiology, 90, 21–69.
- Fierstine, H. L. 2006. Fossil history of billfishes (Xiphioidei). Bulletin of Marine Science, 79, 433–453.
- Fierstine, H. L. & Applegate, S. P. 1968. Billfish remains from southern California with remarks on the importance of the predentary bone. Bulletin of the Southern California Academy of Sciences, 67, 29–39.
- Fierstine, H. L. & Walters, V. 1968. Studies in locomotion and anatomy of scombroid fishes. Memoirs of the Southern California Academy of Sciences, 6, 1–31.
- Fierstine, H. L. & Voigt, N. L. 1996. Use of rostral characters for identifying adult billfishes (Teleostei: Perciformes: Istiophoridae and Xiphiidae). Copeia, 1996, 148–161. doi:10.2307/1446950
- Fierstine, H. L. & Monsch, K. A. 2002. Redescription and phylogenetic relationships of the family Blochiidae (Perciformes: Scombroidei), middle Eocene, Monte Bolca, Italy. Studi e Ricerche sui Giacimenti Terziari di Bolca, Museo Civico di Storia Naturale di Verona, 9, 121–163.
- Fischer, V., Bardet, N., Benson, R. B. J., Arkhangelsky, M. S. & Friedman, M. 2016. Extinction of fish-shaped marine reptiles associated with reduced evolutionary rates and global environmental volatility. Nature Communications, 7, 10825. doi:10.1038/ncomms10825
- Frazier, J. G., Fierstine, H. L., Beavers, S. C., Achaval, F., Suganuma, H., Pitman, R. L., Yamaguchi, Y. & Prigioni, C. M. 1994. Impalement of marine turtles (Reptilia, Chelonia: Cheloniidae and Dermochelyidae) by billfishes (Osteichthyes, Perciformes: Istiophoridae and Xiphiidae). Environmental Biology of Fishes, 39, 85–96.
- Gaudant, J. 2008. Paléobiodiversité et paléoenvironnements: l’exemple des gisements de poissons téléostéens du Messinien préévaporitique d’Oran et du bassin du Chélif (Algérie). Geodiversitas, 30, 141–163.
- Gidmark, N. J., Pos, K., Matheson, B., Ponce, E. & Westneat, M. W. 2019. Functional morphology and biomechanics of feeding in fishes. Pp. 297–332 in V. Bels & I. Whishaw (eds) Feeding in vertebrates. Fascinating life sciences. Springer, Cham, Switzerland. doi:10.1007/978-3-030-13739-7_9
- Graves, J. E. & McDowell, J. R. 1995. Inter-ocean genetic divergence of istiophorid billfishes. Marine Biology, 122, 193–203.
- Graves, J. E. & McDowell, J. R. 2012. Inter-annual variability in the proportion of roundscale spearfish (Tetrapturus georgii) and white marlin (Kajikia albida) in the western North Atlantic Ocean. Collective Volumes of Scientific Papers ICCAT, 68, 1543–1547.
- Graves, J. E. & McDowell, J. R. 2015. Population structure of istiophorid billfishes. Fisheries Research, 166, 21–28. doi:10.1016/j.fishres.2014.08.016
- Greenwood, P. H., Rosen, D. E., Weitzman, S. H. & Myers, G. S. 1966. Phyletic studies of teleostean fishes, with a provisional classification of living forms. Bulletin of American Museum of Natural History, 131, 341–345.
- Gregory, W. K. 1933. Fish skulls: a study of the evolution of natural mechanisms. Transactions of the American Philosophical Society, 23, i–vii + 75–481. doi:10.2307/3231917
- Gregory, W. K. & Conrad, G. M. 1937. The comparative osteology of the swordfish (Xiphias) and the sailfish (Istiophorus). American Museum Novitates, 952, 1–25.
- Habegger, M. L., Dean, M. N., Dunlop, J. W. C., Mullins, G., Stokes, M., Huber, D. R., Winters, D. & Motta, P. J. 2015. Feeding in billfishes: inferring the role of the rostrum from a biomechanical standpoint. Journal of Experimental Biology, 218, 824–836. doi:10.1242/jeb.106146
- Habegger, M. L., Huber, D. H., Lajeunesse, M. J. & Motta, P. J. 2017. Theoretical calculations of bite force in billfishes. Journal of Zoology, 303, 15–26. doi:10.1111/jzo.12465
- Habegger, L., Motta, P., Huber, D., Pulaski, D., Grosse, I. & Dumont, E. 2020. Feeding biomechanics in billfishes: investigating the role of the rostrum through finite element analysis. The Anatomical Record, 303, 44–52. doi:10.1002/ar.24059
- Hanner, R., Floyd, R., Bernard, A., Collette, B. B. & Shivji, M. S. 2011. DNA barcoding of billfishes. Mitochondrial DNA, 22, 27–36.
- Hansen, M. J., Krause, S., Breuker, M., Kurvers, R. H. J. M., Dhellemmes, F., Viblanc, P. E., Müller, J., Mahlow, C., Boswell, K., Marras, S., Domenici, P., Wilson, A. D. M., Herbert-Read, J. E., Steffensen, J. F., Fritsch, G., Hildebrandt, T. B., Zaslansky, P., Bach, P., Sabarros, P. S. & Krause, J. 2020. Linking hunting weaponry to attack strategies in sailfish and striped marlin. Proceedings of the Royal Society B, 287, 20192228. doi:10.1098/rspb.2019.2228
- Hebrank, J. H., Hebrank, M. R., Long, J. H. Jr, Block, B. & Wainwright, S. A. 1990. Backbone mechanics of the blue marlin Makaira nigricans (Pisces, Istiophoridae). Journal of Experimental Biology, 148, 449–459.
- Hendy, A. J. W. 2013. Spatial and stratigraphic variation of marine paleoenvironments in the middle–upper Miocene Gatun Formation, Isthmus of Panama. Palaios, 28, 210–227.
- Herbert-Read, J. E., Romanczuk, P., Krause, S., Strömbom, D., Couillaud, P., Domenici, P., Kurvers, R. H. J. M., Marras, S., Steffensen, J. F. & Wilson, A. D. M. 2016. Proto-cooperation: group hunting sailfish improve hunting success by alternating attacks on grouping prey. Proceedings of the Royal Society B, 283, 20161671.
- Hoolihan, J. P., Premanandh, J., D’Aloia-Palmieri, M.-A. & Benzie, J. A. H. 2004. Intraspecific phylogeographic isolation of Arabian Gulf sailfish Istiophorus platypterus inferred from mitochondrial DNA. Marine Biology, 145, 465–475. doi:10.1007/s00227-004-1346-2
- Johnson, D. G. & Patterson, C. 1993. Percomorph phylogeny: a survey of acanthomorphs and a new proposal. Bulletin of Marine Science, 52, 554–626.
- Jollie, M. 1986. A primer of bone names for the understanding of the actinopterygian head and pectoral girdle skeletons. Canadian Journal of Zoology, 64, 365–379.
- Josse, J., Pagès, J. & Husson, F. 2011. Multiple imputation in principal component analysis. Advances in Data Analysis and Classification, 5, 231–246.
- Josse, J. & Husson, F. 2012. Handling missing values in exploratory multivariate data analysis methods. Journal de la Société Française de Statistique, 153, 79–99.
- Josse, J. & Husson, F. 2016. missMDA: a package for handling missing values in multivariate data analysis. Journal of Statistical Software, 70, 1–31.
- Júnior, T. V., Maria Vooren, C. & Paula Lessa, R. 2004. Feeding habits of four species of Istiophoridae (Pisces: Perciformes) from northeastern Brazil. Environmental Biology of Fishes, 70, 293–304. doi:10.1023/B:EBFI.0000033345.53182.b9
- Kurvers, R. H. J. M., Krause, S., Viblanc, P. E., Herbert-Read, J. E., Zaslansky, P., Domenici, P., Marras, S., Steffensen, J. F., Svendsen, M. B. S., Wilson, A. D. M., Couillaud, P., Boswell, K. M. & Krause, J. 2017. The evolution of lateralization in group hunting sailfish. Current Biology, 27, 521–526. doi:10.1016/j.cub.2016.12.044
- Lacépède, B. G. E. 1802. Histoire Naturelle des Poissons. Volume 4. Chez Plassan, Paris, xliv + 728pp.
- Landini, W. & Menesini, E. 1984. Messinian marine fish communities of the Mediterranean Sea. Atti della Società Toscana di Scienze Naturali, Serie A, 91, 279–290.
- Lériche, M. 1926. Les Poissons Néogènes de la Belgique. Bulletin de la Société Belge de Géologie, de Paléontologie et d’Hydrologie, Procés-Verbaux, 32, 412.
- Lewis, P. O. 2001. A likelihood approach to estimating phylogeny from discrete morphological character data. Systematic Biology, 50, 913—925. doi:10.1080/106351501753462876
- Little, A. G., Lougheed, S. C. & Moyes, C. D. 2010. Evolutionary affinity of billfishes (Xiphiidae and Istiophoridae) and flatfishes (Plueronectiformes): independent and trans-subordinal origins of endothermy in teleost fishes. Molecular Phylogenetics and Evolution, 56, 897–904. doi:10.1016/j.ympev.2010.04.022
- Louwye, S., Marquet, R., Bosselaers, M. & Lambert, O. 2010. Stratigraphy of an early–middle Miocene sequence near Antwerp in northern Belgium (southern North Sea basin). Geologica Belgica, 13, 269–284.
- Maddison, W. P. 2017. Mesquite: a modular system for evolutionary analysis. Version 2.0. Accessed at: http://mesquiteproject.org, accessed 12 September 2021.
- Marras, S., Noda, T., Steffensen, J. F., Svendsen, M. B. S., Krause, J., Wilson, A. D. M., Kurvers, R. H. J. M., Herbert-Read, J., Boswell, K. M. & Domenici, P. 2015. Not so fast: swimming behavior of sailfish during predator–prey interactions using high-speed video and accelerometry. Integrative and Comparative Biology, 55, 719–727. doi:10.1093/icb/icv017
- McCurry, M. R., Fitzgerald, E. M. G., Evans, A. R., Adams, J. W. & McHenry, C. R. 2017a. Skull shape reflects prey size niche in toothed whales. Biological Journal of the Linnean Society, 121, 936–946. doi:10.1093/biolinnean/blx032
- McCurry, M. R., Walmsley, C. W., Fitzgerald, E. M. G. & McHenry, C. R. 2017b. The biomechanical consequences of longirostry in crocodilians and odontocetes. Journal of Biomechanics, 56, 61–70. doi:10.1016/j.jbiomech.2017.03.003
- McCurry, M. R. & Pyenson, N. D. 2019. Hyper-longirostry and kinematic disparity in extinct toothed whales. Paleobiology, 45, 21–29. doi:10.1017/pab.2018.33
- Morrow, J. E. & Posner, G. S. 1957. On the morphology of the pectoral girdle in the genus Makaira. Bulletin of the Bingham Oceanographic Collection, Peabody Museum of Natural History, Yale University, 26, 88–105.
- Müller, J. 1845. Über den Bau und die Grenzen der Ganoiden und über das natürliche System der Fische. Physikalische und Mathematische Abhandlungen der Königlichen Academie der Wissenschaften in Berlin, 1, 117–216.
- Nakamura, H. 1955. Report of an investigation of the spearfishes of Formosan waters. Special Scientific Report Fisheries No. 153. US Department of the Interior, Fish and Wildlife Service, Washington, 46 pp.
- Nakamura, I. 1983. Systematics of the billfishes (Xiphiidae and Istiophoridae). Seto Marine Biological Laboratory, Kyoto, 396 pp.
- Nakamura, I. 1985. FAO species catalogue. Billfishes of the world. An annotated and illustrated catalogue of marlins, sailfishes, spearfishes and swordfishes known to date. FAO Fish Biological Synopses 125, Volume 5. University of Kyoto Mauzuru, Kyoto 65 pp.
- Nelson, J. S., Grande, T. C. & Wilson, M. V. H. 2016. Fishes of the world. John Wiley & Sons, Hoboken, New Jersey, 752 pp.
- Nguyen, L.-T., Schmidt, H. A., von Haeseler, A. & Minh, B. Q. 2015. IQ-TREE: a fast and effective stochastic algorithm for estimating maximum-likelihood phylogenies. Molecular Biology and Evolution, 32, 268–274. doi:10.1093/molbev/msu300
- Oakeshott, R. E. 1960. The archaeology of weapons: arms and armour from prehistory to the age of chivalry. The Boydell Press, Rochester, NY, 400 pp.
- Parham, J. F., Barron, J. A. & Velez-Juarbe, J. 2022. Middle and late Miocene marine mammal assemblages from the Monterey Formation of Orange County, California. In I. Aiello, J. Barron & C. Ravelo (eds) Understanding the Monterey Formation and similar biosiliceous units across space and time. Geological Society of America Special Paper, 556. doi:10.1130/2021/2556(10)
- Pepperell, J. G. 2010. Fishes of the open ocean: a natural history & illustrated guide. University of Chicago Press, Chicago, 266 pp.
- Prager, M. H., Prince, E. D. & Lee, D. W. 1995. Empirical length and weight conversion equations for blue marlin, white marlin, and sailfish from the North Atlantic Ocean. Bulletin of Marine Science, 56, 201–210.
- Pyenson, N. D., Vélez-Juarbe, J., Gutstein, C. S., Little, H., Vigil, D. & O’Dea, A. 2015. Isthminia panamensis, a new fossil inioid (Mammalia, Cetacea) from the Chagres Formation of Panama and the evolution of ‘river dolphins’ in the Americas. PeerJ, 3, e1227. doi:10.7717/peerj.1227
- R Core Team. 2013. R: A language and environment for statistical computing. Accessed at: http:/www.R-project.org/, accessed 7 May 2021.
- Rafinesque, C. S. 1810. Caratteri di alcuni nuovi generi e nuove specie di animali e piante della sicilia, con varie osservazioni sopra i medisimi. Per le stampe di Sanfilippo, Palermo, Italy, 105 pp.
- Regan, C. T. 1923. The skeleton of Lepidosteus, with remarks on the origin and evolution of the lower Neopterygian fishes. Proceedings of the Zoological Society of London, 93, 445–461.
- Robins, C. R. & de Sylva, D. P. 1960. Description and relationships of the longbill spearfish, Tetrapturus belone, based on western North Atlantic specimens. Bulletin of Marine Science, 10, 383–413.
- Robins, C. R. & de Sylva, D. P. 1963. A new western Atlantic spearfish, Tetrapturus pfluegeri, with a redescription of the Mediterranean spearfish Tetrapturus belone. Bulletin of Marine Science, 13, 84–122.
- Rojo, A. L. 2017. Dictionary of evolutionary fish osteology. CRC Press, Boca Raton, Florida, 282 pp. doi:10.4324/9781315150932
- Roman, F. 1922. La faune de vertèbres des sables de Montpellier I. Les Baleinoptères. Travaux du laboratoire de géologie de la Faculté des Sciences de Lyon, 2, 5–40.
- Rosen, D. E. 1973. Interrelationships of higher Euteleostean fishes. Pp. 397–513 in P. H. Greenwood, R. S. Miles & C. Patterson (eds) Interrelationships of fishes. Academic Press, London.
- Rubin, D. B. 1976. Inference and missing data. Biometrika, 63, 581–592.
- Rütimeyer, L. 1857. Über Echeiziphis, ein neues Cetaceen-Genus. Verhandlungen der Naturforschenden Gesellschaft, 1, 555–567.
- Sanderson, M. J. & Hufford, L. 1996. Homoplasy: the recurrence of similarity in evolution. Academic Press, San Diego, 339 pp.
- Santini, F. & Sorenson, L. 2013. First molecular timetree of billfishes (Istiophoriformes: Acanthomorpha) shows a Late Miocene radiation of marlins and allies. Italian Journal of Zoology, 80, 481–489. doi:10.1080/11250003.2013.848945
- Schultz, O. 1987. Taxonomische Neugruppierung der Überfamile Xiphioidea (Pisces, Osteichthyes. Annalen des Naturhistorischen Museums in Wien, Serie A, 89, 95–202.
- Schultz, O. 1998. Die Knorpel-und Knochenfischfauna (excl. Otolithen) aus dem Karpat des Korneuburger Beckens (Niederösterreich). Beiträge zur Paläontologie, 23, 295–323.
- Schultz, O. 2013. Catalogus Fossilium Austriae Band 3: Pisces: Ein systematisches Verzeichnis aller auf österreichischem Gebiet festgestellten Fossilien (W. E. Piller, Ed.; 1st ed.). Austrian Academy of Sciences Press, Wien. doi:10.2307/j.ctt1vw0qw3
- Schwarzhans, W. & Aguilera, O. 2013. Otoliths of the Myctophidae from the Neogene of tropical America. Palaeo Ichthyologica, 13, 83–150.
- Schwenk, K. 2000. Tetrapod feeding in the context of vertebrate morphology. Pp. 3–20 in K. Schwenk (ed.) Feeding: form, function and evolution in tetrapod vertebrates. Academic Press, San Diego, CA.
- Schwenk, K. & Rubega, M. 2005. Diversity of vertebrate feeding systems. Pp. 1–41 in J. M. Strack & T. Wang (eds) Physiological and ecological adaptations to feeding in vertebrates. Science Publishers, Enfield, UK.
- Shimose, T., Yokawa, K., Saito, H. & Tachihara, K. 2007. Evidence for use of the bill by blue marlin, Makaira nigricans, during feeding. Ichthyological Research, 54, 420–422.
- Silverman, B. W. 1986. Density estimation for statistics and data analysis. Chapman and Hall/CRC Press, London/New York, 176 pp.
- Snyder, S. W., Mauger, L. L. & Akers, W. H. 1983. Planktonic foraminifera and biostratigraphy of the Yorktown Formation, Lee Creek Mine. Smithsonian Contributions to Paleobiology, 53, 455–482.
- Swofford, D. L. & Sullivan, J. 2003. Phylogeny inference based on parsimony and other methods using PAUP*. Pp. 267–312 in M. Salemmi & A. M. Vandame (eds) The phylogenetic handbook: a practical approach to DNA and protein phylogeny. Cambridge University Press, New York.
- Talbot, F. H. & Penrith, M. J. 1962. Spearing behavior in feeding in the black marlin, Istiompax marlina. Copeia, 1962, 468.
- Van Beneden, P. J. 1871. Recherches sur quelques poissons fossiles de Belgique. Bulletin de l’Académie Royale des Sciences, des Lettres et des Beaux-Arts de Belgique, 2e série, 31, 493–518.
- Van der Elst, R. P. & Roxbourg, M. 1981. Use of the bill during feeding in the black marlin (Makaira indica). Copeia, 1981, 215.
- Velez-Juarbe, J., Wood, A. R., De Gracia, C. & Hendy, A. J. W. 2015. Evolutionary patterns among living and fossil kogiid sperm whales: evidence from the Neogene of Central America. PLoS ONE, 10, e0123909. doi:10.1371/journal.pone.0123909
- Videler, J. J., Haydar, D., Snoek, R., Hoving, H.-J. T., Szabo, B. G., Snoek, O., Hoving, H.-J. T. & Szabo, B. G. 2016. Lubricating the swordfish head. Journal of Experimental Biology, 219, 1953–1956. doi:10.1242/jeb.139634
- Vigil, D. I. & Laurito, C. 2014. Nuevos restos de un odontoceti fósil (mammalia: Cetacea, Physeteroidea) para el Mioceno tardío de Panamá, América Central. Revista Geológica de América Central, 50, 213–217.
- Westneat, M. W. 2004. Evolution of levers and linkages in the feeding mechanisms of fishes. Integrative and Comparative Biology, 44, 378–389.
- Williams, S. M., McDowell, J. R., Bennett, M., Graves, J. E. & Ovenden, J. R. 2018. Analysis of whole mitochondrial genome sequences increases phylogenetic resolution of istiophorid billfishes. Bulletin of Marine Science, 94, 73–84.